Consent Manager Tag v2.0 (for TCF 2.0) -->
Farnell PDF
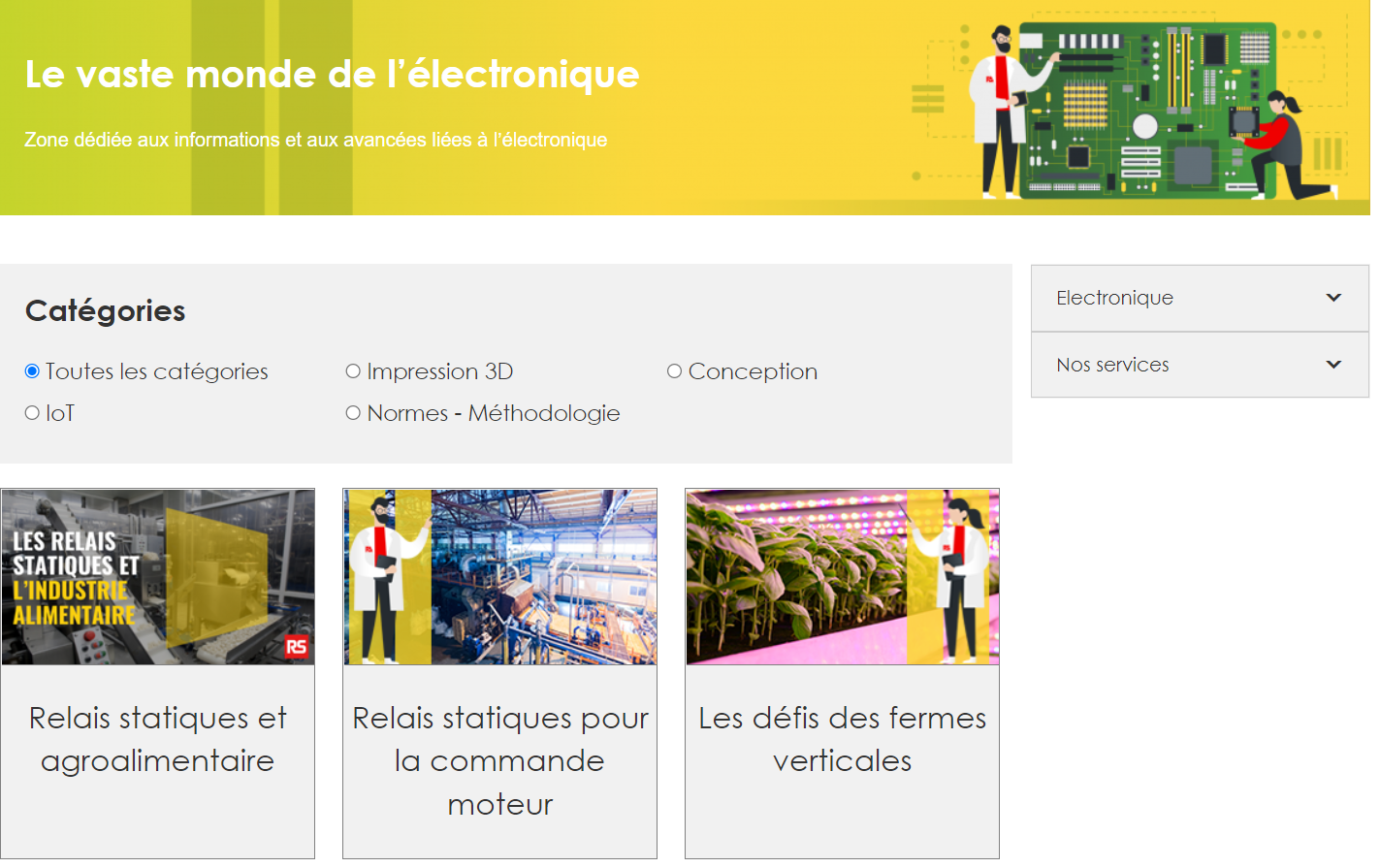
PIC18F2420/2520/4420/4520 Enhanced Flash ... - Farnell - Farnell Element 14
PIC18F2420/2520/4420/4520 Enhanced Flash ... - Farnell - Farnell Element 14
- Revenir à l'accueil

Farnell Element 14 :
Farnell-Full-Datashe..> 15-Jul-2014 17:08 951K
![[TXT]](http://www.audentia-gestion.fr/icons/text.gif)
Farnell-pmbta13_pmbt..> 15-Jul-2014 17:06 959K
![[TXT]](http://www.audentia-gestion.fr/icons/text.gif)
Farnell-EE-SPX303N-4..> 15-Jul-2014 17:06 969K
![[TXT]](http://www.audentia-gestion.fr/icons/text.gif)
Farnell-Datasheet-NX..> 15-Jul-2014 17:06 1.0M
![[TXT]](http://www.audentia-gestion.fr/icons/text.gif)
Farnell-Datasheet-Fa..> 15-Jul-2014 17:05 1.0M
![[TXT]](http://www.audentia-gestion.fr/icons/text.gif)
Farnell-MIDAS-un-tra..> 15-Jul-2014 17:05 1.0M
![[TXT]](http://www.audentia-gestion.fr/icons/text.gif)
Farnell-SERIAL-TFT-M..> 15-Jul-2014 17:05 1.0M
![[TXT]](http://www.audentia-gestion.fr/icons/text.gif)
Farnell-MCOC1-Farnel..> 15-Jul-2014 17:05 1.0M
![[TXT]](http://www.audentia-gestion.fr/icons/text.gif)
Farnell-TMR-2-series..> 15-Jul-2014 16:48 787K
![[TXT]](http://www.audentia-gestion.fr/icons/text.gif)
Farnell-DC-DC-Conver..> 15-Jul-2014 16:48 781K
![[TXT]](http://www.audentia-gestion.fr/icons/text.gif)
Farnell-Full-Datashe..> 15-Jul-2014 16:47 803K
![[TXT]](http://www.audentia-gestion.fr/icons/text.gif)
Farnell-TMLM-Series-..> 15-Jul-2014 16:47 810K
![[TXT]](http://www.audentia-gestion.fr/icons/text.gif)
Farnell-TEL-5-Series..> 15-Jul-2014 16:47 814K
![[TXT]](http://www.audentia-gestion.fr/icons/text.gif)
Farnell-TXL-series-t..> 15-Jul-2014 16:47 829K
![[TXT]](http://www.audentia-gestion.fr/icons/text.gif)
Farnell-TEP-150WI-Se..> 15-Jul-2014 16:47 837K
![[TXT]](http://www.audentia-gestion.fr/icons/text.gif)
Farnell-AC-DC-Power-..> 15-Jul-2014 16:47 845K
![[TXT]](http://www.audentia-gestion.fr/icons/text.gif)
Farnell-TIS-Instruct..> 15-Jul-2014 16:47 845K
![[TXT]](http://www.audentia-gestion.fr/icons/text.gif)
Farnell-TOS-tracopow..> 15-Jul-2014 16:47 852K
![[TXT]](http://www.audentia-gestion.fr/icons/text.gif)
Farnell-TCL-DC-traco..> 15-Jul-2014 16:46 858K
![[TXT]](http://www.audentia-gestion.fr/icons/text.gif)
Farnell-TIS-series-t..> 15-Jul-2014 16:46 875K
![[TXT]](http://www.audentia-gestion.fr/icons/text.gif)
Farnell-TMR-2-Series..> 15-Jul-2014 16:46 897K
![[TXT]](http://www.audentia-gestion.fr/icons/text.gif)
Farnell-TMR-3-WI-Ser..> 15-Jul-2014 16:46 939K
![[TXT]](http://www.audentia-gestion.fr/icons/text.gif)
Farnell-TEN-8-WI-Ser..> 15-Jul-2014 16:46 939K
![[TXT]](http://www.audentia-gestion.fr/icons/text.gif)
Farnell-Full-Datashe..> 15-Jul-2014 16:46 947K
Farnell-HIP4081A-Int..> 07-Jul-2014 19:47 1.0M
![[TXT]](http://www.audentia-gestion.fr/icons/text.gif)
Farnell-ISL6251-ISL6..> 07-Jul-2014 19:47 1.1M
![[TXT]](http://www.audentia-gestion.fr/icons/text.gif)
Farnell-DG411-DG412-..> 07-Jul-2014 19:47 1.0M
![[TXT]](http://www.audentia-gestion.fr/icons/text.gif)
Farnell-3367-ARALDIT..> 07-Jul-2014 19:46 1.2M
![[TXT]](http://www.audentia-gestion.fr/icons/text.gif)
Farnell-ICM7228-Inte..> 07-Jul-2014 19:46 1.1M
![[TXT]](http://www.audentia-gestion.fr/icons/text.gif)
Farnell-Data-Sheet-K..> 07-Jul-2014 19:46 1.2M
![[TXT]](http://www.audentia-gestion.fr/icons/text.gif)
Farnell-Silica-Gel-M..> 07-Jul-2014 19:46 1.2M
![[TXT]](http://www.audentia-gestion.fr/icons/text.gif)
Farnell-TKC2-Dusters..> 07-Jul-2014 19:46 1.2M
![[TXT]](http://www.audentia-gestion.fr/icons/text.gif)
Farnell-CRC-HANDCLEA..> 07-Jul-2014 19:46 1.2M
![[TXT]](http://www.audentia-gestion.fr/icons/text.gif)
Farnell-760G-French-..> 07-Jul-2014 19:45 1.2M
![[TXT]](http://www.audentia-gestion.fr/icons/text.gif)
Farnell-Decapant-KF-..> 07-Jul-2014 19:45 1.2M
![[TXT]](http://www.audentia-gestion.fr/icons/text.gif)
Farnell-1734-ARALDIT..> 07-Jul-2014 19:45 1.2M
![[TXT]](http://www.audentia-gestion.fr/icons/text.gif)
Farnell-Araldite-Fus..> 07-Jul-2014 19:45 1.2M
![[TXT]](http://www.audentia-gestion.fr/icons/text.gif)
Farnell-fiche-de-don..> 07-Jul-2014 19:44 1.4M
![[TXT]](http://www.audentia-gestion.fr/icons/text.gif)
Farnell-safety-data-..> 07-Jul-2014 19:44 1.4M
![[TXT]](http://www.audentia-gestion.fr/icons/text.gif)
Farnell-A-4-Hardener..> 07-Jul-2014 19:44 1.4M
![[TXT]](http://www.audentia-gestion.fr/icons/text.gif)
Farnell-CC-Debugger-..> 07-Jul-2014 19:44 1.5M
![[TXT]](http://www.audentia-gestion.fr/icons/text.gif)
Farnell-MSP430-Hardw..> 07-Jul-2014 19:43 1.8M
![[TXT]](http://www.audentia-gestion.fr/icons/text.gif)
Farnell-SmartRF06-Ev..> 07-Jul-2014 19:43 1.6M
![[TXT]](http://www.audentia-gestion.fr/icons/text.gif)
Farnell-CC2531-USB-H..> 07-Jul-2014 19:43 1.8M
![[TXT]](http://www.audentia-gestion.fr/icons/text.gif)
Farnell-Alimentation..> 07-Jul-2014 19:43 1.8M
![[TXT]](http://www.audentia-gestion.fr/icons/text.gif)
Farnell-BK889B-PONT-..> 07-Jul-2014 19:42 1.8M
![[TXT]](http://www.audentia-gestion.fr/icons/text.gif)
Farnell-User-Guide-M..> 07-Jul-2014 19:41 2.0M
![[TXT]](http://www.audentia-gestion.fr/icons/text.gif)
Farnell-T672-3000-Se..> 07-Jul-2014 19:41 2.0M
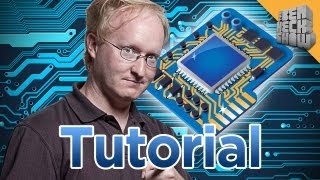
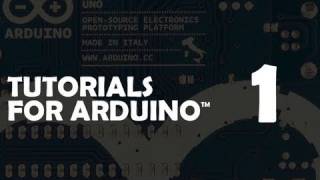
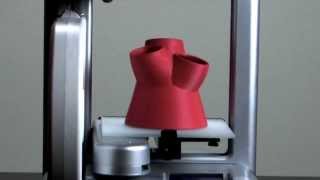
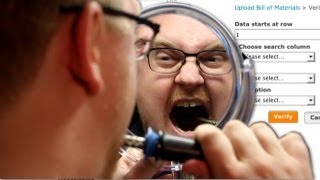
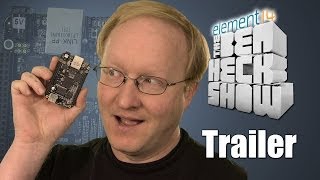
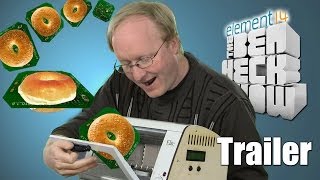
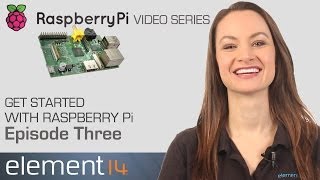
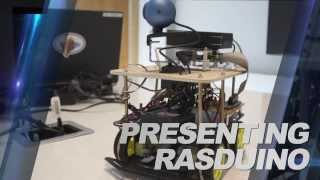
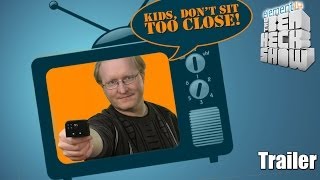
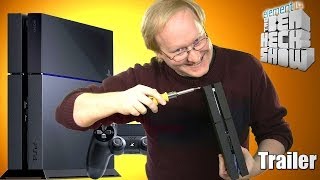
See the trailer for the next exciting episode of The Ben Heck show. Check back on Friday to be among the first to see the exclusive full show on element…
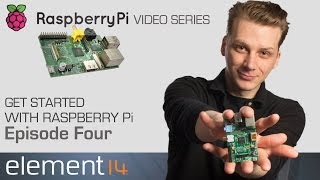
Connect your Raspberry Pi to a breadboard, download some code and create a push-button audio play project.
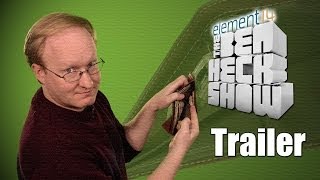
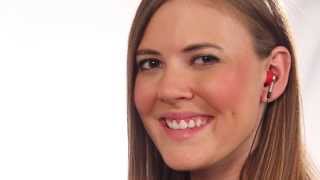
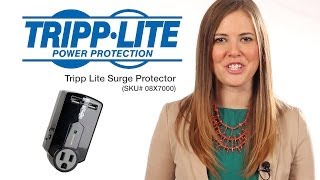
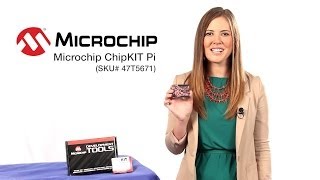
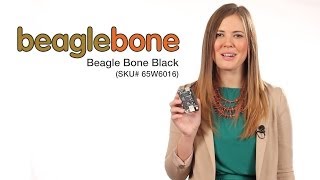
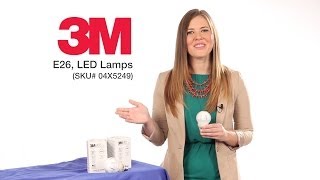
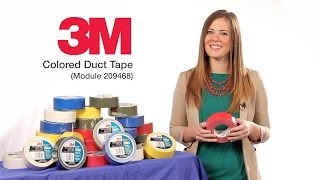
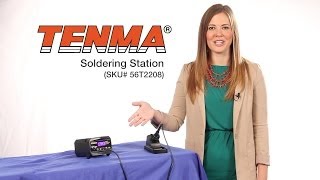
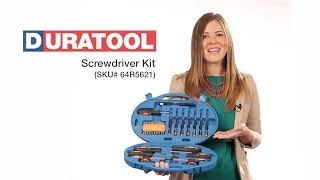
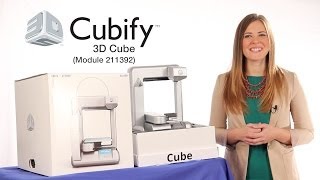
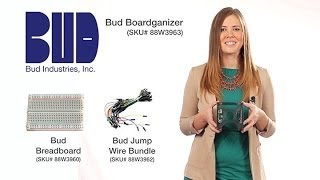
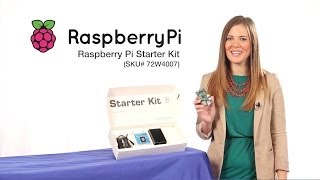
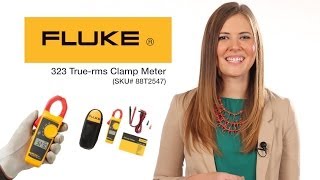
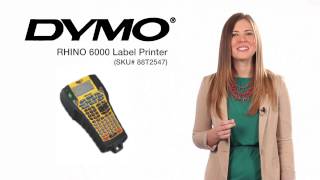
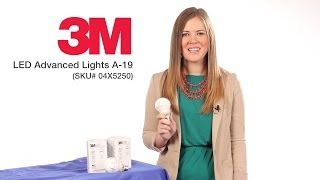
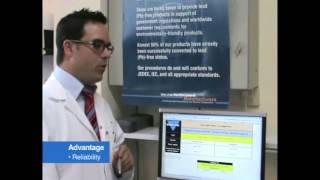
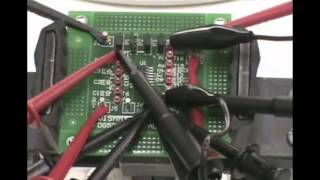
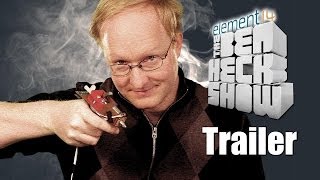
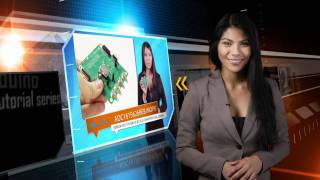
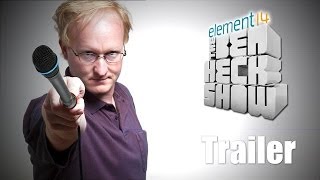
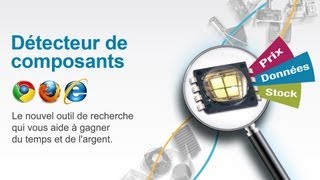
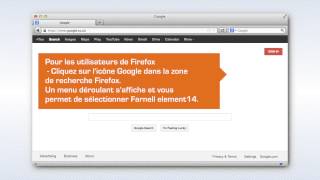
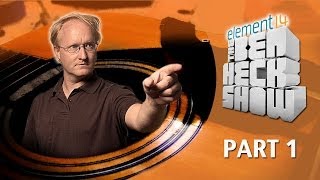
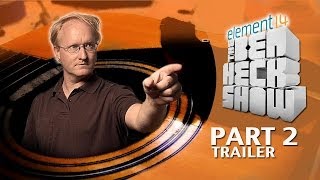
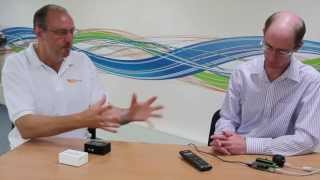
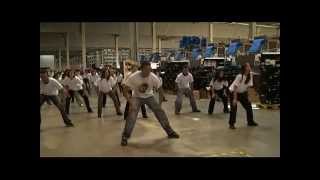
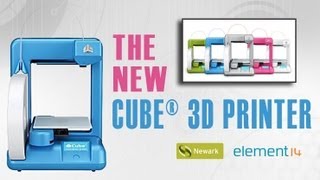










Puce électronique / Microchip :
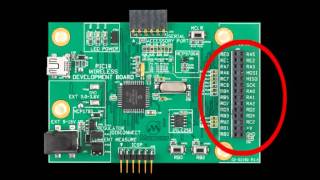
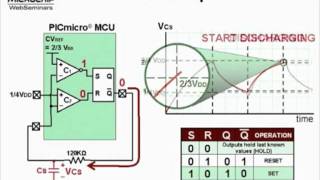
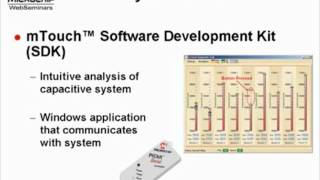
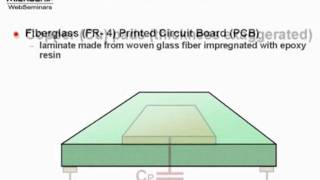
Sans fil - Wireless :
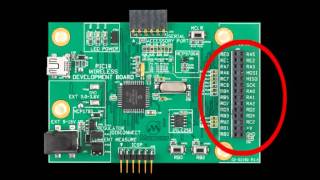
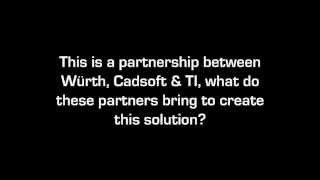
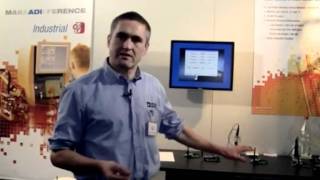
Texas instrument :
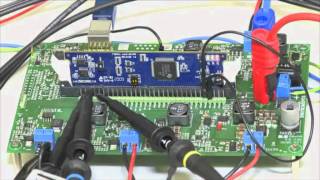
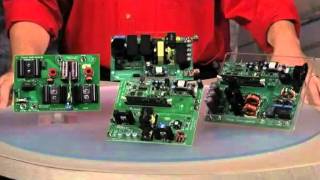
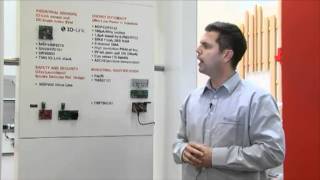
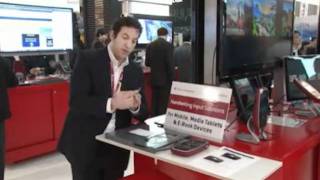
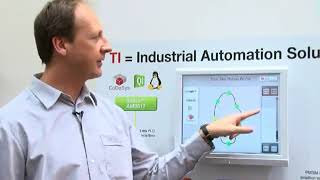
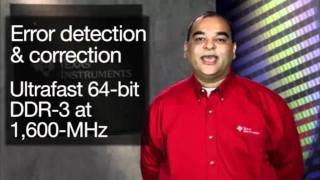
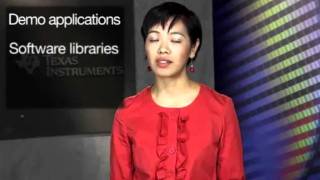
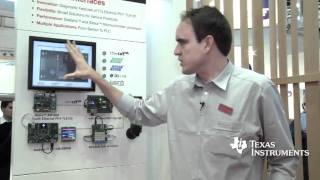
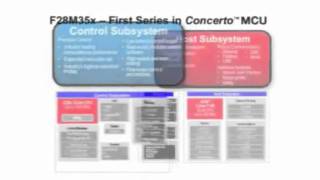
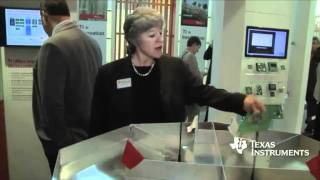
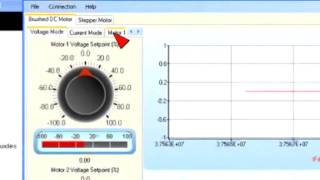
Ordinateurs :
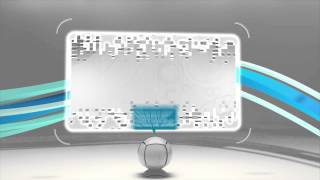
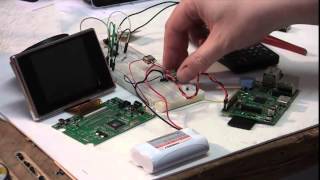
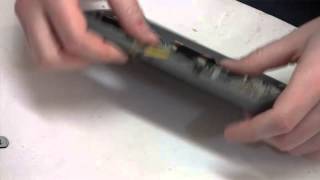
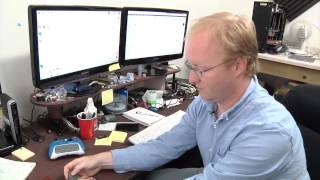
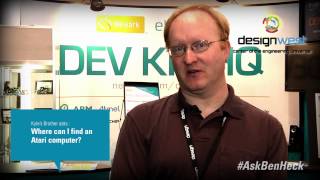
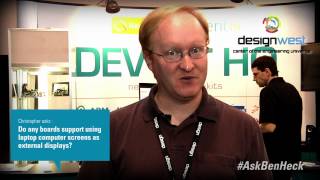
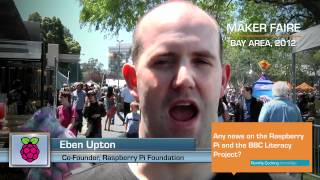
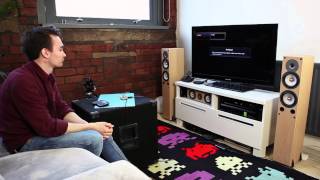
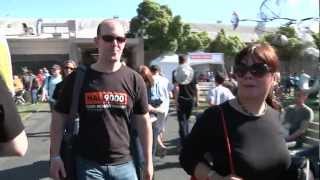
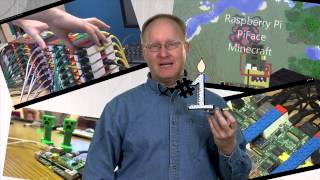
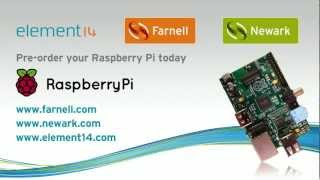
Logiciels :
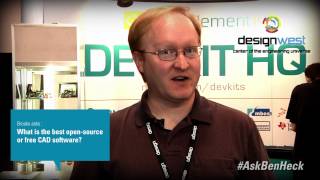
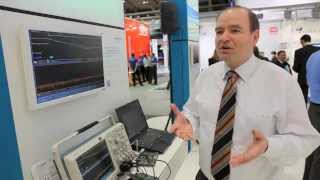
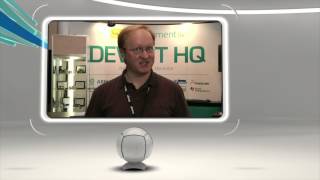
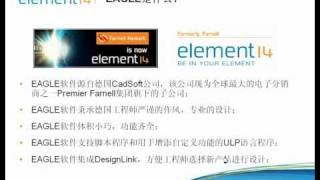
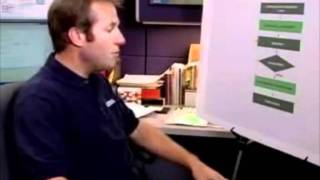
Tutoriels :
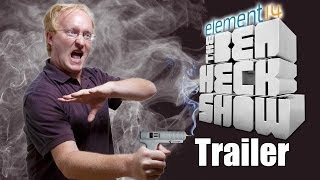
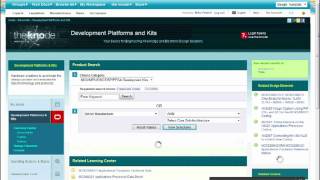
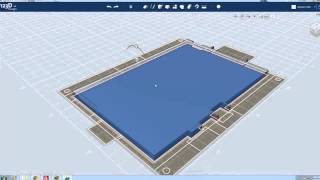
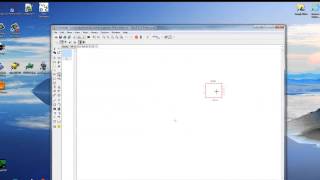
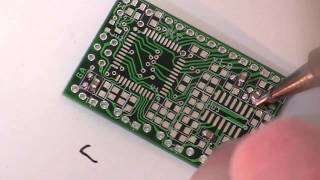
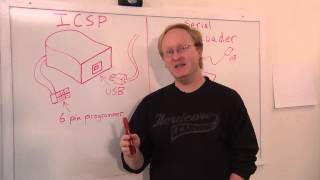
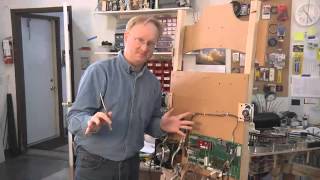
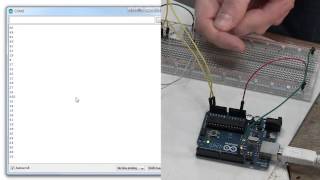
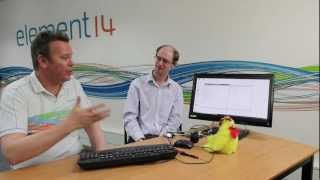
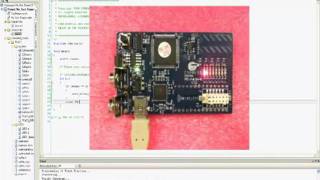
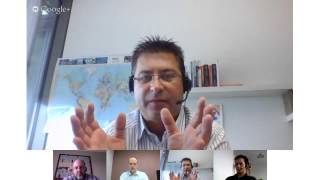
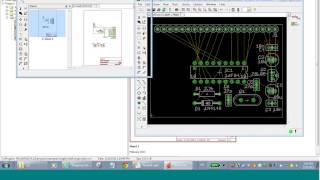
Autres documentations :
![[TXT]](http://www.audentia-gestion.fr/icons/text.gif)
Farnell-MCOC1-Farnel..> 16-Jul-2014 09:04 1.0M
![[TXT]](http://www.audentia-gestion.fr/icons/text.gif)
Farnell-SL3S1203_121..> 16-Jul-2014 09:04 1.1M
![[TXT]](http://www.audentia-gestion.fr/icons/text.gif)
Farnell-PN512-Full-N..> 16-Jul-2014 09:03 1.4M
![[TXT]](http://www.audentia-gestion.fr/icons/text.gif)
Farnell-SL3S4011_402..> 16-Jul-2014 09:03 1.1M
![[TXT]](http://www.audentia-gestion.fr/icons/text.gif)
Farnell-LPC408x-7x 3..> 16-Jul-2014 09:03 1.6M
![[TXT]](http://www.audentia-gestion.fr/icons/text.gif)
Farnell-PCF8574-PCF8..> 16-Jul-2014 09:03 1.7M
![[TXT]](http://www.audentia-gestion.fr/icons/text.gif)
Farnell-LPC81xM-32-b..> 16-Jul-2014 09:02 2.0M
![[TXT]](http://www.audentia-gestion.fr/icons/text.gif)
Farnell-LPC1769-68-6..> 16-Jul-2014 09:02 1.9M
![[TXT]](http://www.audentia-gestion.fr/icons/text.gif)
Farnell-Download-dat..> 16-Jul-2014 09:02 2.2M
![[TXT]](http://www.audentia-gestion.fr/icons/text.gif)
Farnell-LPC3220-30-4..> 16-Jul-2014 09:02 2.2M
![[TXT]](http://www.audentia-gestion.fr/icons/text.gif)
Farnell-LPC11U3x-32-..> 16-Jul-2014 09:01 2.4M
![[TXT]](http://www.audentia-gestion.fr/icons/text.gif)
Farnell-SL3ICS1002-1..> 16-Jul-2014 09:01 2.5M
![[TXT]](http://www.audentia-gestion.fr/icons/text.gif)
Farnell-T672-3000-Se..> 08-Jul-2014 18:59 2.0M
![[TXT]](http://www.audentia-gestion.fr/icons/text.gif)
Farnell-tesa®pack63..> 08-Jul-2014 18:56 2.0M
![[TXT]](http://www.audentia-gestion.fr/icons/text.gif)
Farnell-Encodeur-USB..> 08-Jul-2014 18:56 2.0M
![[TXT]](http://www.audentia-gestion.fr/icons/text.gif)
Farnell-CC2530ZDK-Us..> 08-Jul-2014 18:55 2.1M
![[TXT]](http://www.audentia-gestion.fr/icons/text.gif)
Farnell-2020-Manuel-..> 08-Jul-2014 18:55 2.1M
![[TXT]](http://www.audentia-gestion.fr/icons/text.gif)
Farnell-Synchronous-..> 08-Jul-2014 18:54 2.1M
![[TXT]](http://www.audentia-gestion.fr/icons/text.gif)
Farnell-Arithmetic-L..> 08-Jul-2014 18:54 2.1M
![[TXT]](http://www.audentia-gestion.fr/icons/text.gif)
Farnell-NA555-NE555-..> 08-Jul-2014 18:53 2.2M
![[TXT]](http://www.audentia-gestion.fr/icons/text.gif)
Farnell-4-Bit-Magnit..> 08-Jul-2014 18:53 2.2M
![[TXT]](http://www.audentia-gestion.fr/icons/text.gif)
Farnell-LM555-Timer-..> 08-Jul-2014 18:53 2.2M
![[TXT]](http://www.audentia-gestion.fr/icons/text.gif)
Farnell-L293d-Texas-..> 08-Jul-2014 18:53 2.2M
![[TXT]](http://www.audentia-gestion.fr/icons/text.gif)
Farnell-SN54HC244-SN..> 08-Jul-2014 18:52 2.3M
![[TXT]](http://www.audentia-gestion.fr/icons/text.gif)
Farnell-MAX232-MAX23..> 08-Jul-2014 18:52 2.3M
![[TXT]](http://www.audentia-gestion.fr/icons/text.gif)
Farnell-High-precisi..> 08-Jul-2014 18:51 2.3M
![[TXT]](http://www.audentia-gestion.fr/icons/text.gif)
Farnell-SMU-Instrume..> 08-Jul-2014 18:51 2.3M
![[TXT]](http://www.audentia-gestion.fr/icons/text.gif)
Farnell-900-Series-B..> 08-Jul-2014 18:50 2.3M
![[TXT]](http://www.audentia-gestion.fr/icons/text.gif)
Farnell-BA-Series-Oh..> 08-Jul-2014 18:50 2.3M
![[TXT]](http://www.audentia-gestion.fr/icons/text.gif)
Farnell-UTS-Series-S..> 08-Jul-2014 18:49 2.5M
![[TXT]](http://www.audentia-gestion.fr/icons/text.gif)
Farnell-270-Series-O..> 08-Jul-2014 18:49 2.3M
![[TXT]](http://www.audentia-gestion.fr/icons/text.gif)
Farnell-UTS-Series-S..> 08-Jul-2014 18:49 2.8M
![[TXT]](http://www.audentia-gestion.fr/icons/text.gif)
Farnell-Tiva-C-Serie..> 08-Jul-2014 18:49 2.6M
![[TXT]](http://www.audentia-gestion.fr/icons/text.gif)
Farnell-UTO-Souriau-..> 08-Jul-2014 18:48 2.8M
![[TXT]](http://www.audentia-gestion.fr/icons/text.gif)
Farnell-Clipper-Seri..> 08-Jul-2014 18:48 2.8M
![[TXT]](http://www.audentia-gestion.fr/icons/text.gif)
Farnell-SOURIAU-Cont..> 08-Jul-2014 18:47 3.0M
![[TXT]](http://www.audentia-gestion.fr/icons/text.gif)
Farnell-851-Series-P..> 08-Jul-2014 18:47 3.0M
Farnell-SL59830-Inte..> 06-Jul-2014 10:07 1.0M
![[TXT]](http://www.audentia-gestion.fr/icons/text.gif)
Farnell-ALF1210-PDF.htm 06-Jul-2014 10:06 4.0M
![[TXT]](http://www.audentia-gestion.fr/icons/text.gif)
Farnell-AD7171-16-Bi..> 06-Jul-2014 10:06 1.0M
![[TXT]](http://www.audentia-gestion.fr/icons/text.gif)
Farnell-Low-Noise-24..> 06-Jul-2014 10:05 1.0M
![[TXT]](http://www.audentia-gestion.fr/icons/text.gif)
Farnell-ESCON-Featur..> 06-Jul-2014 10:05 938K
![[TXT]](http://www.audentia-gestion.fr/icons/text.gif)
Farnell-74LCX573-Fai..> 06-Jul-2014 10:05 1.9M
![[TXT]](http://www.audentia-gestion.fr/icons/text.gif)
Farnell-1N4148WS-Fai..> 06-Jul-2014 10:04 1.9M
![[TXT]](http://www.audentia-gestion.fr/icons/text.gif)
Farnell-FAN6756-Fair..> 06-Jul-2014 10:04 850K
![[TXT]](http://www.audentia-gestion.fr/icons/text.gif)
Farnell-Datasheet-Fa..> 06-Jul-2014 10:04 861K
![[TXT]](http://www.audentia-gestion.fr/icons/text.gif)
Farnell-ES1F-ES1J-fi..> 06-Jul-2014 10:04 867K
![[TXT]](http://www.audentia-gestion.fr/icons/text.gif)
Farnell-QRE1113-Fair..> 06-Jul-2014 10:03 879K
![[TXT]](http://www.audentia-gestion.fr/icons/text.gif)
Farnell-2N7002DW-Fai..> 06-Jul-2014 10:03 886K
![[TXT]](http://www.audentia-gestion.fr/icons/text.gif)
Farnell-FDC2512-Fair..> 06-Jul-2014 10:03 886K
![[TXT]](http://www.audentia-gestion.fr/icons/text.gif)
Farnell-FDV301N-Digi..> 06-Jul-2014 10:03 886K
![[TXT]](http://www.audentia-gestion.fr/icons/text.gif)
Farnell-S1A-Fairchil..> 06-Jul-2014 10:03 896K
![[TXT]](http://www.audentia-gestion.fr/icons/text.gif)
Farnell-BAV99-Fairch..> 06-Jul-2014 10:03 896K
![[TXT]](http://www.audentia-gestion.fr/icons/text.gif)
Farnell-74AC00-74ACT..> 06-Jul-2014 10:03 911K
![[TXT]](http://www.audentia-gestion.fr/icons/text.gif)
Farnell-NaPiOn-Panas..> 06-Jul-2014 10:02 911K
![[TXT]](http://www.audentia-gestion.fr/icons/text.gif)
Farnell-LQ-RELAYS-AL..> 06-Jul-2014 10:02 924K
![[TXT]](http://www.audentia-gestion.fr/icons/text.gif)
Farnell-ev-relays-ae..> 06-Jul-2014 10:02 926K
![[TXT]](http://www.audentia-gestion.fr/icons/text.gif)
Farnell-ESCON-Featur..> 06-Jul-2014 10:02 931K
![[TXT]](http://www.audentia-gestion.fr/icons/text.gif)
Farnell-Amplifier-In..> 06-Jul-2014 10:02 940K
![[TXT]](http://www.audentia-gestion.fr/icons/text.gif)
Farnell-Serial-File-..> 06-Jul-2014 10:02 941K
![[TXT]](http://www.audentia-gestion.fr/icons/text.gif)
Farnell-Both-the-Del..> 06-Jul-2014 10:01 948K
![[TXT]](http://www.audentia-gestion.fr/icons/text.gif)
Farnell-Videk-PDF.htm 06-Jul-2014 10:01 948K
![[TXT]](http://www.audentia-gestion.fr/icons/text.gif)
Farnell-EPCOS-173438..> 04-Jul-2014 10:43 3.3M
![[TXT]](http://www.audentia-gestion.fr/icons/text.gif)
Farnell-Sensorless-C..> 04-Jul-2014 10:42 3.3M
![[TXT]](http://www.audentia-gestion.fr/icons/text.gif)
Farnell-197.31-KB-Te..> 04-Jul-2014 10:42 3.3M
![[TXT]](http://www.audentia-gestion.fr/icons/text.gif)
Farnell-PIC12F609-61..> 04-Jul-2014 10:41 3.7M
![[TXT]](http://www.audentia-gestion.fr/icons/text.gif)
Farnell-PADO-semi-au..> 04-Jul-2014 10:41 3.7M
![[TXT]](http://www.audentia-gestion.fr/icons/text.gif)
Farnell-03-iec-runds..> 04-Jul-2014 10:40 3.7M
![[TXT]](http://www.audentia-gestion.fr/icons/text.gif)
Farnell-ACC-Silicone..> 04-Jul-2014 10:40 3.7M
![[TXT]](http://www.audentia-gestion.fr/icons/text.gif)
Farnell-Series-TDS10..> 04-Jul-2014 10:39 4.0M
![[TXT]](http://www.audentia-gestion.fr/icons/text.gif)
Farnell-03-iec-runds..> 04-Jul-2014 10:40 3.7M
![[TXT]](http://www.audentia-gestion.fr/icons/text.gif)
Farnell-0430300011-D..> 14-Jun-2014 18:13 2.0M
![[TXT]](http://www.audentia-gestion.fr/icons/text.gif)
Farnell-06-6544-8-PD..> 26-Mar-2014 17:56 2.7M
![[TXT]](http://www.audentia-gestion.fr/icons/text.gif)
Farnell-3M-Polyimide..> 21-Mar-2014 08:09 3.9M
![[TXT]](http://www.audentia-gestion.fr/icons/text.gif)
Farnell-3M-VolitionT..> 25-Mar-2014 08:18 3.3M
![[TXT]](http://www.audentia-gestion.fr/icons/text.gif)
Farnell-10BQ060-PDF.htm 14-Jun-2014 09:50 2.4M
![[TXT]](http://www.audentia-gestion.fr/icons/text.gif)
Farnell-10TPB47M-End..> 14-Jun-2014 18:16 3.4M
![[TXT]](http://www.audentia-gestion.fr/icons/text.gif)
Farnell-12mm-Size-In..> 14-Jun-2014 09:50 2.4M
![[TXT]](http://www.audentia-gestion.fr/icons/text.gif)
Farnell-24AA024-24LC..> 23-Jun-2014 10:26 3.1M
![[TXT]](http://www.audentia-gestion.fr/icons/text.gif)
Farnell-50A-High-Pow..> 20-Mar-2014 17:31 2.9M
![[TXT]](http://www.audentia-gestion.fr/icons/text.gif)
Farnell-197.31-KB-Te..> 04-Jul-2014 10:42 3.3M
![[TXT]](http://www.audentia-gestion.fr/icons/text.gif)
Farnell-1907-2006-PD..> 26-Mar-2014 17:56 2.7M
![[TXT]](http://www.audentia-gestion.fr/icons/text.gif)
Farnell-5910-PDF.htm 25-Mar-2014 08:15 3.0M
![[TXT]](http://www.audentia-gestion.fr/icons/text.gif)
Farnell-6517b-Electr..> 29-Mar-2014 11:12 3.3M
![[TXT]](http://www.audentia-gestion.fr/icons/text.gif)
Farnell-A-True-Syste..> 29-Mar-2014 11:13 3.3M
![[TXT]](http://www.audentia-gestion.fr/icons/text.gif)
Farnell-ACC-Silicone..> 04-Jul-2014 10:40 3.7M
![[TXT]](http://www.audentia-gestion.fr/icons/text.gif)
Farnell-AD524-PDF.htm 20-Mar-2014 17:33 2.8M
![[TXT]](http://www.audentia-gestion.fr/icons/text.gif)
Farnell-ADL6507-PDF.htm 14-Jun-2014 18:19 3.4M
![[TXT]](http://www.audentia-gestion.fr/icons/text.gif)
Farnell-ADSP-21362-A..> 20-Mar-2014 17:34 2.8M
![[TXT]](http://www.audentia-gestion.fr/icons/text.gif)
Farnell-ALF1210-PDF.htm 04-Jul-2014 10:39 4.0M
![[TXT]](http://www.audentia-gestion.fr/icons/text.gif)
Farnell-ALF1225-12-V..> 01-Apr-2014 07:40 3.4M
![[TXT]](http://www.audentia-gestion.fr/icons/text.gif)
Farnell-ALF2412-24-V..> 01-Apr-2014 07:39 3.4M
![[TXT]](http://www.audentia-gestion.fr/icons/text.gif)
Farnell-AN10361-Phil..> 23-Jun-2014 10:29 2.1M
![[TXT]](http://www.audentia-gestion.fr/icons/text.gif)
Farnell-ARADUR-HY-13..> 26-Mar-2014 17:55 2.8M
![[TXT]](http://www.audentia-gestion.fr/icons/text.gif)
Farnell-ARALDITE-201..> 21-Mar-2014 08:12 3.7M
![[TXT]](http://www.audentia-gestion.fr/icons/text.gif)
Farnell-ARALDITE-CW-..> 26-Mar-2014 17:56 2.7M
![[TXT]](http://www.audentia-gestion.fr/icons/text.gif)
Farnell-ATMEL-8-bit-..> 19-Mar-2014 18:04 2.1M
![[TXT]](http://www.audentia-gestion.fr/icons/text.gif)
Farnell-ATMEL-8-bit-..> 11-Mar-2014 07:55 2.1M
![[TXT]](http://www.audentia-gestion.fr/icons/text.gif)
Farnell-ATmega640-VA..> 14-Jun-2014 09:49 2.5M
![[TXT]](http://www.audentia-gestion.fr/icons/text.gif)
Farnell-ATtiny20-PDF..> 25-Mar-2014 08:19 3.6M
![[TXT]](http://www.audentia-gestion.fr/icons/text.gif)
Farnell-ATtiny26-L-A..> 13-Jun-2014 18:40 1.8M
![[TXT]](http://www.audentia-gestion.fr/icons/text.gif)
Farnell-Alimentation..> 14-Jun-2014 18:24 2.5M
![[TXT]](http://www.audentia-gestion.fr/icons/text.gif)
Farnell-Alimentation..> 01-Apr-2014 07:42 3.4M
![[TXT]](http://www.audentia-gestion.fr/icons/text.gif)
Farnell-Amplificateu..> 29-Mar-2014 11:11 3.3M
![[TXT]](http://www.audentia-gestion.fr/icons/text.gif)
Farnell-An-Improved-..> 14-Jun-2014 09:49 2.5M
![[TXT]](http://www.audentia-gestion.fr/icons/text.gif)
Farnell-Atmel-ATmega..> 19-Mar-2014 18:03 2.2M
![[TXT]](http://www.audentia-gestion.fr/icons/text.gif)
Farnell-Avvertenze-e..> 14-Jun-2014 18:20 3.3M
![[TXT]](http://www.audentia-gestion.fr/icons/text.gif)
Farnell-BC846DS-NXP-..> 13-Jun-2014 18:42 1.6M
![[TXT]](http://www.audentia-gestion.fr/icons/text.gif)
Farnell-BC847DS-NXP-..> 23-Jun-2014 10:24 3.3M
![[TXT]](http://www.audentia-gestion.fr/icons/text.gif)
Farnell-BF545A-BF545..> 23-Jun-2014 10:28 2.1M
![[TXT]](http://www.audentia-gestion.fr/icons/text.gif)
Farnell-BK2650A-BK26..> 29-Mar-2014 11:10 3.3M
![[TXT]](http://www.audentia-gestion.fr/icons/text.gif)
Farnell-BT151-650R-N..> 13-Jun-2014 18:40 1.7M
![[TXT]](http://www.audentia-gestion.fr/icons/text.gif)
Farnell-BTA204-800C-..> 13-Jun-2014 18:42 1.6M
![[TXT]](http://www.audentia-gestion.fr/icons/text.gif)
Farnell-BUJD203AX-NX..> 13-Jun-2014 18:41 1.7M
![[TXT]](http://www.audentia-gestion.fr/icons/text.gif)
Farnell-BYV29F-600-N..> 13-Jun-2014 18:42 1.6M
![[TXT]](http://www.audentia-gestion.fr/icons/text.gif)
Farnell-BYV79E-serie..> 10-Mar-2014 16:19 1.6M
![[TXT]](http://www.audentia-gestion.fr/icons/text.gif)
Farnell-BZX384-serie..> 23-Jun-2014 10:29 2.1M
![[TXT]](http://www.audentia-gestion.fr/icons/text.gif)
Farnell-Battery-GBA-..> 14-Jun-2014 18:13 2.0M
![[TXT]](http://www.audentia-gestion.fr/icons/text.gif)
Farnell-C.A-6150-C.A..> 14-Jun-2014 18:24 2.5M
![[TXT]](http://www.audentia-gestion.fr/icons/text.gif)
Farnell-C.A 8332B-C...> 01-Apr-2014 07:40 3.4M
![[TXT]](http://www.audentia-gestion.fr/icons/text.gif)
Farnell-CC2560-Bluet..> 29-Mar-2014 11:14 2.8M
![[TXT]](http://www.audentia-gestion.fr/icons/text.gif)
Farnell-CD4536B-Type..> 14-Jun-2014 18:13 2.0M
![[TXT]](http://www.audentia-gestion.fr/icons/text.gif)
Farnell-CIRRUS-LOGIC..> 10-Mar-2014 17:20 2.1M
![[TXT]](http://www.audentia-gestion.fr/icons/text.gif)
Farnell-CS5532-34-BS..> 01-Apr-2014 07:39 3.5M
![[TXT]](http://www.audentia-gestion.fr/icons/text.gif)
Farnell-Cannon-ZD-PD..> 11-Mar-2014 08:13 2.8M
![[TXT]](http://www.audentia-gestion.fr/icons/text.gif)
Farnell-Ceramic-tran..> 14-Jun-2014 18:19 3.4M
![[TXT]](http://www.audentia-gestion.fr/icons/text.gif)
Farnell-Circuit-Note..> 26-Mar-2014 18:00 2.8M
![[TXT]](http://www.audentia-gestion.fr/icons/text.gif)
Farnell-Circuit-Note..> 26-Mar-2014 18:00 2.8M
![[TXT]](http://www.audentia-gestion.fr/icons/text.gif)
Farnell-Cles-electro..> 21-Mar-2014 08:13 3.9M
![[TXT]](http://www.audentia-gestion.fr/icons/text.gif)
Farnell-Conception-d..> 11-Mar-2014 07:49 2.4M
![[TXT]](http://www.audentia-gestion.fr/icons/text.gif)
Farnell-Connectors-N..> 14-Jun-2014 18:12 2.1M
![[TXT]](http://www.audentia-gestion.fr/icons/text.gif)
Farnell-Construction..> 14-Jun-2014 18:25 2.5M
![[TXT]](http://www.audentia-gestion.fr/icons/text.gif)
Farnell-Controle-de-..> 11-Mar-2014 08:16 2.8M
![[TXT]](http://www.audentia-gestion.fr/icons/text.gif)
Farnell-Cordless-dri..> 14-Jun-2014 18:13 2.0M
![[TXT]](http://www.audentia-gestion.fr/icons/text.gif)
Farnell-Current-Tran..> 26-Mar-2014 17:58 2.7M
![[TXT]](http://www.audentia-gestion.fr/icons/text.gif)
Farnell-Current-Tran..> 26-Mar-2014 17:58 2.7M
![[TXT]](http://www.audentia-gestion.fr/icons/text.gif)
Farnell-Current-Tran..> 26-Mar-2014 17:59 2.7M
![[TXT]](http://www.audentia-gestion.fr/icons/text.gif)
Farnell-Current-Tran..> 26-Mar-2014 17:59 2.7M
![[TXT]](http://www.audentia-gestion.fr/icons/text.gif)
Farnell-DC-Fan-type-..> 14-Jun-2014 09:48 2.5M
![[TXT]](http://www.audentia-gestion.fr/icons/text.gif)
Farnell-DC-Fan-type-..> 14-Jun-2014 09:51 1.8M
![[TXT]](http://www.audentia-gestion.fr/icons/text.gif)
Farnell-Davum-TMC-PD..> 14-Jun-2014 18:27 2.4M
![[TXT]](http://www.audentia-gestion.fr/icons/text.gif)
Farnell-De-la-puissa..> 29-Mar-2014 11:10 3.3M
![[TXT]](http://www.audentia-gestion.fr/icons/text.gif)
Farnell-Directive-re..> 25-Mar-2014 08:16 3.0M
![[TXT]](http://www.audentia-gestion.fr/icons/text.gif)
Farnell-Documentatio..> 14-Jun-2014 18:26 2.5M
![[TXT]](http://www.audentia-gestion.fr/icons/text.gif)
Farnell-Download-dat..> 13-Jun-2014 18:40 1.8M
![[TXT]](http://www.audentia-gestion.fr/icons/text.gif)
Farnell-ECO-Series-T..> 20-Mar-2014 08:14 2.5M
![[TXT]](http://www.audentia-gestion.fr/icons/text.gif)
Farnell-ELMA-PDF.htm 29-Mar-2014 11:13 3.3M
![[TXT]](http://www.audentia-gestion.fr/icons/text.gif)
Farnell-EMC1182-PDF.htm 25-Mar-2014 08:17 3.0M
![[TXT]](http://www.audentia-gestion.fr/icons/text.gif)
Farnell-EPCOS-173438..> 04-Jul-2014 10:43 3.3M
![[TXT]](http://www.audentia-gestion.fr/icons/text.gif)
Farnell-EPCOS-Sample..> 11-Mar-2014 07:53 2.2M
![[TXT]](http://www.audentia-gestion.fr/icons/text.gif)
Farnell-ES2333-PDF.htm 11-Mar-2014 08:14 2.8M
![[TXT]](http://www.audentia-gestion.fr/icons/text.gif)
Farnell-Ed.081002-DA..> 19-Mar-2014 18:02 2.5M
![[TXT]](http://www.audentia-gestion.fr/icons/text.gif)
Farnell-F28069-Picco..> 14-Jun-2014 18:14 2.0M
![[TXT]](http://www.audentia-gestion.fr/icons/text.gif)
Farnell-F42202-PDF.htm 19-Mar-2014 18:00 2.5M
![[TXT]](http://www.audentia-gestion.fr/icons/text.gif)
Farnell-FDS-ITW-Spra..> 14-Jun-2014 18:22 3.3M
![[TXT]](http://www.audentia-gestion.fr/icons/text.gif)
Farnell-FICHE-DE-DON..> 10-Mar-2014 16:17 1.6M
![[TXT]](http://www.audentia-gestion.fr/icons/text.gif)
Farnell-Fastrack-Sup..> 23-Jun-2014 10:25 3.3M
![[TXT]](http://www.audentia-gestion.fr/icons/text.gif)
Farnell-Ferric-Chlor..> 29-Mar-2014 11:14 2.8M
![[TXT]](http://www.audentia-gestion.fr/icons/text.gif)
Farnell-Fiche-de-don..> 14-Jun-2014 09:47 2.5M
![[TXT]](http://www.audentia-gestion.fr/icons/text.gif)
Farnell-Fiche-de-don..> 14-Jun-2014 18:26 2.5M
![[TXT]](http://www.audentia-gestion.fr/icons/text.gif)
Farnell-Fluke-1730-E..> 14-Jun-2014 18:23 2.5M
![[TXT]](http://www.audentia-gestion.fr/icons/text.gif)
Farnell-GALVA-A-FROI..> 26-Mar-2014 17:56 2.7M
![[TXT]](http://www.audentia-gestion.fr/icons/text.gif)
Farnell-GALVA-MAT-Re..> 26-Mar-2014 17:57 2.7M
![[TXT]](http://www.audentia-gestion.fr/icons/text.gif)
Farnell-GN-RELAYS-AG..> 20-Mar-2014 08:11 2.6M
![[TXT]](http://www.audentia-gestion.fr/icons/text.gif)
Farnell-HC49-4H-Crys..> 14-Jun-2014 18:20 3.3M
![[TXT]](http://www.audentia-gestion.fr/icons/text.gif)
Farnell-HFE1600-Data..> 14-Jun-2014 18:22 3.3M
![[TXT]](http://www.audentia-gestion.fr/icons/text.gif)
Farnell-HI-70300-Sol..> 14-Jun-2014 18:27 2.4M
![[TXT]](http://www.audentia-gestion.fr/icons/text.gif)
Farnell-HUNTSMAN-Adv..> 10-Mar-2014 16:17 1.7M
![[TXT]](http://www.audentia-gestion.fr/icons/text.gif)
Farnell-Haute-vitess..> 11-Mar-2014 08:17 2.4M
![[TXT]](http://www.audentia-gestion.fr/icons/text.gif)
Farnell-IP4252CZ16-8..> 13-Jun-2014 18:41 1.7M
![[TXT]](http://www.audentia-gestion.fr/icons/text.gif)
Farnell-Instructions..> 19-Mar-2014 18:01 2.5M
![[TXT]](http://www.audentia-gestion.fr/icons/text.gif)
Farnell-KSZ8851SNL-S..> 23-Jun-2014 10:28 2.1M
![[TXT]](http://www.audentia-gestion.fr/icons/text.gif)
Farnell-L-efficacite..> 11-Mar-2014 07:52 2.3M
![[TXT]](http://www.audentia-gestion.fr/icons/text.gif)
Farnell-LCW-CQ7P.CC-..> 25-Mar-2014 08:19 3.2M
![[TXT]](http://www.audentia-gestion.fr/icons/text.gif)
Farnell-LME49725-Pow..> 14-Jun-2014 09:49 2.5M
![[TXT]](http://www.audentia-gestion.fr/icons/text.gif)
Farnell-LOCTITE-542-..> 25-Mar-2014 08:15 3.0M
![[TXT]](http://www.audentia-gestion.fr/icons/text.gif)
Farnell-LOCTITE-3463..> 25-Mar-2014 08:19 3.0M
![[TXT]](http://www.audentia-gestion.fr/icons/text.gif)
Farnell-LUXEON-Guide..> 11-Mar-2014 07:52 2.3M
![[TXT]](http://www.audentia-gestion.fr/icons/text.gif)
Farnell-Leaded-Trans..> 23-Jun-2014 10:26 3.2M
![[TXT]](http://www.audentia-gestion.fr/icons/text.gif)
Farnell-Les-derniers..> 11-Mar-2014 07:50 2.3M
![[TXT]](http://www.audentia-gestion.fr/icons/text.gif)
Farnell-Loctite3455-..> 25-Mar-2014 08:16 3.0M
![[TXT]](http://www.audentia-gestion.fr/icons/text.gif)
Farnell-Low-cost-Enc..> 13-Jun-2014 18:42 1.7M
![[TXT]](http://www.audentia-gestion.fr/icons/text.gif)
Farnell-Lubrifiant-a..> 26-Mar-2014 18:00 2.7M
![[TXT]](http://www.audentia-gestion.fr/icons/text.gif)
Farnell-MC3510-PDF.htm 25-Mar-2014 08:17 3.0M
![[TXT]](http://www.audentia-gestion.fr/icons/text.gif)
Farnell-MC21605-PDF.htm 11-Mar-2014 08:14 2.8M
![[TXT]](http://www.audentia-gestion.fr/icons/text.gif)
Farnell-MCF532x-7x-E..> 29-Mar-2014 11:14 2.8M
![[TXT]](http://www.audentia-gestion.fr/icons/text.gif)
Farnell-MICREL-KSZ88..> 11-Mar-2014 07:54 2.2M
![[TXT]](http://www.audentia-gestion.fr/icons/text.gif)
Farnell-MICROCHIP-PI..> 19-Mar-2014 18:02 2.5M
![[TXT]](http://www.audentia-gestion.fr/icons/text.gif)
Farnell-MOLEX-39-00-..> 10-Mar-2014 17:19 1.9M
![[TXT]](http://www.audentia-gestion.fr/icons/text.gif)
Farnell-MOLEX-43020-..> 10-Mar-2014 17:21 1.9M
![[TXT]](http://www.audentia-gestion.fr/icons/text.gif)
Farnell-MOLEX-43160-..> 10-Mar-2014 17:21 1.9M
![[TXT]](http://www.audentia-gestion.fr/icons/text.gif)
Farnell-MOLEX-87439-..> 10-Mar-2014 17:21 1.9M
![[TXT]](http://www.audentia-gestion.fr/icons/text.gif)
Farnell-MPXV7002-Rev..> 20-Mar-2014 17:33 2.8M
![[TXT]](http://www.audentia-gestion.fr/icons/text.gif)
Farnell-MX670-MX675-..> 14-Jun-2014 09:46 2.5M
![[TXT]](http://www.audentia-gestion.fr/icons/text.gif)
Farnell-Microchip-MC..> 13-Jun-2014 18:27 1.8M
![[TXT]](http://www.audentia-gestion.fr/icons/text.gif)
Farnell-Microship-PI..> 11-Mar-2014 07:53 2.2M
![[TXT]](http://www.audentia-gestion.fr/icons/text.gif)
Farnell-Midas-Active..> 14-Jun-2014 18:17 3.4M
![[TXT]](http://www.audentia-gestion.fr/icons/text.gif)
Farnell-Midas-MCCOG4..> 14-Jun-2014 18:11 2.1M
![[TXT]](http://www.audentia-gestion.fr/icons/text.gif)
Farnell-Miniature-Ci..> 26-Mar-2014 17:55 2.8M
![[TXT]](http://www.audentia-gestion.fr/icons/text.gif)
Farnell-Mistral-PDF.htm 14-Jun-2014 18:12 2.1M
![[TXT]](http://www.audentia-gestion.fr/icons/text.gif)
Farnell-Molex-83421-..> 14-Jun-2014 18:17 3.4M
![[TXT]](http://www.audentia-gestion.fr/icons/text.gif)
Farnell-Molex-COMMER..> 14-Jun-2014 18:16 3.4M
![[TXT]](http://www.audentia-gestion.fr/icons/text.gif)
Farnell-Molex-Crimp-..> 10-Mar-2014 16:27 1.7M
![[TXT]](http://www.audentia-gestion.fr/icons/text.gif)
Farnell-Multi-Functi..> 20-Mar-2014 17:38 3.0M
![[TXT]](http://www.audentia-gestion.fr/icons/text.gif)
Farnell-NTE_SEMICOND..> 11-Mar-2014 07:52 2.3M
![[TXT]](http://www.audentia-gestion.fr/icons/text.gif)
Farnell-NXP-74VHC126..> 10-Mar-2014 16:17 1.6M
![[TXT]](http://www.audentia-gestion.fr/icons/text.gif)
Farnell-NXP-BT136-60..> 11-Mar-2014 07:52 2.3M
![[TXT]](http://www.audentia-gestion.fr/icons/text.gif)
Farnell-NXP-PBSS9110..> 10-Mar-2014 17:21 1.9M
![[TXT]](http://www.audentia-gestion.fr/icons/text.gif)
Farnell-NXP-PCA9555 ..> 11-Mar-2014 07:54 2.2M
![[TXT]](http://www.audentia-gestion.fr/icons/text.gif)
Farnell-NXP-PMBFJ620..> 10-Mar-2014 16:16 1.7M
![[TXT]](http://www.audentia-gestion.fr/icons/text.gif)
Farnell-NXP-PSMN1R7-..> 10-Mar-2014 16:17 1.6M
![[TXT]](http://www.audentia-gestion.fr/icons/text.gif)
Farnell-NXP-PSMN7R0-..> 10-Mar-2014 17:19 2.1M
![[TXT]](http://www.audentia-gestion.fr/icons/text.gif)
Farnell-NXP-TEA1703T..> 11-Mar-2014 08:15 2.8M
![[TXT]](http://www.audentia-gestion.fr/icons/text.gif)
Farnell-Nilï¬-sk-E-..> 14-Jun-2014 09:47 2.5M
![[TXT]](http://www.audentia-gestion.fr/icons/text.gif)
Farnell-Novembre-201..> 20-Mar-2014 17:38 3.3M
![[TXT]](http://www.audentia-gestion.fr/icons/text.gif)
Farnell-OMRON-Master..> 10-Mar-2014 16:26 1.8M
![[TXT]](http://www.audentia-gestion.fr/icons/text.gif)
Farnell-OSLON-SSL-Ce..> 19-Mar-2014 18:03 2.1M
![[TXT]](http://www.audentia-gestion.fr/icons/text.gif)
Farnell-OXPCIE958-FB..> 13-Jun-2014 18:40 1.8M
![[TXT]](http://www.audentia-gestion.fr/icons/text.gif)
Farnell-PADO-semi-au..> 04-Jul-2014 10:41 3.7M
![[TXT]](http://www.audentia-gestion.fr/icons/text.gif)
Farnell-PBSS5160T-60..> 19-Mar-2014 18:03 2.1M
![[TXT]](http://www.audentia-gestion.fr/icons/text.gif)
Farnell-PDTA143X-ser..> 20-Mar-2014 08:12 2.6M
![[TXT]](http://www.audentia-gestion.fr/icons/text.gif)
Farnell-PDTB123TT-NX..> 13-Jun-2014 18:43 1.5M
![[TXT]](http://www.audentia-gestion.fr/icons/text.gif)
Farnell-PESD5V0F1BL-..> 13-Jun-2014 18:43 1.5M
![[TXT]](http://www.audentia-gestion.fr/icons/text.gif)
Farnell-PESD9X5.0L-P..> 13-Jun-2014 18:43 1.6M
![[TXT]](http://www.audentia-gestion.fr/icons/text.gif)
Farnell-PIC12F609-61..> 04-Jul-2014 10:41 3.7M
![[TXT]](http://www.audentia-gestion.fr/icons/text.gif)
Farnell-PIC18F2455-2..> 23-Jun-2014 10:27 3.1M
![[TXT]](http://www.audentia-gestion.fr/icons/text.gif)
Farnell-PIC24FJ256GB..> 14-Jun-2014 09:51 2.4M
![[TXT]](http://www.audentia-gestion.fr/icons/text.gif)
Farnell-PMBT3906-PNP..> 13-Jun-2014 18:44 1.5M
![[TXT]](http://www.audentia-gestion.fr/icons/text.gif)
Farnell-PMBT4403-PNP..> 23-Jun-2014 10:27 3.1M
![[TXT]](http://www.audentia-gestion.fr/icons/text.gif)
Farnell-PMEG4002EL-N..> 14-Jun-2014 18:18 3.4M
![[TXT]](http://www.audentia-gestion.fr/icons/text.gif)
Farnell-PMEG4010CEH-..> 13-Jun-2014 18:43 1.6M
![[TXT]](http://www.audentia-gestion.fr/icons/text.gif)
Farnell-Panasonic-15..> 23-Jun-2014 10:29 2.1M
![[TXT]](http://www.audentia-gestion.fr/icons/text.gif)
Farnell-Panasonic-EC..> 20-Mar-2014 17:36 2.6M
![[TXT]](http://www.audentia-gestion.fr/icons/text.gif)
Farnell-Panasonic-EZ..> 20-Mar-2014 08:10 2.6M
![[TXT]](http://www.audentia-gestion.fr/icons/text.gif)
Farnell-Panasonic-Id..> 20-Mar-2014 17:35 2.6M
![[TXT]](http://www.audentia-gestion.fr/icons/text.gif)
Farnell-Panasonic-Ne..> 20-Mar-2014 17:36 2.6M
![[TXT]](http://www.audentia-gestion.fr/icons/text.gif)
Farnell-Panasonic-Ra..> 20-Mar-2014 17:37 2.6M
![[TXT]](http://www.audentia-gestion.fr/icons/text.gif)
Farnell-Panasonic-TS..> 20-Mar-2014 08:12 2.6M
![[TXT]](http://www.audentia-gestion.fr/icons/text.gif)
Farnell-Panasonic-Y3..> 20-Mar-2014 08:11 2.6M
![[TXT]](http://www.audentia-gestion.fr/icons/text.gif)
Farnell-Pico-Spox-Wi..> 10-Mar-2014 16:16 1.7M
![[TXT]](http://www.audentia-gestion.fr/icons/text.gif)
Farnell-Pompes-Charg..> 24-Apr-2014 20:23 3.3M
![[TXT]](http://www.audentia-gestion.fr/icons/text.gif)
Farnell-Ponts-RLC-po..> 14-Jun-2014 18:23 3.3M
![[TXT]](http://www.audentia-gestion.fr/icons/text.gif)
Farnell-Portable-Ana..> 29-Mar-2014 11:16 2.8M
![[TXT]](http://www.audentia-gestion.fr/icons/text.gif)
Farnell-Premier-Farn..> 21-Mar-2014 08:11 3.8M
![[TXT]](http://www.audentia-gestion.fr/icons/text.gif)
Farnell-Produit-3430..> 14-Jun-2014 09:48 2.5M
![[TXT]](http://www.audentia-gestion.fr/icons/text.gif)
Farnell-Proskit-SS-3..> 10-Mar-2014 16:26 1.8M
![[TXT]](http://www.audentia-gestion.fr/icons/text.gif)
Farnell-Puissance-ut..> 11-Mar-2014 07:49 2.4M
![[TXT]](http://www.audentia-gestion.fr/icons/text.gif)
Farnell-Q48-PDF.htm 23-Jun-2014 10:29 2.1M
![[TXT]](http://www.audentia-gestion.fr/icons/text.gif)
Farnell-Radial-Lead-..> 20-Mar-2014 08:12 2.6M
![[TXT]](http://www.audentia-gestion.fr/icons/text.gif)
Farnell-Realiser-un-..> 11-Mar-2014 07:51 2.3M
![[TXT]](http://www.audentia-gestion.fr/icons/text.gif)
Farnell-Reglement-RE..> 21-Mar-2014 08:08 3.9M
![[TXT]](http://www.audentia-gestion.fr/icons/text.gif)
Farnell-Repartiteurs..> 14-Jun-2014 18:26 2.5M
![[TXT]](http://www.audentia-gestion.fr/icons/text.gif)
Farnell-S-TRI-SWT860..> 21-Mar-2014 08:11 3.8M
![[TXT]](http://www.audentia-gestion.fr/icons/text.gif)
Farnell-SB175-Connec..> 11-Mar-2014 08:14 2.8M
![[TXT]](http://www.audentia-gestion.fr/icons/text.gif)
Farnell-SMBJ-Transil..> 29-Mar-2014 11:12 3.3M
![[TXT]](http://www.audentia-gestion.fr/icons/text.gif)
Farnell-SOT-23-Multi..> 11-Mar-2014 07:51 2.3M
![[TXT]](http://www.audentia-gestion.fr/icons/text.gif)
Farnell-SPLC780A1-16..> 14-Jun-2014 18:25 2.5M
![[TXT]](http://www.audentia-gestion.fr/icons/text.gif)
Farnell-SSC7102-Micr..> 23-Jun-2014 10:25 3.2M
![[TXT]](http://www.audentia-gestion.fr/icons/text.gif)
Farnell-SVPE-series-..> 14-Jun-2014 18:15 2.0M
![[TXT]](http://www.audentia-gestion.fr/icons/text.gif)
Farnell-Sensorless-C..> 04-Jul-2014 10:42 3.3M
![[TXT]](http://www.audentia-gestion.fr/icons/text.gif)
Farnell-Septembre-20..> 20-Mar-2014 17:46 3.7M
![[TXT]](http://www.audentia-gestion.fr/icons/text.gif)
Farnell-Serie-PicoSc..> 19-Mar-2014 18:01 2.5M
![[TXT]](http://www.audentia-gestion.fr/icons/text.gif)
Farnell-Serie-Standa..> 14-Jun-2014 18:23 3.3M
![[TXT]](http://www.audentia-gestion.fr/icons/text.gif)
Farnell-Series-2600B..> 20-Mar-2014 17:30 3.0M
![[TXT]](http://www.audentia-gestion.fr/icons/text.gif)
Farnell-Series-TDS10..> 04-Jul-2014 10:39 4.0M
![[TXT]](http://www.audentia-gestion.fr/icons/text.gif)
Farnell-Signal-PCB-R..> 14-Jun-2014 18:11 2.1M
![[TXT]](http://www.audentia-gestion.fr/icons/text.gif)
Farnell-Strangkuhlko..> 21-Mar-2014 08:09 3.9M
![[TXT]](http://www.audentia-gestion.fr/icons/text.gif)
Farnell-Supercapacit..> 26-Mar-2014 17:57 2.7M
![[TXT]](http://www.audentia-gestion.fr/icons/text.gif)
Farnell-TDK-Lambda-H..> 14-Jun-2014 18:21 3.3M
![[TXT]](http://www.audentia-gestion.fr/icons/text.gif)
Farnell-TEKTRONIX-DP..> 10-Mar-2014 17:20 2.0M
![[TXT]](http://www.audentia-gestion.fr/icons/text.gif)
Farnell-Tektronix-AC..> 13-Jun-2014 18:44 1.5M
![[TXT]](http://www.audentia-gestion.fr/icons/text.gif)
Farnell-Telemetres-l..> 20-Mar-2014 17:46 3.7M
![[TXT]](http://www.audentia-gestion.fr/icons/text.gif)
Farnell-Termometros-..> 14-Jun-2014 18:14 2.0M
![[TXT]](http://www.audentia-gestion.fr/icons/text.gif)
Farnell-The-essentia..> 10-Mar-2014 16:27 1.7M
![[TXT]](http://www.audentia-gestion.fr/icons/text.gif)
Farnell-U2270B-PDF.htm 14-Jun-2014 18:15 3.4M
![[TXT]](http://www.audentia-gestion.fr/icons/text.gif)
Farnell-USB-Buccanee..> 14-Jun-2014 09:48 2.5M
![[TXT]](http://www.audentia-gestion.fr/icons/text.gif)
Farnell-USB1T11A-PDF..> 19-Mar-2014 18:03 2.1M
![[TXT]](http://www.audentia-gestion.fr/icons/text.gif)
Farnell-V4N-PDF.htm 14-Jun-2014 18:11 2.1M
![[TXT]](http://www.audentia-gestion.fr/icons/text.gif)
Farnell-WetTantalum-..> 11-Mar-2014 08:14 2.8M
![[TXT]](http://www.audentia-gestion.fr/icons/text.gif)
Farnell-XPS-AC-Octop..> 14-Jun-2014 18:11 2.1M
![[TXT]](http://www.audentia-gestion.fr/icons/text.gif)
Farnell-XPS-MC16-XPS..> 11-Mar-2014 08:15 2.8M
![[TXT]](http://www.audentia-gestion.fr/icons/text.gif)
Farnell-YAGEO-DATA-S..> 11-Mar-2014 08:13 2.8M
![[TXT]](http://www.audentia-gestion.fr/icons/text.gif)
Farnell-ZigBee-ou-le..> 11-Mar-2014 07:50 2.4M
![[TXT]](http://www.audentia-gestion.fr/icons/text.gif)
Farnell-celpac-SUL84..> 21-Mar-2014 08:11 3.8M
![[TXT]](http://www.audentia-gestion.fr/icons/text.gif)
Farnell-china_rohs_o..> 21-Mar-2014 10:04 3.9M
![[TXT]](http://www.audentia-gestion.fr/icons/text.gif)
Farnell-cree-Xlamp-X..> 20-Mar-2014 17:34 2.8M
![[TXT]](http://www.audentia-gestion.fr/icons/text.gif)
Farnell-cree-Xlamp-X..> 20-Mar-2014 17:35 2.7M
![[TXT]](http://www.audentia-gestion.fr/icons/text.gif)
Farnell-cree-Xlamp-X..> 20-Mar-2014 17:31 2.9M
![[TXT]](http://www.audentia-gestion.fr/icons/text.gif)
Farnell-cree-Xlamp-m..> 20-Mar-2014 17:32 2.9M
![[TXT]](http://www.audentia-gestion.fr/icons/text.gif)
Farnell-cree-Xlamp-m..> 20-Mar-2014 17:32 2.9M
![[TXT]](http://www.audentia-gestion.fr/icons/text.gif)
Farnell-ir1150s_fr.p..> 29-Mar-2014 11:11 3.3M
![[TXT]](http://www.audentia-gestion.fr/icons/text.gif)
Farnell-manual-bus-p..> 10-Mar-2014 16:29 1.9M
![[TXT]](http://www.audentia-gestion.fr/icons/text.gif)
Farnell-propose-plus..> 11-Mar-2014 08:19 2.8M
![[TXT]](http://www.audentia-gestion.fr/icons/text.gif)
Farnell-techfirst_se..> 21-Mar-2014 08:08 3.9M
![[TXT]](http://www.audentia-gestion.fr/icons/text.gif)
Farnell-testo-205-20..> 20-Mar-2014 17:37 3.0M
![[TXT]](http://www.audentia-gestion.fr/icons/text.gif)
Farnell-testo-470-Fo..> 20-Mar-2014 17:38 3.0M
![[TXT]](http://www.audentia-gestion.fr/icons/text.gif)
Farnell-uC-OS-III-Br..> 10-Mar-2014 17:20 2.0M
![[TXT]](http://www.audentia-gestion.fr/icons/text.gif)
Sefram-7866HD.pdf-PD..> 29-Mar-2014 11:46 472K
![[TXT]](http://www.audentia-gestion.fr/icons/text.gif)
Sefram-CAT_ENREGISTR..> 29-Mar-2014 11:46 461K
![[TXT]](http://www.audentia-gestion.fr/icons/text.gif)
Sefram-CAT_MESUREURS..> 29-Mar-2014 11:46 435K
![[TXT]](http://www.audentia-gestion.fr/icons/text.gif)
Sefram-GUIDE_SIMPLIF..> 29-Mar-2014 11:46 481K
![[TXT]](http://www.audentia-gestion.fr/icons/text.gif)
Sefram-GUIDE_SIMPLIF..> 29-Mar-2014 11:46 442K
![[TXT]](http://www.audentia-gestion.fr/icons/text.gif)
Sefram-GUIDE_SIMPLIF..> 29-Mar-2014 11:46 422K
![[TXT]](http://www.audentia-gestion.fr/icons/text.gif)
Sefram-SP270.pdf-PDF..> 29-Mar-2014 11:46 464K
© 2007 Microchip Technology Inc. Preliminary DS39631B
PIC18F2420/2520/4420/4520
Data Sheet
Enhanced Flash Microcontrollers
with 10-Bit A/D and nanoWatt Technology
DS39631B-page ii Preliminary © 2007 Microchip Technology Inc.
Information contained in this publication regarding device
applications and the like is provided only for your convenience
and may be superseded by updates. It is your responsibility to
ensure that your application meets with your specifications.
MICROCHIP MAKES NO REPRESENTATIONS OR
WARRANTIES OF ANY KIND WHETHER EXPRESS OR
IMPLIED, WRITTEN OR ORAL, STATUTORY OR
OTHERWISE, RELATED TO THE INFORMATION,
INCLUDING BUT NOT LIMITED TO ITS CONDITION,
QUALITY, PERFORMANCE, MERCHANTABILITY OR
FITNESS FOR PURPOSE. Microchip disclaims all liability
arising from this information and its use. Use of Microchip
devices in life support and/or safety applications is entirely at
the buyer’s risk, and the buyer agrees to defend, indemnify and
hold harmless Microchip from any and all damages, claims,
suits, or expenses resulting from such use. No licenses are
conveyed, implicitly or otherwise, under any Microchip
intellectual property rights.
Trademarks
The Microchip name and logo, the Microchip logo, Accuron,
dsPIC, KEELOQ, microID, MPLAB, PIC, PICmicro, PICSTART,
PRO MATE, PowerSmart, rfPIC and SmartShunt are
registered trademarks of Microchip Technology Incorporated
in the U.S.A. and other countries.
AmpLab, FilterLab, Migratable Memory, MXDEV, MXLAB,
SEEVAL, SmartSensor and The Embedded Control Solutions
Company are registered trademarks of Microchip Technology
Incorporated in the U.S.A.
Analog-for-the-Digital Age, Application Maestro, CodeGuard,
dsPICDEM, dsPICDEM.net, dsPICworks, ECAN,
ECONOMONITOR, FanSense, FlexROM, fuzzyLAB,
In-Circuit Serial Programming, ICSP, ICEPIC, Linear Active
Thermistor, Mindi, MiWi, MPASM, MPLIB, MPLINK, PICkit,
PICDEM, PICDEM.net, PICLAB, PICtail, PowerCal,
PowerInfo, PowerMate, PowerTool, REAL ICE, rfLAB,
rfPICDEM, Select Mode, Smart Serial, SmartTel, Total
Endurance, UNI/O, WiperLock and ZENA are trademarks of
Microchip Technology Incorporated in the U.S.A. and other
countries.
SQTP is a service mark of Microchip Technology Incorporated
in the U.S.A.
All other trademarks mentioned herein are property of their
respective companies.
© 2007, Microchip Technology Incorporated, Printed in the
U.S.A., All Rights Reserved.
Printed on recycled paper.
Note the following details of the code protection feature on Microchip devices:
• Microchip products meet the specification contained in their particular Microchip Data Sheet.
• Microchip believes that its family of products is one of the most secure families of its kind on the market today, when used in the
intended manner and under normal conditions.
• There are dishonest and possibly illegal methods used to breach the code protection feature. All of these methods, to our
knowledge, require using the Microchip products in a manner outside the operating specifications contained in Microchip’s Data
Sheets. Most likely, the person doing so is engaged in theft of intellectual property.
• Microchip is willing to work with the customer who is concerned about the integrity of their code.
• Neither Microchip nor any other semiconductor manufacturer can guarantee the security of their code. Code protection does not
mean that we are guaranteeing the product as “unbreakable.”
Code protection is constantly evolving. We at Microchip are committed to continuously improving the code protection features of our
products. Attempts to break Microchip’s code protection feature may be a violation of the Digital Millennium Copyright Act. If such acts
allow unauthorized access to your software or other copyrighted work, you may have a right to sue for relief under that Act.
Microchip received ISO/TS-16949:2002 certification for its worldwide
headquarters, design and wafer fabrication facilities in Chandler and
Tempe, Arizona, Gresham, Oregon and Mountain View, California. The
Company’s quality system processes and procedures are for its PIC®
MCUs and dsPIC DSCs, KEELOQ® code hopping devices, Serial
EEPROMs, microperipherals, nonvolatile memory and analog
products. In addition, Microchip’s quality system for the design and
manufacture of development systems is ISO 9001:2000 certified.
© 2007 Microchip Technology Inc. Preliminary DS39631B-page 1
PIC18F2420/2520/4420/4520
Power Managed Modes:
• Run: CPU on, peripherals on
• Idle: CPU off, peripherals on
• Sleep: CPU off, peripherals off
• Idle mode currents down to 5.8 μA typical
• Sleep mode current down to 0.1 μA typical
• Timer1 Oscillator: 1.8 μA, 32 kHz, 2V
• Watchdog Timer: 2.1 μA
• Two-Speed Oscillator Start-up
Peripheral Highlights:
• High-current sink/source 25 mA/25 mA
• Three programmable external interrupts
• Four input change interrupts
• Up to 2 Capture/Compare/PWM (CCP) modules,
one with Auto-Shutdown (28-pin devices)
• Enhanced Capture/Compare/PWM (ECCP)
module (40/44-pin devices only):
- One, two or four PWM outputs
- Selectable polarity
- Programmable dead time
- Auto-Shutdown and Auto-Restart
• Master Synchronous Serial Port (MSSP) module
supporting 3-wire SPI™ (all 4 modes) and I2C™
Master and Slave Modes
• Enhanced Addressable USART module:
- Supports RS-485, RS-232 and LIN 1.2
- RS-232 operation using internal oscillator
block (no external crystal required)
- Auto-Wake-up on Start bit
- Auto-Baud Detect
• 10-bit, up to 13-channel Analog-to-Digital
Converter module (A/D):
- Auto-acquisition capability
- Conversion available during Sleep
• Dual analog comparators with input multiplexing)
Flexible Oscillator Structure:
• Four Crystal modes, up to 40 MHz
• 4X Phase Lock Loop (available for crystal and
internal oscillators)
• Two External RC modes, up to 4 MHz
• Two External Clock modes, up to 40 MHz
• Internal oscillator block:
- 8 user selectable frequencies, from 31 kHz to 8 MHz
- Provides a complete range of clock speeds
from 31 kHz to 32 MHz when used with PLL
- User tunable to compensate for frequency drift
• Secondary oscillator using Timer1 @ 32 kHz
• Fail-Safe Clock Monitor:
- Allows for safe shutdown if peripheral clock stops
Special Microcontroller Features:
• C compiler optimized architecture:
- Optional extended instruction set designed to
optimize re-entrant code
• 100,000 erase/write cycle Enhanced Flash
program memory typical
• 1,000,000 erase/write cycle Data EEPROM
memory typical
• Flash/Data EEPROM Retention: 100 years typical
• Self-programmable under software control
• Priority levels for interrupts
• 8 x 8 Single-Cycle Hardware Multiplier
• Extended Watchdog Timer (WDT):
- Programmable period from 4 ms to 131s
• Single-supply 5V In-Circuit Serial
Programming™ (ICSP™) via two pins
• In-Circuit Debug (ICD) via two pins
• Wide operating voltage range: 2.0V to 5.5V
• Programmable 16-level High/Low-Voltage
Detection (HLVD) module:
- Supports interrupt on High/Low-Voltage
Detection
• Programmable Brown-out Reset (BOR
- With software enable option
28/40/44-Pin Enhanced Flash Microcontrollers with
10-Bit A/D and nanoWatt Technology
PIC18F2420/2520/4420/4520
DS39631B-page 2 Preliminary © 2007 Microchip Technology Inc.
-
Device
Program Memory Data Memory
I/O
10-bit
A/D (ch)
CCP/
ECCP
(PWM)
MSSP
EUSART
Comp.
Timers
Flash 8/16-bit
(bytes)
# Single-Word
Instructions
SRAM
(bytes)
EEPROM
(bytes)
SPI
Master
I2C
PIC18F2420 16K 8192 768 256 25 10 2/0 Y Y 1 2 1/3
PIC18F2520 32K 16384 1536 256 25 10 2/0 Y Y 1 2 1/3
PIC18F4420 16K 8192 768 256 36 13 1/1 Y Y 1 2 1/3
PIC18F4520 32K 16384 1536 256 36 13 1/1 Y Y 1 2 1/3
© 2007 Microchip Technology Inc. Preliminary DS39631B-page 3
PIC18F2420/2520/4420/4520
Pin Diagrams
RB7/KBI3/PGD
RB6/KBI2/PGC
RB5/KBI1/PGM
RB4/KBI0/AN11
RB3/AN9/CCP2(1)
RB2/INT2/AN8
RB1/INT1/AN10
RB0/INT0/FLT0/AN12
VDD
VSS
RD7/PSP7/P1D
RD6/PSP6/P1C
RD5/PSP5/P1B
RD4/PSP4
RC7/RX/DT
RC6/TX/CK
RC5/SDO
RC4/SDI/SDA
RD3/PSP3
RD2/PSP2
MCLR/VPP/RE3
RA0/AN0
RA1/AN1
RA2/AN2/VREF-/CVREF
RA3/AN3/VREF+
RA4/T0CKI/C1OUT
RA5/AN4/SS/HLVDIN/C2OUT
RE0/RD/AN5
RE1/WR/AN6
RE2/CS/AN7
VDD
VSS
OSC1/CLKI/RA7
OSC2/CLKO/RA6
RC0/T1OSO/T13CKI
RC1/T1OSI/CCP2(1)
RC2/CCP1/P1A
RC3/SCK/SCL
RD0/PSP0
RD1/PSP1
1
2
3
4
5
6
7
8
9
10
11
12
13
14
15
16
17
18
19
20
40
39
38
37
36
35
34
33
32
31
30
29
28
27
26
25
24
23
22
21
PIC18F4520 PIC18F2520
10
11
2
3
4
5
6
1
8
7
9
12
13
14 15
16
17
18
19
20
23
24
25
26
27
28
22
21
MCLR/VPP/RE3
RA0/AN0
RA1/AN1
RA2/AN2/VREF-/CVREF
RA3/AN3/VREF+
RA4/T0CKI/C1OUT
RA5/AN4/SS/HLVDIN/C2OUT
VSS
OSC1/CLKI/RA7
OSC2/CLKO/RA6
RC0/T1OSO/T13CKI
RC1/T1OSI/CCP2(1)
RC2/CCP1
RC3/SCK/SCL
RB7/KBI3/PGD
RB6//KBI2/PGC
RB5/KBI1/PGM
RB4/KBI0/AN11
RB3/AN9/CCP2(1)
RB2/INT2/AN8
RB1/INT1/AN10
RB0/INT0/FLT0/AN12
VDD
VSS
RC7/RX/DT
RC6/TX/CK
RC5/SDO
RC4/SDI/SDA
40-pin PDIP
28-pin PDIP, SOIC
PIC18F4420 PIC18F2420
Note 1: RB3 is the alternate pin for CCP2 multiplexing.
1011
2
3
6
1
18
19
20
21
22
12 13 14
15
8
7
16
17
2827 2625 2423
9
PIC18F2420
RC0/T1OSO/T13CKI
5
4
RB7/KBI3/PGD
RB6/KBI2/PGC
RB5/KBI1/PGM
RB4KBI0/AN11
RB3/AN9/CCP2(1)
RB2/INT2/AN8
RB1/INT1/AN10
RB0/INT0/FLT0/AN12
VDD
VSS
RC7/RX/DT
RC6/TX/CK
RC5/SDO
RC4/SDI/SDA
MCLR/VPP/RE3
RA0/AN0
RA1/AN1
RA2/AN2/VREF-/CVREF
RA3/AN3/VREF+
RA4/T0CKI/C1OUT
RA5/AN4/SS/HLVDIN/C2OUT
VSS
OSC1/CLKI/RA7
OSC2/CLKO/RA6
RC1/T1OSI/CCP2(1)
RC2/CCP1
RC3/SCK/SCL
PIC18F2520
28-pin QFN
PIC18F2420/2520/4420/4520
DS39631B-page 4 Preliminary © 2007 Microchip Technology Inc.
Pin Diagrams (Cont.’d)
Note 1: RB3 is the alternate pin for CCP2 multiplexing.
10
11
2
345
6
1
18
19
20
21
22
12
13
14
15
38
8 7
44
43
42
41
40
39
16
17
29
30
31
32
33
23
24
25
26
27
28
36
34
35
9
PIC18F4420
37
RA3/AN3/VREF+
RA2/AN2/VREF-/CVREF
RA1/AN1
RA0/AN0
MCLR/VPP/RE3
RB3/AN9/CCP2(1)
RB7/KBI3/PGD
RB6/KBI2/PGC
RB5/KBI1/PGM
RB4/KBI0/AN11
NC
RC6/TX/CK
RC5/SDO
RC4/SDI/SDA
RD3/PSP3
RD2/PSP2
RD1/PSP1
RD0/PSP0
RC3/SCK/SCL
RC2/CCP1/P1A
RC1/T1OSI/CCP2(1)
RC0/T1OSO/T13CKI
OSC2/CLKO/RA6
OSC1/CLKI/RA7
VSS
VSS
VDD
VDD
RE2/CS/AN7
RE1/WR/AN6
RE0/RD/AN5
RA5/AN4/SS/HLVDIN/C2OUT
RA4/T0CKI/C1OUT
RC7/RX/DT
RD4/PSP4
RD5/PSP5/P1B
RD6/PSP6/P1C
RD7/PSP7/P1D
VSS
VDD
VDD
RB0/INT0/FLT0/AN12
RB1/INT1/AN10
RB2/INT2/AN8
44-pin QFN
PIC18F4520
10
11
2
345
6
1
18
19
20
21
22
12
13
14
15
38
8 7
44
43
42
41
40
39
16
17
29
30
31
32
33
23
24
25
26
27
28
36
34
35
9
PIC18F4420
37
RA3/AN3/VREF+
RA2/AN2/VREF-/CVREF
RA1/AN1
RA0/AN0
MCLR/VPP/RE3
NC
RB7/KBI3/PGD
RB6/KBI2/PGC
RB5/KBI1/PGM
RB4/KBI0/AN11
NC
RC6/TX/CK
RC5/SDO
RC4/SDI/SDA
RD3/PSP3
RD2/PSP2
RD1/PSP1
RD0/PSP0
RC3/SCK/SCL
RC2/CCP1/P1A
RC1/T1OSI/CCP2(1)
NC
NC
RC0/T1OSO/T13CKI
OSC2/CLKO/RA6
OSC1/CLKI/RA7
VSS
VDD
RE2/CS/AN7
RE1/WR/AN6
RE0/RD/AN5
RA5/AN4/SS/HLVDIN/C2OUT
RA4/T0CKI/C1OUT
RC7/RX/DT
RD4/PSP4
RD5/PSP5/P1B
RD6/PSP6/P1C
RD7/PSP7/P1D
VSS
VDD
RB0/INT0/FLT0/AN12
RB1/INT1/AN10
RB2/INT2/AN8
RB3/AN9/CCP2(1)
44-pin TQFP
PIC18F4520
© 2007 Microchip Technology Inc. Preliminary DS39631B-page 5
PIC18F2420/2520/4420/4520
Table of Contents
1.0 Device Overview .......................................................................................................................................................................... 7
2.0 Oscillator Configurations ............................................................................................................................................................ 23
3.0 Power Managed Modes ............................................................................................................................................................. 33
4.0 Reset .......................................................................................................................................................................................... 41
5.0 Memory Organization................................................................................................................................................................. 53
6.0 Flash Program Memory.............................................................................................................................................................. 73
7.0 Data EEPROM Memory ............................................................................................................................................................. 83
8.0 8 x 8 Hardware Multiplier............................................................................................................................................................ 89
9.0 Interrupts .................................................................................................................................................................................... 91
10.0 I/O Ports ................................................................................................................................................................................... 105
11.0 Timer0 Module ......................................................................................................................................................................... 123
12.0 Timer1 Module ......................................................................................................................................................................... 127
13.0 Timer2 Module ......................................................................................................................................................................... 133
14.0 Timer3 Module ......................................................................................................................................................................... 135
15.0 Capture/Compare/Pwm (CCP) Modules .................................................................................................................................. 139
16.0 Enhanced Capture/Compare/PWM (ECCP) Module................................................................................................................ 147
17.0 Master Synchronous Serial Port (MSSP) Module .................................................................................................................... 161
18.0 Enhanced Universal Synchronous Receiver Transmitter (EUSART)....................................................................................... 201
19.0 10-Bit Analog-to-Digital Converter (A/D) Module ..................................................................................................................... 223
20.0 Comparator Module.................................................................................................................................................................. 233
21.0 Comparator Voltage Reference Module................................................................................................................................... 239
22.0 High/Low-Voltage Detect (HLVD)............................................................................................................................................. 243
23.0 Special Features of the CPU.................................................................................................................................................... 249
24.0 Instruction Set Summary .......................................................................................................................................................... 267
25.0 Development Support............................................................................................................................................................... 317
26.0 Electrical Characteristics .......................................................................................................................................................... 323
27.0 DC and AC Characteristics Graphs and Tables....................................................................................................................... 361
28.0 Packaging Information.............................................................................................................................................................. 363
Appendix A: Revision History............................................................................................................................................................. 371
Appendix B: Device Differences ........................................................................................................................................................ 371
Appendix C: Conversion Considerations ........................................................................................................................................... 372
Appendix D: Migration from Baseline to Enhanced Devices.............................................................................................................. 372
Appendix E: Migration from Mid-Range to Enhanced Devices .......................................................................................................... 373
Appendix F: Migration from High-End to Enhanced Devices............................................................................................................. 373
Index .................................................................................................................................................................................................. 375
On-Line Support................................................................................................................................................................................. 385
Systems Information and Upgrade Hot Line ...................................................................................................................................... 385
Reader Response .............................................................................................................................................................................. 386
PIC18F2420/2520/4420/4520 Product Identification System ............................................................................................................ 387
PIC18F2420/2520/4420/4520
DS39631B-page 6 Preliminary © 2007 Microchip Technology Inc.
TO OUR VALUED CUSTOMERS
It is our intention to provide our valued customers with the best documentation possible to ensure successful use of your Microchip
products. To this end, we will continue to improve our publications to better suit your needs. Our publications will be refined and
enhanced as new volumes and updates are introduced.
If you have any questions or comments regarding this publication, please contact the Marketing Communications Department via
E-mail at docerrors@microchip.com or fax the Reader Response Form in the back of this data sheet to (480) 792-4150. We
welcome your feedback.
Most Current Data Sheet
To obtain the most up-to-date version of this data sheet, please register at our Worldwide Web site at:
http://www.microchip.com
You can determine the version of a data sheet by examining its literature number found on the bottom outside corner of any page.
The last character of the literature number is the version number, (e.g., DS30000A is version A of document DS30000).
Errata
An errata sheet, describing minor operational differences from the data sheet and recommended workarounds, may exist for current
devices. As device/documentation issues become known to us, we will publish an errata sheet. The errata will specify the revision
of silicon and revision of document to which it applies.
To determine if an errata sheet exists for a particular device, please check with one of the following:
• Microchip’s Worldwide Web site; http://www.microchip.com
• Your local Microchip sales office (see last page)
When contacting a sales office, please specify which device, revision of silicon and data sheet (include literature number) you are
using.
Customer Notification System
Register on our web site at www.microchip.com to receive the most current information on all of our products.
© 2007 Microchip Technology Inc. Preliminary DS39631B-page 7
PIC18F2420/2520/4420/4520
1.0 DEVICE OVERVIEW
This document contains device specific information for
the following devices:
This family offers the advantages of all PIC18 microcontrollers
– namely, high computational performance
at an economical price – with the addition of highendurance,
Enhanced Flash program memory. On top
of these features, the PIC18F2420/2520/4420/4520
family introduces design enhancements that make
these microcontrollers a logical choice for many highperformance,
power sensitive applications.
1.1 New Core Features
1.1.1 nanoWatt TECHNOLOGY
All of the devices in the PIC18F2420/2520/4420/4520
family incorporate a range of features that can significantly
reduce power consumption during operation.
Key items include:
• Alternate Run Modes: By clocking the controller
from the Timer1 source or the internal oscillator
block, power consumption during code execution
can be reduced by as much as 90%.
• Multiple Idle Modes: The controller can also run
with its CPU core disabled but the peripherals still
active. In these states, power consumption can be
reduced even further, to as little as 4% of normal
operation requirements.
• On-the-fly Mode Switching: The power
managed modes are invoked by user code during
operation, allowing the user to incorporate powersaving
ideas into their application’s software
design.
• Low Consumption in Key Modules: The
power requirements for both Timer1 and the
Watchdog Timer are minimized. See
Section 26.0 “Electrical Characteristics”
for values.
1.1.2 MULTIPLE OSCILLATOR OPTIONS
AND FEATURES
All of the devices in the PIC18F2420/2520/4420/4520
family offer ten different oscillator options, allowing
users a wide range of choices in developing application
hardware. These include:
• Four Crystal modes, using crystals or ceramic
resonators
• Two External Clock modes, offering the option of
using two pins (oscillator input and a divide-by-4
clock output) or one pin (oscillator input, with the
second pin reassigned as general I/O)
• Two External RC Oscillator modes with the same
pin options as the External Clock modes
• An internal oscillator block which provides an
8 MHz clock and an INTRC source (approximately
31 kHz), as well as a range of 6 user
selectable clock frequencies, between 125 kHz to
4 MHz, for a total of 8 clock frequencies. This
option frees the two oscillator pins for use as
additional general purpose I/O.
• A Phase Lock Loop (PLL) frequency multiplier,
available to both the high-speed crystal and internal
oscillator modes, which allows clock speeds of
up to 40 MHz. Used with the internal oscillator, the
PLL gives users a complete selection of clock
speeds, from 31 kHz to 32 MHz – all without using
an external crystal or clock circuit.
Besides its availability as a clock source, the internal
oscillator block provides a stable reference source that
gives the family additional features for robust
operation:
• Fail-Safe Clock Monitor: This option constantly
monitors the main clock source against a reference
signal provided by the internal oscillator. If a
clock failure occurs, the controller is switched to
the internal oscillator block, allowing for continued
low-speed operation or a safe application
shutdown.
• Two-Speed Start-up: This option allows the
internal oscillator to serve as the clock source
from Power-on Reset, or wake-up from Sleep
mode, until the primary clock source is available.
• PIC18F2420 • PIC18LF2420
• PIC18F2520 • PIC18LF2520
• PIC18F4420 • PIC18LF4420
• PIC18F4520 • PIC18LF4520
PIC18F2420/2520/4420/4520
DS39631B-page 8 Preliminary © 2007 Microchip Technology Inc.
1.2 Other Special Features
• Memory Endurance: The Enhanced Flash cells
for both program memory and data EEPROM are
rated to last for many thousands of erase/write
cycles – up to 100,000 for program memory and
1,000,000 for EEPROM. Data retention without
refresh is conservatively estimated to be greater
than 40 years.
• Self-programmability: These devices can write
to their own program memory spaces under internal
software control. By using a bootloader routine
located in the protected Boot Block at the top
of program memory, it becomes possible to create
an application that can update itself in the field.
• Extended Instruction Set: The PIC18F2420/
2520/4420/4520 family introduces an optional
extension to the PIC18 instruction set, which adds
8 new instructions and an Indexed Addressing
mode. This extension, enabled as a device configuration
option, has been specifically designed
to optimize re-entrant application code originally
developed in high-level languages, such as C.
• Enhanced CCP module: In PWM mode, this
module provides 1, 2 or 4 modulated outputs for
controlling half-bridge and full-bridge drivers.
Other features include Auto-Shutdown, for disabling
PWM outputs on interrupt or other select
conditions and Auto-Restart, to reactivate outputs
once the condition has cleared.
• Enhanced Addressable USART: This serial
communication module is capable of standard
RS-232 operation and provides support for the LIN
bus protocol. Other enhancements include
automatic baud rate detection and a 16-bit Baud
Rate Generator for improved resolution. When the
microcontroller is using the internal oscillator
block, the USART provides stable operation for
applications that talk to the outside world without
using an external crystal (or its accompanying
power requirement).
• 10-bit A/D Converter: This module incorporates
programmable acquisition time, allowing for a
channel to be selected and a conversion to be
initiated without waiting for a sampling period and
thus, reduce code overhead.
• Extended Watchdog Timer (WDT): This
enhanced version incorporates a 16-bit prescaler,
allowing an extended time-out range that is stable
across operating voltage and temperature. See
Section 26.0 “Electrical Characteristics” for
time-out periods.
1.3 Details on Individual Family
Members
Devices in the PIC18F2420/2520/4420/4520 family are
available in 28-pin and 40/44-pin packages. Block
diagrams for the two groups are shown in Figure 1-1
and Figure 1-2.
The devices are differentiated from each other in five
ways:
1. Flash program memory (16 Kbytes for
PIC18F2420/4420 devices and 32 Kbytes for
PIC18F2520/4520).
2. A/D channels (10 for 28-pin devices, 13 for
40/44-pin devices).
3. I/O ports (3 bidirectional ports on 28-pin devices,
5 bidirectional ports on 40/44-pin devices).
4. CCP and Enhanced CCP implementation
(28-pin devices have 2 standard CCP modules,
40/44-pin devices have one standard CCP
module and one ECCP module).
5. Parallel Slave Port (present only on 40/44-pin
devices).
All other features for devices in this family are identical.
These are summarized in Table 1-1.
The pinouts for all devices are listed in Table 1-2 and
Table 1-3.
Like all Microchip PIC18 devices, members of the
PIC18F2420/2520/4420/4520 family are available as
both standard and low-voltage devices. Standard
devices with Enhanced Flash memory, designated with
an “F” in the part number (such as PIC18F2420),
accommodate an operating VDD range of 4.2V to 5.5V.
Low-voltage parts, designated by “LF” (such as
PIC18LF2420), function over an extended VDD range
of 2.0V to 5.5V.
© 2007 Microchip Technology Inc. Preliminary DS39631B-page 9
PIC18F2420/2520/4420/4520
TABLE 1-1: DEVICE FEATURES
Features PIC18F2420 PIC18F2520 PIC18F4420 PIC18F4520
Operating Frequency DC – 40 MHz DC – 40 MHz DC – 40 MHz DC – 40 MHz
Program Memory (Bytes) 16384 32768 16384 32768
Program Memory
(Instructions)
8192 16384 8192 16384
Data Memory (Bytes) 768 1536 768 1536
Data EEPROM Memory (Bytes) 256 256 256 256
Interrupt Sources 19 19 20 20
I/O Ports Ports A, B, C, (E) Ports A, B, C, (E) Ports A, B, C, D, E Ports A, B, C, D, E
Timers 4 4 4 4
Capture/Compare/PWM Modules 2 2 1 1
Enhanced
Capture/Compare/PWM Modules
0 0 1 1
Serial Communications MSSP,
Enhanced USART
MSSP,
Enhanced USART
MSSP,
Enhanced USART
MSSP,
Enhanced USART
Parallel Communications (PSP) No No Yes Yes
10-bit Analog-to-Digital Module 10 Input Channels 10 Input Channels 13 Input Channels 13 Input Channels
Resets (and Delays) POR, BOR,
RESET Instruction,
Stack Full, Stack
Underflow (PWRT, OST),
MCLR (optional), WDT
POR, BOR,
RESET Instruction,
Stack Full, Stack
Underflow (PWRT, OST),
MCLR (optional), WDT
POR, BOR,
RESET Instruction,
Stack Full, Stack
Underflow (PWRT, OST),
MCLR (optional), WDT
POR, BOR,
RESET Instruction,
Stack Full, Stack
Underflow (PWRT, OST),
MCLR (optional), WDT
Programmable
High/Low-Voltage Detect
Yes Yes Yes Yes
Programmable Brown-out Reset Yes Yes Yes Yes
Instruction Set 75 Instructions;
83 with Extended
Instruction Set enabled
75 Instructions;
83 with Extended
Instruction Set enabled
75 Instructions;
83 with Extended
Instruction Set enabled
75 Instructions;
83 with Extended
Instruction Set enabled
Packages 28-pin PDIP
28-pin SOIC
28-pin QFN
28-pin PDIP
28-pin SOIC
28-pin QFN
40-pin PDIP
44-pin QFN
44-pin TQFP
40-pin PDIP
44-pin QFN
44-pin TQFP
PIC18F2420/2520/4420/4520
DS39631B-page 10 Preliminary © 2007 Microchip Technology Inc.
FIGURE 1-1: PIC18F2420/2520 (28-PIN) BLOCK DIAGRAM
Instruction
Decode and
Control
PORTA
PORTB
PORTC
RA4/T0CKI/C1OUT
RA5/AN4/SS/HLVDIN/C2OUT
RB0/INT0/FLT0/AN12
RC0/T1OSO/T13CKI
RC1/T1OSI/CCP2(1)
RC2/CCP1
RC3/SCK/SCL
RC4/SDI/SDA
RC5/SDO
RC6/TX/CK
RC7/RX/DT
RA3/AN3/VREF+
RA2/AN2/VREF-/CVREF
RA1/AN1
RA0/AN0
RB1/INT1/AN10
Data Latch
Data Memory
( 3.9 Kbytes )
Address Latch
Data Address<12>
12
BSR FSR0 Access
FSR1
FSR2
inc/dec
logic
Address
4 12 4
PCH PCL
PCLATH
8
31 Level Stack
Program Counter
PRODH PRODL
8 x 8 Multiply
8
BITOP
8 8
ALU<8>
Address Latch
Program Memory
(16/32 Kbytes)
Data Latch
20
8
8
Table Pointer<21>
inc/dec logic
21
8
Data Bus<8>
Table Latch
8
IR
12
3
ROM Latch
RB2/INT2/AN8
RB3/AN9/CCP2(1)
PCLATU
PCU
OSC2/CLKO(3)/RA6
Note 1: CCP2 is multiplexed with RC1 when configuration bit CCP2MX is set, or RB3 when CCP2MX is not set.
2: RE3 is only available when MCLR functionality is disabled.
3: OSC1/CLKI and OSC2/CLKO are only available in select oscillator modes and when these pins are not being used as digital I/O.
Refer to Section 2.0 “Oscillator Configurations” for additional information.
RB4/KBI0/AN11
RB5/KBI1/PGM
RB6/KBI2/PGC
RB7/KBI3/PGD
Comparator MSSP EUSART 10-bit
ADC
Timer0 Timer1 Timer2 Timer3
CCP2
HLVD
CCP1
BOR Data
EEPROM
W
Instruction Bus <16>
STKPTR Bank
8
State machine
control signals
Decode
8
8
Power-up
Timer
Oscillator
Start-up Timer
Power-on
Reset
Watchdog
Timer
OSC1(3)
OSC2(3)
VDD,
Brown-out
Reset
Internal
Oscillator
Fail-Safe
Clock Monitor
Precision
Reference
Band Gap
VSS
MCLR(2)
Block
INTRC
Oscillator
8 MHz
Oscillator
Single-Supply
Programming
In-Circuit
Debugger
T1OSO
OSC1/CLKI(3)/RA7
T1OSI
PORTE
MCLR/VPP/RE3(2)
© 2007 Microchip Technology Inc. Preliminary DS39631B-page 11
PIC18F2420/2520/4420/4520
FIGURE 1-2: PIC18F4420/4520 (40/44-PIN) BLOCK DIAGRAM
Instruction
Decode and
Control
Data Latch
Data Memory
( 3.9 Kbytes )
Address Latch
Data Address<12>
12
BSR FSR0 Access
FSR1
FSR2
inc/dec
logic
Address
4 12 4
PCH PCL
PCLATH
8
31 Level Stack
Program Counter
PRODH PRODL
8 x 8 Multiply
8
BITOP
8 8
ALU<8>
Address Latch
Program Memory
(16/32 Kbytes)
Data Latch
20
8
8
Table Pointer<21>
inc/dec logic
21
8
Data Bus<8>
Table Latch
8
IR
12
3
ROM Latch
PORTD
RD0/PSP0
PCLATU
PCU
PORTE
MCLR/VPP/RE3(2)
RE2/CS/AN7
RE0/RD/AN5
RE1/WR/AN6
Note 1: CCP2 is multiplexed with RC1 when configuration bit CCP2MX is set, or RB3 when CCP2MX is not set.
2: RE3 is only available when MCLR functionality is disabled.
3: OSC1/CLKI and OSC2/CLKO are only available in select oscillator modes and when these pins are not being used as digital I/O.
Refer to Section 2.0 “Oscillator Configurations” for additional information.
:RD4/PSP4
Comparator MSSP EUSART 10-bit
ADC
Timer0 Timer1 Timer2 Timer3
CCP2
HLVD
ECCP1
BOR Data
EEPROM
W
Instruction Bus <16>
STKPTR Bank
8
State machine
control signals
Decode
8
8
Power-up
Timer
Oscillator
Start-up Timer
Power-on
Reset
Watchdog
Timer
OSC1(3)
OSC2(3)
VDD,
Brown-out
Reset
Internal
Oscillator
Fail-Safe
Clock Monitor
Precision
Reference
Band Gap
VSS
MCLR(2)
Block
INTRC
Oscillator
8 MHz
Oscillator
Single-Supply
Programming
In-Circuit
Debugger
T1OSI
T1OSO
RD5/PSP5/P1B
RD6/PSP6/P1C
RD7/PSP7/P1D
PORTA
PORTB
PORTC
RA4/T0CKI/C1OUT
RA5/AN4/SS/HLVDIN/C2OUT
RB0/INT0/FLT0/AN12
RC0/T1OSO/T13CKI
RC1/T1OSI/CCP2(1)
RC2/CCP1/P1A
RC3/SCK/SCL
RC4/SDI/SDA
RC5/SDO
RC6/TX/CK
RC7/RX/DT
RA3/AN3/VREF+
RA2/AN2/VREF-/CVREF
RA1/AN1
RA0/AN0
RB1/INT1/AN10
RB2/INT2/AN8
RB3/AN9/CCP2(1)
OSC2/CLKO(3)/RA6
RB4/KBI0/AN11
RB5/KBI1/PGM
RB6/KBI2/PGC
RB7/KBI3/PGD
OSC1/CLKI(3)/RA7
PIC18F2420/2520/4420/4520
DS39631B-page 12 Preliminary © 2007 Microchip Technology Inc.
TABLE 1-2: PIC18F2420/2520 PINOUT I/O DESCRIPTIONS
Pin Name
Pin Number
Pin
Type
Buffer
Type
PDIP, Description
SOIC
QFN
MCLR/VPP/RE3
MCLR
VPP
RE3
1 26
I
P
I
ST
ST
Master Clear (input) or programming voltage (input).
Master Clear (Reset) input. This pin is an active-low
Reset to the device.
Programming voltage input.
Digital input.
OSC1/CLKI/RA7
OSC1
CLKI
RA7
9 6
I
I
I/O
ST
CMOS
TTL
Oscillator crystal or external clock input.
Oscillator crystal input or external clock source input.
ST buffer when configured in RC mode; CMOS otherwise.
External clock source input. Always associated with pin
function OSC1. (See related OSC1/CLKI, OSC2/CLKO
pins.)
General purpose I/O pin.
OSC2/CLKO/RA6
OSC2
CLKO
RA6
10 7
O
O
I/O
—
—
TTL
Oscillator crystal or clock output.
Oscillator crystal output. Connects to crystal or
resonator in Crystal Oscillator mode.
In RC mode, OSC2 pin outputs CLKO which has 1/4 the
frequency of OSC1 and denotes the instruction cycle rate.
General purpose I/O pin.
Legend: TTL = TTL compatible input CMOS = CMOS compatible input or output
ST = Schmitt Trigger input with CMOS levels I = Input
O = Output P = Power
Note 1: Default assignment for CCP2 when configuration bit CCP2MX is set.
2: Alternate assignment for CCP2 when configuration bit CCP2MX is cleared.
© 2007 Microchip Technology Inc. Preliminary DS39631B-page 13
PIC18F2420/2520/4420/4520
PORTA is a bidirectional I/O port.
RA0/AN0
RA0
AN0
2 27
I/O
I
TTL
Analog
Digital I/O.
Analog input 0.
RA1/AN1
RA1
AN1
3 28
I/O
I
TTL
Analog
Digital I/O.
Analog input 1.
RA2/AN2/VREF-/CVREF
RA2
AN2
VREFCVREF
4 1
I/O
I
I
O
TTL
Analog
Analog
Analog
Digital I/O.
Analog input 2.
A/D reference voltage (low) input.
Comparator reference voltage output.
RA3/AN3/VREF+
RA3
AN3
VREF+
5 2
I/O
I
I
TTL
Analog
Analog
Digital I/O.
Analog input 3.
A/D reference voltage (high) input.
RA4/T0CKI/C1OUT
RA4
T0CKI
C1OUT
6 3
I/O
I
O
ST
ST
—
Digital I/O.
Timer0 external clock input.
Comparator 1 output.
RA5/AN4/SS/HLVDIN/
C2OUT
RA5
AN4
SS
HLVDIN
C2OUT
7 4
I/O
I
I
I
O
TTL
Analog
TTL
Analog
—
Digital I/O.
Analog input 4.
SPI™ slave select input.
High/Low-Voltage Detect input.
Comparator 2 output.
RA6 See the OSC2/CLKO/RA6 pin.
RA7 See the OSC1/CLKI/RA7 pin.
TABLE 1-2: PIC18F2420/2520 PINOUT I/O DESCRIPTIONS (CONTINUED)
Pin Name
Pin Number
Pin
Type
Buffer
Type
PDIP, Description
SOIC
QFN
Legend: TTL = TTL compatible input CMOS = CMOS compatible input or output
ST = Schmitt Trigger input with CMOS levels I = Input
O = Output P = Power
Note 1: Default assignment for CCP2 when configuration bit CCP2MX is set.
2: Alternate assignment for CCP2 when configuration bit CCP2MX is cleared.
PIC18F2420/2520/4420/4520
DS39631B-page 14 Preliminary © 2007 Microchip Technology Inc.
PORTB is a bidirectional I/O port. PORTB can be software
programmed for internal weak pull-ups on all inputs.
RB0/INT0/FLT0/AN12
RB0
INT0
FLT0
AN12
21 18
I/O
I
I
I
TTL
ST
ST
Analog
Digital I/O.
External interrupt 0.
PWM Fault input for CCP1.
Analog input 12.
RB1/INT1/AN10
RB1
INT1
AN10
22 19
I/O
I
I
TTL
ST
Analog
Digital I/O.
External interrupt 1.
Analog input 10.
RB2/INT2/AN8
RB2
INT2
AN8
23 20
I/O
I
I
TTL
ST
Analog
Digital I/O.
External interrupt 2.
Analog input 8.
RB3/AN9/CCP2
RB3
AN9
CCP2(1)
24 21
I/O
I
I/O
TTL
Analog
ST
Digital I/O.
Analog input 9.
Capture 2 input/Compare 2 output/PWM 2 output.
RB4/KBI0/AN11
RB4
KBI0
AN11
25 22
I/O
I
I
TTL
TTL
Analog
Digital I/O.
Interrupt-on-change pin.
Analog input 11.
RB5/KBI1/PGM
RB5
KBI1
PGM
26 23
I/O
I
I/O
TTL
TTL
ST
Digital I/O.
Interrupt-on-change pin.
Low-Voltage ICSP™ Programming enable pin.
RB6/KBI2/PGC
RB6
KBI2
PGC
27 24
I/O
I
I/O
TTL
TTL
ST
Digital I/O.
Interrupt-on-change pin.
In-Circuit Debugger and ICSP programming clock pin.
RB7/KBI3/PGD
RB7
KBI3
PGD
28 25
I/O
I
I/O
TTL
TTL
ST
Digital I/O.
Interrupt-on-change pin.
In-Circuit Debugger and ICSP programming data pin.
TABLE 1-2: PIC18F2420/2520 PINOUT I/O DESCRIPTIONS (CONTINUED)
Pin Name
Pin Number
Pin
Type
Buffer
Type
PDIP, Description
SOIC
QFN
Legend: TTL = TTL compatible input CMOS = CMOS compatible input or output
ST = Schmitt Trigger input with CMOS levels I = Input
O = Output P = Power
Note 1: Default assignment for CCP2 when configuration bit CCP2MX is set.
2: Alternate assignment for CCP2 when configuration bit CCP2MX is cleared.
© 2007 Microchip Technology Inc. Preliminary DS39631B-page 15
PIC18F2420/2520/4420/4520
PORTC is a bidirectional I/O port.
RC0/T1OSO/T13CKI
RC0
T1OSO
T13CKI
11 8
I/O
O
I
ST
—
ST
Digital I/O.
Timer1 oscillator output.
Timer1/Timer3 external clock input.
RC1/T1OSI/CCP2
RC1
T1OSI
CCP2(2)
12 9
I/O
I
I/O
ST
Analog
ST
Digital I/O.
Timer1 oscillator input.
Capture 2 input/Compare 2 output/PWM 2 output.
RC2/CCP1
RC2
CCP1
13 10
I/O
I/O
ST
ST
Digital I/O.
Capture 1 input/Compare 1 output/PWM 1 output.
RC3/SCK/SCL
RC3
SCK
SCL
14 11
I/O
I/O
I/O
ST
ST
ST
Digital I/O.
Synchronous serial clock input/output for SPI™ mode.
Synchronous serial clock input/output for I2C™ mode.
RC4/SDI/SDA
RC4
SDI
SDA
15 12
I/O
I
I/O
ST
ST
ST
Digital I/O.
SPI data in.
I2C data I/O.
RC5/SDO
RC5
SDO
16 13
I/O
O
ST
—
Digital I/O.
SPI data out.
RC6/TX/CK
RC6
TX
CK
17 14
I/O
O
I/O
ST
—
ST
Digital I/O.
EUSART asynchronous transmit.
EUSART synchronous clock (see related RX/DT).
RC7/RX/DT
RC7
RX
DT
18 15
I/O
I
I/O
ST
ST
ST
Digital I/O.
EUSART asynchronous receive.
EUSART synchronous data (see related TX/CK).
RE3 — — — — See MCLR/VPP/RE3 pin.
VSS 8, 19 5, 16 P — Ground reference for logic and I/O pins.
VDD 20 17 P — Positive supply for logic and I/O pins.
TABLE 1-2: PIC18F2420/2520 PINOUT I/O DESCRIPTIONS (CONTINUED)
Pin Name
Pin Number
Pin
Type
Buffer
Type
PDIP, Description
SOIC
QFN
Legend: TTL = TTL compatible input CMOS = CMOS compatible input or output
ST = Schmitt Trigger input with CMOS levels I = Input
O = Output P = Power
Note 1: Default assignment for CCP2 when configuration bit CCP2MX is set.
2: Alternate assignment for CCP2 when configuration bit CCP2MX is cleared.
PIC18F2420/2520/4420/4520
DS39631B-page 16 Preliminary © 2007 Microchip Technology Inc.
TABLE 1-3: PIC18F4420/4520 PINOUT I/O DESCRIPTIONS
Pin Name
Pin Number Pin
Type
Buffer
Type
Description
PDIP QFN TQFP
MCLR/VPP/RE3
MCLR
VPP
RE3
1 18 18
I
P
I
ST
ST
Master Clear (input) or programming voltage (input).
Master Clear (Reset) input. This pin is an active-low
Reset to the device.
Programming voltage input.
Digital input.
OSC1/CLKI/RA7
OSC1
CLKI
RA7
13 32 30
I
I
I/O
ST
CMOS
TTL
Oscillator crystal or external clock input.
Oscillator crystal input or external clock source input.
ST buffer when configured in RC mode;
analog otherwise.
External clock source input. Always associated with
pin function OSC1. (See related OSC1/CLKI,
OSC2/CLKO pins.)
General purpose I/O pin.
OSC2/CLKO/RA6
OSC2
CLKO
RA6
14 33 31
O
O
I/O
—
—
TTL
Oscillator crystal or clock output.
Oscillator crystal output. Connects to crystal
or resonator in Crystal Oscillator mode.
In RC mode, OSC2 pin outputs CLKO which
has 1/4 the frequency of OSC1 and denotes
the instruction cycle rate.
General purpose I/O pin.
Legend: TTL = TTL compatible input CMOS = CMOS compatible input or output
ST = Schmitt Trigger input with CMOS levels I = Input
O = Output P = Power
Note 1: Default assignment for CCP2 when configuration bit CCP2MX is set.
2: Alternate assignment for CCP2 when configuration bit CCP2MX is cleared.
© 2007 Microchip Technology Inc. Preliminary DS39631B-page 17
PIC18F2420/2520/4420/4520
PORTA is a bidirectional I/O port.
RA0/AN0
RA0
AN0
2 19 19
I/O
I
TTL
Analog
Digital I/O.
Analog input 0.
RA1/AN1
RA1
AN1
3 20 20
I/O
I
TTL
Analog
Digital I/O.
Analog input 1.
RA2/AN2/VREF-/CVREF
RA2
AN2
VREFCVREF
4 21 21
I/O
I
I
O
TTL
Analog
Analog
Analog
Digital I/O.
Analog input 2.
A/D reference voltage (low) input.
Comparator reference voltage output.
RA3/AN3/VREF+
RA3
AN3
VREF+
5 22 22
I/O
I
I
TTL
Analog
Analog
Digital I/O.
Analog input 3.
A/D reference voltage (high) input.
RA4/T0CKI/C1OUT
RA4
T0CKI
C1OUT
6 23 23
I/O
I
O
ST
ST
—
Digital I/O.
Timer0 external clock input.
Comparator 1 output.
RA5/AN4/SS/HLVDIN/
C2OUT
RA5
AN4
SS
HLVDIN
C2OUT
7 24 24
I/O
I
I
I
O
TTL
Analog
TTL
Analog
—
Digital I/O.
Analog input 4.
SPI slave select input.
High/Low-Voltage Detect input.
Comparator 2 output.
RA6 See the OSC2/CLKO/RA6 pin.
RA7 See the OSC1/CLKI/RA7 pin.
TABLE 1-3: PIC18F4420/4520 PINOUT I/O DESCRIPTIONS (CONTINUED)
Pin Name
Pin Number Pin
Type
Buffer
Type
Description
PDIP QFN TQFP
Legend: TTL = TTL compatible input CMOS = CMOS compatible input or output
ST = Schmitt Trigger input with CMOS levels I = Input
O = Output P = Power
Note 1: Default assignment for CCP2 when configuration bit CCP2MX is set.
2: Alternate assignment for CCP2 when configuration bit CCP2MX is cleared.
PIC18F2420/2520/4420/4520
DS39631B-page 18 Preliminary © 2007 Microchip Technology Inc.
PORTB is a bidirectional I/O port. PORTB can be
software programmed for internal weak pull-ups on all
inputs.
RB0/INT0/FLT0/AN12
RB0
INT0
FLT0
AN12
33 9 8
I/O
I
I
I
TTL
ST
ST
Analog
Digital I/O.
External interrupt 0.
PWM Fault input for Enhanced CCP1.
Analog input 12.
RB1/INT1/AN10
RB1
INT1
AN10
34 10 9
I/O
I
I
TTL
ST
Analog
Digital I/O.
External interrupt 1.
Analog input 10.
RB2/INT2/AN8
RB2
INT2
AN8
35 11 10
I/O
I
I
TTL
ST
Analog
Digital I/O.
External interrupt 2.
Analog input 8.
RB3/AN9/CCP2
RB3
AN9
CCP2(1)
36 12 11
I/O
I
I/O
TTL
Analog
ST
Digital I/O.
Analog input 9.
Capture 2 input/Compare 2 output/PWM 2 output.
RB4/KBI0/AN11
RB4
KBI0
AN11
37 14 14
I/O
I
I
TTL
TTL
Analog
Digital I/O.
Interrupt-on-change pin.
Analog input 11.
RB5/KBI1/PGM
RB5
KBI1
PGM
38 15 15
I/O
I
I/O
TTL
TTL
ST
Digital I/O.
Interrupt-on-change pin.
Low-Voltage ICSP™ Programming enable pin.
RB6/KBI2/PGC
RB6
KBI2
PGC
39 16 16
I/O
I
I/O
TTL
TTL
ST
Digital I/O.
Interrupt-on-change pin.
In-Circuit Debugger and ICSP programming
clock pin.
RB7/KBI3/PGD
RB7
KBI3
PGD
40 17 17
I/O
I
I/O
TTL
TTL
ST
Digital I/O.
Interrupt-on-change pin.
In-Circuit Debugger and ICSP programming
data pin.
TABLE 1-3: PIC18F4420/4520 PINOUT I/O DESCRIPTIONS (CONTINUED)
Pin Name
Pin Number Pin
Type
Buffer
Type
Description
PDIP QFN TQFP
Legend: TTL = TTL compatible input CMOS = CMOS compatible input or output
ST = Schmitt Trigger input with CMOS levels I = Input
O = Output P = Power
Note 1: Default assignment for CCP2 when configuration bit CCP2MX is set.
2: Alternate assignment for CCP2 when configuration bit CCP2MX is cleared.
© 2007 Microchip Technology Inc. Preliminary DS39631B-page 19
PIC18F2420/2520/4420/4520
PORTC is a bidirectional I/O port.
RC0/T1OSO/T13CKI
RC0
T1OSO
T13CKI
15 34 32
I/O
O
I
ST
—
ST
Digital I/O.
Timer1 oscillator output.
Timer1/Timer3 external clock input.
RC1/T1OSI/CCP2
RC1
T1OSI
CCP2(2)
16 35 35
I/O
I
I/O
ST
CMOS
ST
Digital I/O.
Timer1 oscillator input.
Capture 2 input/Compare 2 output/PWM 2 output.
RC2/CCP1/P1A
RC2
CCP1
P1A
17 36 36
I/O
I/O
O
ST
ST
—
Digital I/O.
Capture 1 input/Compare 1 output/PWM 1 output.
Enhanced CCP1 output.
RC3/SCK/SCL
RC3
SCK
SCL
18 37 37
I/O
I/O
I/O
ST
ST
ST
Digital I/O.
Synchronous serial clock input/output for
SPI™ mode.
Synchronous serial clock input/output for I2C™ mode.
RC4/SDI/SDA
RC4
SDI
SDA
23 42 42
I/O
I
I/O
ST
ST
ST
Digital I/O.
SPI data in.
I2C data I/O.
RC5/SDO
RC5
SDO
24 43 43
I/O
O
ST
—
Digital I/O.
SPI data out.
RC6/TX/CK
RC6
TX
CK
25 44 44
I/O
O
I/O
ST
—
ST
Digital I/O.
EUSART asynchronous transmit.
EUSART synchronous clock (see related RX/DT).
RC7/RX/DT
RC7
RX
DT
26 1 1
I/O
I
I/O
ST
ST
ST
Digital I/O.
EUSART asynchronous receive.
EUSART synchronous data (see related TX/CK).
TABLE 1-3: PIC18F4420/4520 PINOUT I/O DESCRIPTIONS (CONTINUED)
Pin Name
Pin Number Pin
Type
Buffer
Type
Description
PDIP QFN TQFP
Legend: TTL = TTL compatible input CMOS = CMOS compatible input or output
ST = Schmitt Trigger input with CMOS levels I = Input
O = Output P = Power
Note 1: Default assignment for CCP2 when configuration bit CCP2MX is set.
2: Alternate assignment for CCP2 when configuration bit CCP2MX is cleared.
PIC18F2420/2520/4420/4520
DS39631B-page 20 Preliminary © 2007 Microchip Technology Inc.
PORTD is a bidirectional I/O port or a Parallel Slave
Port (PSP) for interfacing to a microprocessor port.
These pins have TTL input buffers when PSP module
is enabled.
RD0/PSP0
RD0
PSP0
19 38 38
I/O
I/O
ST
TTL
Digital I/O.
Parallel Slave Port data.
RD1/PSP1
RD1
PSP1
20 39 39
I/O
I/O
ST
TTL
Digital I/O.
Parallel Slave Port data.
RD2/PSP2
RD2
PSP2
21 40 40
I/O
I/O
ST
TTL
Digital I/O.
Parallel Slave Port data.
RD3/PSP3
RD3
PSP3
22 41 41
I/O
I/O
ST
TTL
Digital I/O.
Parallel Slave Port data.
RD4/PSP4
RD4
PSP4
27 2 2
I/O
I/O
ST
TTL
Digital I/O.
Parallel Slave Port data.
RD5/PSP5/P1B
RD5
PSP5
P1B
28 3 3
I/O
I/O
O
ST
TTL
—
Digital I/O.
Parallel Slave Port data.
Enhanced CCP1 output.
RD6/PSP6/P1C
RD6
PSP6
P1C
29 4 4
I/O
I/O
O
ST
TTL
—
Digital I/O.
Parallel Slave Port data.
Enhanced CCP1 output.
RD7/PSP7/P1D
RD7
PSP7
P1D
30 5 5
I/O
I/O
O
ST
TTL
—
Digital I/O.
Parallel Slave Port data.
Enhanced CCP1 output.
TABLE 1-3: PIC18F4420/4520 PINOUT I/O DESCRIPTIONS (CONTINUED)
Pin Name
Pin Number Pin
Type
Buffer
Type
Description
PDIP QFN TQFP
Legend: TTL = TTL compatible input CMOS = CMOS compatible input or output
ST = Schmitt Trigger input with CMOS levels I = Input
O = Output P = Power
Note 1: Default assignment for CCP2 when configuration bit CCP2MX is set.
2: Alternate assignment for CCP2 when configuration bit CCP2MX is cleared.
© 2007 Microchip Technology Inc. Preliminary DS39631B-page 21
PIC18F2420/2520/4420/4520
PORTE is a bidirectional I/O port.
RE0/RD/AN5
RE0
RD
AN5
8 25 25
I/O
I
I
ST
TTL
Analog
Digital I/O.
Read control for Parallel Slave Port
(see also WR and CS pins).
Analog input 5.
RE1/WR/AN6
RE1
WR
AN6
9 26 26
I/O
I
I
ST
TTL
Analog
Digital I/O.
Write control for Parallel Slave Port
(see CS and RD pins).
Analog input 6.
RE2/CS/AN7
RE2
CS
AN7
10 27 27
I/O
I
I
ST
TTL
Analog
Digital I/O.
Chip Select control for Parallel Slave Port
(see related RD and WR).
Analog input 7.
RE3 — — — — — See MCLR/VPP/RE3 pin.
VSS 12, 31 6, 30,
31
6, 29 P — Ground reference for logic and I/O pins.
VDD 11, 32 7, 8,
28, 29
7, 28 P — Positive supply for logic and I/O pins.
NC — 13 12, 13,
33, 34
— — No connect.
TABLE 1-3: PIC18F4420/4520 PINOUT I/O DESCRIPTIONS (CONTINUED)
Pin Name
Pin Number Pin
Type
Buffer
Type
Description
PDIP QFN TQFP
Legend: TTL = TTL compatible input CMOS = CMOS compatible input or output
ST = Schmitt Trigger input with CMOS levels I = Input
O = Output P = Power
Note 1: Default assignment for CCP2 when configuration bit CCP2MX is set.
2: Alternate assignment for CCP2 when configuration bit CCP2MX is cleared.
PIC18F2420/2520/4420/4520
DS39631B-page 22 Preliminary © 2007 Microchip Technology Inc.
NOTES:
© 2007 Microchip Technology Inc. Preliminary DS39631B-page 23
PIC18F2420/2520/4420/4520
2.0 OSCILLATOR
CONFIGURATIONS
2.1 Oscillator Types
PIC18F2420/2520/4420/4520 devices can be operated
in ten different oscillator modes. The user can program
the configuration bits, FOSC3:FOSC0, in Configuration
Register 1H to select one of these ten modes:
1. LP Low-Power Crystal
2. XT Crystal/Resonator
3. HS High-Speed Crystal/Resonator
4. HSPLL High-Speed Crystal/Resonator
with PLL enabled
5. RC External Resistor/Capacitor with
FOSC/4 output on RA6
6. RCIO External Resistor/Capacitor with I/O
on RA6
7. INTIO1 Internal Oscillator with FOSC/4 output
on RA6 and I/O on RA7
8. INTIO2 Internal Oscillator with I/O on RA6
and RA7
9. EC External Clock with FOSC/4 output
10. ECIO External Clock with I/O on RA6
2.2 Crystal Oscillator/Ceramic
Resonators
In XT, LP, HS or HSPLL Oscillator modes, a crystal or
ceramic resonator is connected to the OSC1 and
OSC2 pins to establish oscillation. Figure 2-1 shows
the pin connections.
The oscillator design requires the use of a parallel cut
crystal.
FIGURE 2-1: CRYSTAL/CERAMIC
RESONATOR OPERATION
(XT, LP, HS OR HSPLL
CONFIGURATION)
TABLE 2-1: CAPACITOR SELECTION FOR
CERAMIC RESONATORS
Note: Use of a series cut crystal may give a frequency
out of the crystal manufacturer’s
specifications.
Typical Capacitor Values Used:
Mode Freq OSC1 OSC2
XT 3.58 MHz
4.19 MHz
4 MHz
4 MHz
15 pF
15 pF
30 pF
50 pF
15 pF
15 pF
30 pF
50 pF
Capacitor values are for design guidance only.
Different capacitor values may be required to produce
acceptable oscillator operation. The user should test
the performance of the oscillator over the expected
VDD and temperature range for the application.
See the notes following Table 2-2 for additional
information.
Note: When using resonators with frequencies
above 3.5 MHz, the use of HS mode,
rather than XT mode, is recommended.
HS mode may be used at any VDD for
which the controller is rated. If HS is
selected, it is possible that the gain of the
oscillator will overdrive the resonator.
Therefore, a series resistor should be
placed between the OSC2 pin and the
resonator. As a good starting point, the
recommended value of RS is 330Ω.
Note 1: See Table 2-1 and Table 2-2 for initial values of
C1 and C2.
2: A series resistor (RS) may be required for AT
strip cut crystals.
3: RF varies with the oscillator mode chosen.
C1(1)
C2(1)
XTAL
OSC2
OSC1
RF(3)
Sleep
To
Logic
PIC18FXXXX
RS(2)
Internal
PIC18F2420/2520/4420/4520
DS39631B-page 24 Preliminary © 2007 Microchip Technology Inc.
TABLE 2-2: CAPACITOR SELECTION FOR
CRYSTAL OSCILLATOR
An external clock source may also be connected to the
OSC1 pin in the HS mode, as shown in Figure 2-2.
FIGURE 2-2: EXTERNAL CLOCK INPUT
OPERATION (HS OSC
CONFIGURATION)
2.3 External Clock Input
The EC and ECIO Oscillator modes require an external
clock source to be connected to the OSC1 pin. There is
no oscillator start-up time required after a Power-on
Reset or after an exit from Sleep mode.
In the EC Oscillator mode, the oscillator frequency
divided by 4 is available on the OSC2 pin. This signal
may be used for test purposes or to synchronize other
logic. Figure 2-3 shows the pin connections for the EC
Oscillator mode.
FIGURE 2-3: EXTERNAL CLOCK
INPUT OPERATION
(EC CONFIGURATION)
The ECIO Oscillator mode functions like the EC mode,
except that the OSC2 pin becomes an additional general
purpose I/O pin. The I/O pin becomes bit 6 of
PORTA (RA6). Figure 2-4 shows the pin connections
for the ECIO Oscillator mode.
FIGURE 2-4: EXTERNAL CLOCK
INPUT OPERATION
(ECIO CONFIGURATION)
Osc Type
Crystal
Freq
Typical Capacitor Values
Tested:
C1 C2
LP 32 kHz 30 pF 30 pF
XT 1 MHz
4 MHz
15 pF
15 pF
15 pF
15 pF
HS 4 MHz
10 MHz
20 MHz
25 MHz
25 MHz
15 pF
15 pF
15 pF
0 pF
15 pF
15 pF
15 pF
15 pF
5 pF
15 pF
Capacitor values are for design guidance only.
These capacitors were tested with the crystals listed
below for basic start-up and operation. These values
are not optimized.
Different capacitor values may be required to produce
acceptable oscillator operation. The user should test
the performance of the oscillator over the expected
VDD and temperature range for the application.
See the notes following this table for additional
information.
Crystals Used:
32 kHz 4 MHz
25 MHz 10 MHz
1 MHz 20 MHz
Note 1: Higher capacitance increases the stability
of the oscillator but also increases the
start-up time.
2: When operating below 3V VDD, or when
using certain ceramic resonators at any
voltage, it may be necessary to use the
HS mode or switch to a crystal oscillator.
3: Since each resonator/crystal has its own
characteristics, the user should consult
the resonator/crystal manufacturer for
appropriate values of external
components.
4: Rs may be required to avoid overdriving
crystals with low drive level specification.
5: Always verify oscillator performance over
the VDD and temperature range that is
expected for the application.
OSC1
Open OSC2
Clock from
Ext. System PIC18FXXXX
(HS Mode)
OSC1/CLKI
FOSC/4 OSC2/CLKO
Clock from
Ext. System PIC18FXXXX
OSC1/CLKI
RA6 I/O (OSC2)
Clock from
Ext. System PIC18FXXXX
© 2007 Microchip Technology Inc. Preliminary DS39631B-page 25
PIC18F2420/2520/4420/4520
2.4 RC Oscillator
For timing insensitive applications, the “RC” and
“RCIO” device options offer additional cost savings.
The actual oscillator frequency is a function of several
factors:
• supply voltage
• values of the external resistor (REXT) and
capacitor (CEXT)
• operating temperature
Given the same device, operating voltage and temperature
and component values, there will also be unit-to-unit
frequency variations. These are due to factors such as:
• normal manufacturing variation
• difference in lead frame capacitance between
package types (especially for low CEXT values)
• variations within the tolerance of limits of REXT
and CEXT
In the RC Oscillator mode, the oscillator frequency
divided by 4 is available on the OSC2 pin. This signal
may be used for test purposes or to synchronize other
logic. Figure 2-5 shows how the R/C combination is
connected.
FIGURE 2-5: RC OSCILLATOR MODE
The RCIO Oscillator mode (Figure 2-6) functions like
the RC mode, except that the OSC2 pin becomes an
additional general purpose I/O pin. The I/O pin
becomes bit 6 of PORTA (RA6).
FIGURE 2-6: RCIO OSCILLATOR MODE
2.5 PLL Frequency Multiplier
A Phase Locked Loop (PLL) circuit is provided as an
option for users who wish to use a lower frequency
oscillator circuit or to clock the device up to its highest
rated frequency from a crystal oscillator. This may be
useful for customers who are concerned with EMI due
to high-frequency crystals or users who require higher
clock speeds from an internal oscillator.
2.5.1 HSPLL OSCILLATOR MODE
The HSPLL mode makes use of the HS mode oscillator
for frequencies up to 10 MHz. A PLL then multiplies the
oscillator output frequency by 4 to produce an internal
clock frequency up to 40 MHz. The PLLEN bit is not
available in this oscillator mode.
The PLL is only available to the crystal oscillator when
the FOSC3:FOSC0 configuration bits are programmed
for HSPLL mode (= 0110).
FIGURE 2-7: PLL BLOCK DIAGRAM
(HS MODE)
2.5.2 PLL AND INTOSC
The PLL is also available to the internal oscillator block
in selected oscillator modes. In this configuration, the
PLL is enabled in software and generates a clock output
of up to 32 MHz. The operation of INTOSC with the
PLL is described in Section 2.6.4 “PLL in INTOSC
Modes”.
OSC2/CLKO
CEXT
REXT
PIC18FXXXX
OSC1
FOSC/4
Internal
Clock
VDD
VSS
Recommended values: 3 kΩ ≤ REXT ≤ 100 kΩ
CEXT > 20 pF
CEXT
REXT
PIC18FXXXX
OSC1 Internal
Clock
VDD
VSS
Recommended values: 3 kΩ ≤ REXT ≤ 100 kΩ
CEXT > 20 pF
RA6 I/O (OSC2)
MUX
VCO
Loop
Filter
Crystal
Osc
OSC2
OSC1
PLL Enable
FIN
FOUT
SYSCLK
Phase
Comparator
HS Oscillator Enable
÷4
(from Configuration Register 1H)
HS Mode
PIC18F2420/2520/4420/4520
DS39631B-page 26 Preliminary © 2007 Microchip Technology Inc.
2.6 Internal Oscillator Block
The PIC18F2420/2520/4420/4520 devices include an
internal oscillator block which generates two different
clock signals; either can be used as the microcontroller’s
clock source. This may eliminate the need for
external oscillator circuits on the OSC1 and/or OSC2
pins.
The main output (INTOSC) is an 8 MHz clock source,
which can be used to directly drive the device clock. It
also drives a postscaler, which can provide a range of
clock frequencies from 31 kHz to 4 MHz. The INTOSC
output is enabled when a clock frequency from 125 kHz
to 8 MHz is selected.
The other clock source is the internal RC oscillator
(INTRC), which provides a nominal 31 kHz output.
INTRC is enabled if it is selected as the device clock
source; it is also enabled automatically when any of the
following are enabled:
• Power-up Timer
• Fail-Safe Clock Monitor
• Watchdog Timer
• Two-Speed Start-up
These features are discussed in greater detail in
Section 23.0 “Special Features of the CPU”.
The clock source frequency (INTOSC direct, INTRC
direct or INTOSC postscaler) is selected by configuring
the IRCF bits of the OSCCON register (page 30).
2.6.1 INTIO MODES
Using the internal oscillator as the clock source eliminates
the need for up to two external oscillator pins,
which can then be used for digital I/O. Two distinct
configurations are available:
• In INTIO1 mode, the OSC2 pin outputs FOSC/4,
while OSC1 functions as RA7 for digital input and
output.
• In INTIO2 mode, OSC1 functions as RA7 and
OSC2 functions as RA6, both for digital input and
output.
2.6.2 INTOSC OUTPUT FREQUENCY
The internal oscillator block is calibrated at the factory
to produce an INTOSC output frequency of 8.0 MHz.
The INTRC oscillator operates independently of the
INTOSC source. Any changes in INTOSC across
voltage and temperature are not necessarily reflected
by changes in INTRC and vice versa.
2.6.3 OSCTUNE REGISTER
The internal oscillator’s output has been calibrated at
the factory but can be adjusted in the user’s application.
This is done by writing to the OSCTUNE register
(Register 2-1).
When the OSCTUNE register is modified, the INTOSC
frequency will begin shifting to the new frequency. The
INTRC clock will reach the new frequency within
8 clock cycles (approximately 8 * 32 μs = 256 μs). The
INTOSC clock will stabilize within 1 ms. Code execution
continues during this shift. There is no indication
that the shift has occurred.
The OSCTUNE register also implements the INTSRC
and PLLEN bits, which control certain features of the
internal oscillator block. The INTSRC bit allows users
to select which internal oscillator provides the clock
source when the 31 kHz frequency option is selected.
This is covered in greater detail in Section 2.7.1
“Oscillator Control Register”.
The PLLEN bit controls the operation of the frequency
multiplier, PLL, in internal oscillator modes.
2.6.4 PLL IN INTOSC MODES
The 4x frequency multiplier can be used with the internal
oscillator block to produce faster device clock
speeds than are normally possible with an internal
oscillator. When enabled, the PLL produces a clock
speed of up to 32 MHz.
Unlike HSPLL mode, the PLL is controlled through
software. The control bit, PLLEN (OSCTUNE<6>), is
used to enable or disable its operation.
The PLL is available when the device is configured to
use the internal oscillator block as its primary clock
source (FOSC3:FOSC0 = 1001 or 1000). Additionally,
the PLL will only function when the selected output frequency
is either 4 MHz or 8 MHz (OSCCON<6:4> = 111
or 110). If both of these conditions are not met, the PLL
is disabled.
The PLLEN control bit is only functional in those internal
oscillator modes where the PLL is available. In all
other modes, it is forced to ‘0’ and is effectively
unavailable.
2.6.5 INTOSC FREQUENCY DRIFT
The factory calibrates the internal oscillator block
output (INTOSC) for 8 MHz. However, this frequency
may drift as VDD or temperature changes, which can
affect the controller operation in a variety of ways. It is
possible to adjust the INTOSC frequency by modifying
the value in the OSCTUNE register. This has no effect
on the INTRC clock source frequency.
Tuning the INTOSC source requires knowing when to
make the adjustment, in which direction it should be
made and in some cases, how large a change is
needed. Three compensation techniques are discussed
in Section 2.6.5.1 “Compensating with the USART”,
Section 2.6.5.2 “Compensating with the Timers” and
Section 2.6.5.3 “Compensating with the CCP Module
in Capture Mode”, but other techniques may be used.
© 2007 Microchip Technology Inc. Preliminary DS39631B-page 27
PIC18F2420/2520/4420/4520
REGISTER 2-1: OSCTUNE: OSCILLATOR TUNING REGISTER
2.6.5.1 Compensating with the USART
An adjustment may be required when the USART
begins to generate framing errors or receives data with
errors while in Asynchronous mode. Framing errors
indicate that the device clock frequency is too high; to
adjust for this, decrement the value in OSCTUNE to
reduce the clock frequency. On the other hand, errors
in data may suggest that the clock speed is too low; to
compensate, increment OSCTUNE to increase the
clock frequency.
2.6.5.2 Compensating with the Timers
This technique compares device clock speed to some
reference clock. Two timers may be used; one timer is
clocked by the peripheral clock, while the other is
clocked by a fixed reference source, such as the
Timer1 oscillator.
Both timers are cleared, but the timer clocked by the
reference generates interrupts. When an interrupt
occurs, the internally clocked timer is read and both
timers are cleared. If the internally clocked timer value
is greater than expected, then the internal oscillator
block is running too fast. To adjust for this, decrement
the OSCTUNE register.
2.6.5.3 Compensating with the CCP Module
in Capture Mode
A CCP module can use free running Timer1 (or
Timer3), clocked by the internal oscillator block and an
external event with a known period (i.e., AC power frequency).
The time of the first event is captured in the
CCPRxH:CCPRxL registers and is recorded for use
later. When the second event causes a capture, the
time of the first event is subtracted from the time of the
second event. Since the period of the external event is
known, the time difference between events can be
calculated.
If the measured time is much greater than the calculated
time, the internal oscillator block is running too
fast; to compensate, decrement the OSCTUNE register.
If the measured time is much less than the calculated
time, the internal oscillator block is running too slow; to
compensate, increment the OSCTUNE register.
R/W-0 R/W-0(1) U-0 R/W-0 R/W-0 R/W-0 R/W-0 R/W-0
INTSRC PLLEN(1) — TUN4 TUN3 TUN2 TUN1 TUN0
bit 7 bit 0
bit 7 INTSRC: Internal Oscillator Low-Frequency Source Select bit
1 = 31.25 kHz device clock derived from 8 MHz INTOSC source (divide-by-256 enabled)
0 = 31 kHz device clock derived directly from INTRC internal oscillator
bit 6 PLLEN: Frequency Multiplier PLL for INTOSC Enable bit(1)
1 = PLL enabled for INTOSC (4 MHz and 8 MHz only)
0 = PLL disabled
Note 1: Available only in certain oscillator configurations; otherwise, this bit is unavailable
and reads as ‘0’. See Section 2.6.4 “PLL in INTOSC Modes” for details.
bit 5 Unimplemented: Read as ‘0’
bit 4-0 TUN4:TUN0: Frequency Tuning bits
01111 = Maximum frequency
• •
• •
00001
00000 = Center frequency. Oscillator module is running at the calibrated frequency.
11111
• •
• •
10000 = Minimum frequency
Legend:
R = Readable bit W = Writable bit U = Unimplemented bit, read as ‘0’
-n = Value at POR ‘1’ = Bit is set ‘0’ = Bit is cleared x = Bit is unknown
PIC18F2420/2520/4420/4520
DS39631B-page 28 Preliminary © 2007 Microchip Technology Inc.
2.7 Clock Sources and Oscillator
Switching
Like previous PIC18 devices, the PIC18F2420/2520/
4420/4520 family includes a feature that allows the
device clock source to be switched from the main oscillator
to an alternate low-frequency clock source.
PIC18F2420/2520/4420/4520 devices offer two alternate
clock sources. When an alternate clock source is enabled,
the various power managed operating modes are available.
Essentially, there are three clock sources for these
devices:
• Primary oscillators
• Secondary oscillators
• Internal oscillator block
The primary oscillators include the External Crystal
and Resonator modes, the External RC modes, the
External Clock modes and the internal oscillator block.
The particular mode is defined by the FOSC3:FOSC0
configuration bits. The details of these modes are
covered earlier in this chapter.
The secondary oscillators are those external sources
not connected to the OSC1 or OSC2 pins. These
sources may continue to operate even after the
controller is placed in a power managed mode.
PIC18F2420/2520/4420/4520 devices offer the Timer1
oscillator as a secondary oscillator. This oscillator, in all
power managed modes, is often the time base for
functions such as a real-time clock.
Most often, a 32.768 kHz watch crystal is connected
between the RC0/T1OSO/T13CKI and RC1/T1OSI
pins. Like the LP mode oscillator circuit, loading
capacitors are also connected from each pin to ground.
The Timer1 oscillator is discussed in greater detail in
Section 12.3 “Timer1 Oscillator”.
In addition to being a primary clock source, the internal
oscillator block is available as a power managed
mode clock source. The INTRC source is also used as
the clock source for several special features, such as
the WDT and Fail-Safe Clock Monitor.
The clock sources for the PIC18F2420/2520/4420/4520
devices are shown in Figure 2-8. See Section 23.0
“Special Features of the CPU” for Configuration
register details.
FIGURE 2-8: PIC18F2420/2520/4420/4520 CLOCK DIAGRAM
PIC18F2420/2520/4420/4520
4 x PLL
FOSC3:FOSC0
Secondary Oscillator
T1OSCEN
Enable
Oscillator
T1OSO
T1OSI
Clock Source Option
for other Modules
OSC1
OSC2
Sleep HSPLL, INTOSC/PLL
LP, XT, HS, RC, EC
T1OSC
CPU
Peripherals
IDLEN Postscaler
MUX
MUX
8 MHz
4 MHz
2 MHz
1 MHz
500 kHz
125 kHz
250 kHz
OSCCON<6:4>
111
110
101
100
011
010
001
000
31 kHz
INTRC
Source
Internal
Oscillator
Block
WDT, PWRT, FSCM
8 MHz
Internal Oscillator
(INTOSC)
OSCCON<6:4>
Clock
Control
OSCCON<1:0>
Source
8 MHz
31 kHz (INTRC)
OSCTUNE<6>
0
1
OSCTUNE<7>
and Two-Speed Start-up
Primary Oscillator
© 2007 Microchip Technology Inc. Preliminary DS39631B-page 29
PIC18F2420/2520/4420/4520
2.7.1 OSCILLATOR CONTROL REGISTER
The OSCCON register (Register 2-2) controls several
aspects of the device clock’s operation, both in full
power operation and in power managed modes.
The System Clock Select bits, SCS1:SCS0, select the
clock source. The available clock sources are the
primary clock (defined by the FOSC3:FOSC0 configuration
bits), the secondary clock (Timer1 oscillator) and
the internal oscillator block. The clock source changes
immediately after one or more of the bits is written to,
following a brief clock transition interval. The SCS bits
are cleared on all forms of Reset.
The Internal Oscillator Frequency Select bits
(IRCF2:IRCF0) select the frequency output of the
internal oscillator block to drive the device clock. The
choices are the INTRC source, the INTOSC source
(8 MHz) or one of the frequencies derived from the
INTOSC postscaler (31.25 kHz to 4 MHz). If the
internal oscillator block is supplying the device clock,
changing the states of these bits will have an immediate
change on the internal oscillator’s output. On
device Resets, the default output frequency of the
internal oscillator block is set at 1 MHz.
When a nominal output frequency of 31 kHz is selected
(IRCF2:IRCF0 = 000), users may choose which internal
oscillator acts as the source. This is done with the
INTSRC bit in the OSCTUNE register (OSCTUNE<7>).
Setting this bit selects INTOSC as a 31.25 kHz clock
source by enabling the divide-by-256 output of the
INTOSC postscaler. Clearing INTSRC selects INTRC
(nominally 31 kHz) as the clock source.
This option allows users to select the tunable and more
precise INTOSC as a clock source, while maintaining
power savings with a very low clock speed. Regardless
of the setting of INTSRC, INTRC always remains the
clock source for features such as the Watchdog Timer
and the Fail-Safe Clock Monitor.
The OSTS, IOFS and T1RUN bits indicate which clock
source is currently providing the device clock. The
OSTS bit indicates that the Oscillator Start-up Timer
has timed out and the primary clock is providing the
device clock in primary clock modes. The IOFS bit
indicates when the internal oscillator block has stabilized
and is providing the device clock in RC Clock
modes. The T1RUN bit (T1CON<6>) indicates when
the Timer1 oscillator is providing the device clock in
secondary clock modes. In power managed modes,
only one of these three bits will be set at any time. If
none of these bits are set, the INTRC is providing the
clock or the internal oscillator block has just started and
is not yet stable.
The IDLEN bit determines if the device goes into Sleep
mode or one of the Idle modes when the SLEEP
instruction is executed.
The use of the flag and control bits in the OSCCON
register is discussed in more detail in Section 3.0
“Power Managed Modes”.
2.7.2 OSCILLATOR TRANSITIONS
PIC18F2420/2520/4420/4520 devices contain circuitry
to prevent clock “glitches” when switching between
clock sources. A short pause in the device clock occurs
during the clock switch. The length of this pause is the
sum of two cycles of the old clock source and three to
four cycles of the new clock source. This formula
assumes that the new clock source is stable.
Clock transitions are discussed in greater detail in
Section 3.1.2 “Entering Power Managed Modes”.
Note 1: The Timer1 oscillator must be enabled to
select the secondary clock source. The
Timer1 oscillator is enabled by setting the
T1OSCEN bit in the Timer1 Control register
(T1CON<3>). If the Timer1 oscillator
is not enabled, then any attempt to select
a secondary clock source will be ignored.
2: It is recommended that the Timer1
oscillator be operating and stable before
selecting the secondary clock source or a
very long delay may occur while the
Timer1 oscillator starts.
PIC18F2420/2520/4420/4520
DS39631B-page 30 Preliminary © 2007 Microchip Technology Inc.
REGISTER 2-2: OSCCON REGISTER
R/W-0 R/W-1 R/W-0 R/W-0 R(1) R-0 R/W-0 R/W-0
IDLEN IRCF2 IRCF1 IRCF0 OSTS IOFS SCS1 SCS0
bit 7 bit 0
bit 7 IDLEN: Idle Enable bit
1 = Device enters Idle mode on SLEEP instruction
0 = Device enters Sleep mode on SLEEP instruction
bit 6-4 IRCF2:IRCF0: Internal Oscillator Frequency Select bits
111 = 8 MHz (INTOSC drives clock directly)
110 = 4 MHz
101 = 2 MHz
100 = 1 MHz(3)
011 = 500 kHz
010 = 250 kHz
001 = 125 kHz
000 = 31 kHz (from either INTOSC/256 or INTRC directly)(2)
bit 3 OSTS: Oscillator Start-up Time-out Status bit(1)
1 = Oscillator start-up time-out timer has expired; primary oscillator is running
0 = Oscillator start-up time-out timer is running; primary oscillator is not ready
bit 2 IOFS: INTOSC Frequency Stable bit
1 = INTOSC frequency is stable
0 = INTOSC frequency is not stable
bit 1-0 SCS1:SCS0: System Clock Select bits
1x = Internal oscillator block
01 = Secondary (Timer1) oscillator
00 = Primary oscillator
Note 1: Reset state depends on state of the IESO configuration bit.
2: Source selected by the INTSRC bit (OSCTUNE<7>), see text.
3: Default output frequency of INTOSC on Reset.
Legend:
R = Readable bit W = Writable bit U = Unimplemented bit, read as ‘0’
-n = Value at POR ‘1’ = Bit is set ‘0’ = Bit is cleared x = Bit is unknown
© 2007 Microchip Technology Inc. Preliminary DS39631B-page 31
PIC18F2420/2520/4420/4520
2.8 Effects of Power Managed Modes
on the Various Clock Sources
When PRI_IDLE mode is selected, the designated primary
oscillator continues to run without interruption.
For all other power managed modes, the oscillator
using the OSC1 pin is disabled. The OSC1 pin (and
OSC2 pin, if used by the oscillator) will stop oscillating.
In secondary clock modes (SEC_RUN and
SEC_IDLE), the Timer1 oscillator is operating and providing
the device clock. The Timer1 oscillator may also
run in all power managed modes if required to clock
Timer1 or Timer3.
In internal oscillator modes (RC_RUN and RC_IDLE),
the internal oscillator block provides the device clock
source. The 31 kHz INTRC output can be used directly
to provide the clock and may be enabled to support
various special features, regardless of the power
managed mode (see Section 23.2 “Watchdog Timer
(WDT)”, Section 23.3 “Two-Speed Start-up” and
Section 23.4 “Fail-Safe Clock Monitor” for more
information on WDT, Fail-Safe Clock Monitor and Two-
Speed Start-up). The INTOSC output at 8 MHz may be
used directly to clock the device or may be divided
down by the postscaler. The INTOSC output is disabled
if the clock is provided directly from the INTRC output.
If the Sleep mode is selected, all clock sources are
stopped. Since all the transistor switching currents
have been stopped, Sleep mode achieves the lowest
current consumption of the device (only leakage
currents).
Enabling any on-chip feature that will operate during
Sleep will increase the current consumed during Sleep.
The INTRC is required to support WDT operation. The
Timer1 oscillator may be operating to support a realtime
clock. Other features may be operating that do not
require a device clock source (i.e., SSP slave, PSP,
INTn pins and others). Peripherals that may add
significant current consumption are listed in
Section 26.2 “DC Characteristics”.
2.9 Power-up Delays
Power-up delays are controlled by two timers, so that
no external Reset circuitry is required for most applications.
The delays ensure that the device is kept in
Reset until the device power supply is stable under normal
circumstances and the primary clock is operating
and stable. For additional information on power-up
delays, see Section 4.5 “Device Reset Timers”.
The first timer is the Power-up Timer (PWRT), which
provides a fixed delay on power-up (parameter 33,
Table 26-10). It is enabled by clearing (= 0) the
PWRTEN configuration bit.
The second timer is the Oscillator Start-up Timer
(OST), intended to keep the chip in Reset until the
crystal oscillator is stable (LP, XT and HS modes). The
OST does this by counting 1024 oscillator cycles
before allowing the oscillator to clock the device.
When the HSPLL Oscillator mode is selected, the
device is kept in Reset for an additional 2 ms, following
the HS mode OST delay, so the PLL can lock to the
incoming clock frequency.
There is a delay of interval TCSD (parameter 38,
Table 26-10), following POR, while the controller
becomes ready to execute instructions. This delay runs
concurrently with any other delays. This may be the
only delay that occurs when any of the EC, RC or INTIO
modes are used as the primary clock source.
TABLE 2-3: OSC1 AND OSC2 PIN STATES IN SLEEP MODE
OSC Mode OSC1 Pin OSC2 Pin
RC, INTIO1 Floating, external resistor should pull high At logic low (clock/4 output)
RCIO Floating, external resistor should pull high Configured as PORTA, bit 6
INTIO2 Configured as PORTA, bit 7 Configured as PORTA, bit 6
ECIO Floating, pulled by external clock Configured as PORTA, bit 6
EC Floating, pulled by external clock At logic low (clock/4 output)
LP, XT and HS Feedback inverter disabled at quiescent
voltage level
Feedback inverter disabled at quiescent
voltage level
Note: See Table 4-2 in Section 4.0 “Reset” for time-outs due to Sleep and MCLR Reset.
PIC18F2420/2520/4420/4520
DS39631B-page 32 Preliminary © 2007 Microchip Technology Inc.
NOTES:
© 2007 Microchip Technology Inc. DS39631B-page 33
PIC18F2420/2520/4420/4520
3.0 POWER MANAGED MODES
PIC18F2420/2520/4420/4520 devices offer a total of
seven operating modes for more efficient power management.
These modes provide a variety of options for
selective power conservation in applications where
resources may be limited (i.e., battery-powered
devices).
There are three categories of power managed modes:
• Run modes
• Idle modes
• Sleep mode
These categories define which portions of the device
are clocked and sometimes, what speed. The Run and
Idle modes may use any of the three available clock
sources (primary, secondary or internal oscillator
block); the Sleep mode does not use a clock source.
The power managed modes include several powersaving
features offered on previous PIC® devices. One
is the clock switching feature, offered in other PIC18
devices, allowing the controller to use the Timer1 oscillator
in place of the primary oscillator. Also included is
the Sleep mode, offered by all PIC devices, where all
device clocks are stopped.
3.1 Selecting Power Managed Modes
Selecting a power managed mode requires two
decisions: if the CPU is to be clocked or not and the
selection of a clock source. The IDLEN bit
(OSCCON<7>) controls CPU clocking, while the
SCS1:SCS0 bits (OSCCON<1:0>) select the clock
source. The individual modes, bit settings, clock sources
and affected modules are summarized in Table 3-1.
3.1.1 CLOCK SOURCES
The SCS1:SCS0 bits allow the selection of one of three
clock sources for power managed modes. They are:
• the primary clock, as defined by the
FOSC3:FOSC0 configuration bits
• the secondary clock (the Timer1 oscillator)
• the internal oscillator block (for RC modes)
3.1.2 ENTERING POWER MANAGED
MODES
Switching from one power managed mode to another
begins by loading the OSCCON register. The
SCS1:SCS0 bits select the clock source and determine
which Run or Idle mode is to be used. Changing these
bits causes an immediate switch to the new clock
source, assuming that it is running. The switch may
also be subject to clock transition delays. These are
discussed in Section 3.1.3 “Clock Transitions and
Status Indicators” and subsequent sections.
Entry to the Power Managed Idle or Sleep modes is
triggered by the execution of a SLEEP instruction. The
actual mode that results depends on the status of the
IDLEN bit.
Depending on the current mode and the mode being
switched to, a change to a power managed mode does
not always require setting all of these bits. Many
transitions may be done by changing the oscillator select
bits, or changing the IDLEN bit, prior to issuing a SLEEP
instruction. If the IDLEN bit is already configured
correctly, it may only be necessary to perform a SLEEP
instruction to switch to the desired mode.
TABLE 3-1: POWER MANAGED MODES
Mode
OSCCON Bits Module Clocking
IDLEN(1) Available Clock and Oscillator Source
<7>
SCS1:SCS0
<1:0>
CPU Peripherals
Sleep 0 N/A Off Off None – All clocks are disabled
PRI_RUN N/A 00 Clocked Clocked Primary – LP, XT, HS, HSPLL, RC, EC and
Internal Oscillator Block(2).
This is the normal full power execution mode.
SEC_RUN N/A 01 Clocked Clocked Secondary – Timer1 Oscillator
RC_RUN N/A 1x Clocked Clocked Internal Oscillator Block(2)
PRI_IDLE 1 00 Off Clocked Primary – LP, XT, HS, HSPLL, RC, EC
SEC_IDLE 1 01 Off Clocked Secondary – Timer1 Oscillator
RC_IDLE 1 1x Off Clocked Internal Oscillator Block(2)
Note 1: IDLEN reflects its value when the SLEEP instruction is executed.
2: Includes INTOSC and INTOSC postscaler, as well as the INTRC source.
PIC18F2420/2520/4420/4520
DS39631B-page 34 © 2007 Microchip Technology Inc.
3.1.3 CLOCK TRANSITIONS AND STATUS
INDICATORS
The length of the transition between clock sources is
the sum of two cycles of the old clock source and three
to four cycles of the new clock source. This formula
assumes that the new clock source is stable.
Three bits indicate the current clock source and its
status. They are:
• OSTS (OSCCON<3>)
• IOFS (OSCCON<2>)
• T1RUN (T1CON<6>)
In general, only one of these bits will be set while in a
given power managed mode. When the OSTS bit is
set, the primary clock is providing the device clock.
When the IOFS bit is set, the INTOSC output is
providing a stable 8 MHz clock source to a divider that
actually drives the device clock. When the T1RUN bit is
set, the Timer1 oscillator is providing the clock. If none
of these bits are set, then either the INTRC clock
source is clocking the device, or the INTOSC source is
not yet stable.
If the internal oscillator block is configured as the primary
clock source by the FOSC3:FOSC0 configuration
bits, then both the OSTS and IOFS bits may be set
when in PRI_RUN or PRI_IDLE modes. This indicates
that the primary clock (INTOSC output) is generating a
stable 8 MHz output. Entering another RC Power
Managed mode at the same frequency would clear the
OSTS bit.
3.1.4 MULTIPLE SLEEP COMMANDS
The power managed mode that is invoked with the
SLEEP instruction is determined by the setting of the
IDLEN bit at the time the instruction is executed. If
another SLEEP instruction is executed, the device will
enter the power managed mode specified by IDLEN at
that time. If IDLEN has changed, the device will enter
the new power managed mode specified by the new
setting.
3.2 Run Modes
In the Run modes, clocks to both the core and
peripherals are active. The difference between these
modes is the clock source.
3.2.1 PRI_RUN MODE
The PRI_RUN mode is the normal, full power execution
mode of the microcontroller. This is also the default
mode upon a device Reset, unless Two-Speed Start-up
is enabled (see Section 23.3 “Two-Speed Start-up”
for details). In this mode, the OSTS bit is set. The IOFS
bit may be set if the internal oscillator block is the primary
clock source (see Section 2.7.1 “Oscillator
Control Register”).
3.2.2 SEC_RUN MODE
The SEC_RUN mode is the compatible mode to the
“clock switching” feature offered in other PIC18
devices. In this mode, the CPU and peripherals are
clocked from the Timer1 oscillator. This gives users the
option of lower power consumption while still using a
high accuracy clock source.
SEC_RUN mode is entered by setting the SCS1:SCS0
bits to ‘01’. The device clock source is switched to the
Timer1 oscillator (see Figure 3-1), the primary oscillator
is shut down, the T1RUN bit (T1CON<6>) is set and
the OSTS bit is cleared.
On transitions from SEC_RUN mode to PRI_RUN, the
peripherals and CPU continue to be clocked from the
Timer1 oscillator while the primary clock is started.
When the primary clock becomes ready, a clock switch
back to the primary clock occurs (see Figure 3-2).
When the clock switch is complete, the T1RUN bit is
cleared, the OSTS bit is set and the primary clock is
providing the clock. The IDLEN and SCS bits are not
affected by the wake-up; the Timer1 oscillator
continues to run.
Note 1: Caution should be used when modifying a
single IRCF bit. If VDD is less than 3V, it is
possible to select a higher clock speed
than is supported by the low VDD.
Improper device operation may result if
the VDD/FOSC specifications are violated.
2: Executing a SLEEP instruction does not
necessarily place the device into Sleep
mode. It acts as the trigger to place the
controller into either the Sleep mode or
one of the Idle modes, depending on the
setting of the IDLEN bit.
Note: The Timer1 oscillator should already be
running prior to entering SEC_RUN mode.
If the T1OSCEN bit is not set when the
SCS1:SCS0 bits are set to ‘01’, entry to
SEC_RUN mode will not occur. If the
Timer1 oscillator is enabled, but not yet
running, device clocks will be delayed until
the oscillator has started; in such situations,
initial oscillator operation is far from
stable and unpredictable operation may
result.
© 2007 Microchip Technology Inc. DS39631B-page 35
PIC18F2420/2520/4420/4520
FIGURE 3-1: TRANSITION TIMING FOR ENTRY TO SEC_RUN MODE
FIGURE 3-2: TRANSITION TIMING FROM SEC_RUN MODE TO PRI_RUN MODE (HSPLL)
3.2.3 RC_RUN MODE
In RC_RUN mode, the CPU and peripherals are
clocked from the internal oscillator block using the
INTOSC multiplexer. In this mode, the primary clock is
shut down. When using the INTRC source, this mode
provides the best power conservation of all the Run
modes, while still executing code. It works well for user
applications which are not highly timing sensitive or do
not require high-speed clocks at all times.
If the primary clock source is the internal oscillator
block (either INTRC or INTOSC), there are no distinguishable
differences between PRI_RUN and
RC_RUN modes during execution. However, a clock
switch delay will occur during entry to and exit from
RC_RUN mode. Therefore, if the primary clock source
is the internal oscillator block, the use of RC_RUN
mode is not recommended.
This mode is entered by setting the SCS1 bit to ‘1’.
Although it is ignored, it is recommended that the SCS0
bit also be cleared; this is to maintain software compatibility
with future devices. When the clock source is
switched to the INTOSC multiplexer (see Figure 3-3),
the primary oscillator is shut down and the OSTS bit is
cleared. The IRCF bits may be modified at any time to
immediately change the clock speed.
Q2 Q3 Q4
OSC1
Peripheral
Program
Q1
T1OSI
Q1
Counter
Clock
CPU
Clock
PC PC + 2
1 2 3 n-1 n
Clock Transition(1)
Q2 Q3 Q4 Q1 Q2 Q3
PC + 4
Note 1: Clock transition typically occurs within 2-4 TOSC.
Q1 Q3 Q4
OSC1
Peripheral
Program PC
T1OSI
PLL Clock
Q1
PC + 4
Q2
Output
Q3 Q4 Q1
CPU Clock
PC + 2
Clock
Counter
Q2 Q2 Q3
Note1: TOST = 1024 TOSC; TPLL = 2 ms (approx). These intervals are not shown to scale.
2: Clock transition typically occurs within 2-4 TOSC.
SCS1:SCS0 bits changed
TPLL(1)
1 2 n-1 n
Clock
OSTS bit set
Transition(2)
TOST(1)
Note: Caution should be used when modifying a
single IRCF bit. If VDD is less than 3V, it is
possible to select a higher clock speed
than is supported by the low VDD.
Improper device operation may result if
the VDD/FOSC specifications are violated.
PIC18F2420/2520/4420/4520
DS39631B-page 36 © 2007 Microchip Technology Inc.
If the IRCF bits and the INTSRC bit are all clear, the
INTOSC output is not enabled and the IOFS bit will
remain clear; there will be no indication of the current
clock source. The INTRC source is providing the
device clocks.
If the IRCF bits are changed from all clear (thus,
enabling the INTOSC output) or if INTSRC is set, the
IOFS bit becomes set after the INTOSC output
becomes stable. Clocks to the device continue while
the INTOSC source stabilizes after an interval of
TIOBST.
If the IRCF bits were previously at a non-zero value, or
if INTSRC was set before setting SCS1 and the
INTOSC source was already stable, the IOFS bit will
remain set.
On transitions from RC_RUN mode to PRI_RUN mode,
the device continues to be clocked from the INTOSC
multiplexer while the primary clock is started. When the
primary clock becomes ready, a clock switch to the primary
clock occurs (see Figure 3-4). When the clock
switch is complete, the IOFS bit is cleared, the OSTS
bit is set and the primary clock is providing the device
clock. The IDLEN and SCS bits are not affected by the
switch. The INTRC source will continue to run if either
the WDT or the Fail-Safe Clock Monitor is enabled.
FIGURE 3-3: TRANSITION TIMING TO RC_RUN MODE
FIGURE 3-4: TRANSITION TIMING FROM RC_RUN MODE TO PRI_RUN MODE
Q2 Q3 Q4
OSC1
Peripheral
Program
Q1
INTRC
Q1
Counter
Clock
CPU
Clock
PC PC + 2
1 2 3 n-1 n
Clock Transition(1)
Q2 Q3 Q4 Q1 Q2 Q3
PC + 4
Note 1: Clock transition typically occurs within 2-4 TOSC.
Q1 Q3 Q4
OSC1
Peripheral
Program PC
INTOSC
PLL Clock
Q1
PC + 4
Q2
Output
Q3 Q4 Q1
CPU Clock
PC + 2
Clock
Counter
Q2 Q2 Q3
Note1: TOST = 1024 TOSC; TPLL = 2 ms (approx). These intervals are not shown to scale.
2: Clock transition typically occurs within 2-4 TOSC.
SCS1:SCS0 bits changed
TPLL(1)
1 2 n-1 n
Clock
OSTS bit set
Transition(2)
Multiplexer
TOST(1)
© 2007 Microchip Technology Inc. DS39631B-page 37
PIC18F2420/2520/4420/4520
3.3 Sleep Mode
The Power Managed Sleep mode in the PIC18F2420/
2520/4420/4520 devices is identical to the legacy
Sleep mode offered in all other PIC devices. It is
entered by clearing the IDLEN bit (the default state on
device Reset) and executing the SLEEP instruction.
This shuts down the selected oscillator (Figure 3-5). All
clock source status bits are cleared.
Entering the Sleep mode from any other mode does not
require a clock switch. This is because no clocks are
needed once the controller has entered Sleep. If the
WDT is selected, the INTRC source will continue to
operate. If the Timer1 oscillator is enabled, it will also
continue to run.
When a wake event occurs in Sleep mode (by interrupt,
Reset or WDT time-out), the device will not be clocked
until the clock source selected by the SCS1:SCS0 bits
becomes ready (see Figure 3-6), or it will be clocked
from the internal oscillator block if either the Two-
Speed Start-up or the Fail-Safe Clock Monitor are
enabled (see Section 23.0 “Special Features of the
CPU”). In either case, the OSTS bit is set when the
primary clock is providing the device clocks. The
IDLEN and SCS bits are not affected by the wake-up.
3.4 Idle Modes
The Idle modes allow the controller’s CPU to be
selectively shut down while the peripherals continue to
operate. Selecting a particular Idle mode allows users
to further manage power consumption.
If the IDLEN bit is set to a ‘1’ when a SLEEP instruction is
executed, the peripherals will be clocked from the clock
source selected using the SCS1:SCS0 bits; however, the
CPU will not be clocked. The clock source status bits are
not affected. Setting IDLEN and executing a SLEEP
instruction provides a quick method of switching from a
given Run mode to its corresponding Idle mode.
If the WDT is selected, the INTRC source will continue
to operate. If the Timer1 oscillator is enabled, it will also
continue to run.
Since the CPU is not executing instructions, the only
exits from any of the Idle modes are by interrupt, WDT
time-out or a Reset. When a wake event occurs, CPU
execution is delayed by an interval of TCSD
(parameter 38, Table 26-10) while it becomes ready to
execute code. When the CPU begins executing code,
it resumes with the same clock source for the current
Idle mode. For example, when waking from RC_IDLE
mode, the internal oscillator block will clock the CPU
and peripherals (in other words, RC_RUN mode). The
IDLEN and SCS bits are not affected by the wake-up.
While in any Idle mode or the Sleep mode, a WDT
time-out will result in a WDT wake-up to the Run mode
currently specified by the SCS1:SCS0 bits.
FIGURE 3-5: TRANSITION TIMING FOR ENTRY TO SLEEP MODE
FIGURE 3-6: TRANSITION TIMING FOR WAKE FROM SLEEP (HSPLL)
Q2 Q3 Q4
OSC1
Peripheral
Sleep
Program
Q1 Q1
Counter
Clock
CPU
Clock
PC PC + 2
Q3 Q4 Q1 Q2
OSC1
Peripheral
Program PC
PLL Clock
Q3 Q4
Output
CPU Clock
Q1 Q2 Q3 Q4 Q1 Q2
Clock
Counter PC + 6 PC + 4
Q1 Q2 Q3 Q4
Wake Event
Note1: TOST = 1024 TOSC; TPLL = 2 ms (approx). These intervals are not shown to scale.
TOST(1) TPLL(1)
OSTS bit set
PC + 2
PIC18F2420/2520/4420/4520
DS39631B-page 38 © 2007 Microchip Technology Inc.
3.4.1 PRI_IDLE MODE
This mode is unique among the three Low-Power Idle
modes, in that it does not disable the primary device
clock. For timing sensitive applications, this allows for
the fastest resumption of device operation with its more
accurate primary clock source, since the clock source
does not have to “warm-up” or transition from another
oscillator.
PRI_IDLE mode is entered from PRI_RUN mode by
setting the IDLEN bit and executing a SLEEP instruction.
If the device is in another Run mode, set IDLEN
first, then clear the SCS bits and execute SLEEP.
Although the CPU is disabled, the peripherals continue
to be clocked from the primary clock source specified
by the FOSC3:FOSC0 configuration bits. The OSTS bit
remains set (see Figure 3-7).
When a wake event occurs, the CPU is clocked from the
primary clock source. A delay of interval TCSD is
required between the wake event and when code
execution starts. This is required to allow the CPU to
become ready to execute instructions. After the wakeup,
the OSTS bit remains set. The IDLEN and SCS bits
are not affected by the wake-up (see Figure 3-8).
3.4.2 SEC_IDLE MODE
In SEC_IDLE mode, the CPU is disabled but the
peripherals continue to be clocked from the Timer1
oscillator. This mode is entered from SEC_RUN by setting
the IDLEN bit and executing a SLEEP instruction. If
the device is in another Run mode, set the IDLEN bit
first, then set the SCS1:SCS0 bits to ‘01’ and execute
SLEEP. When the clock source is switched to the
Timer1 oscillator, the primary oscillator is shut down,
the OSTS bit is cleared and the T1RUN bit is set.
When a wake event occurs, the peripherals continue to
be clocked from the Timer1 oscillator. After an interval
of TCSD following the wake event, the CPU begins executing
code being clocked by the Timer1 oscillator. The
IDLEN and SCS bits are not affected by the wake-up;
the Timer1 oscillator continues to run (see Figure 3-8).
FIGURE 3-7: TRANSITION TIMING FOR ENTRY TO IDLE MODE
FIGURE 3-8: TRANSITION TIMING FOR WAKE FROM IDLE TO RUN MODE
Note: The Timer1 oscillator should already be
running prior to entering SEC_IDLE mode.
If the T1OSCEN bit is not set when the
SLEEP instruction is executed, the SLEEP
instruction will be ignored and entry to
SEC_IDLE mode will not occur. If the
Timer1 oscillator is enabled but not yet
running, peripheral clocks will be delayed
until the oscillator has started. In such situations,
initial oscillator operation is far
from stable and unpredictable operation
may result.
Q1
Peripheral
Program PC PC + 2
OSC1
Q3 Q4 Q1
CPU Clock
Clock
Counter
Q2
OSC1
Peripheral
Program PC
CPU Clock
Q1 Q3 Q4
Clock
Counter
Q2
Wake Event
TCSD
© 2007 Microchip Technology Inc. DS39631B-page 39
PIC18F2420/2520/4420/4520
3.4.3 RC_IDLE MODE
In RC_IDLE mode, the CPU is disabled but the peripherals
continue to be clocked from the internal oscillator
block using the INTOSC multiplexer. This mode allows
for controllable power conservation during Idle periods.
From RC_RUN, this mode is entered by setting the
IDLEN bit and executing a SLEEP instruction. If the
device is in another Run mode, first set IDLEN, then set
the SCS1 bit and execute SLEEP. Although its value is
ignored, it is recommended that SCS0 also be cleared;
this is to maintain software compatibility with future
devices. The INTOSC multiplexer may be used to
select a higher clock frequency by modifying the IRCF
bits before executing the SLEEP instruction. When the
clock source is switched to the INTOSC multiplexer, the
primary oscillator is shut down and the OSTS bit is
cleared.
If the IRCF bits are set to any non-zero value, or the
INTSRC bit is set, the INTOSC output is enabled. The
IOFS bit becomes set, after the INTOSC output
becomes stable, after an interval of TIOBST
(parameter 39, Table 26-10). Clocks to the peripherals
continue while the INTOSC source stabilizes. If the
IRCF bits were previously at a non-zero value, or
INTSRC was set before the SLEEP instruction was executed
and the INTOSC source was already stable, the
IOFS bit will remain set. If the IRCF bits and INTSRC
are all clear, the INTOSC output will not be enabled, the
IOFS bit will remain clear and there will be no indication
of the current clock source.
When a wake event occurs, the peripherals continue to
be clocked from the INTOSC multiplexer. After a delay
of TCSD following the wake event, the CPU begins executing
code being clocked by the INTOSC multiplexer.
The IDLEN and SCS bits are not affected by the wakeup.
The INTRC source will continue to run if either the
WDT or the Fail-Safe Clock Monitor is enabled.
3.5 Exiting Idle and Sleep Modes
An exit from Sleep mode or any of the Idle modes is
triggered by an interrupt, a Reset or a WDT time-out.
This section discusses the triggers that cause exits
from power managed modes. The clocking subsystem
actions are discussed in each of the power managed
modes (see Section 3.2 “Run Modes”, Section 3.3
“Sleep Mode” and Section 3.4 “Idle Modes”).
3.5.1 EXIT BY INTERRUPT
Any of the available interrupt sources can cause the
device to exit from an Idle mode or the Sleep mode to
a Run mode. To enable this functionality, an interrupt
source must be enabled by setting its enable bit in one
of the INTCON or PIE registers. The exit sequence is
initiated when the corresponding interrupt flag bit is set.
On all exits from Idle or Sleep modes by interrupt, code
execution branches to the interrupt vector if the GIE/
GIEH bit (INTCON<7>) is set. Otherwise, code execution
continues or resumes without branching (see
Section 9.0 “Interrupts”).
A fixed delay of interval TCSD following the wake event
is required when leaving Sleep and Idle modes. This
delay is required for the CPU to prepare for execution.
Instruction execution resumes on the first clock cycle
following this delay.
3.5.2 EXIT BY WDT TIME-OUT
A WDT time-out will cause different actions depending
on which power managed mode the device is in when
the time-out occurs.
If the device is not executing code (all Idle modes and
Sleep mode), the time-out will result in an exit from the
power managed mode (see Section 3.2 “Run Modes”
and Section 3.3 “Sleep Mode”). If the device is executing
code (all Run modes), the time-out will result in
a WDT Reset (see Section 23.2 “Watchdog Timer
(WDT)”).
The WDT timer and postscaler are cleared by
executing a SLEEP or CLRWDT instruction, the loss of a
currently selected clock source (if the Fail-Safe Clock
Monitor is enabled) and modifying the IRCF bits in the
OSCCON register if the internal oscillator block is the
device clock source.
3.5.3 EXIT BY RESET
Normally, the device is held in Reset by the Oscillator
Start-up Timer (OST) until the primary clock becomes
ready. At that time, the OSTS bit is set and the device
begins executing code. If the internal oscillator block is
the new clock source, the IOFS bit is set instead.
The exit delay time from Reset to the start of code
execution depends on both the clock sources before
and after the wake-up and the type of oscillator if the
new clock source is the primary clock. Exit delays are
summarized in Table 3-2.
Code execution can begin before the primary clock
becomes ready. If either the Two-Speed Start-up (see
Section 23.3 “Two-Speed Start-up”) or Fail-Safe
Clock Monitor (see Section 23.4 “Fail-Safe Clock
Monitor”) is enabled, the device may begin execution
as soon as the Reset source has cleared. Execution is
clocked by the INTOSC multiplexer driven by the internal
oscillator block. Execution is clocked by the internal
oscillator block until either the primary clock becomes
ready or a power managed mode is entered before the
primary clock becomes ready; the primary clock is then
shut down.
PIC18F2420/2520/4420/4520
DS39631B-page 40 © 2007 Microchip Technology Inc.
3.5.4 EXIT WITHOUT AN OSCILLATOR
START-UP DELAY
Certain exits from power managed modes do not
invoke the OST at all. There are two cases:
• PRI_IDLE mode, where the primary clock source
is not stopped and
• the primary clock source is not any of the LP, XT,
HS or HSPLL modes.
In these instances, the primary clock source either
does not require an oscillator start-up delay since it is
already running (PRI_IDLE), or normally does not
require an oscillator start-up delay (RC, EC and INTIO
Oscillator modes). However, a fixed delay of interval
TCSD following the wake event is still required when
leaving Sleep and Idle modes to allow the CPU to
prepare for execution. Instruction execution resumes
on the first clock cycle following this delay.
TABLE 3-2: EXIT DELAY ON WAKE-UP BY RESET FROM SLEEP MODE OR ANY IDLE MODE
(BY CLOCK SOURCES)
Clock Source
before Wake-up
Clock Source
after Wake-up
Exit Delay
Clock Ready Status
Bit (OSCCON)
Primary Device Clock
(PRI_IDLE mode)
LP, XT, HS
TCSD HSPLL (1) OSTS
EC, RC
INTOSC(2) IOFS
T1OSC or INTRC(1)
LP, XT, HS TOST(3)
HSPLL TOST + trc OSTS
(3)
EC, RC TCSD(1)
INTOSC(1) TIOBST(4) IOFS
INTOSC(2)
LP, XT, HS TOST(4)
HSPLL TOST + trc OSTS
(3)
EC, RC TCSD(1)
INTOSC(1) None IOFS
None
(Sleep mode)
LP, XT, HS TOST(3)
HSPLL TOST + trc OSTS
(3)
EC, RC TCSD(1)
INTOSC(1) TIOBST(4) IOFS
Note 1: TCSD (parameter 38) is a required delay when waking from Sleep and all Idle modes and runs concurrently
with any other required delays (see Section 3.4 “Idle Modes”). On Reset, INTOSC defaults to 1 MHz.
2: Includes both the INTOSC 8 MHz source and postscaler derived frequencies.
3: TOST is the Oscillator Start-up Timer (parameter 32). trc is the PLL Lock-out Timer (parameter F12); it is
also designated as TPLL.
4: Execution continues during TIOBST (parameter 39), the INTOSC stabilization period.
© 2007 Microchip Technology Inc. Preliminary DS39631B-page 41
PIC18F2420/2520/4420/4520
4.0 RESET
The PIC18F2420/2520/4420/4520 devices differentiate
between various kinds of Reset:
a) Power-on Reset (POR)
b) MCLR Reset during normal operation
c) MCLR Reset during power managed modes
d) Watchdog Timer (WDT) Reset (during
execution)
e) Programmable Brown-out Reset (BOR)
f) RESET Instruction
g) Stack Full Reset
h) Stack Underflow Reset
This section discusses Resets generated by MCLR,
POR and BOR and covers the operation of the various
start-up timers. Stack Reset events are covered in
Section 5.1.2.4 “Stack Full and Underflow Resets”.
WDT Resets are covered in Section 23.2 “Watchdog
Timer (WDT)”.
A simplified block diagram of the On-Chip Reset Circuit
is shown in Figure 4-1.
4.1 RCON Register
Device Reset events are tracked through the RCON
register (Register 4-1). The lower five bits of the register
indicate that a specific Reset event has occurred. In
most cases, these bits can only be cleared by the event
and must be set by the application after the event. The
state of these flag bits, taken together, can be read to
indicate the type of Reset that just occurred. This is
described in more detail in Section 4.6 “Reset State
of Registers”.
The RCON register also has control bits for setting
interrupt priority (IPEN) and software control of the
BOR (SBOREN). Interrupt priority is discussed in
Section 9.0 “Interrupts”. BOR is covered in
Section 4.4 “Brown-out Reset (BOR)”.
FIGURE 4-1: SIMPLIFIED BLOCK DIAGRAM OF ON-CHIP RESET CIRCUIT
External Reset
MCLR
VDD
OSC1
WDT
Time-out
VDD Rise
Detect
OST/PWRT
INTRC(1)
POR Pulse
OST
10-bit Ripple Counter
PWRT
11-bit Ripple Counter
Enable OST(2)
Enable PWRT
Note 1: This is the INTRC source from the internal oscillator block and is separate from the RC oscillator of the CLKI pin.
2: See Table 4-2 for time-out situations.
Brown-out
Reset
BOREN
RESET
Instruction
Stack
Pointer
Stack Full/Underflow Reset
Sleep
( )_IDLE
1024 Cycles
32 μs 65.5 ms
MCLRE
S
R Q
Chip_Reset
PIC18F2420/2520/4420/4520
DS39631B-page 42 Preliminary © 2007 Microchip Technology Inc.
REGISTER 4-1: RCON REGISTER
R/W-0 R/W-1(1) U-0 R/W-1 R-1 R-1 R/W-0(2) R/W-0
IPEN SBOREN — RI TO PD POR BOR
bit 7 bit 0
bit 7 IPEN: Interrupt Priority Enable bit
1 = Enable priority levels on interrupts
0 = Disable priority levels on interrupts (PIC16CXXX Compatibility mode)
bit 6 SBOREN: BOR Software Enable bit(1)
If BOREN1:BOREN0 = 01:
1 = BOR is enabled
0 = BOR is disabled
If BOREN1:BOREN0 = 00, 10 or 11:
Bit is disabled and read as ‘0’.
bit 5 Unimplemented: Read as ‘0’
bit 4 RI: RESET Instruction Flag bit
1 = The RESET instruction was not executed (set by firmware only)
0 = The RESET instruction was executed causing a device Reset (must be set in software after
a Brown-out Reset occurs)
bit 3 TO: Watchdog Time-out Flag bit
1 = Set by power-up, CLRWDT instruction or SLEEP instruction
0 = A WDT time-out occurred
bit 2 PD: Power-down Detection Flag bit
1 = Set by power-up or by the CLRWDT instruction
0 = Set by execution of the SLEEP instruction
bit 1 POR: Power-on Reset Status bit(2)
1 = A Power-on Reset has not occurred (set by firmware only)
0 = A Power-on Reset occurred (must be set in software after a Power-on Reset occurs)
bit 0 BOR: Brown-out Reset Status bit
1 = A Brown-out Reset has not occurred (set by firmware only)
0 = A Brown-out Reset occurred (must be set in software after a Brown-out Reset occurs)
Note 1: If SBOREN is enabled, its Reset state is ‘1’; otherwise, it is ‘0’.
2: The actual Reset value of POR is determined by the type of device Reset. See the
notes following this register and Section 4.6 “Reset State of Registers” for
additional information.
Legend:
R = Readable bit W = Writable bit U = Unimplemented bit, read as ‘0’
-n = Value at POR ‘1’ = Bit is set ‘0’ = Bit is cleared x = Bit is unknown
Note 1: It is recommended that the POR bit be set after a Power-on Reset has been
detected so that subsequent Power-on Resets may be detected.
2: Brown-out Reset is said to have occurred when BOR is ‘0’ and POR is ‘1’ (assuming
that POR was set to ‘1’ by software immediately after POR).
© 2007 Microchip Technology Inc. Preliminary DS39631B-page 43
PIC18F2420/2520/4420/4520
4.2 Master Clear (MCLR)
The MCLR pin provides a method for triggering an
external Reset of the device. A Reset is generated by
holding the pin low. These devices have a noise filter in
the MCLR Reset path which detects and ignores small
pulses.
The MCLR pin is not driven low by any internal Resets,
including the WDT.
In PIC18F2420/2520/4420/4520 devices, the MCLR
input can be disabled with the MCLRE configuration bit.
When MCLR is disabled, the pin becomes a digital
input. See Section 10.5 “PORTE, TRISE and LATE
Registers” for more information.
4.3 Power-on Reset (POR)
A Power-on Reset pulse is generated on-chip
whenever VDD rises above a certain threshold. This
allows the device to start in the initialized state when
VDD is adequate for operation.
To take advantage of the POR circuitry, tie the MCLR
pin through a resistor (1 kΩ to 10 kΩ) to VDD. This will
eliminate external RC components usually needed to
create a Power-on Reset delay. A minimum rise rate for
VDD is specified (parameter D004). For a slow rise
time, see Figure 4-2.
When the device starts normal operation (i.e., exits the
Reset condition), device operating parameters (voltage,
frequency, temperature, etc.) must be met to
ensure operation. If these conditions are not met, the
device must be held in Reset until the operating
conditions are met.
POR events are captured by the POR bit (RCON<1>).
The state of the bit is set to ‘0’ whenever a POR occurs;
it does not change for any other Reset event. POR is
not reset to ‘1’ by any hardware event. To capture
multiple events, the user manually resets the bit to ‘1’
in software following any POR.
FIGURE 4-2: EXTERNAL POWER-ON
RESET CIRCUIT (FOR
SLOW VDD POWER-UP)
Note 1: External Power-on Reset circuit is required
only if the VDD power-up slope is too slow.
The diode D helps discharge the capacitor
quickly when VDD powers down.
2: R < 40 kΩ is recommended to make sure that
the voltage drop across R does not violate
the device’s electrical specification.
3: R1 ≥ 1 kΩ will limit any current flowing into
MCLR from external capacitor C, in the event
of MCLR/VPP pin breakdown, due to
Electrostatic Discharge (ESD) or Electrical
Overstress (EOS).
C
R1
D R
VDD
MCLR
PIC18FXXXX
VDD
PIC18F2420/2520/4420/4520
DS39631B-page 44 Preliminary © 2007 Microchip Technology Inc.
4.4 Brown-out Reset (BOR)
PIC18F2420/2520/4420/4520 devices implement a
BOR circuit that provides the user with a number of
configuration and power-saving options. The BOR is
controlled by the BORV1:BORV0 and
BOREN1:BOREN0 configuration bits. There are a total
of four BOR configurations which are summarized in
Table 4-1.
The BOR threshold is set by the BORV1:BORV0 bits. If
BOR is enabled (any values of BOREN1:BOREN0,
except ‘00’), any drop of VDD below VBOR (parameter
D005) for greater than TBOR (parameter 35) will reset
the device. A Reset may or may not occur if VDD falls
below VBOR for less than TBOR. The chip will remain in
Brown-out Reset until VDD rises above VBOR.
If the Power-up Timer is enabled, it will be invoked after
VDD rises above VBOR; it then will keep the chip in
Reset for an additional time delay, TPWRT
(parameter 33). If VDD drops below VBOR while the
Power-up Timer is running, the chip will go back into a
Brown-out Reset and the Power-up Timer will be
initialized. Once VDD rises above VBOR, the Power-up
Timer will execute the additional time delay.
BOR and the Power-on Timer (PWRT) are
independently configured. Enabling BOR Reset does
not automatically enable the PWRT.
4.4.1 SOFTWARE ENABLED BOR
When BOREN1:BOREN0 = 01, the BOR can be
enabled or disabled by the user in software. This is
done with the control bit, SBOREN (RCON<6>).
Setting SBOREN enables the BOR to function as
previously described. Clearing SBOREN disables the
BOR entirely. The SBOREN bit operates only in this
mode; otherwise it is read as ‘0’.
Placing the BOR under software control gives the user
the additional flexibility of tailoring the application to its
environment without having to reprogram the device to
change BOR configuration. It also allows the user to
tailor device power consumption in software by eliminating
the incremental current that the BOR consumes.
While the BOR current is typically very small, it may
have some impact in low-power applications.
4.4.2 DETECTING BOR
When BOR is enabled, the BOR bit always resets to ‘0’
on any BOR or POR event. This makes it difficult to
determine if a BOR event has occurred just by reading
the state of BOR alone. A more reliable method is to
simultaneously check the state of both POR and BOR.
This assumes that the POR bit is reset to ‘1’ in software
immediately after any POR event. If BOR is ‘0’ while
POR is ‘1’, it can be reliably assumed that a BOR event
has occurred.
4.4.3 DISABLING BOR IN SLEEP MODE
When BOREN1:BOREN0 = 10, the BOR remains
under hardware control and operates as previously
described. Whenever the device enters Sleep mode,
however, the BOR is automatically disabled. When the
device returns to any other operating mode, BOR is
automatically re-enabled.
This mode allows for applications to recover from
brown-out situations, while actively executing code,
when the device requires BOR protection the most. At
the same time, it saves additional power in Sleep mode
by eliminating the small incremental BOR current.
TABLE 4-1: BOR CONFIGURATIONS
Note: Even when BOR is under software control,
the BOR Reset voltage level is still set by
the BORV1:BORV0 configuration bits. It
cannot be changed in software.
BOR Configuration Status of
SBOREN
(RCON<6>)
BOR Operation
BOREN1 BOREN0
0 0 Unavailable BOR disabled; must be enabled by reprogramming the configuration bits.
0 1 Available BOR enabled in software; operation controlled by SBOREN.
1 0 Unavailable BOR enabled in hardware in Run and Idle modes, disabled during
Sleep mode.
1 1 Unavailable BOR enabled in hardware; must be disabled by reprogramming the
configuration bits.
© 2007 Microchip Technology Inc. Preliminary DS39631B-page 45
PIC18F2420/2520/4420/4520
4.5 Device Reset Timers
PIC18F2420/2520/4420/4520 devices incorporate three
separate on-chip timers that help regulate the Power-on
Reset process. Their main function is to ensure that the
device clock is stable before code is executed. These
timers are:
• Power-up Timer (PWRT)
• Oscillator Start-up Timer (OST)
• PLL Lock Time-out
4.5.1 POWER-UP TIMER (PWRT)
The Power-up Timer (PWRT) of PIC18F2420/2520/
4420/4520 devices is an 11-bit counter which uses
the INTRC source as the clock input. This yields an
approximate time interval of 2048 x 32 μs = 65.6ms.
While the PWRT is counting, the device is held in
Reset.
The power-up time delay depends on the INTRC clock
and will vary from chip to chip due to temperature and
process variation. See DC parameter 33 for details.
The PWRT is enabled by clearing the PWRTEN
configuration bit.
4.5.2 OSCILLATOR START-UP TIMER
(OST)
The Oscillator Start-up Timer (OST) provides a 1024
oscillator cycle (from OSC1 input) delay after the
PWRT delay is over (parameter 33). This ensures that
the crystal oscillator or resonator has started and
stabilized.
The OST time-out is invoked only for XT, LP, HS and
HSPLL modes and only on Power-on Reset, or on exit
from most power managed modes.
4.5.3 PLL LOCK TIME-OUT
With the PLL enabled in its PLL mode, the time-out
sequence following a Power-on Reset is slightly different
from other oscillator modes. A separate timer is
used to provide a fixed time-out that is sufficient for the
PLL to lock to the main oscillator frequency. This PLL
lock time-out (TPLL) is typically 2 ms and follows the
oscillator start-up time-out.
4.5.4 TIME-OUT SEQUENCE
On power-up, the time-out sequence is as follows:
1. After the POR pulse has cleared, PWRT time-out
is invoked (if enabled).
2. Then, the OST is activated.
The total time-out will vary based on oscillator configuration
and the status of the PWRT. Figure 4-3,
Figure 4-4, Figure 4-5, Figure 4-6 and Figure 4-7 all
depict time-out sequences on power-up, with the
Power-up Timer enabled and the device operating in
HS Oscillator mode. Figures 4-3 through 4-6 also
apply to devices operating in XT or LP modes. For
devices in RC mode and with the PWRT disabled, on
the other hand, there will be no time-out at all.
Since the time-outs occur from the POR pulse, if MCLR
is kept low long enough, all time-outs will expire. Bringing
MCLR high will begin execution immediately
(Figure 4-5). This is useful for testing purposes or to
synchronize more than one PIC18FXXXX device
operating in parallel.
TABLE 4-2: TIME-OUT IN VARIOUS SITUATIONS
Oscillator
Configuration
Power-up(2) and Brown-out Exit from
PWRTEN = 0 PWRTEN = 1 Power Managed Mode
HSPLL 66 ms(1) + 1024 TOSC + 2 ms(2) 1024 TOSC + 2 ms(2) 1024 TOSC + 2 ms(2)
HS, XT, LP 66 ms(1) + 1024 TOSC 1024 TOSC 1024 TOSC
EC, ECIO 66 ms(1) — —
RC, RCIO 66 ms(1) — —
INTIO1, INTIO2 66 ms(1) — —
Note 1: 66 ms (65.5 ms) is the nominal Power-up Timer (PWRT) delay.
2: 2 ms is the nominal time required for the PLL to lock.
PIC18F2420/2520/4420/4520
DS39631B-page 46 Preliminary © 2007 Microchip Technology Inc.
FIGURE 4-3: TIME-OUT SEQUENCE ON POWER-UP (MCLR TIED TO VDD, VDD RISE < TPWRT)
FIGURE 4-4: TIME-OUT SEQUENCE ON POWER-UP (MCLR NOT TIED TO VDD): CASE 1
FIGURE 4-5: TIME-OUT SEQUENCE ON POWER-UP (MCLR NOT TIED TO VDD): CASE 2
TPWRT
TOST
VDD
MCLR
INTERNAL POR
PWRT TIME-OUT
OST TIME-OUT
INTERNAL RESET
TPWRT
TOST
VDD
MCLR
INTERNAL POR
PWRT TIME-OUT
OST TIME-OUT
INTERNAL RESET
VDD
MCLR
INTERNAL POR
PWRT TIME-OUT
OST TIME-OUT
INTERNAL RESET
TPWRT
TOST
© 2007 Microchip Technology Inc. Preliminary DS39631B-page 47
PIC18F2420/2520/4420/4520
FIGURE 4-6: SLOW RISE TIME (MCLR TIED TO VDD, VDD RISE > TPWRT)
FIGURE 4-7: TIME-OUT SEQUENCE ON POR W/PLL ENABLED (MCLR TIED TO VDD)
VDD
MCLR
INTERNAL POR
PWRT TIME-OUT
OST TIME-OUT
INTERNAL RESET
0V
5V
TPWRT
TOST
TPWRT
TOST
VDD
MCLR
INTERNAL POR
PWRT TIME-OUT
OST TIME-OUT
INTERNAL RESET
PLL TIME-OUT
TPLL
Note: TOST = 1024 clock cycles.
TPLL ≈ 2 ms max. First three stages of the PWRT timer.
PIC18F2420/2520/4420/4520
DS39631B-page 48 Preliminary © 2007 Microchip Technology Inc.
4.6 Reset State of Registers
Most registers are unaffected by a Reset. Their status
is unknown on POR and unchanged by all other
Resets. The other registers are forced to a “Reset
state” depending on the type of Reset that occurred.
Most registers are not affected by a WDT wake-up,
since this is viewed as the resumption of normal operation.
Status bits from the RCON register, RI, TO, PD,
POR and BOR, are set or cleared differently in different
Reset situations, as indicated in Table 4-3. These bits
are used in software to determine the nature of the
Reset.
Table 4-4 describes the Reset states for all of the
Special Function Registers. These are categorized by
Power-on and Brown-out Resets, Master Clear and
WDT Resets and WDT wake-ups.
TABLE 4-3: STATUS BITS, THEIR SIGNIFICANCE AND THE INITIALIZATION CONDITION
FOR RCON REGISTER
Condition
Program
Counter
RCON Register STKPTR Register
SBOREN RI TO PD POR BOR STKFUL STKUNF
Power-on Reset 0000h 1 1 1 1 0 0 0 0
RESET Instruction 0000h u(2) 0 u u u u u u
Brown-out Reset 0000h u(2) 1 1 1 u 0 u u
MCLR during Power Managed
Run Modes
0000h u(2) u 1 u u u u u
MCLR during Power Managed
Idle Modes and Sleep Mode
0000h u(2) u 1 0 u u u u
WDT Time-out during Full Power
or Power Managed Run Mode
0000h u(2) u 0 u u u u u
MCLR during Full Power
Execution
0000h u(2) u u u u u u u
Stack Full Reset (STVREN = 1) 0000h u(2) u u u u u 1 u
Stack Underflow Reset
(STVREN = 1)
0000h u(2) u u u u u u 1
Stack Underflow Error (not an
actual Reset, STVREN = 0)
0000h u(2) u u u u u u 1
WDT Time-out during Power
Managed Idle or Sleep Modes
PC + 2 u(2) u 0 0 u u u u
Interrupt Exit from Power
Managed Modes
PC + 2(1) u(2) u u 0 u u u u
Legend: u = unchanged
Note 1: When the wake-up is due to an interrupt and the GIEH or GIEL bits are set, the PC is loaded with the
interrupt vector (008h or 0018h).
2: Reset state is ‘1’ for POR and unchanged for all other Resets when software BOR is enabled
(BOREN1:BOREN0 configuration bits = 01 and SBOREN = 1). Otherwise, the Reset state is ‘0’.
© 2007 Microchip Technology Inc. Preliminary DS39631B-page 49
PIC18F2420/2520/4420/4520
TABLE 4-4: INITIALIZATION CONDITIONS FOR ALL REGISTERS
Register Applicable Devices
Power-on Reset,
Brown-out Reset
MCLR Resets,
WDT Reset,
RESET Instruction,
Stack Resets
Wake-up via WDT
or Interrupt
TOSU 2420 2520 4420 4520 ---0 0000 ---0 0000 ---0 uuuu(3)
TOSH 2420 2520 4420 4520 0000 0000 0000 0000 uuuu uuuu(3)
TOSL 2420 2520 4420 4520 0000 0000 0000 0000 uuuu uuuu(3)
STKPTR 2420 2520 4420 4520 00-0 0000 uu-0 0000 uu-u uuuu(3)
PCLATU 2420 2520 4420 4520 ---0 0000 ---0 0000 ---u uuuu
PCLATH 2420 2520 4420 4520 0000 0000 0000 0000 uuuu uuuu
PCL 2420 2520 4420 4520 0000 0000 0000 0000 PC + 2(2)
TBLPTRU 2420 2520 4420 4520 --00 0000 --00 0000 --uu uuuu
TBLPTRH 2420 2520 4420 4520 0000 0000 0000 0000 uuuu uuuu
TBLPTRL 2420 2520 4420 4520 0000 0000 0000 0000 uuuu uuuu
TABLAT 2420 2520 4420 4520 0000 0000 0000 0000 uuuu uuuu
PRODH 2420 2520 4420 4520 xxxx xxxx uuuu uuuu uuuu uuuu
PRODL 2420 2520 4420 4520 xxxx xxxx uuuu uuuu uuuu uuuu
INTCON 2420 2520 4420 4520 0000 000x 0000 000u uuuu uuuu(1)
INTCON2 2420 2520 4420 4520 1111 -1-1 1111 -1-1 uuuu -u-u(1)
INTCON3 2420 2520 4420 4520 11-0 0-00 11-0 0-00 uu-u u-uu(1)
INDF0 2420 2520 4420 4520 N/A N/A N/A
POSTINC0 2420 2520 4420 4520 N/A N/A N/A
POSTDEC0 2420 2520 4420 4520 N/A N/A N/A
PREINC0 2420 2520 4420 4520 N/A N/A N/A
PLUSW0 2420 2520 4420 4520 N/A N/A N/A
FSR0H 2420 2520 4420 4520 ---- 0000 ---- 0000 ---- uuuu
FSR0L 2420 2520 4420 4520 xxxx xxxx uuuu uuuu uuuu uuuu
WREG 2420 2520 4420 4520 xxxx xxxx uuuu uuuu uuuu uuuu
INDF1 2420 2520 4420 4520 N/A N/A N/A
POSTINC1 2420 2520 4420 4520 N/A N/A N/A
POSTDEC1 2420 2520 4420 4520 N/A N/A N/A
PREINC1 2420 2520 4420 4520 N/A N/A N/A
PLUSW1 2420 2520 4420 4520 N/A N/A N/A
Legend: u = unchanged, x = unknown, - = unimplemented bit, read as ‘0’, q = value depends on condition.
Shaded cells indicate conditions do not apply for the designated device.
Note 1: One or more bits in the INTCONx or PIRx registers will be affected (to cause wake-up).
2: When the wake-up is due to an interrupt and the GIEL or GIEH bit is set, the PC is loaded with the interrupt vector
(0008h or 0018h).
3: When the wake-up is due to an interrupt and the GIEL or GIEH bit is set, the TOSU, TOSH and TOSL are updated with
the current value of the PC. The STKPTR is modified to point to the next location in the hardware stack.
4: See Table 4-3 for Reset value for specific condition.
5: Bits 6 and 7 of PORTA, LATA and TRISA are enabled, depending on the oscillator mode selected. When not enabled
as PORTA pins, they are disabled and read ‘0’.
PIC18F2420/2520/4420/4520
DS39631B-page 50 Preliminary © 2007 Microchip Technology Inc.
FSR1H 2420 2520 4420 4520 ---- 0000 ---- 0000 ---- uuuu
FSR1L 2420 2520 4420 4520 xxxx xxxx uuuu uuuu uuuu uuuu
BSR 2420 2520 4420 4520 ---- 0000 ---- 0000 ---- uuuu
INDF2 2420 2520 4420 4520 N/A N/A N/A
POSTINC2 2420 2520 4420 4520 N/A N/A N/A
POSTDEC2 2420 2520 4420 4520 N/A N/A N/A
PREINC2 2420 2520 4420 4520 N/A N/A N/A
PLUSW2 2420 2520 4420 4520 N/A N/A N/A
FSR2H 2420 2520 4420 4520 ---- 0000 ---- 0000 ---- uuuu
FSR2L 2420 2520 4420 4520 xxxx xxxx uuuu uuuu uuuu uuuu
STATUS 2420 2520 4420 4520 ---x xxxx ---u uuuu ---u uuuu
TMR0H 2420 2520 4420 4520 0000 0000 0000 0000 uuuu uuuu
TMR0L 2420 2520 4420 4520 xxxx xxxx uuuu uuuu uuuu uuuu
T0CON 2420 2520 4420 4520 1111 1111 1111 1111 uuuu uuuu
OSCCON 2420 2520 4420 4520 0100 q000 0100 q000 uuuu uuqu
HLVDCON 2420 2520 4420 4520 0-00 0101 0-00 0101 u-uu uuuu
WDTCON 2420 2520 4420 4520 ---- ---0 ---- ---0 ---- ---u
RCON(4) 2420 2520 4420 4520 0q-1 11q0 0q-q qquu uq-u qquu
TMR1H 2420 2520 4420 4520 xxxx xxxx uuuu uuuu uuuu uuuu
TMR1L 2420 2520 4420 4520 xxxx xxxx uuuu uuuu uuuu uuuu
T1CON 2420 2520 4420 4520 0000 0000 u0uu uuuu uuuu uuuu
TMR2 2420 2520 4420 4520 0000 0000 0000 0000 uuuu uuuu
PR2 2420 2520 4420 4520 1111 1111 1111 1111 1111 1111
T2CON 2420 2520 4420 4520 -000 0000 -000 0000 -uuu uuuu
SSPBUF 2420 2520 4420 4520 xxxx xxxx uuuu uuuu uuuu uuuu
SSPADD 2420 2520 4420 4520 0000 0000 0000 0000 uuuu uuuu
SSPSTAT 2420 2520 4420 4520 0000 0000 0000 0000 uuuu uuuu
SSPCON1 2420 2520 4420 4520 0000 0000 0000 0000 uuuu uuuu
SSPCON2 2420 2520 4420 4520 0000 0000 0000 0000 uuuu uuuu
TABLE 4-4: INITIALIZATION CONDITIONS FOR ALL REGISTERS (CONTINUED)
Register Applicable Devices
Power-on Reset,
Brown-out Reset
MCLR Resets,
WDT Reset,
RESET Instruction,
Stack Resets
Wake-up via WDT
or Interrupt
Legend: u = unchanged, x = unknown, - = unimplemented bit, read as ‘0’, q = value depends on condition.
Shaded cells indicate conditions do not apply for the designated device.
Note 1: One or more bits in the INTCONx or PIRx registers will be affected (to cause wake-up).
2: When the wake-up is due to an interrupt and the GIEL or GIEH bit is set, the PC is loaded with the interrupt vector
(0008h or 0018h).
3: When the wake-up is due to an interrupt and the GIEL or GIEH bit is set, the TOSU, TOSH and TOSL are updated with
the current value of the PC. The STKPTR is modified to point to the next location in the hardware stack.
4: See Table 4-3 for Reset value for specific condition.
5: Bits 6 and 7 of PORTA, LATA and TRISA are enabled, depending on the oscillator mode selected. When not enabled
as PORTA pins, they are disabled and read ‘0’.
© 2007 Microchip Technology Inc. Preliminary DS39631B-page 51
PIC18F2420/2520/4420/4520
ADRESH 2420 2520 4420 4520 xxxx xxxx uuuu uuuu uuuu uuuu
ADRESL 2420 2520 4420 4520 xxxx xxxx uuuu uuuu uuuu uuuu
ADCON0 2420 2520 4420 4520 --00 0000 --00 0000 --uu uuuu
ADCON1 2420 2520 4420 4520 --00 0qqq --00 0qqq --uu uuuu
ADCON2 2420 2520 4420 4520 0-00 0000 0-00 0000 u-uu uuuu
CCPR1H 2420 2520 4420 4520 xxxx xxxx uuuu uuuu uuuu uuuu
CCPR1L 2420 2520 4420 4520 xxxx xxxx uuuu uuuu uuuu uuuu
CCP1CON
2420 2520 4420 4520 0000 0000 0000 0000 uuuu uuuu
2420 2520 4420 4520 --00 0000 --00 0000 --uu uuuu
CCPR2H 2420 2520 4420 4520 xxxx xxxx uuuu uuuu uuuu uuuu
CCPR2L 2420 2520 4420 4520 xxxx xxxx uuuu uuuu uuuu uuuu
CCP2CON 2420 2520 4420 4520 --00 0000 --00 0000 --uu uuuu
BAUDCON 2420 2520 4420 4520 01-0 0-00 01-0 0-00 --uu uuuu
PWM1CON 2420 2520 4420 4520 0000 0000 0000 0000 uuuu uuuu
ECCP1AS
2420 2520 4420 4520 0000 0000 0000 0000 uuuu uuuu
2420 2520 4420 4520 0000 00-- 0000 00-- uuuu uu--
CVRCON 2420 2520 4420 4520 0000 0000 0000 0000 uuuu uuuu
CMCON 2420 2520 4420 4520 0000 0111 0000 0111 uuuu uuuu
TMR3H 2420 2520 4420 4520 xxxx xxxx uuuu uuuu uuuu uuuu
TMR3L 2420 2520 4420 4520 xxxx xxxx uuuu uuuu uuuu uuuu
T3CON 2420 2520 4420 4520 0000 0000 uuuu uuuu uuuu uuuu
SPBRGH 2420 2520 4420 4520 0000 0000 0000 0000 uuuu uuuu
SPBRG 2420 2520 4420 4520 0000 0000 0000 0000 uuuu uuuu
RCREG 2420 2520 4420 4520 0000 0000 0000 0000 uuuu uuuu
TXREG 2420 2520 4420 4520 0000 0000 0000 0000 uuuu uuuu
TXSTA 2420 2520 4420 4520 0000 0010 0000 0010 uuuu uuuu
RCSTA 2420 2520 4420 4520 0000 000x 0000 000x uuuu uuuu
EEADR 2420 2520 4420 4520 0000 0000 0000 0000 uuuu uuuu
EEDATA 2420 2520 4420 4520 0000 0000 0000 0000 uuuu uuuu
EECON2 2420 2520 4420 4520 0000 0000 0000 0000 0000 0000
EECON1 2420 2520 4420 4520 xx-0 x000 uu-0 u000 uu-0 u000
TABLE 4-4: INITIALIZATION CONDITIONS FOR ALL REGISTERS (CONTINUED)
Register Applicable Devices
Power-on Reset,
Brown-out Reset
MCLR Resets,
WDT Reset,
RESET Instruction,
Stack Resets
Wake-up via WDT
or Interrupt
Legend: u = unchanged, x = unknown, - = unimplemented bit, read as ‘0’, q = value depends on condition.
Shaded cells indicate conditions do not apply for the designated device.
Note 1: One or more bits in the INTCONx or PIRx registers will be affected (to cause wake-up).
2: When the wake-up is due to an interrupt and the GIEL or GIEH bit is set, the PC is loaded with the interrupt vector
(0008h or 0018h).
3: When the wake-up is due to an interrupt and the GIEL or GIEH bit is set, the TOSU, TOSH and TOSL are updated with
the current value of the PC. The STKPTR is modified to point to the next location in the hardware stack.
4: See Table 4-3 for Reset value for specific condition.
5: Bits 6 and 7 of PORTA, LATA and TRISA are enabled, depending on the oscillator mode selected. When not enabled
as PORTA pins, they are disabled and read ‘0’.
PIC18F2420/2520/4420/4520
DS39631B-page 52 Preliminary © 2007 Microchip Technology Inc.
IPR2 2420 2520 4420 4520 11-1 1111 11-1 1111 uu-u uuuu
PIR2 2420 2520 4420 4520 00-0 0000 00-0 0000 uu-u uuuu(1)
PIE2 2420 2520 4420 4520 00-0 0000 00-0 0000 uu-u uuuu
IPR1
2420 2520 4420 4520 1111 1111 1111 1111 uuuu uuuu
2420 2520 4420 4520 -111 1111 -111 1111 -uuu uuuu
PIR1
2420 2520 4420 4520 0000 0000 0000 0000 uuuu uuuu(1)
2420 2520 4420 4520 -000 0000 -000 0000 -uuu uuuu(1)
PIE1
2420 2520 4420 4520 0000 0000 0000 0000 uuuu uuuu
2420 2520 4420 4520 -000 0000 -000 0000 -uuu uuuu
OSCTUNE 2420 2520 4420 4520 00-0 0000 00-0 0000 uu-u uuuu
TRISE 2420 2520 4420 4520 0000 -111 0000 -111 uuuu -uuu
TRISD 2420 2520 4420 4520 1111 1111 1111 1111 uuuu uuuu
TRISC 2420 2520 4420 4520 1111 1111 1111 1111 uuuu uuuu
TRISB 2420 2520 4420 4520 1111 1111 1111 1111 uuuu uuuu
TRISA(5) 2420 2520 4420 4520 1111 1111(5) 1111 1111(5) uuuu uuuu(5)
LATE 2420 2520 4420 4520 ---- -xxx ---- -uuu ---- -uuu
LATD 2420 2520 4420 4520 xxxx xxxx uuuu uuuu uuuu uuuu
LATC 2420 2520 4420 4520 xxxx xxxx uuuu uuuu uuuu uuuu
LATB 2420 2520 4420 4520 xxxx xxxx uuuu uuuu uuuu uuuu
LATA(5) 2420 2520 4420 4520 xxxx xxxx(5) uuuu uuuu(5) uuuu uuuu(5)
PORTE 2420 2520 4420 4520 ---- xxxx ---- uuuu ---- uuuu
PORTD 2420 2520 4420 4520 xxxx xxxx uuuu uuuu uuuu uuuu
PORTC 2420 2520 4420 4520 xxxx xxxx uuuu uuuu uuuu uuuu
PORTB 2420 2520 4420 4520 xxxx xxxx uuuu uuuu uuuu uuuu
PORTA(5) 2420 2520 4420 4520 xx0x 0000(5) uu0u 0000(5) uuuu uuuu(5)
TABLE 4-4: INITIALIZATION CONDITIONS FOR ALL REGISTERS (CONTINUED)
Register Applicable Devices
Power-on Reset,
Brown-out Reset
MCLR Resets,
WDT Reset,
RESET Instruction,
Stack Resets
Wake-up via WDT
or Interrupt
Legend: u = unchanged, x = unknown, - = unimplemented bit, read as ‘0’, q = value depends on condition.
Shaded cells indicate conditions do not apply for the designated device.
Note 1: One or more bits in the INTCONx or PIRx registers will be affected (to cause wake-up).
2: When the wake-up is due to an interrupt and the GIEL or GIEH bit is set, the PC is loaded with the interrupt vector
(0008h or 0018h).
3: When the wake-up is due to an interrupt and the GIEL or GIEH bit is set, the TOSU, TOSH and TOSL are updated with
the current value of the PC. The STKPTR is modified to point to the next location in the hardware stack.
4: See Table 4-3 for Reset value for specific condition.
5: Bits 6 and 7 of PORTA, LATA and TRISA are enabled, depending on the oscillator mode selected. When not enabled
as PORTA pins, they are disabled and read ‘0’.
© 2007 Microchip Technology Inc. Preliminary DS39631B-page 53
PIC18F2420/2520/4420/4520
5.0 MEMORY ORGANIZATION
There are three types of memory in PIC18 Enhanced
microcontroller devices:
• Program Memory
• Data RAM
• Data EEPROM
As Harvard architecture devices, the data and program
memories use separate busses; this allows for concurrent
access of the two memory spaces. The data
EEPROM, for practical purposes, can be regarded as
a peripheral device, since it is addressed and accessed
through a set of control registers.
Additional detailed information on the operation of the
Flash program memory is provided in Section 6.0
“Flash Program Memory”. Data EEPROM is
discussed separately in Section 7.0 “Data EEPROM
Memory”.
5.1 Program Memory Organization
PIC18 microcontrollers implement a 21-bit program
counter, which is capable of addressing a 2-Mbyte
program memory space. Accessing a location between
the upper boundary of the physically implemented
memory and the 2-Mbyte address will return all ‘0’s (a
NOP instruction).
The PIC18F2420 and PIC18F4420 each have
16 Kbytes of Flash memory and can store up to 8,192
single-word instructions. The PIC18F2520 and
PIC18F4520 each have 32 Kbytes of Flash memory
and can store up to 16,384 single-word instructions.
PIC18 devices have two interrupt vectors. The Reset
vector address is at 0000h and the interrupt vector
addresses are at 0008h and 0018h.
The program memory map for PIC18F2420/2520/
4420/4520 devices is shown in Figure 5-1.
FIGURE 5-1: PROGRAM MEMORY MAP AND STACK FOR
PIC18F2420/2520/4420/4520 DEVICES
PC<20:0>
Stack Level 1
•
Stack Level 31
Reset Vector
Low Priority Interrupt Vector
••
CALL,RCALL,RETURN
RETFIE,RETLW
21
0000h
0018h
On-Chip
Program Memory
High Priority Interrupt Vector 0008h
User Memory Space
1FFFFFh
4000h
3FFFh
Read ‘0’
200000h
PIC18FX4X0
PIC18FX5X0
8000h
7FFFh
On-Chip
Program Memory
Read ‘0’
PIC18F2420/2520/4420/4520
DS39631B-page 54 Preliminary © 2007 Microchip Technology Inc.
5.1.1 PROGRAM COUNTER
The Program Counter (PC) specifies the address of the
instruction to fetch for execution. The PC is 21 bits wide
and is contained in three separate 8-bit registers. The
low byte, known as the PCL register, is both readable
and writable. The high byte, or PCH register, contains
the PC<15:8> bits; it is not directly readable or writable.
Updates to the PCH register are performed through the
PCLATH register. The upper byte is called PCU. This
register contains the PC<20:16> bits; it is also not
directly readable or writable. Updates to the PCU
register are performed through the PCLATU register.
The contents of PCLATH and PCLATU are transferred
to the program counter by any operation that writes
PCL. Similarly, the upper two bytes of the program
counter are transferred to PCLATH and PCLATU by an
operation that reads PCL. This is useful for computed
offsets to the PC (see Section 5.1.4.1 “Computed
GOTO”).
The PC addresses bytes in the program memory. To
prevent the PC from becoming misaligned with word
instructions, the Least Significant bit of PCL is fixed to
a value of ‘0’. The PC increments by 2 to address
sequential instructions in the program memory.
The CALL, RCALL, GOTO and program branch
instructions write to the program counter directly. For
these instructions, the contents of PCLATH and
PCLATU are not transferred to the program counter.
5.1.2 RETURN ADDRESS STACK
The return address stack allows any combination of up
to 31 program calls and interrupts to occur. The PC is
pushed onto the stack when a CALL or RCALL instruction
is executed or an interrupt is Acknowledged. The
PC value is pulled off the stack on a RETURN, RETLW
or a RETFIE instruction. PCLATU and PCLATH are not
affected by any of the RETURN or CALL instructions.
The stack operates as a 31-word by 21-bit RAM and a
5-bit stack pointer, STKPTR. The stack space is not
part of either program or data space. The stack pointer
is readable and writable and the address on the top of
the stack is readable and writable through the top-ofstack
Special File Registers. Data can also be pushed
to, or popped from the stack, using these registers.
A CALL type instruction causes a push onto the stack;
the stack pointer is first incremented and the location
pointed to by the stack pointer is written with the
contents of the PC (already pointing to the instruction
following the CALL). A RETURN type instruction causes
a pop from the stack; the contents of the location
pointed to by the STKPTR are transferred to the PC
and then the stack pointer is decremented.
The stack pointer is initialized to ‘00000’ after all
Resets. There is no RAM associated with the location
corresponding to a stack pointer value of ‘00000’; this
is only a Reset value. Status bits indicate if the stack is
full or has overflowed or has underflowed.
5.1.2.1 Top-of-Stack Access
Only the top of the return address stack (TOS) is
readable and writable. A set of three registers,
TOSU:TOSH:TOSL, hold the contents of the stack location
pointed to by the STKPTR register (Figure 5-2). This
allows users to implement a software stack if necessary.
After a CALL, RCALL or interrupt, the software can read
the pushed value by reading the TOSU:TOSH:TOSL
registers. These values can be placed on a user defined
software stack. At return time, the software can return
these values to TOSU:TOSH:TOSL and do a return.
The user must disable the global interrupt enable bits
while accessing the stack to prevent inadvertent stack
corruption.
FIGURE 5-2: RETURN ADDRESS STACK AND ASSOCIATED REGISTERS
00011
001A34h
11111
11110
11101
00010
00001
00000
00010
Return Address Stack <20:0>
Top-of-Stack
000D58h
TOSU TOSH TOSL
00h 1Ah 34h
STKPTR<4:0>
Top-of-Stack Registers Stack Pointer
© 2007 Microchip Technology Inc. Preliminary DS39631B-page 55
PIC18F2420/2520/4420/4520
5.1.2.2 Return Stack Pointer (STKPTR)
The STKPTR register (Register 5-1) contains the stack
pointer value, the STKFUL (stack full) status bit and the
STKUNF (stack underflow) status bits. The value of the
stack pointer can be 0 through 31. The stack pointer
increments before values are pushed onto the stack
and decrements after values are popped off the stack.
On Reset, the stack pointer value will be zero. The user
may read and write the stack pointer value. This feature
can be used by a Real-Time Operating System (RTOS)
for return stack maintenance.
After the PC is pushed onto the stack 31 times (without
popping any values off the stack), the STKFUL bit is
set. The STKFUL bit is cleared by software or by a
POR.
The action that takes place when the stack becomes
full depends on the state of the STVREN (Stack Overflow
Reset Enable) configuration bit. (Refer to
Section 23.1 “Configuration Bits” for a description of
the device configuration bits.) If STVREN is set
(default), the 31st push will push the (PC + 2) value
onto the stack, set the STKFUL bit and reset the
device. The STKFUL bit will remain set and the stack
pointer will be set to zero.
If STVREN is cleared, the STKFUL bit will be set on the
31st push and the stack pointer will increment to 31.
Any additional pushes will not overwrite the 31st push
and STKPTR will remain at 31.
When the stack has been popped enough times to
unload the stack, the next pop will return a value of zero
to the PC and sets the STKUNF bit, while the stack
pointer remains at zero. The STKUNF bit will remain
set until cleared by software or until a POR occurs.
5.1.2.3 PUSH and POP Instructions
Since the Top-of-Stack is readable and writable, the
ability to push values onto the stack and pull values off
the stack without disturbing normal program execution
is a desirable feature. The PIC18 instruction set
includes two instructions, PUSH and POP, that permit
the TOS to be manipulated under software control.
TOSU, TOSH and TOSL can be modified to place data
or a return address on the stack.
The PUSH instruction places the current PC value onto
the stack. This increments the stack pointer and loads
the current PC value onto the stack.
The POP instruction discards the current TOS by decrementing
the stack pointer. The previous value pushed
onto the stack then becomes the TOS value.
REGISTER 5-1: STKPTR REGISTER
Note: Returning a value of zero to the PC on an
underflow has the effect of vectoring the
program to the Reset vector, where the
stack conditions can be verified and
appropriate actions can be taken. This is
not the same as a Reset, as the contents
of the SFRs are not affected.
R/C-0 R/C-0 U-0 R/W-0 R/W-0 R/W-0 R/W-0 R/W-0
STKFUL(1) STKUNF(1) — SP4 SP3 SP2 SP1 SP0
bit 7 bit 0
bit 7 STKFUL: Stack Full Flag bit(1)
1 = Stack became full or overflowed
0 = Stack has not become full or overflowed
bit 6 STKUNF: Stack Underflow Flag bit(1)
1 = Stack underflow occurred
0 = Stack underflow did not occur
bit 5 Unimplemented: Read as ‘0’
bit 4-0 SP4:SP0: Stack Pointer Location bits
Note 1: Bit 7 and bit 6 are cleared by user software or by a POR.
Legend:
R = Readable bit W = Writable bit U = Unimplemented C = Clearable only bit
-n = Value at POR ‘1’ = Bit is set ‘0’ = Bit is cleared x = Bit is unknown
PIC18F2420/2520/4420/4520
DS39631B-page 56 Preliminary © 2007 Microchip Technology Inc.
5.1.2.4 Stack Full and Underflow Resets
Device Resets on stack overflow and stack underflow
conditions are enabled by setting the STVREN bit in
Configuration Register 4L. When STVREN is set, a full
or underflow will set the appropriate STKFUL or
STKUNF bit and then cause a device Reset. When
STVREN is cleared, a full or underflow condition will set
the appropriate STKFUL or STKUNF bit but not cause
a device Reset. The STKFUL or STKUNF bits are
cleared by the user software or a Power-on Reset.
5.1.3 FAST REGISTER STACK
A fast register stack is provided for the Status, WREG
and BSR registers, to provide a “fast return” option for
interrupts. The stack for each register is only one level
deep and is neither readable nor writable. It is loaded
with the current value of the corresponding register
when the processor vectors for an interrupt. All interrupt
sources will push values into the stack registers.
The values in the registers are then loaded back into
their associated registers if the RETFIE, FAST
instruction is used to return from the interrupt.
If both low and high priority interrupts are enabled, the
stack registers cannot be used reliably to return from
low priority interrupts. If a high priority interrupt occurs
while servicing a low priority interrupt, the stack register
values stored by the low priority interrupt will be
overwritten. In these cases, users must save the key
registers in software during a low priority interrupt.
If interrupt priority is not used, all interrupts may use the
fast register stack for returns from interrupt. If no interrupts
are used, the fast register stack can be used to
restore the Status, WREG and BSR registers at the end
of a subroutine call. To use the fast register stack for a
subroutine call, a CALL label, FAST instruction must
be executed to save the Status, WREG and BSR
registers to the fast register stack. A RETURN, FAST
instruction is then executed to restore these registers
from the fast register stack.
Example 5-1 shows a source code example that uses
the fast register stack during a subroutine call and
return.
EXAMPLE 5-1: FAST REGISTER STACK
CODE EXAMPLE
5.1.4 LOOK-UP TABLES IN PROGRAM
MEMORY
There may be programming situations that require the
creation of data structures, or look-up tables, in
program memory. For PIC18 devices, look-up tables
can be implemented in two ways:
• Computed GOTO
• Table Reads
5.1.4.1 Computed GOTO
A computed GOTO is accomplished by adding an offset
to the program counter. An example is shown in
Example 5-2.
A look-up table can be formed with an ADDWF PCL
instruction and a group of RETLW nn instructions. The
W register is loaded with an offset into the table before
executing a call to that table. The first instruction of the
called routine is the ADDWF PCL instruction. The next
instruction executed will be one of the RETLW nn
instructions that returns the value ‘nn’ to the calling
function.
The offset value (in WREG) specifies the number of
bytes that the program counter should advance and
should be multiples of 2 (LSb = 0).
In this method, only one data byte may be stored in
each instruction location and room on the return
address stack is required.
EXAMPLE 5-2: COMPUTED GOTO USING
AN OFFSET VALUE
5.1.4.2 Table Reads and Table Writes
A better method of storing data in program memory
allows two bytes of data to be stored in each instruction
location.
Look-up table data may be stored two bytes per program
word by using table reads and writes. The Table
Pointer (TBLPTR) register specifies the byte address
and the Table Latch (TABLAT) register contains the
data that is read from or written to program memory.
Data is transferred to or from program memory one
byte at a time.
Table read and table write operations are discussed
further in Section 6.1 “Table Reads and Table
Writes”.
CALL SUB1, FAST ;STATUS, WREG, BSR
;SAVED IN FAST REGISTER
;STACK
••
SUB1 ••
RETURN, FAST ;RESTORE VALUES SAVED
;IN FAST REGISTER STACK
MOVF OFFSET, W
CALL TABLE
ORG nn00h
TABLE ADDWF PCL
RETLW nnh
RETLW nnh
RETLW nnh
.
.
.
© 2007 Microchip Technology Inc. Preliminary DS39631B-page 57
PIC18F2420/2520/4420/4520
5.2 PIC18 Instruction Cycle
5.2.1 CLOCKING SCHEME
The microcontroller clock input, whether from an internal
or external source, is internally divided by four to
generate four non-overlapping quadrature clocks (Q1,
Q2, Q3 and Q4). Internally, the program counter is
incremented on every Q1; the instruction is fetched
from the program memory and latched into the instruction
register during Q4. The instruction is decoded and
executed during the following Q1 through Q4. The
clocks and instruction execution flow are shown in
Figure 5-3.
5.2.2 INSTRUCTION FLOW/PIPELINING
An “Instruction Cycle” consists of four Q cycles: Q1
through Q4. The instruction fetch and execute are
pipelined in such a manner that a fetch takes one
instruction cycle, while the decode and execute take
another instruction cycle. However, due to the pipelining,
each instruction effectively executes in one
cycle. If an instruction causes the program counter to
change (e.g., GOTO), then two cycles are required to
complete the instruction (Example 5-3).
A fetch cycle begins with the Program Counter (PC)
incrementing in Q1.
In the execution cycle, the fetched instruction is latched
into the Instruction Register (IR) in cycle Q1. This
instruction is then decoded and executed during the
Q2, Q3 and Q4 cycles. Data memory is read during Q2
(operand read) and written during Q4 (destination
write).
FIGURE 5-3: CLOCK/INSTRUCTION CYCLE
EXAMPLE 5-3: INSTRUCTION PIPELINE FLOW
Q1 Q2 Q3 Q4 Q1 Q2 Q3 Q4 Q1 Q2 Q3 Q4
OSC1
Q1
Q2
Q3
Q4
PC
OSC2/CLKO
(RC mode)
PC PC + 2 PC + 4
Fetch INST (PC)
Execute INST (PC – 2)
Fetch INST (PC + 2)
Execute INST (PC)
Fetch INST (PC + 4)
Execute INST (PC + 2)
Internal
Phase
Clock
All instructions are single cycle, except for any program branches. These take two cycles since the fetch instruction
is “flushed” from the pipeline while the new instruction is being fetched and then executed.
TCY0 TCY1 TCY2 TCY3 TCY4 TCY5
1. MOVLW 55h Fetch 1 Execute 1
2. MOVWF PORTB Fetch 2 Execute 2
3. BRA SUB_1 Fetch 3 Execute 3
4. BSF PORTA, BIT3 (Forced NOP) Fetch 4 Flush (NOP)
5. Instruction @ address SUB_1 Fetch SUB_1 Execute SUB_1
PIC18F2420/2520/4420/4520
DS39631B-page 58 Preliminary © 2007 Microchip Technology Inc.
5.2.3 INSTRUCTIONS IN PROGRAM
MEMORY
The program memory is addressed in bytes. Instructions
are stored as two bytes or four bytes in program
memory. The Least Significant Byte of an instruction
word is always stored in a program memory location
with an even address (LSb = 0). To maintain alignment
with instruction boundaries, the PC increments in steps
of 2 and the LSb will always read ‘0’ (see Section 5.1.1
“Program Counter”).
Figure 5-4 shows an example of how instruction words
are stored in the program memory.
The CALL and GOTO instructions have the absolute program
memory address embedded into the instruction.
Since instructions are always stored on word boundaries,
the data contained in the instruction is a word
address. The word address is written to PC<20:1>,
which accesses the desired byte address in program
memory. Instruction #2 in Figure 5-4 shows how the
instruction GOTO 0006h is encoded in the program
memory. Program branch instructions, which encode a
relative address offset, operate in the same manner. The
offset value stored in a branch instruction represents the
number of single-word instructions that the PC will be
offset by. Section 24.0 “Instruction Set Summary”
provides further details of the instruction set.
FIGURE 5-4: INSTRUCTIONS IN PROGRAM MEMORY
5.2.4 TWO-WORD INSTRUCTIONS
The standard PIC18 instruction set has four two-word
instructions: CALL, MOVFF, GOTO and LSFR. In all
cases, the second word of the instructions always has
‘1111’ as its four Most Significant bits; the other 12 bits
are literal data, usually a data memory address.
The use of ‘1111’ in the 4 MSbs of an instruction specifies
a special form of NOP. If the instruction is executed
in proper sequence – immediately after the first word –
the data in the second word is accessed and used by
the instruction sequence. If the first word is skipped for
some reason and the second word is executed by itself,
a NOP is executed instead. This is necessary for cases
when the two-word instruction is preceded by a conditional
instruction that changes the PC. Example 5-4
shows how this works.
EXAMPLE 5-4: TWO-WORD INSTRUCTIONS
Word Address
LSB = 1 LSB = 0 ↓
Program Memory
Byte Locations →
000000h
000002h
000004h
000006h
Instruction 1: MOVLW 055h 0Fh 55h 000008h
Instruction 2: GOTO 0006h EFh 03h 00000Ah
F0h 00h 00000Ch
Instruction 3: MOVFF 123h, 456h C1h 23h 00000Eh
F4h 56h 000010h
000012h
000014h
Note: See Section 5.6 “PIC18 Instruction
Execution and the Extended Instruction
Set” for information on two-word
instructions in the extended instruction set.
CASE 1:
Object Code Source Code
0110 0110 0000 0000 TSTFSZ REG1 ; is RAM location 0?
1100 0001 0010 0011 MOVFF REG1, REG2 ; No, skip this word
1111 0100 0101 0110 ; Execute this word as a NOP
0010 0100 0000 0000 ADDWF REG3 ; continue code
CASE 2:
Object Code Source Code
0110 0110 0000 0000 TSTFSZ REG1 ; is RAM location 0?
1100 0001 0010 0011 MOVFF REG1, REG2 ; Yes, execute this word
1111 0100 0101 0110 ; 2nd word of instruction
0010 0100 0000 0000 ADDWF REG3 ; continue code
© 2007 Microchip Technology Inc. Preliminary DS39631B-page 59
PIC18F2420/2520/4420/4520
5.3 Data Memory Organization
The data memory in PIC18 devices is implemented as
static RAM. Each register in the data memory has a
12-bit address, allowing up to 4096 bytes of data
memory. The memory space is divided into as many as
16 banks that contain 256 bytes each; PIC18F2420/
2520/4420/4520 devices implement all 16 banks.
Figure 5-5 shows the data memory organization for the
PIC18F2420/2520/4420/4520 devices.
The data memory contains Special Function Registers
(SFRs) and General Purpose Registers (GPRs). The
SFRs are used for control and status of the controller
and peripheral functions, while GPRs are used for data
storage and scratchpad operations in the user’s
application. Any read of an unimplemented location will
read as ‘0’s.
The instruction set and architecture allow operations
across all banks. The entire data memory may be
accessed by Direct, Indirect or Indexed Addressing
modes. Addressing modes are discussed later in this
subsection.
To ensure that commonly used registers (SFRs and
select GPRs) can be accessed in a single cycle, PIC18
devices implement an Access Bank. This is a 256-byte
memory space that provides fast access to SFRs and
the lower portion of GPR Bank 0 without using the
BSR. Section 5.3.2 “Access Bank” provides a
detailed description of the Access RAM.
5.3.1 BANK SELECT REGISTER (BSR)
Large areas of data memory require an efficient
addressing scheme to make rapid access to any
address possible. Ideally, this means that an entire
address does not need to be provided for each read or
write operation. For PIC18 devices, this is accomplished
with a RAM banking scheme. This divides the
memory space into 16 contiguous banks of 256 bytes.
Depending on the instruction, each location can be
addressed directly by its full 12-bit address, or an 8-bit
low-order address and a 4-bit bank pointer.
Most instructions in the PIC18 instruction set make use
of the bank pointer, known as the Bank Select Register
(BSR). This SFR holds the 4 Most Significant bits of a
location’s address; the instruction itself includes the
8 Least Significant bits. Only the four lower bits of the
BSR are implemented (BSR3:BSR0). The upper four
bits are unused; they will always read ‘0’ and cannot be
written to. The BSR can be loaded directly by using the
MOVLB instruction.
The value of the BSR indicates the bank in data
memory; the 8 bits in the instruction show the location
in the bank and can be thought of as an offset from the
bank’s lower boundary. The relationship between the
BSR’s value and the bank division in data memory is
shown in Figure 5-7.
Since up to 16 registers may share the same low-order
address, the user must always be careful to ensure that
the proper bank is selected before performing a data
read or write. For example, writing what should be
program data to an 8-bit address of F9h while the BSR
is 0Fh will end up resetting the program counter.
While any bank can be selected, only those banks that
are actually implemented can be read or written to.
Writes to unimplemented banks are ignored, while
reads from unimplemented banks will return ‘0’s. Even
so, the Status register will still be affected as if the
operation was successful. The data memory map in
Figure 5-5 indicates which banks are implemented.
In the core PIC18 instruction set, only the MOVFF
instruction fully specifies the 12-bit address of the
source and target registers. This instruction ignores the
BSR completely when it executes. All other instructions
include only the low-order address as an operand and
must use either the BSR or the Access Bank to locate
their target registers.
Note: The operation of some aspects of data
memory are changed when the PIC18
extended instruction set is enabled. See
Section 5.5 “Data Memory and the
Extended Instruction Set” for more
information.
PIC18F2420/2520/4420/4520
DS39631B-page 60 Preliminary © 2007 Microchip Technology Inc.
FIGURE 5-5: DATA MEMORY MAP FOR PIC18F2420/4420 DEVICES
Bank 0
Bank 1
Bank 14
Bank 15
BSR<3:0> Data Memory Map
= 0000
= 0001
= 1111
080h
07Fh
F80h
FFFh
00h
7Fh
80h
FFh
Access Bank
When ‘a’ = 0:
The BSR is ignored and the
Access Bank is used.
The first 128 bytes are
general purpose RAM
(from Bank 0).
The second 128 bytes are
Special Function Registers
(from Bank 15).
When ‘a’ = 1:
The BSR specifies the Bank
used by the instruction.
F7Fh
F00h
EFFh
1FFh
100h
0FFh
Access RAM 000h
FFh
00h
FFh
00h
FFh
00h
GPR
GPR
SFR
Access RAM High
Access RAM Low
Bank 2
= 0110
= 0010
(SFRs)
2FFh
200h
3FFh
300h
4FFh
400h
5FFh
500h
6FFh
600h
7FFh
700h
8FFh
800h
9FFh
900h
AFFh
A00h
BFFh
B00h
CFFh
C00h
DFFh
D00h
E00h
Bank 3
Bank 4
Bank 5
Bank 6
Bank 7
Bank 8
Bank 9
Bank 10
Bank 11
Bank 12
Bank 13
FFh
00h
FFh
00h
FFh
00h
FFh
00h
FFh
00h
FFh
00h
FFh
00h
FFh
00h
FFh
00h
FFh
00h
FFh
00h
FFh
00h
GPR
FFh
00h
= 0011
= 0100
= 0101
= 0111
= 1000
= 1001
= 1010
= 1011
= 1100
= 1101
= 1110
Unused
Read 00h
Unused
© 2007 Microchip Technology Inc. Preliminary DS39631B-page 61
PIC18F2420/2520/4420/4520
FIGURE 5-6: DATA MEMORY MAP FOR PIC18F2520/4520 DEVICES
Bank 0
Bank 1
Bank 14
Bank 15
BSR<3:0> Data Memory Map
= 0000
= 0001
= 1111
080h
07Fh
F80h
FFFh
00h
7Fh
80h
FFh
Access Bank
When ‘a’ = 0:
The BSR is ignored and the
Access Bank is used.
The first 128 bytes are
general purpose RAM
(from Bank 0).
The second 128 bytes are
Special Function Registers
(from Bank 15).
When ‘a’ = 1:
The BSR specifies the Bank
used by the instruction.
F7Fh
F00h
EFFh
1FFh
100h
0FFh
Access RAM 000h
FFh
00h
FFh
00h
FFh
00h
GPR
GPR
SFR
Access RAM High
Access RAM Low
Bank 2
= 0110
= 0010
(SFRs)
2FFh
200h
3FFh
300h
4FFh
400h
5FFh
500h
6FFh
600h
7FFh
700h
8FFh
800h
9FFh
900h
AFFh
A00h
BFFh
B00h
CFFh
C00h
DFFh
D00h
E00h
Bank 3
Bank 4
Bank 5
Bank 6
Bank 7
Bank 8
Bank 9
Bank 10
Bank 11
Bank 12
Bank 13
FFh
00h
FFh
00h
FFh
00h
FFh
00h
FFh
00h
FFh
00h
FFh
00h
FFh
00h
FFh
00h
FFh
00h
FFh
00h
FFh
00h
GPR
FFh
00h
= 0011
= 0100
= 0101
= 0111
= 1000
= 1001
= 1010
= 1011
= 1100
= 1101
= 1110
Unused
Read 00h
Unused
GPR
GPR
GPR
PIC18F2420/2520/4420/4520
DS39631B-page 62 Preliminary © 2007 Microchip Technology Inc.
FIGURE 5-7: USE OF THE BANK SELECT REGISTER (DIRECT ADDRESSING)
5.3.2 ACCESS BANK
While the use of the BSR with an embedded 8-bit
address allows users to address the entire range of
data memory, it also means that the user must always
ensure that the correct bank is selected. Otherwise,
data may be read from or written to the wrong location.
This can be disastrous if a GPR is the intended target
of an operation, but an SFR is written to instead.
Verifying and/or changing the BSR for each read or
write to data memory can become very inefficient.
To streamline access for the most commonly used data
memory locations, the data memory is configured with
an Access Bank, which allows users to access a
mapped block of memory without specifying a BSR.
The Access Bank consists of the first 128 bytes of
memory (00h-7Fh) in Bank 0 and the last 128 bytes of
memory (80h-FFh) in Block 15. The lower half is known
as the “Access RAM” and is composed of GPRs. This
upper half is also where the device’s SFRs are
mapped. These two areas are mapped contiguously in
the Access Bank and can be addressed in a linear
fashion by an 8-bit address (Figure 5-5).
The Access Bank is used by core PIC18 instructions
that include the Access RAM bit (the ‘a’ parameter in
the instruction). When ‘a’ is equal to ‘1’, the instruction
uses the BSR and the 8-bit address included in the
opcode for the data memory address. When ‘a’ is ‘0’,
however, the instruction is forced to use the Access
Bank address map; the current value of the BSR is
ignored entirely.
Using this “forced” addressing allows the instruction to
operate on a data address in a single cycle, without
updating the BSR first. For 8-bit addresses of 80h and
above, this means that users can evaluate and operate
on SFRs more efficiently. The Access RAM below 80h
is a good place for data values that the user might need
to access rapidly, such as immediate computational
results or common program variables. Access RAM
also allows for faster and more code efficient context
saving and switching of variables.
The mapping of the Access Bank is slightly different
when the extended instruction set is enabled (XINST
configuration bit = 1). This is discussed in more detail
in Section 5.5.3 “Mapping the Access Bank in
Indexed Literal Offset Mode”.
5.3.3 GENERAL PURPOSE REGISTER
FILE
PIC18 devices may have banked memory in the GPR
area. This is data RAM, which is available for use by all
instructions. GPRs start at the bottom of Bank 0
(address 000h) and grow upwards towards the bottom of
the SFR area. GPRs are not initialized by a Power-on
Reset and are unchanged on all other Resets.
Note 1: The Access RAM bit of the instruction can be used to force an override of the selected bank (BSR<3:0>) to
the registers of the Access Bank.
2: The MOVFF instruction embeds the entire 12-bit address in the instruction.
Data Memory
Bank Select(2)
7 0
From Opcode(2)
0 0 0 0
000h
100h
200h
300h
F00h
E00h
FFFh
Bank 0
Bank 1
Bank 2
Bank 14
Bank 15
00h
FFh
00h
FFh
00h
FFh
00h
FFh
00h
FFh
00h
FFh
Bank 3
through
Bank 13
0 0 1 1 1 1 1 1 1 1 1 1
7 0
BSR(1)
© 2007 Microchip Technology Inc. Preliminary DS39631B-page 63
PIC18F2420/2520/4420/4520
5.3.4 SPECIAL FUNCTION REGISTERS
The Special Function Registers (SFRs) are registers
used by the CPU and peripheral modules for controlling
the desired operation of the device. These registers are
implemented as static RAM. SFRs start at the top of
data memory (FFFh) and extend downward to occupy
the top half of Bank 15 (F80h to FFFh). A list of these
registers is given in Table 5-1 and Table 5-2.
The SFRs can be classified into two sets: those associated
with the “core” device functionality (ALU, Resets
and interrupts) and those related to the peripheral functions.
The reset and interrupt registers are described in
their respective chapters, while the ALU’s Status register
is described later in this section. Registers related to
the operation of a peripheral feature are described in
the chapter for that peripheral.
The SFRs are typically distributed among the
peripherals whose functions they control. Unused SFR
locations are unimplemented and read as ‘0’s.
TABLE 5-1: SPECIAL FUNCTION REGISTER MAP FOR PIC18F2420/2520/4420/4520 DEVICES
Address Name Address Name Address Name Address Name
FFFh TOSU FDFh INDF2(1) FBFh CCPR1H F9Fh IPR1
FFEh TOSH FDEh POSTINC2(1) FBEh CCPR1L F9Eh PIR1
FFDh TOSL FDDh POSTDEC2(1) FBDh CCP1CON F9Dh PIE1
FFCh STKPTR FDCh PREINC2(1) FBCh CCPR2H F9Ch —(2)
FFBh PCLATU FDBh PLUSW2(1) FBBh CCPR2L F9Bh OSCTUNE
FFAh PCLATH FDAh FSR2H FBAh CCP2CON F9Ah —(2)
FF9h PCL FD9h FSR2L FB9h —(2) F99h —(2)
FF8h TBLPTRU FD8h STATUS FB8h BAUDCON F98h —(2)
FF7h TBLPTRH FD7h TMR0H FB7h PWM1CON(3) F97h —(2)
FF6h TBLPTRL FD6h TMR0L FB6h ECCP1AS(3) F96h TRISE(3)
FF5h TABLAT FD5h T0CON FB5h CVRCON F95h TRISD(3)
FF4h PRODH FD4h —(2) FB4h CMCON F94h TRISC
FF3h PRODL FD3h OSCCON FB3h TMR3H F93h TRISB
FF2h INTCON FD2h HLVDCON FB2h TMR3L F92h TRISA
FF1h INTCON2 FD1h WDTCON FB1h T3CON F91h —(2)
FF0h INTCON3 FD0h RCON FB0h SPBRGH F90h —(2)
FEFh INDF0(1) FCFh TMR1H FAFh SPBRG F8Fh —(2)
FEEh POSTINC0(1) FCEh TMR1L FAEh RCREG F8Eh —(2)
FEDh POSTDEC0(1) FCDh T1CON FADh TXREG F8Dh LATE(3)
FECh PREINC0(1) FCCh TMR2 FACh TXSTA F8Ch LATD(3)
FEBh PLUSW0(1) FCBh PR2 FABh RCSTA F8Bh LATC
FEAh FSR0H FCAh T2CON FAAh —(2) F8Ah LATB
FE9h FSR0L FC9h SSPBUF FA9h EEADR F89h LATA
FE8h WREG FC8h SSPADD FA8h EEDATA F88h —(2)
FE7h INDF1(1) FC7h SSPSTAT FA7h EECON2(1) F87h —(2)
FE6h POSTINC1(1) FC6h SSPCON1 FA6h EECON1 F86h —(2)
FE5h POSTDEC1(1) FC5h SSPCON2 FA5h —(2) F85h —(2)
FE4h PREINC1(1) FC4h ADRESH FA4h —(2) F84h PORTE(3)
FE3h PLUSW1(1) FC3h ADRESL FA3h —(2) F83h PORTD(3)
FE2h FSR1H FC2h ADCON0 FA2h IPR2 F82h PORTC
FE1h FSR1L FC1h ADCON1 FA1h PIR2 F81h PORTB
FE0h BSR FC0h ADCON2 FA0h PIE2 F80h PORTA
Note 1: This is not a physical register.
2: Unimplemented registers are read as ‘0’.
3: This register is not available on 28-pin devices.
PIC18F2420/2520/4420/4520
DS39631B-page 64 Preliminary © 2007 Microchip Technology Inc.
TABLE 5-2: REGISTER FILE SUMMARY (PIC18F2420/2520/4420/4520)
File Name Bit 7 Bit 6 Bit 5 Bit 4 Bit 3 Bit 2 Bit 1 Bit 0 Value on
POR, BOR
Details
on page:
TOSU — — — Top-of-Stack Upper Byte (TOS<20:16>) ---0 0000 49, 54
TOSH Top-of-Stack, High Byte (TOS<15:8>) 0000 0000 49, 54
TOSL Top-of-Stack, Low Byte (TOS<7:0>) 0000 0000 49, 54
STKPTR STKFUL STKUNF — SP4 SP3 SP2 SP1 SP0 00-0 0000 49, 55
PCLATU — — — Holding Register for PC<20:16> ---0 0000 49, 54
PCLATH Holding Register for PC<15:8> 0000 0000 49, 54
PCL PC, Low Byte (PC<7:0>) 0000 0000 49, 54
TBLPTRU — — bit 21 Program Memory Table Pointer Upper Byte (TBLPTR<20:16>) --00 0000 49, 76
TBLPTRH Program Memory Table Pointer, High Byte (TBLPTR<15:8>) 0000 0000 49, 76
TBLPTRL Program Memory Table Pointer, Low Byte (TBLPTR<7:0>) 0000 0000 49, 76
TABLAT Program Memory Table Latch 0000 0000 49, 76
PRODH Product Register, High Byte xxxx xxxx 49, 89
PRODL Product Register, Low Byte xxxx xxxx 49, 89
INTCON GIE/GIEH PEIE/GIEL TMR0IE INT0IE RBIE TMR0IF INT0IF RBIF 0000 000x 49, 93
INTCON2 RBPU INTEDG0 INTEDG1 INTEDG2 — TMR0IP — RBIP 1111 -1-1 49, 94
INTCON3 INT2IP INT1IP — INT2IE INT1IE — INT2IF INT1IF 11-0 0-00 49, 95
INDF0 Uses contents of FSR0 to address data memory – value of FSR0 not changed (not a physical register) N/A 49, 69
POSTINC0 Uses contents of FSR0 to address data memory – value of FSR0 post-incremented (not a physical register) N/A 49, 69
POSTDEC0 Uses contents of FSR0 to address data memory – value of FSR0 post-decremented (not a physical register) N/A 49, 69
PREINC0 Uses contents of FSR0 to address data memory – value of FSR0 pre-incremented (not a physical register) N/A 49, 69
PLUSW0 Uses contents of FSR0 to address data memory – value of FSR0 pre-incremented (not a physical register) –
value of FSR0 offset by W
N/A 49, 69
FSR0H — — — — Indirect Data Memory Address Pointer 0, High Byte ---- 0000 49, 69
FSR0L Indirect Data Memory Address Pointer 0, Low Byte xxxx xxxx 49, 69
WREG Working Register xxxx xxxx 49
INDF1 Uses contents of FSR1 to address data memory – value of FSR1 not changed (not a physical register) N/A 49, 69
POSTINC1 Uses contents of FSR1 to address data memory – value of FSR1 post-incremented (not a physical register) N/A 49, 69
POSTDEC1 Uses contents of FSR1 to address data memory – value of FSR1 post-decremented (not a physical register) N/A 49, 69
PREINC1 Uses contents of FSR1 to address data memory – value of FSR1 pre-incremented (not a physical register) N/A 49, 69
PLUSW1 Uses contents of FSR1 to address data memory – value of FSR1 pre-incremented (not a physical register) –
value of FSR1 offset by W
N/A 49, 69
FSR1H — — — — Indirect Data Memory Address Pointer 1, High Byte ---- 0000 50, 69
FSR1L Indirect Data Memory Address Pointer 1, Low Byte xxxx xxxx 50, 69
BSR — — — — Bank Select Register ---- 0000 50, 59
INDF2 Uses contents of FSR2 to address data memory – value of FSR2 not changed (not a physical register) N/A 50, 69
POSTINC2 Uses contents of FSR2 to address data memory – value of FSR2 post-incremented (not a physical register) N/A 50, 69
POSTDEC2 Uses contents of FSR2 to address data memory – value of FSR2 post-decremented (not a physical register) N/A 50, 69
PREINC2 Uses contents of FSR2 to address data memory – value of FSR2 pre-incremented (not a physical register) N/A 50, 69
PLUSW2 Uses contents of FSR2 to address data memory – value of FSR2 pre-incremented (not a physical register) –
value of FSR2 offset by W
N/A 50, 69
FSR2H — — — — Indirect Data Memory Address Pointer 2, High Byte ---- 0000 50, 69
FSR2L Indirect Data Memory Address Pointer 2, Low Byte xxxx xxxx 50, 69
STATUS — — — N OV Z DC C ---x xxxx 50, 67
Legend: x = unknown, u = unchanged, — = unimplemented, q = value depends on condition
Note 1: The SBOREN bit is only available when the BOREN1:BOREN0 configuration bits = 01; otherwise it is disabled and reads as ‘0’. See
Section 4.4 “Brown-out Reset (BOR)”.
2: These registers and/or bits are not implemented on 28-pin devices and are read as ‘0’. Reset values are shown for 40/44-pin devices;
individual unimplemented bits should be interpreted as ‘-’.
3: The PLLEN bit is only available in specific oscillator configuration; otherwise it is disabled and reads as ‘0’. See Section 2.6.4 “PLL in
INTOSC Modes”.
4: The RE3 bit is only available when Master Clear Reset is disabled (MCLRE configuration bit = 0). Otherwise, RE3 reads as ‘0’. This bit is
read-only.
5: RA6/RA7 and their associated latch and direction bits are individually configured as port pins based on various primary oscillator modes.
When disabled, these bits read as ‘0’.
© 2007 Microchip Technology Inc. Preliminary DS39631B-page 65
PIC18F2420/2520/4420/4520
TMR0H Timer0 Register, High Byte 0000 0000 50, 125
TMR0L Timer0 Register, Low Byte xxxx xxxx 50, 125
T0CON TMR0ON T08BIT T0CS T0SE PSA T0PS2 T0PS1 T0PS0 1111 1111 50, 123
OSCCON IDLEN IRCF2 IRCF1 IRCF0 OSTS IOFS SCS1 SCS0 0100 q000 30, 50
HLVDCON VDIRMAG — IRVST HLVDEN HLVDL3 HLVDL2 HLVDL1 HLVDL0 0-00 0101 50, 245
WDTCON — — — — — — — SWDTEN --- ---0 50, 259
RCON IPEN SBOREN(1) — RI TO PD POR BOR 0q-1 11q0 42, 48,
102
TMR1H Timer1 Register, High Byte xxxx xxxx 50, 131
TMR1L Timer1 Register, Low Bytes xxxx xxxx 50, 131
T1CON RD16 T1RUN T1CKPS1 T1CKPS0 T1OSCEN T1SYNC TMR1CS TMR1ON 0000 0000 50, 127
TMR2 Timer2 Register 0000 0000 50, 134
PR2 Timer2 Period Register 1111 1111 50, 134
T2CON — T2OUTPS3 T2OUTPS2 T2OUTPS1 T2OUTPS0 TMR2ON T2CKPS1 T2CKPS0 -000 0000 50, 133
SSPBUF SSP Receive Buffer/Transmit Register xxxx xxxx 50, 169,
170
SSPADD SSP Address Register in I2C Slave Mode. SSP Baud Rate Reload Register in I2C Master Mode. 0000 0000 50, 170
SSPSTAT SMP CKE D/A P S R/W UA BF 0000 0000 50, 162,
171
SSPCON1 WCOL SSPOV SSPEN CKP SSPM3 SSPM2 SSPM1 SSPM0 0000 0000 50, 163,
172
SSPCON2 GCEN ACKSTAT ACKDT ACKEN RCEN PEN RSEN SEN 0000 0000 50, 173
ADRESH A/D Result Register, High Byte xxxx xxxx 51, 232
ADRESL A/D Result Register, Low Byte xxxx xxxx 51, 232
ADCON0 — — CHS3 CHS2 CHS1 CHS0 GO/DONE ADON --00 0000 51, 223
ADCON1 — — VCFG1 VCFG0 PCFG3 PCFG2 PCFG1 PCFG0 --00 0qqq 51, 224
ADCON2 ADFM — ACQT2 ACQT1 ACQT0 ADCS2 ADCS1 ADCS0 0-00 0000 51, 225
CCPR1H Capture/Compare/PWM Register 1, High Byte xxxx xxxx 51, 140
CCPR1L Capture/Compare/PWM Register 1, Low Byte xxxx xxxx 51, 140
CCP1CON P1M1(2) P1M0(2) DC1B1 DC1B0 CCP1M3 CCP1M2 CCP1M1 CCP1M0 0000 0000 51, 139,
147
CCPR2H Capture/Compare/PWM Register 2, High Byte xxxx xxxx 51, 140
CCPR2L Capture/Compare/PWM Register 2, Low Byte xxxx xxxx 51, 140
CCP2CON — — DC2B1 DC2B0 CCP2M3 CCP2M2 CCP2M1 CCP2M0 --00 0000 51, 139
BAUDCON ABDOVF RCIDL — SCKP BRG16 — WUE ABDEN 01-0 0-00 51, 204
PWM1CON PRSEN PDC6(2) PDC5(2) PDC4(2) PDC3(2) PDC2(2) PDC1(2) PDC0(2) 0000 0000 51, 156
ECCP1AS ECCPASE ECCPAS2 ECCPAS1 ECCPAS0 PSSAC1 PSSAC0 PSSBD1(2) PSSBD0(2) 0000 0000 51, 157
CVRCON CVREN CVROE CVRR CVRSS CVR3 CVR2 CVR1 CVR0 0000 0000 51, 239
CMCON C2OUT C1OUT C2INV C1INV CIS CM2 CM1 CM0 0000 0111 51, 233
TMR3H Timer3 Register, High Byte xxxx xxxx 51, 137
TMR3L Timer3 Register, Low Byte xxxx xxxx 51, 137
T3CON RD16 T3CCP2 T3CKPS1 T3CKPS0 T3CCP1 T3SYNC TMR3CS TMR3ON 0000 0000 51, 135
TABLE 5-2: REGISTER FILE SUMMARY (PIC18F2420/2520/4420/4520) (CONTINUED)
File Name Bit 7 Bit 6 Bit 5 Bit 4 Bit 3 Bit 2 Bit 1 Bit 0 Value on
POR, BOR
Details
on page:
Legend: x = unknown, u = unchanged, — = unimplemented, q = value depends on condition
Note 1: The SBOREN bit is only available when the BOREN1:BOREN0 configuration bits = 01; otherwise it is disabled and reads as ‘0’. See
Section 4.4 “Brown-out Reset (BOR)”.
2: These registers and/or bits are not implemented on 28-pin devices and are read as ‘0’. Reset values are shown for 40/44-pin devices;
individual unimplemented bits should be interpreted as ‘-’.
3: The PLLEN bit is only available in specific oscillator configuration; otherwise it is disabled and reads as ‘0’. See Section 2.6.4 “PLL in
INTOSC Modes”.
4: The RE3 bit is only available when Master Clear Reset is disabled (MCLRE configuration bit = 0). Otherwise, RE3 reads as ‘0’. This bit is
read-only.
5: RA6/RA7 and their associated latch and direction bits are individually configured as port pins based on various primary oscillator modes.
When disabled, these bits read as ‘0’.
PIC18F2420/2520/4420/4520
DS39631B-page 66 Preliminary © 2007 Microchip Technology Inc.
SPBRGH EUSART Baud Rate Generator Register, High Byte 0000 0000 51, 206
SPBRG EUSART Baud Rate Generator Register, Low Byte 0000 0000 51, 206
RCREG EUSART Receive Register 0000 0000 51, 213
TXREG EUSART Transmit Register 0000 0000 51, 211
TXSTA CSRC TX9 TXEN SYNC SENDB BRGH TRMT TX9D 0000 0010 51, 202
RCSTA SPEN RX9 SREN CREN ADDEN FERR OERR RX9D 0000 000x 51, 203
EEADR EEPROM Address Register 0000 0000 51, 74, 83
EEDATA EEPROM Data Register 0000 0000 51, 74, 83
EECON2 EEPROM Control Register 2 (not a physical register) 0000 0000 51, 74, 83
EECON1 EEPGD CFGS — FREE WRERR WREN WR RD xx-0 x000 51, 75, 84
IPR2 OSCFIP CMIP — EEIP BCLIP HLVDIP TMR3IP CCP2IP 11-1 1111 52, 101
PIR2 OSCFIF CMIF — EEIF BCLIF HLVDIF TMR3IF CCP2IF 00-0 0000 52, 97
PIE2 OSCFIE CMIE — EEIE BCLIE HLVDIE TMR3IE CCP2IE 00-0 0000 52, 99
IPR1 PSPIP(2) ADIP RCIP TXIP SSPIP CCP1IP TMR2IP TMR1IP 1111 1111 52, 100
PIR1 PSPIF(2) ADIF RCIF TXIF SSPIF CCP1IF TMR2IF TMR1IF 0000 0000 52, 96
PIE1 PSPIE(2) ADIE RCIE TXIE SSPIE CCP1IE TMR2IE TMR1IE 0000 0000 52, 98
OSCTUNE INTSRC PLLEN(3) — TUN4 TUN3 TUN2 TUN1 TUN0 0q-0 0000 27, 52
TRISE(2) IBF OBF IBOV PSPMODE — TRISE2 TRISE1 TRISE0 0000 -111 52, 118
TRISD(2) PORTD Data Direction Control Register 1111 1111 52, 114
TRISC PORTC Data Direction Control Register 1111 1111 52, 111
TRISB PORTB Data Direction Control Register 1111 1111 52, 108
TRISA TRISA7(5) TRISA6(5) Data Direction Control Register for PORTA 1111 1111 52, 105
LATE(2) — — — — — PORTE Data Latch Register
(Read and Write to Data Latch)
---- -xxx 52, 117
LATD(2) PORTD Data Latch Register (Read and Write to Data Latch) xxxx xxxx 52, 114
LATC PORTC Data Latch Register (Read and Write to Data Latch) xxxx xxxx 52, 111
LATB PORTB Data Latch Register (Read and Write to Data Latch) xxxx xxxx 52, 108
LATA LATA7(5) LATA6(5) PORTA Data Latch Register (Read and Write to Data Latch) xxxx xxxx 52, 105
PORTE — — — — RE3(4) RE2(2) RE1(2) RE0(2) ---- xxxx 52, 117
PORTD(2) RD7 RD6 RD5 RD4 RD3 RD2 RD1 RD0 xxxx xxxx 52, 114
PORTC RC7 RC6 RC5 RC4 RC3 RC2 RC1 RC0 xxxx xxxx 52, 111
PORTB RB7 RB6 RB5 RB4 RB3 RB2 RB1 RB0 xxxx xxxx 52, 108
PORTA RA7(5) RA6(5) RA5 RA4 RA3 RA2 RA1 RA0 xx0x 0000 52, 105
TABLE 5-2: REGISTER FILE SUMMARY (PIC18F2420/2520/4420/4520) (CONTINUED)
File Name Bit 7 Bit 6 Bit 5 Bit 4 Bit 3 Bit 2 Bit 1 Bit 0 Value on
POR, BOR
Details
on page:
Legend: x = unknown, u = unchanged, — = unimplemented, q = value depends on condition
Note 1: The SBOREN bit is only available when the BOREN1:BOREN0 configuration bits = 01; otherwise it is disabled and reads as ‘0’. See
Section 4.4 “Brown-out Reset (BOR)”.
2: These registers and/or bits are not implemented on 28-pin devices and are read as ‘0’. Reset values are shown for 40/44-pin devices;
individual unimplemented bits should be interpreted as ‘-’.
3: The PLLEN bit is only available in specific oscillator configuration; otherwise it is disabled and reads as ‘0’. See Section 2.6.4 “PLL in
INTOSC Modes”.
4: The RE3 bit is only available when Master Clear Reset is disabled (MCLRE configuration bit = 0). Otherwise, RE3 reads as ‘0’. This bit is
read-only.
5: RA6/RA7 and their associated latch and direction bits are individually configured as port pins based on various primary oscillator modes.
When disabled, these bits read as ‘0’.
© 2007 Microchip Technology Inc. Preliminary DS39631B-page 67
PIC18F2420/2520/4420/4520
5.3.5 STATUS REGISTER
The Status register, shown in Register 5-2, contains the
arithmetic status of the ALU. As with any other SFR, it
can be the operand for any instruction.
If the Status register is the destination for an instruction
that affects the Z, DC, C, OV or N bits, the results of the
instruction are not written; instead, the Status register
is updated according to the instruction performed.
Therefore, the result of an instruction with the Status
register as its destination may be different than
intended. As an example, CLRF STATUS will set the Z
bit and leave the remaining status bits unchanged
(‘000u u1uu’).
It is recommended that only BCF, BSF, SWAPF, MOVFF
and MOVWF instructions are used to alter the Status
register, because these instructions do not affect the Z,
C, DC, OV or N bits in the Status register.
For other instructions that do not affect Status bits, see
the instruction set summaries in Table 24-2 and
Table 24-3.
REGISTER 5-2: STATUS REGISTER
Note: The C and DC bits operate as the borrow
and digit borrow bits, respectively, in
subtraction.
U-0 U-0 U-0 R/W-x R/W-x R/W-x R/W-x R/W-x
— — — N OV Z DC C
bit 7 bit 0
bit 7-5 Unimplemented: Read as ‘0’
bit 4 N: Negative bit
This bit is used for signed arithmetic (2’s complement). It indicates whether the result was
negative (ALU MSB = 1).
1 = Result was negative
0 = Result was positive
bit 3 OV: Overflow bit
This bit is used for signed arithmetic (2’s complement). It indicates an overflow of the 7-bit
magnitude which causes the sign bit (bit 7 of the result) to change state.
1 = Overflow occurred for signed arithmetic (in this arithmetic operation)
0 = No overflow occurred
bit 2 Z: Zero bit
1 = The result of an arithmetic or logic operation is zero
0 = The result of an arithmetic or logic operation is not zero
bit 1 DC: Digit Carry/borrow bit
For ADDWF, ADDLW, SUBLW and SUBWF instructions:
1 = A carry-out from the 4th low-order bit of the result occurred
0 = No carry-out from the 4th low-order bit of the result
Note: For borrow, the polarity is reversed. A subtraction is executed by adding the 2’s
complement of the second operand. For rotate (RRF, RLF) instructions, this bit is
loaded with either bit 4 or bit 3 of the source register.
bit 0 C: Carry/borrow bit
For ADDWF, ADDLW, SUBLW and SUBWF instructions:
1 = A carry-out from the Most Significant bit of the result occurred
0 = No carry-out from the Most Significant bit of the result occurred
Note: For borrow, the polarity is reversed. A subtraction is executed by adding the 2’s
complement of the second operand. For rotate (RRF, RLF) instructions, this bit is
loaded with either the high or low-order bit of the source register.
Legend:
R = Readable bit W = Writable bit U = Unimplemented bit, read as ‘0’
-n = Value at POR ‘1’ = Bit is set ‘0’ = Bit is cleared x = Bit is unknown
PIC18F2420/2520/4420/4520
DS39631B-page 68 Preliminary © 2007 Microchip Technology Inc.
5.4 Data Addressing Modes
While the program memory can be addressed in only
one way – through the program counter – information
in the data memory space can be addressed in several
ways. For most instructions, the addressing mode is
fixed. Other instructions may use up to three modes,
depending on which operands are used and whether or
not the extended instruction set is enabled.
The addressing modes are:
• Inherent
• Literal
• Direct
• Indirect
An additional addressing mode, Indexed Literal Offset,
is available when the extended instruction set is
enabled (XINST configuration bit = 1). Its operation is
discussed in greater detail in Section 5.5.1 “Indexed
Addressing with Literal Offset”.
5.4.1 INHERENT AND LITERAL
ADDRESSING
Many PIC18 control instructions do not need any argument
at all; they either perform an operation that globally
affects the device or they operate implicitly on one
register. This addressing mode is known as Inherent
Addressing. Examples include SLEEP, RESET and DAW.
Other instructions work in a similar way but require an
additional explicit argument in the opcode. This is
known as Literal Addressing mode because they
require some literal value as an argument. Examples
include ADDLW and MOVLW, which respectively, add or
move a literal value to the W register. Other examples
include CALL and GOTO, which include a 20-bit
program memory address.
5.4.2 DIRECT ADDRESSING
Direct addressing specifies all or part of the source
and/or destination address of the operation within the
opcode itself. The options are specified by the
arguments accompanying the instruction.
In the core PIC18 instruction set, bit-oriented and byteoriented
instructions use some version of direct
addressing by default. All of these instructions include
some 8-bit literal address as their Least Significant
Byte. This address specifies either a register address in
one of the banks of data RAM (Section 5.3.3 “General
Purpose Register File”) or a location in the Access
Bank (Section 5.3.2 “Access Bank”) as the data
source for the instruction.
The Access RAM bit ‘a’ determines how the address is
interpreted. When ‘a’ is ‘1’, the contents of the BSR
(Section 5.3.1 “Bank Select Register (BSR)”) are
used with the address to determine the complete 12-bit
address of the register. When ‘a’ is ‘0’, the address is
interpreted as being a register in the Access Bank.
Addressing that uses the Access RAM is sometimes
also known as Direct Forced Addressing mode.
A few instructions, such as MOVFF, include the entire
12-bit address (either source or destination) in their
opcodes. In these cases, the BSR is ignored entirely.
The destination of the operation’s results is determined
by the destination bit ‘d’. When ‘d’ is ‘1’, the results are
stored back in the source register, overwriting its original
contents. When ‘d’ is ‘0’, the results are stored in
the W register. Instructions without the ‘d’ argument
have a destination that is implicit in the instruction; their
destination is either the target register being operated
on or the W register.
5.4.3 INDIRECT ADDRESSING
Indirect addressing allows the user to access a location
in data memory without giving a fixed address in the
instruction. This is done by using File Select Registers
(FSRs) as pointers to the locations to be read or written
to. Since the FSRs are themselves located in RAM as
Special File Registers, they can also be directly manipulated
under program control. This makes FSRs very
useful in implementing data structures, such as tables
and arrays in data memory.
The registers for indirect addressing are also
implemented with Indirect File Operands (INDFs) that
permit automatic manipulation of the pointer value with
auto-incrementing, auto-decrementing or offsetting
with another value. This allows for efficient code, using
loops, such as the example of clearing an entire RAM
bank in Example 5-5.
EXAMPLE 5-5: HOW TO CLEAR RAM
(BANK 1) USING
INDIRECT ADDRESSING
Note: The execution of some instructions in the
core PIC18 instruction set are changed
when the PIC18 extended instruction set is
enabled. See Section 5.5 “Data Memory
and the Extended Instruction Set” for
more information.
LFSR FSR0, 100h ;
NEXT CLRF POSTINC0 ; Clear INDF
; register then
; inc pointer
BTFSS FSR0H, 1 ; All done with
; Bank1?
BRA NEXT ; NO, clear next
CONTINUE ; YES, continue
© 2007 Microchip Technology Inc. Preliminary DS39631B-page 69
PIC18F2420/2520/4420/4520
5.4.3.1 FSR Registers and the INDF
Operand
At the core of indirect addressing are three sets of registers:
FSR0, FSR1 and FSR2. Each represents a pair
of 8-bit registers, FSRnH and FSRnL. The four upper
bits of the FSRnH register are not used so each FSR
pair holds a 12-bit value. This represents a value that
can address the entire range of the data memory in a
linear fashion. The FSR register pairs, then, serve as
pointers to data memory locations.
Indirect addressing is accomplished with a set of
Indirect File Operands, INDF0 through INDF2. These
can be thought of as “virtual” registers: they are
mapped in the SFR space but are not physically implemented.
Reading or writing to a particular INDF register
actually accesses its corresponding FSR register pair.
A read from INDF1, for example, reads the data at the
address indicated by FSR1H:FSR1L. Instructions that
use the INDF registers as operands actually use the
contents of their corresponding FSR as a pointer to the
instruction’s target. The INDF operand is just a
convenient way of using the pointer.
Because indirect addressing uses a full 12-bit address,
data RAM banking is not necessary. Thus, the current
contents of the BSR and the Access RAM bit have no
effect on determining the target address.
5.4.3.2 FSR Registers and POSTINC,
POSTDEC, PREINC and PLUSW
In addition to the INDF operand, each FSR register pair
also has four additional indirect operands. Like INDF,
these are “virtual” registers that cannot be indirectly
read or written to. Accessing these registers actually
accesses the associated FSR register pair, but also
performs a specific action on it stored value. They are:
• POSTDEC: accesses the FSR value, then
automatically decrements it by 1 afterwards
• POSTINC: accesses the FSR value, then
automatically increments it by 1 afterwards
• PREINC: increments the FSR value by 1, then
uses it in the operation
• PLUSW: adds the signed value of the W register
(range of -127 to 128) to that of the FSR and uses
the new value in the operation.
In this context, accessing an INDF register uses the
value in the FSR registers without changing them. Similarly,
accessing a PLUSW register gives the FSR value
offset by that in the W register; neither value is actually
changed in the operation. Accessing the other virtual
registers changes the value of the FSR registers.
Operations on the FSRs with POSTDEC, POSTINC
and PREINC affect the entire register pair; that is, rollovers
of the FSRnL register from FFh to 00h carry over
to the FSRnH register. On the other hand, results of
these operations do not change the value of any flags
in the Status register (e.g., Z, N, OV, etc.).
FIGURE 5-8: INDIRECT ADDRESSING
FSR1H:FSR1L
7 0
Data Memory
000h
100h
200h
300h
F00h
E00h
FFFh
Bank 0
Bank 1
Bank 2
Bank 14
Bank 15
Bank 3
through
Bank 13
ADDWF, INDF1, 1
7 0
Using an instruction with one of the
indirect addressing registers as the
operand....
...uses the 12-bit address stored in
the FSR pair associated with that
register....
...to determine the data memory
location to be used in that operation.
In this case, the FSR1 pair contains
ECCh. This means the contents of
location ECCh will be added to that
of the W register and stored back in
ECCh.
x x x x 1 1 1 0 1 1 0 0 1 1 0 0
PIC18F2420/2520/4420/4520
DS39631B-page 70 Preliminary © 2007 Microchip Technology Inc.
The PLUSW register can be used to implement a form
of indexed addressing in the data memory space. By
manipulating the value in the W register, users can
reach addresses that are fixed offsets from pointer
addresses. In some applications, this can be used to
implement some powerful program control structure,
such as software stacks, inside of data memory.
5.4.3.3 Operations by FSRs on FSRs
Indirect addressing operations that target other FSRs
or virtual registers represent special cases. For example,
using an FSR to point to one of the virtual registers
will not result in successful operations. As a specific
case, assume that FSR0H:FSR0L contains FE7h, the
address of INDF1. Attempts to read the value of the
INDF1 using INDF0 as an operand will return 00h.
Attempts to write to INDF1 using INDF0 as the operand
will result in a NOP.
On the other hand, using the virtual registers to write to
an FSR pair may not occur as planned. In these cases,
the value will be written to the FSR pair but without any
incrementing or decrementing. Thus, writing to INDF2
or POSTDEC2 will write the same value to the
FSR2H:FSR2L.
Since the FSRs are physical registers mapped in the
SFR space, they can be manipulated through all direct
operations. Users should proceed cautiously when
working on these registers, particularly if their code
uses indirect addressing.
Similarly, operations by indirect addressing are generally
permitted on all other SFRs. Users should exercise
the appropriate caution that they do not inadvertently
change settings that might affect the operation of the
device.
5.5 Data Memory and the Extended
Instruction Set
Enabling the PIC18 extended instruction set (XINST
configuration bit = 1) significantly changes certain
aspects of data memory and its addressing. Specifically,
the use of the Access Bank for many of the core
PIC18 instructions is different; this is due to the introduction
of a new addressing mode for the data memory
space.
What does not change is just as important. The size of
the data memory space is unchanged, as well as its
linear addressing. The SFR map remains the same.
Core PIC18 instructions can still operate in both Direct
and Indirect Addressing mode; inherent and literal
instructions do not change at all. Indirect addressing
with FSR0 and FSR1 also remain unchanged.
5.5.1 INDEXED ADDRESSING WITH
LITERAL OFFSET
Enabling the PIC18 extended instruction set changes
the behavior of indirect addressing using the FSR2
register pair within Access RAM. Under the proper
conditions, instructions that use the Access Bank – that
is, most bit-oriented and byte-oriented instructions –
can invoke a form of indexed addressing using an
offset specified in the instruction. This special addressing
mode is known as Indexed Addressing with Literal
Offset, or Indexed Literal Offset mode.
When using the extended instruction set, this
addressing mode requires the following:
• The use of the Access Bank is forced (‘a’ = 0) and
• The file address argument is less than or equal to
5Fh.
Under these conditions, the file address of the instruction
is not interpreted as the lower byte of an address
(used with the BSR in direct addressing), or as an 8-bit
address in the Access Bank. Instead, the value is
interpreted as an offset value to an address pointer,
specified by FSR2. The offset and the contents of
FSR2 are added to obtain the target address of the
operation.
5.5.2 INSTRUCTIONS AFFECTED BY
INDEXED LITERAL OFFSET MODE
Any of the core PIC18 instructions that can use direct
addressing are potentially affected by the Indexed
Literal Offset Addressing mode. This includes all
byte-oriented and bit-oriented instructions, or almost
one-half of the standard PIC18 instruction set.
Instructions that only use Inherent or Literal Addressing
modes are unaffected.
Additionally, byte-oriented and bit-oriented instructions
are not affected if they do not use the Access Bank
(Access RAM bit is ‘1’), or include a file address of 60h
or above. Instructions meeting these criteria will
continue to execute as before. A comparison of the different
possible addressing modes when the extended
instruction set is enabled in shown in Figure 5-9.
Those who desire to use byte-oriented or bit-oriented
instructions in the Indexed Literal Offset mode should
note the changes to assembler syntax for this mode.
This is described in more detail in Section 24.2.1
“Extended Instruction Syntax”.
© 2007 Microchip Technology Inc. Preliminary DS39631B-page 71
PIC18F2420/2520/4420/4520
FIGURE 5-9: COMPARING ADDRESSING OPTIONS FOR BIT-ORIENTED AND
BYTE-ORIENTED INSTRUCTIONS (EXTENDED INSTRUCTION SET ENABLED)
EXAMPLE INSTRUCTION: ADDWF, f, d, a (Opcode: 0010 01da ffff ffff)
When ‘a’ = 0 and f ≥ 60h:
The instruction executes in
Direct Forced mode. ‘f’ is interpreted
as a location in the
Access RAM between 060h
and 0FFh. This is the same as
locations 060h to 07Fh
(Bank 0) and F80h to FFFh
(Bank 15) of data memory.
Locations below 60h are not
available in this addressing
mode.
When ‘a’ = 0 and f ≤ 5Fh:
The instruction executes in
Indexed Literal Offset mode. ‘f’
is interpreted as an offset to the
address value in FSR2. The
two are added together to
obtain the address of the target
register for the instruction. The
address can be anywhere in
the data memory space.
Note that in this mode, the
correct syntax is now:
ADDWF [k], d
where ‘k’ is the same as ‘f’.
When ‘a’ = 1 (all values of f):
The instruction executes in
Direct mode (also known as
Direct Long mode). ‘f’ is interpreted
as a location in one of
the 16 banks of the data
memory space. The bank is
designated by the Bank Select
Register (BSR). The address
can be in any implemented
bank in the data memory
space.
000h
060h
100h
F00h
F80h
FFFh
Valid range
00h
60h
80h
FFh
Data Memory
Access RAM
Bank 0
Bank 1
through
Bank 14
Bank 15
SFRs
000h
080h
100h
F00h
F80h
FFFh
Data Memory
Bank 0
Bank 1
through
Bank 14
Bank 15
SFRs
FSR2H FSR2L
001001da ffffffff
001001da ffffffff
000h
080h
100h
F00h
F80h
FFFh
Data Memory
Bank 0
Bank 1
through
Bank 14
Bank 15
SFRs
for ‘f’
BSR
00000000
080h
PIC18F2420/2520/4420/4520
DS39631B-page 72 Preliminary © 2007 Microchip Technology Inc.
5.5.3 MAPPING THE ACCESS BANK IN
INDEXED LITERAL OFFSET MODE
The use of Indexed Literal Offset Addressing mode
effectively changes how the first 96 locations of Access
RAM (00h to 5Fh) are mapped. Rather than containing
just the contents of the bottom half of Bank 0, this mode
maps the contents from Bank 0 and a user defined
“window” that can be located anywhere in the data
memory space. The value of FSR2 establishes the
lower boundary of the addresses mapped into the
window, while the upper boundary is defined by FSR2
plus 95 (5Fh). Addresses in the Access RAM above
5Fh are mapped as previously described (see
Section 5.3.2 “Access Bank”). An example of Access
Bank remapping in this addressing mode is shown in
Figure 5-10.
Remapping of the Access Bank applies only to operations
using the Indexed Literal Offset mode. Operations
that use the BSR (Access RAM bit is ‘1’) will continue
to use direct addressing as before.
5.6 PIC18 Instruction Execution and
the Extended Instruction Set
Enabling the extended instruction set adds eight
additional commands to the existing PIC18 instruction
set. These instructions are executed as described in
Section 24.2 “Extended Instruction Set”.
FIGURE 5-10: REMAPPING THE ACCESS BANK WITH INDEXED LITERAL OFFSET
ADDRESSING
Data Memory
000h
100h
200h
F80h
F00h
FFFh
Bank 1
Bank 15
Bank 2
through
Bank 14
SFRs
05Fh
ADDWF f, d, a
FSR2H:FSR2L = 120h
Locations in the region
from the FSR2 pointer
(120h) to the pointer plus
05Fh (17Fh) are mapped
to the bottom of the
Access RAM (000h-05Fh).
Locations in Bank 0 from
060h to 07Fh are mapped,
as usual, to the middle half
of the Access Bank.
Special File Registers at
F80h through FFFh are
mapped to 80h through
FFh, as usual.
Bank 0 addresses below
5Fh can still be addressed
by using the BSR.
Access Bank
00h
80h
FFh
7Fh
Bank 0
SFRs
Bank 1 “Window”
Bank 0
Bank 0
Window
Example Situation:
07Fh
120h
17Fh
5Fh
Bank 1
© 2007 Microchip Technology Inc. Preliminary DS39631B-page 73
PIC18F2420/2520/4420/4520
6.0 FLASH PROGRAM MEMORY
The Flash program memory is readable, writable and
erasable during normal operation over the entire VDD
range.
A read from program memory is executed on one byte
at a time. A write to program memory is executed on
blocks of 64 bytes at a time. Program memory is
erased in blocks of 64 bytes at a time. A bulk erase
operation may not be issued from user code.
Writing or erasing program memory will cease
instruction fetches until the operation is complete. The
program memory cannot be accessed during the write
or erase, therefore, code cannot execute. An internal
programming timer terminates program memory writes
and erases.
A value written to program memory does not need to be
a valid instruction. Executing a program memory
location that forms an invalid instruction results in a
NOP.
6.1 Table Reads and Table Writes
In order to read and write program memory, there are
two operations that allow the processor to move bytes
between the program memory space and the data RAM:
• Table Read (TBLRD)
• Table Write (TBLWT)
The program memory space is 16 bits wide, while the
data RAM space is 8 bits wide. Table reads and table
writes move data between these two memory spaces
through an 8-bit register (TABLAT).
Table read operations retrieve data from program
memory and places it into the data RAM space.
Figure 6-1 shows the operation of a table read with
program memory and data RAM.
Table write operations store data from the data memory
space into holding registers in program memory. The
procedure to write the contents of the holding registers
into program memory is detailed in Section 6.5 “Writing
to Flash Program Memory”. Figure 6-2 shows the
operation of a table write with program memory and data
RAM.
Table operations work with byte entities. A table block
containing data, rather than program instructions, is not
required to be word aligned. Therefore, a table block can
start and end at any byte address. If a table write is being
used to write executable code into program memory,
program instructions will need to be word aligned.
FIGURE 6-1: TABLE READ OPERATION
Table Pointer(1)
Table Latch (8-bit)
Program Memory
TBLPTRH TBLPTRL
TABLAT
TBLPTRU
Instruction: TBLRD*
Note 1: Table Pointer register points to a byte in program memory.
Program Memory
(TBLPTR)
PIC18F2420/2520/4420/4520
DS39631B-page 74 Preliminary © 2007 Microchip Technology Inc.
FIGURE 6-2: TABLE WRITE OPERATION
6.2 Control Registers
Several control registers are used in conjunction with
the TBLRD and TBLWT instructions. These include the:
• EECON1 register
• EECON2 register
• TABLAT register
• TBLPTR registers
6.2.1 EECON1 AND EECON2 REGISTERS
The EECON1 register (Register 6-1) is the control
register for memory accesses. The EECON2 register is
not a physical register; it is used exclusively in the
memory write and erase sequences. Reading
EECON2 will read all ‘0’s.
The EEPGD control bit determines if the access will be
a program or data EEPROM memory access. When
clear, any subsequent operations will operate on the
data EEPROM memory. When set, any subsequent
operations will operate on the program memory.
The CFGS control bit determines if the access will be
to the configuration/calibration registers or to program
memory/data EEPROM memory. When set,
subsequent operations will operate on configuration
registers regardless of EEPGD (see Section 23.0
“Special Features of the CPU”). When clear, memory
selection access is determined by EEPGD.
The FREE bit, when set, will allow a program memory
erase operation. When FREE is set, the erase
operation is initiated on the next WR command. When
FREE is clear, only writes are enabled.
The WREN bit, when set, will allow a write operation.
On power-up, the WREN bit is clear. The WRERR bit is
set in hardware when the WR bit is set and cleared
when the internal programming timer expires and the
write operation is complete.
The WR control bit initiates write operations. The bit
cannot be cleared, only set, in software; it is cleared in
hardware at the completion of the write operation.
Table Pointer(1) Table Latch (8-bit)
TBLPTRH TBLPTRL TABLAT
Program Memory
(TBLPTR)
TBLPTRU
Instruction: TBLWT*
Note 1: Table Pointer actually points to one of 64 holding registers, the address of which is determined by
TBLPTRL<5:0>. The process for physically writing data to the program memory array is discussed in
Section 6.5 “Writing to Flash Program Memory”.
Holding Registers
Program Memory
Note: During normal operation, the WRERR is
read as ‘1’. This can indicate that a write
operation was prematurely terminated by
a Reset, or a write operation was
attempted improperly.
Note: The EEIF interrupt flag bit (PIR2<4>) is set
when the write is complete. It must be
cleared in software.
© 2007 Microchip Technology Inc. Preliminary DS39631B-page 75
PIC18F2420/2520/4420/4520
REGISTER 6-1: EECON1 REGISTER
R/W-x R/W-x U-0 R/W-0 R/W-x R/W-0 R/S-0 R/S-0
EEPGD CFGS — FREE WRERR WREN WR RD
bit 7 bit 0
bit 7 EEPGD: Flash Program or Data EEPROM Memory Select bit
1 = Access Flash program memory
0 = Access data EEPROM memory
bit 6 CFGS: Flash Program/Data EEPROM or Configuration Select bit
1 = Access Configuration registers
0 = Access Flash program or data EEPROM memory
bit 5 Unimplemented: Read as ‘0’
bit 4 FREE: Flash Row Erase Enable bit
1 = Erase the program memory row addressed by TBLPTR on the next WR command
(cleared by completion of erase operation)
0 = Perform write only
bit 3 WRERR: Flash Program/Data EEPROM Error Flag bit
1 = A write operation is prematurely terminated (any Reset during self-timed programming in
normal operation, or an improper write attempt)
0 = The write operation completed
Note: When a WRERR occurs, the EEPGD and CFGS bits are not cleared.
This allows tracing of the error condition.
bit 2 WREN: Flash Program/Data EEPROM Write Enable bit
1 = Allows write cycles to Flash program/data EEPROM
0 = Inhibits write cycles to Flash program/data EEPROM
bit 1 WR: Write Control bit
1 = Initiates a data EEPROM erase/write cycle or a program memory erase cycle or write cycle.
(The operation is self-timed and the bit is cleared by hardware once write is complete.
The WR bit can only be set (not cleared) in software.)
0 = Write cycle to the EEPROM is complete
bit 0 RD: Read Control bit
1 = Initiates an EEPROM read (Read takes one cycle. RD is cleared in hardware. The RD bit can
only be set (not cleared) in software. RD bit cannot be set when EEPGD = 1 or CFGS = 1.)
0 = Does not initiate an EEPROM read
Legend:
R = Readable bit W = Writable bit
S = Bit can be set by software, but not cleared U = Unimplemented bit, read as ‘0’
-n = Value at POR ‘1’ = Bit is set ‘0’ = Bit is cleared x = Bit is unknown
PIC18F2420/2520/4420/4520
DS39631B-page 76 Preliminary © 2007 Microchip Technology Inc.
6.2.2 TABLAT – TABLE LATCH REGISTER
The Table Latch (TABLAT) is an 8-bit register mapped
into the SFR space. The Table Latch register is used to
hold 8-bit data during data transfers between program
memory and data RAM.
6.2.3 TBLPTR – TABLE POINTER
REGISTER
The Table Pointer (TBLPTR) register addresses a byte
within the program memory. The TBLPTR is comprised
of three SFR registers: Table Pointer Upper Byte, Table
Pointer High Byte and Table Pointer Low Byte
(TBLPTRU:TBLPTRH:TBLPTRL). These three registers
join to form a 22-bit wide pointer. The low-order
21 bits allow the device to address up to 2 Mbytes of
program memory space. The 22nd bit allows access to
the device ID, the user ID and the configuration bits.
The Table Pointer register, TBLPTR, is used by the
TBLRD and TBLWT instructions. These instructions can
update the TBLPTR in one of four ways based on the
table operation. These operations are shown in
Table 6-1. These operations on the TBLPTR only affect
the low-order 21 bits.
6.2.4 TABLE POINTER BOUNDARIES
TBLPTR is used in reads, writes and erases of the
Flash program memory.
When a TBLRD is executed, all 22 bits of the TBLPTR
determine which byte is read from program memory
into TABLAT.
When a TBLWT is executed, the six LSbs of the Table
Pointer register (TBLPTR<5:0>) determine which of the
64 program memory holding registers is written to.
When the timed write to program memory begins (via
the WR bit), the 16 MSbs of the TBLPTR
(TBLPTR<21:6>) determine which program memory
block of 64 bytes is written to. For more detail, see
Section 6.5 “Writing to Flash Program Memory”.
When an erase of program memory is executed, the
16 MSbs of the Table Pointer register (TBLPTR<21:6>)
point to the 64-byte block that will be erased. The Least
Significant bits (TBLPTR<5:0>) are ignored.
Figure 6-3 describes the relevant boundaries of
TBLPTR based on Flash program memory operations.
TABLE 6-1: TABLE POINTER OPERATIONS WITH TBLRD AND TBLWT INSTRUCTIONS
FIGURE 6-3: TABLE POINTER BOUNDARIES BASED ON OPERATION
Example Operation on Table Pointer
TBLRD*
TBLWT*
TBLPTR is not modified
TBLRD*+
TBLWT*+
TBLPTR is incremented after the read/write
TBLRD*-
TBLWT*-
TBLPTR is decremented after the read/write
TBLRD+*
TBLWT+*
TBLPTR is incremented before the read/write
21 16 15 8 7 0
TABLE ERASE/WRITE TABLE WRITE
TABLE READ – TBLPTR<21:0>
TBLPTRU TBLPTRH TBLPTRL
TBLPTR<21:6> TBLPTR<5:0>
© 2007 Microchip Technology Inc. Preliminary DS39631B-page 77
PIC18F2420/2520/4420/4520
6.3 Reading the Flash Program
Memory
The TBLRD instruction is used to retrieve data from
program memory and places it into data RAM. Table
reads from program memory are performed one byte at
a time.
TBLPTR points to a byte address in program space.
Executing TBLRD places the byte pointed to into
TABLAT. In addition, TBLPTR can be modified
automatically for the next table read operation.
The internal program memory is typically organized by
words. The Least Significant bit of the address selects
between the high and low bytes of the word. Figure 6-4
shows the interface between the internal program
memory and the TABLAT.
FIGURE 6-4: READS FROM FLASH PROGRAM MEMORY
EXAMPLE 6-1: READING A FLASH PROGRAM MEMORY WORD
(Even Byte Address)
Program Memory
(Odd Byte Address)
TBLRD
TABLAT
TBLPTR = xxxxx1
FETCH
Instruction Register
(IR) Read Register
TBLPTR = xxxxx0
MOVLW CODE_ADDR_UPPER ; Load TBLPTR with the base
MOVWF TBLPTRU ; address of the word
MOVLW CODE_ADDR_HIGH
MOVWF TBLPTRH
MOVLW CODE_ADDR_LOW
MOVWF TBLPTRL
READ_WORD
TBLRD*+ ; read into TABLAT and increment
MOVF TABLAT, W ; get data
MOVWF WORD_EVEN
TBLRD*+ ; read into TABLAT and increment
MOVFW TABLAT, W ; get data
MOVF WORD_ODD
PIC18F2420/2520/4420/4520
DS39631B-page 78 Preliminary © 2007 Microchip Technology Inc.
6.4 Erasing Flash Program Memory
The minimum erase block is 32 words or 64 bytes. Only
through the use of an external programmer, or through
ICSP control, can larger blocks of program memory be
bulk erased. Word erase in the Flash array is not
supported.
When initiating an erase sequence from the microcontroller
itself, a block of 64 bytes of program memory
is erased. The Most Significant 16 bits of the
TBLPTR<21:6> point to the block being erased.
TBLPTR<5:0> are ignored.
The EECON1 register commands the erase operation.
The EEPGD bit must be set to point to the Flash program
memory. The WREN bit must be set to enable
write operations. The FREE bit is set to select an erase
operation.
For protection, the write initiate sequence for EECON2
must be used.
A long write is necessary for erasing the internal Flash.
Instruction execution is halted while in a long write
cycle. The long write will be terminated by the internal
programming timer.
6.4.1 FLASH PROGRAM MEMORY
ERASE SEQUENCE
The sequence of events for erasing a block of internal
program memory location is:
1. Load Table Pointer register with address of row
being erased.
2. Set the EECON1 register for the erase operation:
• set EEPGD bit to point to program memory;
• clear the CFGS bit to access program memory;
• set WREN bit to enable writes;
• set FREE bit to enable the erase.
3. Disable interrupts.
4. Write 55h to EECON2.
5. Write 0AAh to EECON2.
6. Set the WR bit. This will begin the row erase
cycle.
7. The CPU will stall for duration of the erase
(about 2 ms using internal timer).
8. Re-enable interrupts.
EXAMPLE 6-2: ERASING A FLASH PROGRAM MEMORY ROW
MOVLW CODE_ADDR_UPPER ; load TBLPTR with the base
MOVWF TBLPTRU ; address of the memory block
MOVLW CODE_ADDR_HIGH
MOVWF TBLPTRH
MOVLW CODE_ADDR_LOW
MOVWF TBLPTRL
ERASE_ROW
BSF EECON1, EEPGD ; point to Flash program memory
BCF EECON1, CFGS ; access Flash program memory
BSF EECON1, WREN ; enable write to memory
BSF EECON1, FREE ; enable Row Erase operation
BCF INTCON, GIE ; disable interrupts
Required MOVLW 55h
Sequence MOVWF EECON2 ; write 55h
MOVLW 0AAh
MOVWF EECON2 ; write 0AAh
BSF EECON1, WR ; start erase (CPU stall)
BSF INTCON, GIE ; re-enable interrupts
© 2007 Microchip Technology Inc. Preliminary DS39631B-page 79
PIC18F2420/2520/4420/4520
6.5 Writing to Flash Program Memory
The minimum programming block is 32 words or
64 bytes. Word or byte programming is not supported.
Table writes are used internally to load the holding
registers needed to program the Flash memory. There
are 64 holding registers used by the table writes for
programming.
Since the Table Latch (TABLAT) is only a single byte,
the TBLWT instruction may need to be executed 64
times for each programming operation. All of the table
write operations will essentially be short writes because
only the holding registers are written. At the end of
updating the 64 holding registers, the EECON1 register
must be written to in order to start the programming
operation with a long write.
The long write is necessary for programming the internal
Flash. Instruction execution is halted while in a long
write cycle. The long write will be terminated by the
internal programming timer.
The EEPROM on-chip timer controls the write time.
The write/erase voltages are generated by an on-chip
charge pump, rated to operate over the voltage range
of the device.
FIGURE 6-5: TABLE WRITES TO FLASH PROGRAM MEMORY
6.5.1 FLASH PROGRAM MEMORY WRITE
SEQUENCE
The sequence of events for programming an internal
program memory location should be:
1. Read 64 bytes into RAM.
2. Update data values in RAM as necessary.
3. Load Table Pointer register with address being
erased.
4. Execute the row erase procedure.
5. Load Table Pointer register with address of first
byte being written.
6. Write the 64 bytes into the holding registers with
auto-increment.
7. Set the EECON1 register for the write operation:
• set EEPGD bit to point to program memory;
• clear the CFGS bit to access program memory;
• set WREN to enable byte writes.
8. Disable interrupts.
9. Write 55h to EECON2.
10. Write 0AAh to EECON2.
11. Set the WR bit. This will begin the write cycle.
12. The CPU will stall for duration of the write (about
2 ms using internal timer).
13. Re-enable interrupts.
14. Verify the memory (table read).
This procedure will require about 6 ms to update one
row of 64 bytes of memory. An example of the required
code is given in Example 6-3.
Note: The default value of the holding registers on
device Resets and after write operations is
FFh. A write of FFh to a holding register
does not modify that byte. This means that
individual bytes of program memory may be
modified, provided that the change does not
attempt to change any bit from a ‘0’ to a ‘1’.
When modifying individual bytes, it is not
necessary to load all 64 holding registers
before executing a write operation.
TABLAT
TBLPTR = xxxxx0 TBLPTR = xxxxx1 TBLPTR = xxxx3F
Write Register
TBLPTR = xxxxx2
Program Memory
Holding Register Holding Register Holding Register Holding Register
8 8 8 8
Note: Before setting the WR bit, the Table
Pointer address needs to be within the
intended address range of the 64 bytes in
the holding register.
PIC18F2420/2520/4420/4520
DS39631B-page 80 Preliminary © 2007 Microchip Technology Inc.
EXAMPLE 6-3: WRITING TO FLASH PROGRAM MEMORY
MOVLW D'64 ; number of bytes in erase block
MOVWF COUNTER
MOVLW BUFFER_ADDR_HIGH ; point to buffer
MOVWF FSR0H
MOVLW BUFFER_ADDR_LOW
MOVWF FSR0L
MOVLW CODE_ADDR_UPPER ; Load TBLPTR with the base
MOVWF TBLPTRU ; address of the memory block
MOVLW CODE_ADDR_HIGH
MOVWF TBLPTRH
MOVLW CODE_ADDR_LOW
MOVWF TBLPTRL
READ_BLOCK
TBLRD*+ ; read into TABLAT, and inc
MOVF TABLAT, W ; get data
MOVWF POSTINC0 ; store data
DECFSZ COUNTER ; done?
BRA READ_BLOCK ; repeat
MODIFY_WORD
MOVLW DATA_ADDR_HIGH ; point to buffer
MOVWF FSR0H
MOVLW DATA_ADDR_LOW
MOVWF FSR0L
MOVLW NEW_DATA_LOW ; update buffer word
MOVWF POSTINC0
MOVLW NEW_DATA_HIGH
MOVWF INDF0
ERASE_BLOCK
MOVLW CODE_ADDR_UPPER ; load TBLPTR with the base
MOVWF TBLPTRU ; address of the memory block
MOVLW CODE_ADDR_HIGH
MOVWF TBLPTRH
MOVLW CODE_ADDR_LOW
MOVWF TBLPTRL
BSF EECON1, EEPGD ; point to Flash program memory
BCF EECON1, CFGS ; access Flash program memory
BSF EECON1, WREN ; enable write to memory
BSF EECON1, FREE ; enable Row Erase operation
BCF INTCON, GIE ; disable interrupts
MOVLW 55h
Required MOVWF EECON2 ; write 55h
Sequence MOVLW 0AAh
MOVWF EECON2 ; write 0AAh
BSF EECON1, WR ; start erase (CPU stall)
BSF INTCON, GIE ; re-enable interrupts
TBLRD*- ; dummy read decrement
MOVLW BUFFER_ADDR_HIGH ; point to buffer
MOVWF FSR0H
MOVLW BUFFER_ADDR_LOW
MOVWF FSR0L
WRITE_BUFFER_BACK
MOVLW D’64 ; number of bytes in holding register
MOVWF COUNTER
WRITE_BYTE_TO_HREGS
MOVFF POSTINC0, WREG ; get low byte of buffer data
MOVWF TABLAT ; present data to table latch
TBLWT+* ; write data, perform a short write
; to internal TBLWT holding register.
DECFSZ COUNTER ; loop until buffers are full
BRA WRITE_WORD_TO_HREGS
© 2007 Microchip Technology Inc. Preliminary DS39631B-page 81
PIC18F2420/2520/4420/4520
EXAMPLE 6-3: WRITING TO FLASH PROGRAM MEMORY (CONTINUED)
6.5.2 WRITE VERIFY
Depending on the application, good programming
practice may dictate that the value written to the
memory should be verified against the original value.
This should be used in applications where excessive
writes can stress bits near the specification limit.
6.5.3 UNEXPECTED TERMINATION OF
WRITE OPERATION
If a write is terminated by an unplanned event, such as
loss of power or an unexpected Reset, the memory
location just programmed should be verified and reprogrammed
if needed. If the write operation is interrupted
by a MCLR Reset or a WDT Time-out Reset during
normal operation, the user can check the WRERR bit
and rewrite the location(s) as needed.
6.5.4 PROTECTION AGAINST
SPURIOUS WRITES
To protect against spurious writes to Flash program
memory, the write initiate sequence must also be
followed. See Section 23.0 “Special Features of the
CPU” for more detail.
6.6 Flash Program Operation During
Code Protection
See Section 23.5 “Program Verification and Code
Protection” for details on code protection of Flash
program memory.
TABLE 6-2: REGISTERS ASSOCIATED WITH PROGRAM FLASH MEMORY
PROGRAM_MEMORY
BSF EECON1, EEPGD ; point to Flash program memory
BCF EECON1, CFGS ; access Flash program memory
BSF EECON1, WREN ; enable write to memory
BCF INTCON, GIE ; disable interrupts
MOVLW 55h
Required MOVWF EECON2 ; write 55h
Sequence MOVLW 0AAh
MOVWF EECON2 ; write 0AAh
BSF EECON1, WR ; start program (CPU stall)
BSF INTCON, GIE ; re-enable interrupts
BCF EECON1, WREN ; disable write to memory
Name Bit 7 Bit 6 Bit 5 Bit 4 Bit 3 Bit 2 Bit 1 Bit 0
Reset
Values on
page
TBLPTRU — — bit 21 Program Memory Table Pointer Upper Byte (TBLPTR<20:16>) 49
TBPLTRH Program Memory Table Pointer High Byte (TBLPTR<15:8>) 49
TBLPTRL Program Memory Table Pointer Low Byte (TBLPTR<7:0>) 49
TABLAT Program Memory Table Latch 49
INTCON GIE/GIEH PEIE/GIEL TMR0IE INT0IE RBIE TMR0IF INT0IF RBIF 49
EECON2 EEPROM Control Register 2 (not a physical register) 51
EECON1 EEPGD CFGS — FREE WRERR WREN WR RD 51
IPR2 OSCFIP CMIP — EEIP BCLIP HLVDIP TMR3IP CCP2IP 52
PIR2 OSCFIF CMIF — EEIF BCLIF HLVDIF TMR3IF CCP2IF 52
PIE2 OSCFIE CMIE — EEIE BCLIE HLVDIE TMR3IE CCP2IE 52
Legend: — = unimplemented, read as ‘0’. Shaded cells are not used during Flash/EEPROM access.
PIC18F2420/2520/4420/4520
DS39631B-page 82 Preliminary © 2007 Microchip Technology Inc.
NOTES:
© 2007 Microchip Technology Inc. Preliminary DS39631B-page 83
PIC18F2420/2520/4420/4520
7.0 DATA EEPROM MEMORY
The data EEPROM is a nonvolatile memory array, separate
from the data RAM and program memory, that is
used for long-term storage of program data. It is not
directly mapped in either the register file or program
memory space but is indirectly addressed through the
Special Function Registers (SFRs). The EEPROM is
readable and writable during normal operation over the
entire VDD range.
Five SFRs are used to read and write to the data
EEPROM as well as the program memory. They are:
• EECON1
• EECON2
• EEDATA
• EEADR
The data EEPROM allows byte read and write. When
interfacing to the data memory block, EEDATA holds
the 8-bit data for read/write and the EEADR register
holds the address of the EEPROM location being
accessed.
The EEPROM data memory is rated for high erase/write
cycle endurance. A byte write automatically erases the
location and writes the new data (erase-before-write).
The write time is controlled by an on-chip timer; it will
vary with voltage and temperature as well as from chip
to chip. Please refer to parameter D122 (Table 26-1 in
Section 26.0 “Electrical Characteristics”) for exact
limits.
7.1 EEADR Register
The EEADR register is used to address the data
EEPROM for read and write operations. The 8-bit
range of the register can address a memory range of
256 bytes (00h to FFh).
7.2 EECON1 and EECON2 Registers
Access to the data EEPROM is controlled by two
registers: EECON1 and EECON2. These are the same
registers which control access to the program memory
and are used in a similar manner for the data
EEPROM.
The EECON1 register (Register 7-1) is the control register
for data and program memory access. Control bit
EEPGD determines if the access will be to program or
data EEPROM memory. When clear, operations will
access the data EEPROM memory. When set, program
memory is accessed.
Control bit, CFGS, determines if the access will be to
the configuration registers or to program memory/data
EEPROM memory. When set, subsequent operations
access configuration registers. When CFGS is clear,
the EEPGD bit selects either program Flash or data
EEPROM memory.
The WREN bit, when set, will allow a write operation.
On power-up, the WREN bit is clear. The WRERR bit is
set in hardware when the WR bit is set and cleared
when the internal programming timer expires and the
write operation is complete.
The WR control bit initiates write operations. The bit
can be set but not cleared in software. It is only cleared
in hardware at the completion of the write operation.
Control bits, RD and WR, start read and erase/write
operations, respectively. These bits are set by firmware
and cleared by hardware at the completion of the
operation.
The RD bit cannot be set when accessing program
memory (EEPGD = 1). Program memory is read using
table read instructions. See Section 6.1 “Table Reads
and Table Writes” regarding table reads.
The EECON2 register is not a physical register. It is
used exclusively in the memory write and erase
sequences. Reading EECON2 will read all ‘0’s.
Note: During normal operation, the WRERR
may read as ‘1’. This can indicate that a
write operation was prematurely terminated
by a Reset, or a write operation was
attempted improperly.
Note: The EEIF interrupt flag bit (PIR2<4>) is set
when the write is complete. It must be
cleared in software.
PIC18F2420/2520/4420/4520
DS39631B-page 84 Preliminary © 2007 Microchip Technology Inc.
REGISTER 7-1: EECON1 REGISTER
R/W-x R/W-x U-0 R/W-0 R/W-x R/W-0 R/S-0 R/S-0
EEPGD CFGS — FREE WRERR WREN WR RD
bit 7 bit 0
bit 7 EEPGD: Flash Program or Data EEPROM Memory Select bit
1 = Access Flash program memory
0 = Access data EEPROM memory
bit 6 CFGS: Flash Program/Data EEPROM or Configuration Select bit
1 = Access configuration registers
0 = Access Flash program or data EEPROM memory
bit 5 Unimplemented: Read as ‘0’
bit 4 FREE: Flash Row Erase Enable bit
1 = Erase the program memory row addressed by TBLPTR on the next WR command
(cleared by completion of erase operation)
0 = Perform write only
bit 3 WRERR: Flash Program/Data EEPROM Error Flag bit
1 = A write operation is prematurely terminated (any Reset during self-timed programming in
normal operation, or an improper write attempt)
0 = The write operation completed
Note: When a WRERR occurs, the EEPGD and CFGS bits are not cleared.
This allows tracing of the error condition.
bit 2 WREN: Flash Program/Data EEPROM Write Enable bit
1 = Allows write cycles to Flash program/data EEPROM
0 = Inhibits write cycles to Flash program/data EEPROM
bit 1 WR: Write Control bit
1 = Initiates a data EEPROM erase/write cycle or a program memory erase cycle or write cycle
(The operation is self-timed and the bit is cleared by hardware once write is complete.
The WR bit can only be set (not cleared) in software.)
0 = Write cycle to the EEPROM is complete
bit 0 RD: Read Control bit
1 = Initiates an EEPROM read (Read takes one cycle. RD is cleared in hardware. The RD bit can
only be set (not cleared) in software. RD bit cannot be set when EEPGD = 1 or CFGS = 1.)
0 = Does not initiate an EEPROM read
Legend:
R = Readable bit W = Writable bit
S = Bit can be set by software, but not cleared U = Unimplemented bit, read as ‘0’
-n = Value at POR ‘1’ = Bit is set ‘0’ = Bit is cleared x = Bit is unknown
© 2007 Microchip Technology Inc. Preliminary DS39631B-page 85
PIC18F2420/2520/4420/4520
7.3 Reading the Data EEPROM
Memory
To read a data memory location, the user must write the
address to the EEADR register, clear the EEPGD control
bit (EECON1<7>) and then set control bit, RD
(EECON1<0>). The data is available on the very next
instruction cycle; therefore, the EEDATA register can
be read by the next instruction. EEDATA will hold this
value until another read operation, or until it is written to
by the user (during a write operation).
The basic process is shown in Example 7-1.
7.4 Writing to the Data EEPROM
Memory
To write an EEPROM data location, the address must
first be written to the EEADR register and the data written
to the EEDATA register. The sequence in
Example 7-2 must be followed to initiate the write cycle.
The write will not begin if this sequence is not exactly
followed (write 55h to EECON2, write 0AAh to
EECON2, then set WR bit) for each byte. It is strongly
recommended that interrupts be disabled during this
code segment.
Additionally, the WREN bit in EECON1 must be set to
enable writes. This mechanism prevents accidental
writes to data EEPROM due to unexpected code execution
(i.e., runaway programs). The WREN bit should
be kept clear at all times, except when updating the
EEPROM. The WREN bit is not cleared by hardware.
After a write sequence has been initiated, EECON1,
EEADR and EEDATA cannot be modified. The WR bit
will be inhibited from being set unless the WREN bit is
set. Both WR and WREN cannot be set with the same
instruction.
At the completion of the write cycle, the WR bit is
cleared in hardware and the EEPROM Interrupt Flag
bit, EEIF, is set. The user may either enable this
interrupt or poll this bit. EEIF must be cleared by
software.
7.5 Write Verify
Depending on the application, good programming
practice may dictate that the value written to the
memory should be verified against the original value.
This should be used in applications where excessive
writes can stress bits near the specification limit.
EXAMPLE 7-1: DATA EEPROM READ
EXAMPLE 7-2: DATA EEPROM WRITE
MOVLW DATA_EE_ADDR ;
MOVWF EEADR ; Data Memory Address to read
BCF EECON1, EEPGD ; Point to DATA memory
BCF EECON1, CFGS ; Access EEPROM
BSF EECON1, RD ; EEPROM Read
MOVF EEDATA, W ; W = EEDATA
MOVLW DATA_EE_ADDR ;
MOVWF EEADR ; Data Memory Address to write
MOVLW DATA_EE_DATA ;
MOVWF EEDATA ; Data Memory Value to write
BCF EECON1, EEPGD ; Point to DATA memory
BCF EECON1, CFGS ; Access EEPROM
BSF EECON1, WREN ; Enable writes
BCF INTCON, GIE ; Disable Interrupts
MOVLW 55h ;
Required MOVWF EECON2 ; Write 55h
Sequence MOVLW 0AAh ;
MOVWF EECON2 ; Write 0AAh
BSF EECON1, WR ; Set WR bit to begin write
BSF INTCON, GIE ; Enable Interrupts
; User code execution
BCF EECON1, WREN ; Disable writes on write complete (EEIF set)
PIC18F2420/2520/4420/4520
DS39631B-page 86 Preliminary © 2007 Microchip Technology Inc.
7.6 Operation During Code-Protect
Data EEPROM memory has its own code-protect bits in
configuration words. External read and write
operations are disabled if code protection is enabled.
The microcontroller itself can both read and write to the
internal data EEPROM, regardless of the state of the
code-protect configuration bit. Refer to Section 23.0
“Special Features of the CPU” for additional
information.
7.7 Protection Against Spurious Write
There are conditions when the user may not want to
write to the data EEPROM memory. To protect against
spurious EEPROM writes, various mechanisms have
been implemented. On power-up, the WREN bit is
cleared. In addition, writes to the EEPROM are blocked
during the Power-up Timer period (TPWRT,
parameter 33).
The write initiate sequence and the WREN bit together
help prevent an accidental write during brown-out,
power glitch or software malfunction.
7.8 Using the Data EEPROM
The data EEPROM is a high endurance, byte
addressable array that has been optimized for the
storage of frequently changing information (e.g.,
program variables or other data that are updated
often). Frequently changing values will typically be
updated more often than specification D124. If this is
not the case, an array refresh must be performed. For
this reason, variables that change infrequently (such as
constants, IDs, calibration, etc.) should be stored in
Flash program memory.
A simple data EEPROM refresh routine is shown in
Example 7-3.
EXAMPLE 7-3: DATA EEPROM REFRESH ROUTINE
Note: If data EEPROM is only used to store
constants and/or data that changes rarely,
an array refresh is likely not required. See
specification D124.
CLRF EEADR ; Start at address 0
BCF EECON1, CFGS ; Set for memory
BCF EECON1, EEPGD ; Set for Data EEPROM
BCF INTCON, GIE ; Disable interrupts
BSF EECON1, WREN ; Enable writes
Loop ; Loop to refresh array
BSF EECON1, RD ; Read current address
MOVLW 55h ;
MOVWF EECON2 ; Write 55h
MOVLW 0AAh ;
MOVWF EECON2 ; Write 0AAh
BSF EECON1, WR ; Set WR bit to begin write
BTFSC EECON1, WR ; Wait for write to complete
BRA $-2
INCFSZ EEADR, F ; Increment address
BRA LOOP ; Not zero, do it again
BCF EECON1, WREN ; Disable writes
BSF INTCON, GIE ; Enable interrupts
© 2007 Microchip Technology Inc. Preliminary DS39631B-page 87
PIC18F2420/2520/4420/4520
TABLE 7-1: REGISTERS ASSOCIATED WITH DATA EEPROM MEMORY
Name Bit 7 Bit 6 Bit 5 Bit 4 Bit 3 Bit 2 Bit 1 Bit 0
Reset
Values
on page
INTCON GIE/GIEH PEIE/GIEL TMR0IE INT0IE RBIE TMR0IF INT0IF RBIF 49
EEADR EEPROM Address Register 51
EEDATA EEPROM Data Register 51
EECON2 EEPROM Control Register 2 (not a physical register) 51
EECON1 EEPGD CFGS — FREE WRERR WREN WR RD 51
IPR2 OSCFIP CMIP — EEIP BCLIP HLVDIP TMR3IP CCP2IP 52
PIR2 OSCFIF CMIF — EEIF BCLIF HLVDIF TMR3IF CCP2IF 52
PIE2 OSCFIE CMIE — EEIE BCLIE HLVDIE TMR3IE CCP2IE 52
Legend: — = unimplemented, read as ‘0’. Shaded cells are not used during Flash/EEPROM access.
PIC18F2420/2520/4420/4520
DS39631B-page 88 Preliminary © 2007 Microchip Technology Inc.
NOTES:
© 2007 Microchip Technology Inc. Preliminary DS39631B-page 89
PIC18F2420/2520/4420/4520
8.0 8 x 8 HARDWARE MULTIPLIER
8.1 Introduction
All PIC18 devices include an 8 x 8 hardware multiplier
as part of the ALU. The multiplier performs an unsigned
operation and yields a 16-bit result that is stored in the
product register pair, PRODH:PRODL. The multiplier’s
operation does not affect any flags in the Status
register.
Making multiplication a hardware operation allows it to
be completed in a single instruction cycle. This has the
advantages of higher computational throughput and
reduced code size for multiplication algorithms and
allows the PIC18 devices to be used in many applications
previously reserved for digital signal processors.
A comparison of various hardware and software
multiply operations, along with the savings in memory
and execution time, is shown in Table 8-1.
8.2 Operation
Example 8-1 shows the instruction sequence for an 8 x 8
unsigned multiplication. Only one instruction is required
when one of the arguments is already loaded in the
WREG register.
Example 8-2 shows the sequence to do an 8 x 8 signed
multiplication. To account for the sign bits of the arguments,
each argument’s Most Significant bit (MSb) is
tested and the appropriate subtractions are done.
EXAMPLE 8-1: 8 x 8 UNSIGNED
MULTIPLY ROUTINE
EXAMPLE 8-2: 8 x 8 SIGNED MULTIPLY
ROUTINE
TABLE 8-1: PERFORMANCE COMPARISON FOR VARIOUS MULTIPLY OPERATIONS
MOVF ARG1, W ;
MULWF ARG2 ; ARG1 * ARG2 ->
; PRODH:PRODL
MOVF ARG1, W
MULWF ARG2 ; ARG1 * ARG2 ->
; PRODH:PRODL
BTFSC ARG2, SB ; Test Sign Bit
SUBWF PRODH, F ; PRODH = PRODH
; - ARG1
MOVF ARG2, W
BTFSC ARG1, SB ; Test Sign Bit
SUBWF PRODH, F ; PRODH = PRODH
; - ARG2
Routine Multiply Method
Program
Memory
(Words)
Cycles
(Max)
Time
@ 40 MHz @ 10 MHz @ 4 MHz
8 x 8 unsigned
Without hardware multiply 13 69 6.9 μs 27.6 μs 69 μs
Hardware multiply 1 1 100 ns 400 ns 1 μs
8 x 8 signed
Without hardware multiply 33 91 9.1 μs 36.4 μs 91 μs
Hardware multiply 6 6 600 ns 2.4 μs 6 μs
16 x 16 unsigned
Without hardware multiply 21 242 24.2 μs 96.8 μs 242 μs
Hardware multiply 28 28 2.8 μs 11.2 μs 28 μs
16 x 16 signed
Without hardware multiply 52 254 25.4 μs 102.6 μs 254 μs
Hardware multiply 35 40 4.0 μs 16.0 μs 40 μs
PIC18F2420/2520/4420/4520
DS39631B-page 90 Preliminary © 2007 Microchip Technology Inc.
Example 8-3 shows the sequence to do a 16 x 16
unsigned multiplication. Equation 8-1 shows the
algorithm that is used. The 32-bit result is stored in four
registers (RES3:RES0).
EQUATION 8-1: 16 x 16 UNSIGNED
MULTIPLICATION
ALGORITHM
EXAMPLE 8-3: 16 x 16 UNSIGNED
MULTIPLY ROUTINE
Example 8-4 shows the sequence to do a 16 x 16
signed multiply. Equation 8-2 shows the algorithm
used. The 32-bit result is stored in four registers
(RES3:RES0). To account for the sign bits of the arguments,
the MSb for each argument pair is tested and
the appropriate subtractions are done.
EQUATION 8-2: 16 x 16 SIGNED
MULTIPLICATION
ALGORITHM
EXAMPLE 8-4: 16 x 16 SIGNED
MULTIPLY ROUTINE
RES3:RES0 = ARG1H:ARG1L • ARG2H:ARG2L
= (ARG1H • ARG2H • 216) +
(ARG1H • ARG2L • 28) +
(ARG1L • ARG2H • 28) +
(ARG1L • ARG2L)
MOVF ARG1L, W
MULWF ARG2L ; ARG1L * ARG2L->
; PRODH:PRODL
MOVFF PRODH, RES1 ;
MOVFF PRODL, RES0 ;
;
MOVF ARG1H, W
MULWF ARG2H ; ARG1H * ARG2H->
; PRODH:PRODL
MOVFF PRODH, RES3 ;
MOVFF PRODL, RES2 ;
;
MOVF ARG1L, W
MULWF ARG2H ; ARG1L * ARG2H->
; PRODH:PRODL
MOVF PRODL, W ;
ADDWF RES1, F ; Add cross
MOVF PRODH, W ; products
ADDWFC RES2, F ;
CLRF WREG ;
ADDWFC RES3, F ;
;
MOVF ARG1H, W ;
MULWF ARG2L ; ARG1H * ARG2L->
; PRODH:PRODL
MOVF PRODL, W ;
ADDWF RES1, F ; Add cross
MOVF PRODH, W ; products
ADDWFC RES2, F ;
CLRF WREG ;
ADDWFC RES3, F ;
RES3:RES0 = ARG1H:ARG1L • ARG2H:ARG2L
= (ARG1H • ARG2H • 216) +
(ARG1H • ARG2L • 28) +
(ARG1L • ARG2H • 28) +
(ARG1L • ARG2L) +
(-1 • ARG2H<7> • ARG1H:ARG1L • 216) +
(-1 • ARG1H<7> • ARG2H:ARG2L • 216)
MOVF ARG1L, W
MULWF ARG2L ; ARG1L * ARG2L ->
; PRODH:PRODL
MOVFF PRODH, RES1 ;
MOVFF PRODL, RES0 ;
;
MOVF ARG1H, W
MULWF ARG2H ; ARG1H * ARG2H ->
; PRODH:PRODL
MOVFF PRODH, RES3 ;
MOVFF PRODL, RES2 ;
;
MOVF ARG1L, W
MULWF ARG2H ; ARG1L * ARG2H ->
; PRODH:PRODL
MOVF PRODL, W ;
ADDWF RES1, F ; Add cross
MOVF PRODH, W ; products
ADDWFC RES2, F ;
CLRF WREG ;
ADDWFC RES3, F ;
;
MOVF ARG1H, W ;
MULWF ARG2L ; ARG1H * ARG2L ->
; PRODH:PRODL
MOVF PRODL, W ;
ADDWF RES1, F ; Add cross
MOVF PRODH, W ; products
ADDWFC RES2, F ;
CLRF WREG ;
ADDWFC RES3, F ;
;
BTFSS ARG2H, 7 ; ARG2H:ARG2L neg?
BRA SIGN_ARG1 ; no, check ARG1
MOVF ARG1L, W ;
SUBWF RES2 ;
MOVF ARG1H, W ;
SUBWFB RES3
;
SIGN_ARG1
BTFSS ARG1H, 7 ; ARG1H:ARG1L neg?
BRA CONT_CODE ; no, done
MOVF ARG2L, W ;
SUBWF RES2 ;
MOVF ARG2H, W ;
SUBWFB RES3
;
CONT_CODE
:
© 2007 Microchip Technology Inc. Preliminary DS39631B-page 91
PIC18F2420/2520/4420/4520
9.0 INTERRUPTS
The PIC18F2420/2520/4420/4520 devices have multiple
interrupt sources and an interrupt priority feature
that allows most interrupt sources to be assigned a
high priority level or a low priority level. The high priority
interrupt vector is at 0008h and the low priority interrupt
vector is at 0018h. High priority interrupt events will
interrupt any low priority interrupts that may be in
progress.
There are ten registers which are used to control
interrupt operation. These registers are:
• RCON
• INTCON
• INTCON2
• INTCON3
• PIR1, PIR2
• PIE1, PIE2
• IPR1, IPR2
It is recommended that the Microchip header files supplied
with MPLAB® IDE be used for the symbolic bit
names in these registers. This allows the assembler/
compiler to automatically take care of the placement of
these bits within the specified register.
In general, interrupt sources have three bits to control
their operation. They are:
• Flag bit to indicate that an interrupt event
occurred
• Enable bit that allows program execution to
branch to the interrupt vector address when the
flag bit is set
• Priority bit to select high priority or low priority
The interrupt priority feature is enabled by setting the
IPEN bit (RCON<7>). When interrupt priority is
enabled, there are two bits which enable interrupts
globally. Setting the GIEH bit (INTCON<7>) enables all
interrupts that have the priority bit set (high priority).
Setting the GIEL bit (INTCON<6>) enables all interrupts
that have the priority bit cleared (low priority).
When the interrupt flag, enable bit and appropriate
global interrupt enable bit are set, the interrupt will vector
immediately to address 0008h or 0018h, depending
on the priority bit setting. Individual interrupts can be
disabled through their corresponding enable bits.
When the IPEN bit is cleared (default state), the
interrupt priority feature is disabled and interrupts are
compatible with PIC® mid-range devices. In Compatibility
mode, the interrupt priority bits for each source
have no effect. INTCON<6> is the PEIE bit, which
enables/disables all peripheral interrupt sources.
INTCON<7> is the GIE bit, which enables/disables all
interrupt sources. All interrupts branch to address
0008h in Compatibility mode.
When an interrupt is responded to, the global interrupt
enable bit is cleared to disable further interrupts. If the
IPEN bit is cleared, this is the GIE bit. If interrupt priority
levels are used, this will be either the GIEH or GIEL bit.
High priority interrupt sources can interrupt a low
priority interrupt. Low priority interrupts are not
processed while high priority interrupts are in progress.
The return address is pushed onto the stack and the
PC is loaded with the interrupt vector address (0008h
or 0018h). Once in the Interrupt Service Routine, the
source(s) of the interrupt can be determined by polling
the interrupt flag bits. The interrupt flag bits must be
cleared in software before re-enabling interrupts to
avoid recursive interrupts.
The “return from interrupt” instruction, RETFIE, exits
the interrupt routine and sets the GIE bit (GIEH or GIEL
if priority levels are used), which re-enables interrupts.
For external interrupt events, such as the INT pins or
the PORTB input change interrupt, the interrupt latency
will be three to four instruction cycles. The exact
latency is the same for one or two-cycle instructions.
Individual interrupt flag bits are set, regardless of the
status of their corresponding enable bit or the GIE bit.
Note: Do not use the MOVFF instruction to modify
any of the interrupt control registers while
any interrupt is enabled. Doing so may
cause erratic microcontroller behavior.
PIC18F2420/2520/4420/4520
DS39631B-page 92 Preliminary © 2007 Microchip Technology Inc.
FIGURE 9-1: PIC18 INTERRUPT LOGIC
TMR0IE
GIEH/GIE
GIEL/PEIE
Wake-up if in
Interrupt to CPU
Vector to Location
0008h
INT2IF
INT2IE
INT2IP
INT1IF
INT1IE
INT1IP
TMR0IF
TMR0IE
TMR0IP
RBIF
RBIE
RBIP
IPEN
TMR0IF
TMR0IP
INT1IF
INT1IE
INT1IP
INT2IF
INT2IE
INT2IP
RBIF
RBIE
RBIP
INT0IF
INT0IE
GIEL/PEIE
Interrupt to CPU
Vector to Location
IPEN
IPEN
0018h
SSPIF
SSPIE
SSPIP
SSPIF
SSPIE
SSPIP
ADIF
ADIE
ADIP
RCIF
RCIE
RCIP
Additional Peripheral Interrupts
ADIF
ADIE
ADIP
High Priority Interrupt Generation
Low Priority Interrupt Generation
RCIF
RCIE
RCIP
Additional Peripheral Interrupts
Idle or Sleep modes
GIEH/GIE
© 2007 Microchip Technology Inc. Preliminary DS39631B-page 93
PIC18F2420/2520/4420/4520
9.1 INTCON Registers
The INTCON registers are readable and writable
registers, which contain various enable, priority and
flag bits.
REGISTER 9-1: INTCON REGISTER
Note: Interrupt flag bits are set when an interrupt
condition occurs, regardless of the state of
its corresponding enable bit or the global
enable bit. User software should ensure
the appropriate interrupt flag bits are clear
prior to enabling an interrupt. This feature
allows for software polling.
R/W-0 R/W-0 R/W-0 R/W-0 R/W-0 R/W-0 R/W-0 R/W-x
GIE/GIEH PEIE/GIEL TMR0IE INT0IE RBIE TMR0IF INT0IF RBIF
bit 7 bit 0
bit 7 GIE/GIEH: Global Interrupt Enable bit
When IPEN = 0:
1 = Enables all unmasked interrupts
0 = Disables all interrupts
When IPEN = 1:
1 = Enables all high priority interrupts
0 = Disables all interrupts
bit 6 PEIE/GIEL: Peripheral Interrupt Enable bit
When IPEN = 0:
1 = Enables all unmasked peripheral interrupts
0 = Disables all peripheral interrupts
When IPEN = 1:
1 = Enables all low priority peripheral interrupts
0 = Disables all low priority peripheral interrupts
bit 5 TMR0IE: TMR0 Overflow Interrupt Enable bit
1 = Enables the TMR0 overflow interrupt
0 = Disables the TMR0 overflow interrupt
bit 4 INT0IE: INT0 External Interrupt Enable bit
1 = Enables the INT0 external interrupt
0 = Disables the INT0 external interrupt
bit 3 RBIE: RB Port Change Interrupt Enable bit
1 = Enables the RB port change interrupt
0 = Disables the RB port change interrupt
bit 2 TMR0IF: TMR0 Overflow Interrupt Flag bit
1 = TMR0 register has overflowed (must be cleared in software)
0 = TMR0 register did not overflow
bit 1 INT0IF: INT0 External Interrupt Flag bit
1 = The INT0 external interrupt occurred (must be cleared in software)
0 = The INT0 external interrupt did not occur
bit 0 RBIF: RB Port Change Interrupt Flag bit
1 = At least one of the RB7:RB4 pins changed state (must be cleared in software)
0 = None of the RB7:RB4 pins have changed state
Note: A mismatch condition will continue to set this bit. Reading PORTB will end the
mismatch condition and allow the bit to be cleared.
Legend:
R = Readable bit W = Writable bit U = Unimplemented bit, read as ‘0’
-n = Value at POR ‘1’ = Bit is set ‘0’ = Bit is cleared x = Bit is unknown
PIC18F2420/2520/4420/4520
DS39631B-page 94 Preliminary © 2007 Microchip Technology Inc.
REGISTER 9-2: INTCON2 REGISTER
R/W-1 R/W-1 R/W-1 R/W-1 U-0 R/W-1 U-0 R/W-1
RBPU INTEDG0 INTEDG1 INTEDG2 — TMR0IP — RBIP
bit 7 bit 0
bit 7 RBPU: PORTB Pull-up Enable bit
1 = All PORTB pull-ups are disabled
0 = PORTB pull-ups are enabled by individual port latch values
bit 6 INTEDG0: External Interrupt 0 Edge Select bit
1 = Interrupt on rising edge
0 = Interrupt on falling edge
bit 5 INTEDG1: External Interrupt 1 Edge Select bit
1 = Interrupt on rising edge
0 = Interrupt on falling edge
bit 4 INTEDG2: External Interrupt 2 Edge Select bit
1 = Interrupt on rising edge
0 = Interrupt on falling edge
bit 3 Unimplemented: Read as ‘0’
bit 2 TMR0IP: TMR0 Overflow Interrupt Priority bit
1 = High priority
0 = Low priority
bit 1 Unimplemented: Read as ‘0’
bit 0 RBIP: RB Port Change Interrupt Priority bit
1 = High priority
0 = Low priority
Legend:
R = Readable bit W = Writable bit U = Unimplemented bit, read as ‘0’
-n = Value at POR ‘1’ = Bit is set ‘0’ = Bit is cleared x = Bit is unknown
Note: Interrupt flag bits are set when an interrupt condition occurs, regardless of the state
of its corresponding enable bit or the global enable bit. User software should ensure
the appropriate interrupt flag bits are clear prior to enabling an interrupt. This feature
allows for software polling.
© 2007 Microchip Technology Inc. Preliminary DS39631B-page 95
PIC18F2420/2520/4420/4520
REGISTER 9-3: INTCON3 REGISTER
R/W-1 R/W-1 U-0 R/W-0 R/W-0 U-0 R/W-0 R/W-0
INT2IP INT1IP — INT2IE INT1IE — INT2IF INT1IF
bit 7 bit 0
bit 7 INT2IP: INT2 External Interrupt Priority bit
1 = High priority
0 = Low priority
bit 6 INT1IP: INT1 External Interrupt Priority bit
1 = High priority
0 = Low priority
bit 5 Unimplemented: Read as ‘0’
bit 4 INT2IE: INT2 External Interrupt Enable bit
1 = Enables the INT2 external interrupt
0 = Disables the INT2 external interrupt
bit 3 INT1IE: INT1 External Interrupt Enable bit
1 = Enables the INT1 external interrupt
0 = Disables the INT1 external interrupt
bit 2 Unimplemented: Read as ‘0’
bit 1 INT2IF: INT2 External Interrupt Flag bit
1 = The INT2 external interrupt occurred (must be cleared in software)
0 = The INT2 external interrupt did not occur
bit 0 INT1IF: INT1 External Interrupt Flag bit
1 = The INT1 external interrupt occurred (must be cleared in software)
0 = The INT1 external interrupt did not occur
Legend:
R = Readable bit W = Writable bit U = Unimplemented bit, read as ‘0’
-n = Value at POR ‘1’ = Bit is set ‘0’ = Bit is cleared x = Bit is unknown
Note: Interrupt flag bits are set when an interrupt condition occurs, regardless of the state
of its corresponding enable bit or the global enable bit. User software should ensure
the appropriate interrupt flag bits are clear prior to enabling an interrupt. This feature
allows for software polling.
PIC18F2420/2520/4420/4520
DS39631B-page 96 Preliminary © 2007 Microchip Technology Inc.
9.2 PIR Registers
The PIR registers contain the individual flag bits for the
peripheral interrupts. Due to the number of peripheral
interrupt sources, there are two Peripheral Interrupt
Request Flag registers (PIR1 and PIR2).
REGISTER 9-4: PIR1: PERIPHERAL INTERRUPT REQUEST (FLAG) REGISTER 1
Note 1: Interrupt flag bits are set when an interrupt
condition occurs, regardless of the state of
its corresponding enable bit or the Global
Interrupt Enable bit, GIE (INTCON<7>).
2: User software should ensure the appropriate
interrupt flag bits are cleared prior to
enabling an interrupt and after servicing
that interrupt.
R/W-0 R/W-0 R-0 R-0 R/W-0 R/W-0 R/W-0 R/W-0
PSPIF(1) ADIF RCIF TXIF SSPIF CCP1IF TMR2IF TMR1IF
bit 7 bit 0
bit 7 PSPIF: Parallel Slave Port Read/Write Interrupt Flag bit(1)
1 = A read or a write operation has taken place (must be cleared in software)
0 = No read or write has occurred
Note 1: This bit is unimplemented on 28-pin devices and will read as ‘0’.
bit 6 ADIF: A/D Converter Interrupt Flag bit
1 = An A/D conversion completed (must be cleared in software)
0 = The A/D conversion is not complete
bit 5 RCIF: EUSART Receive Interrupt Flag bit
1 = The EUSART receive buffer, RCREG, is full (cleared when RCREG is read)
0 = The EUSART receive buffer is empty
bit 4 TXIF: EUSART Transmit Interrupt Flag bit
1 = The EUSART transmit buffer, TXREG, is empty (cleared when TXREG is written)
0 = The EUSART transmit buffer is full
bit 3 SSPIF: Master Synchronous Serial Port Interrupt Flag bit
1 = The transmission/reception is complete (must be cleared in software)
0 = Waiting to transmit/receive
bit 2 CCP1IF: CCP1 Interrupt Flag bit
Capture mode:
1 = A TMR1 register capture occurred (must be cleared in software)
0 = No TMR1 register capture occurred
Compare mode:
1 = A TMR1 register compare match occurred (must be cleared in software)
0 = No TMR1 register compare match occurred
PWM mode:
Unused in this mode.
bit 1 TMR2IF: TMR2 to PR2 Match Interrupt Flag bit
1 = TMR2 to PR2 match occurred (must be cleared in software)
0 = No TMR2 to PR2 match occurred
bit 0 TMR1IF: TMR1 Overflow Interrupt Flag bit
1 = TMR1 register overflowed (must be cleared in software)
0 = TMR1 register did not overflow
Legend:
R = Readable bit W = Writable bit U = Unimplemented bit, read as ‘0’
-n = Value at POR ‘1’ = Bit is set ‘0’ = Bit is cleared x = Bit is unknown
© 2007 Microchip Technology Inc. Preliminary DS39631B-page 97
PIC18F2420/2520/4420/4520
REGISTER 9-5: PIR2: PERIPHERAL INTERRUPT REQUEST (FLAG) REGISTER 2
R/W-0 R/W-0 U-0 R/W-0 R/W-0 R/W-0 R/W-0 R/W-0
OSCFIF CMIF — EEIF BCLIF HLVDIF TMR3IF CCP2IF
bit 7 bit 0
bit 7 OSCFIF: Oscillator Fail Interrupt Flag bit
1 = Device oscillator failed, clock input has changed to INTOSC (must be cleared in software)
0 = Device clock operating
bit 6 CMIF: Comparator Interrupt Flag bit
1 = Comparator input has changed (must be cleared in software)
0 = Comparator input has not changed
bit 5 Unimplemented: Read as ‘0’
bit 4 EEIF: Data EEPROM/Flash Write Operation Interrupt Flag bit
1 = The write operation is complete (must be cleared in software)
0 = The write operation is not complete or has not been started
bit 3 BCLIF: Bus Collision Interrupt Flag bit
1 = A bus collision occurred (must be cleared in software)
0 = No bus collision occurred
bit 2 HLVDIF: High/Low-Voltage Detect Interrupt Flag bit
1 = A high/low-voltage condition occurred (direction determined by
VDIRMAG bit, HLVDCON<7>)
0 = A high/low-voltage condition has not occurred
bit 1 TMR3IF: TMR3 Overflow Interrupt Flag bit
1 = TMR3 register overflowed (must be cleared in software)
0 = TMR3 register did not overflow
bit 0 CCP2IF: CCPx Interrupt Flag bit
Capture mode:
1 = A TMR1 register capture occurred (must be cleared in software)
0 = No TMR1 register capture occurred
Compare mode:
1 = A TMR1 register compare match occurred (must be cleared in software)
0 = No TMR1 register compare match occurred
PWM mode:
Unused in this mode.
Legend:
R = Readable bit W = Writable bit U = Unimplemented bit, read as ‘0’
-n = Value at POR ‘1’ = Bit is set ‘0’ = Bit is cleared x = Bit is unknown
PIC18F2420/2520/4420/4520
DS39631B-page 98 Preliminary © 2007 Microchip Technology Inc.
9.3 PIE Registers
The PIE registers contain the individual enable bits for
the peripheral interrupts. Due to the number of peripheral
interrupt sources, there are two Peripheral Interrupt
Enable registers (PIE1 and PIE2). When IPEN = 0, the
PEIE bit must be set to enable any of these peripheral
interrupts.
REGISTER 9-6: PIE1: PERIPHERAL INTERRUPT ENABLE REGISTER 1
R/W-0 R/W-0 R/W-0 R/W-0 R/W-0 R/W-0 R/W-0 R/W-0
PSPIE(1) ADIE RCIE TXIE SSPIE CCP1IE TMR2IE TMR1IE
bit 7 bit 0
bit 7 PSPIE: Parallel Slave Port Read/Write Interrupt Enable bit(1)
1 = Enables the PSP read/write interrupt
0 = Disables the PSP read/write interrupt
Note 1: This bit is unimplemented on 28-pin devices and will read as ‘0’.
bit 6 ADIE: A/D Converter Interrupt Enable bit
1 = Enables the A/D interrupt
0 = Disables the A/D interrupt
bit 5 RCIE: EUSART Receive Interrupt Enable bit
1 = Enables the EUSART receive interrupt
0 = Disables the EUSART receive interrupt
bit 4 TXIE: EUSART Transmit Interrupt Enable bit
1 = Enables the EUSART transmit interrupt
0 = Disables the EUSART transmit interrupt
bit 3 SSPIE: Master Synchronous Serial Port Interrupt Enable bit
1 = Enables the MSSP interrupt
0 = Disables the MSSP interrupt
bit 2 CCP1IE: CCP1 Interrupt Enable bit
1 = Enables the CCP1 interrupt
0 = Disables the CCP1 interrupt
bit 1 TMR2IE: TMR2 to PR2 Match Interrupt Enable bit
1 = Enables the TMR2 to PR2 match interrupt
0 = Disables the TMR2 to PR2 match interrupt
bit 0 TMR1IE: TMR1 Overflow Interrupt Enable bit
1 = Enables the TMR1 overflow interrupt
0 = Disables the TMR1 overflow interrupt
Legend:
R = Readable bit W = Writable bit U = Unimplemented bit, read as ‘0’
-n = Value at POR ‘1’ = Bit is set ‘0’ = Bit is cleared x = Bit is unknown
© 2007 Microchip Technology Inc. Preliminary DS39631B-page 99
PIC18F2420/2520/4420/4520
REGISTER 9-7: PIE2: PERIPHERAL INTERRUPT ENABLE REGISTER 2
R/W-0 R/W-0 U-0 R/W-0 R/W-0 R/W-0 R/W-0 R/W-0
OSCFIE CMIE — EEIE BCLIE HLVDIE TMR3IE CCP2IE
bit 7 bit 0
bit 7 OSCFIE: Oscillator Fail Interrupt Enable bit
1 = Enabled
0 = Disabled
bit 6 CMIE: Comparator Interrupt Enable bit
1 = Enabled
0 = Disabled
bit 5 Unimplemented: Read as ‘0’
bit 4 EEIE: Data EEPROM/Flash Write Operation Interrupt Enable bit
1 = Enabled
0 = Disabled
bit 3 BCLIE: Bus Collision Interrupt Enable bit
1 = Enabled
0 = Disabled
bit 2 HLVDIE: High/Low-Voltage Detect Interrupt Enable bit
1 = Enabled
0 = Disabled
bit 1 TMR3IE: TMR3 Overflow Interrupt Enable bit
1 = Enabled
0 = Disabled
bit 0 CCP2IE: CCP2 Interrupt Enable bit
1 = Enabled
0 = Disabled
Legend:
R = Readable bit W = Writable bit U = Unimplemented bit, read as ‘0’
-n = Value at POR ‘1’ = Bit is set ‘0’ = Bit is cleared x = Bit is unknown
PIC18F2420/2520/4420/4520
DS39631B-page 100 Preliminary © 2007 Microchip Technology Inc.
9.4 IPR Registers
The IPR registers contain the individual priority bits for
the peripheral interrupts. Due to the number of peripheral
interrupt sources, there are two Peripheral Interrupt
Priority registers (IPR1 and IPR2). Using the priority bits
requires that the Interrupt Priority Enable (IPEN) bit be
set.
REGISTER 9-8: IPR1: PERIPHERAL INTERRUPT PRIORITY REGISTER 1
R/W-1 R/W-1 R/W-1 R/W-1 R/W-1 R/W-1 R/W-1 R/W-1
PSPIP(1) ADIP RCIP TXIP SSPIP CCP1IP TMR2IP TMR1IP
bit 7 bit 0
bit 7 PSPIP: Parallel Slave Port Read/Write Interrupt Priority bit(1)
1 = High priority
0 = Low priority
Note 1: This bit is unimplemented on 28-pin devices and will read as ‘0’.
bit 6 ADIP: A/D Converter Interrupt Priority bit
1 = High priority
0 = Low priority
bit 5 RCIP: EUSART Receive Interrupt Priority bit
1 = High priority
0 = Low priority
bit 4 TXIP: EUSART Transmit Interrupt Priority bit
1 = High priority
0 = Low priority
bit 3 SSPIP: Master Synchronous Serial Port Interrupt Priority bit
1 = High priority
0 = Low priority
bit 2 CCP1IP: CCP1 Interrupt Priority bit
1 = High priority
0 = Low priority
bit 1 TMR2IP: TMR2 to PR2 Match Interrupt Priority bit
1 = High priority
0 = Low priority
bit 0 TMR1IP: TMR1 Overflow Interrupt Priority bit
1 = High priority
0 = Low priority
Legend:
R = Readable bit W = Writable bit U = Unimplemented bit, read as ‘0’
-n = Value at POR ‘1’ = Bit is set ‘0’ = Bit is cleared x = Bit is unknown
© 2007 Microchip Technology Inc. Preliminary DS39631B-page 101
PIC18F2420/2520/4420/4520
REGISTER 9-9: IPR2: PERIPHERAL INTERRUPT PRIORITY REGISTER 2
R/W-1 R/W-1 U-0 R/W-1 R/W-1 R/W-1 R/W-1 R/W-1
OSCFIP CMIP — EEIP BCLIP HLVDIP TMR3IP CCP2IP
bit 7 bit 0
bit 7 OSCFIP: Oscillator Fail Interrupt Priority bit
1 = High priority
0 = Low priority
bit 6 CMIP: Comparator Interrupt Priority bit
1 = High priority
0 = Low priority
bit 5 Unimplemented: Read as ‘0’
bit 4 EEIP: Data EEPROM/Flash Write Operation Interrupt Priority bit
1 = High priority
0 = Low priority
bit 3 BCLIP: Bus Collision Interrupt Priority bit
1 = High priority
0 = Low priority
bit 2 HLVDIP: High/Low-Voltage Detect Interrupt Priority bit
1 = High priority
0 = Low priority
bit 1 TMR3IP: TMR3 Overflow Interrupt Priority bit
1 = High priority
0 = Low priority
bit 0 CCP2IP: CCP2 Interrupt Priority bit
1 = High priority
0 = Low priority
Legend:
R = Readable bit W = Writable bit U = Unimplemented bit, read as ‘0’
-n = Value at POR ‘1’ = Bit is set ‘0’ = Bit is cleared x = Bit is unknown
PIC18F2420/2520/4420/4520
DS39631B-page 102 Preliminary © 2007 Microchip Technology Inc.
9.5 RCON Register
The RCON register contains flag bits which are used to
determine the cause of the last Reset or wake-up from
Idle or Sleep modes. RCON also contains the IPEN bit
which enables interrupt priorities.
The operation of the SBOREN bit and the Reset flag
bits is discussed in more detail in Section 4.1 “RCON
Register”.
REGISTER 9-10: RCON REGISTER
R/W-0 R/W-1(1) U-0 R/W-1 R-1 R-1 R/W-0(1) R/W-0
IPEN SBOREN — RI TO PD POR BOR
bit 7 bit 0
bit 7 IPEN: Interrupt Priority Enable bit
1 = Enable priority levels on interrupts
0 = Disable priority levels on interrupts (PIC16XXX Compatibility mode)
bit 6 SBOREN: Software BOR Enable bit(1)
For details of bit operation, see Register 4-1.
Note 1: Actual Reset values are determined by device configuration and the nature of the
device Reset. See Register 4-1 for additional information.
bit 5 Unimplemented: Read as ‘0’
bit 4 RI: RESET Instruction Flag bit
For details of bit operation, see Register 4-1.
bit 3 TO: Watchdog Time-out Flag bit
For details of bit operation, see Register 4-1.
bit 2 PD: Power-down Detection Flag bit
For details of bit operation, see Register 4-1.
bit 1 POR: Power-on Reset Status bit
For details of bit operation, see Register 4-1.
bit 0 BOR: Brown-out Reset Status bit
For details of bit operation, see Register 4-1.
Legend:
R = Readable bit W = Writable bit U = Unimplemented bit, read as ‘0’
-n = Value at POR ‘1’ = Bit is set ‘0’ = Bit is cleared x = Bit is unknown
© 2007 Microchip Technology Inc. Preliminary DS39631B-page 103
PIC18F2420/2520/4420/4520
9.6 INTn Pin Interrupts
External interrupts on the RB0/INT0, RB1/INT1 and
RB2/INT2 pins are edge-triggered. If the corresponding
INTEDGx bit in the INTCON2 register is set (= 1), the
interrupt is triggered by a rising edge; if the bit is clear,
the trigger is on the falling edge. When a valid edge
appears on the RBx/INTx pin, the corresponding flag
bit, INTxF, is set. This interrupt can be disabled by
clearing the corresponding enable bit, INTxE. Flag bit,
INTxF, must be cleared in software in the Interrupt
Service Routine before re-enabling the interrupt.
All external interrupts (INT0, INT1 and INT2) can wakeup
the processor from Idle or Sleep modes if bit INTxE
was set prior to going into those modes. If the Global
Interrupt Enable bit, GIE, is set, the processor will
branch to the interrupt vector following wake-up.
Interrupt priority for INT1 and INT2 is determined by the
value contained in the interrupt priority bits, INT1IP
(INTCON3<6>) and INT2IP (INTCON3<7>). There is
no priority bit associated with INT0. It is always a high
priority interrupt source.
9.7 TMR0 Interrupt
In 8-bit mode (which is the default), an overflow in the
TMR0 register (FFh → 00h) will set flag bit, TMR0IF. In
16-bit mode, an overflow in the TMR0H:TMR0L register
pair (FFFFh → 0000h) will set TMR0IF. The interrupt
can be enabled/disabled by setting/clearing enable bit,
TMR0IE (INTCON<5>). Interrupt priority for Timer0 is
determined by the value contained in the interrupt priority
bit, TMR0IP (INTCON2<2>). See Section 11.0
“Timer0 Module” for further details on the Timer0
module.
9.8 PORTB Interrupt-on-Change
An input change on PORTB<7:4> sets flag bit, RBIF
(INTCON<0>). The interrupt can be enabled/disabled
by setting/clearing enable bit, RBIE (INTCON<3>).
Interrupt priority for PORTB interrupt-on-change is
determined by the value contained in the interrupt
priority bit, RBIP (INTCON2<0>).
9.9 Context Saving During Interrupts
During interrupts, the return PC address is saved on
the stack. Additionally, the WREG, Status and BSR registers
are saved on the fast return stack. If a fast return
from interrupt is not used (see Section 5.3 “Data
Memory Organization”), the user may need to save
the WREG, Status and BSR registers on entry to the
Interrupt Service Routine. Depending on the user’s
application, other registers may also need to be saved.
Example 9-1 saves and restores the WREG, Status
and BSR registers during an Interrupt Service Routine.
EXAMPLE 9-1: SAVING STATUS, WREG AND BSR REGISTERS IN RAM
MOVWF W_TEMP ; W_TEMP is in virtual bank
MOVFF STATUS, STATUS_TEMP ; STATUS_TEMP located anywhere
MOVFF BSR, BSR_TEMP ; BSR_TMEP located anywhere
;
; USER ISR CODE
;
MOVFF BSR_TEMP, BSR ; Restore BSR
MOVF W_TEMP, W ; Restore WREG
MOVFF STATUS_TEMP, STATUS ; Restore STATUS
PIC18F2420/2520/4420/4520
DS39631B-page 104 Preliminary © 2007 Microchip Technology Inc.
NOTES:
© 2007 Microchip Technology Inc. Preliminary DS39631B-page 105
PIC18F2420/2520/4420/4520
10.0 I/O PORTS
Depending on the device selected and features
enabled, there are up to five ports available. Some pins
of the I/O ports are multiplexed with an alternate
function from the peripheral features on the device. In
general, when a peripheral is enabled, that pin may not
be used as a general purpose I/O pin.
Each port has three registers for its operation. These
registers are:
• TRIS register (data direction register)
• PORT register (reads the levels on the pins of the
device)
• LAT register (output latch)
The Data Latch (LAT register) is useful for read-modifywrite
operations on the value that the I/O pins are
driving.
A simplified model of a generic I/O port, without the
interfaces to other peripherals, is shown in Figure 10-1.
FIGURE 10-1: GENERIC I/O PORT
OPERATION
10.1 PORTA, TRISA and LATA Registers
PORTA is a 8-bit wide, bidirectional port. The corresponding
data direction register is TRISA. Setting a
TRISA bit (= 1) will make the corresponding PORTA pin
an input (i.e., put the corresponding output driver in a
high-impedance mode). Clearing a TRISA bit (= 0) will
make the corresponding PORTA pin an output (i.e., put
the contents of the output latch on the selected pin).
Reading the PORTA register reads the status of the
pins, whereas writing to it, will write to the port latch.
The Data Latch (LATA) register is also memory mapped.
Read-modify-write operations on the LATA register read
and write the latched output value for PORTA.
The RA4 pin is multiplexed with the Timer0 module
clock input and one of the comparator outputs to
become the RA4/T0CKI/C1OUT pin. Pins RA6 and
RA7 are multiplexed with the main oscillator pins; they
are enabled as oscillator or I/O pins by the selection of
the main oscillator in the configuration register (see
Section 23.1 “Configuration Bits” for details). When
they are not used as port pins, RA6 and RA7 and their
associated TRIS and LAT bits are read as ‘0’.
The other PORTA pins are multiplexed with analog
inputs, the analog VREF+ and VREF- inputs and the comparator
voltage reference output. The operation of pins
RA3:RA0 and RA5 as A/D converter inputs is selected
by clearing or setting the control bits in the ADCON1
register (A/D Control Register 1).
Pins RA0 through RA5 may also be used as comparator
inputs or outputs by setting the appropriate bits in the
CMCON register. To use RA3:RA0 as digital inputs, it is
also necessary to turn off the comparators.
The RA4/T0CKI/C1OUT pin is a Schmitt Trigger input.
All other PORTA pins have TTL input levels and full
CMOS output drivers.
The TRISA register controls the direction of the PORTA
pins, even when they are being used as analog inputs.
The user must ensure the bits in the TRISA register are
maintained set when using them as analog inputs.
EXAMPLE 10-1: INITIALIZING PORTA
Data
Bus
WR LAT
WR TRIS
RD Port
Data Latch
TRIS Latch
RD TRIS
Input
Buffer
I/O pin(1)
D Q
CK
D Q
CK
EN
Q D
EN
RD LAT
or Port
Note 1: I/O pins have diode protection to VDD and VSS.
Note: On a Power-on Reset, RA5 and RA3:RA0
are configured as analog inputs and read
as ‘0’. RA4 is configured as a digital input.
CLRF PORTA ; Initialize PORTA by
; clearing output
; data latches
CLRF LATA ; Alternate method
; to clear output
; data latches
MOVLW 07h ; Configure A/D
MOVWF ADCON1 ; for digital inputs
MOVWF 07h ; Configure comparators
MOVWF CMCON ; for digital input
MOVLW 0CFh ; Value used to
; initialize data
; direction
MOVWF TRISA ; Set RA<3:0> as inputs
; RA<5:4> as outputs
PIC18F2420/2520/4420/4520
DS39631B-page 106 Preliminary © 2007 Microchip Technology Inc.
TABLE 10-1: PORTA I/O SUMMARY
Pin Function
TRIS
Setting
I/O
I/O
Type
Description
RA0/AN0 RA0 0 O DIG LATA<0> data output; not affected by analog input.
1 I TTL PORTA<0> data input; disabled when analog input enabled.
AN0 1 I ANA A/D input channel 0 and Comparator C1- input. Default input
configuration on POR; does not affect digital output.
RA1/AN1 RA1 0 O DIG LATA<1> data output; not affected by analog input.
1 I TTL PORTA<1> data input; disabled when analog input enabled.
AN1 1 I ANA A/D input channel 1 and Comparator C2- input. Default input
configuration on POR; does not affect digital output.
RA2/AN2/
VREF-/CVREF
RA2 0 O DIG LATA<2> data output; not affected by analog input. Disabled when
CVREF output enabled.
1 I TTL PORTA<2> data input. Disabled when analog functions enabled;
disabled when CVREF output enabled.
AN2 1 I ANA A/D input channel 2 and Comparator C2+ input. Default input
configuration on POR; not affected by analog output.
VREF- 1 I ANA A/D and comparator voltage reference low input.
CVREF x O ANA Comparator voltage reference output. Enabling this feature disables
digital I/O.
RA3/AN3/VREF+ RA3 0 O DIG LATA<3> data output; not affected by analog input.
1 I TTL PORTA<3> data input; disabled when analog input enabled.
AN3 1 I ANA A/D input channel 3 and Comparator C1+ input. Default input
configuration on POR.
VREF+ 1 I ANA A/D and comparator voltage reference high input.
RA4/T0CKI/C1OUT RA4 0 O DIG LATA<4> data output.
1 I ST PORTA<4> data input; default configuration on POR.
T0CKI 1 I ST Timer0 clock input.
C1OUT 0 O DIG Comparator 1 output; takes priority over port data.
RA5/AN4/SS/
HLVDIN/C2OUT
RA5 0 O DIG LATA<5> data output; not affected by analog input.
1 I TTL PORTA<5> data input; disabled when analog input enabled.
AN4 1 I ANA A/D input channel 4. Default configuration on POR.
SS 1 I TTL Slave select input for SSP (MSSP module).
HLVDIN 1 I ANA High/Low-Voltage Detect external trip point input.
C2OUT 0 O DIG Comparator 2 output; takes priority over port data.
OSC2/CLKO/RA6 RA6 0 O DIG LATA<6> data output. Enabled in RCIO, INTIO2 and ECIO modes only.
1 I TTL PORTA<6> data input. Enabled in RCIO, INTIO2 and ECIO modes
only.
OSC2 x O ANA Main oscillator feedback output connection (XT, HS and LP modes).
CLKO x O DIG System cycle clock output (FOSC/4) in RC, INTIO1 and EC Oscillator
modes.
OSC1/CLKI/RA7 RA7 0 O DIG LATA<7> data output. Disabled in external oscillator modes.
1 I TTL PORTA<7> data input. Disabled in external oscillator modes.
OSC1 x I ANA Main oscillator input connection.
CLKI x I ANA Main clock input connection.
Legend: DIG = Digital level output; TTL = TTL input buffer; ST = Schmitt Trigger input buffer; ANA = Analog level input/output;
x = Don’t care (TRIS bit does not affect port direction or is overridden for this option).
© 2007 Microchip Technology Inc. Preliminary DS39631B-page 107
PIC18F2420/2520/4420/4520
TABLE 10-2: SUMMARY OF REGISTERS ASSOCIATED WITH PORTA
Name Bit 7 Bit 6 Bit 5 Bit 4 Bit 3 Bit 2 Bit 1 Bit 0
Reset
Values
on page
PORTA RA7(1) RA6(1) RA5 RA4 RA3 RA2 RA1 RA0 52
LATA LATA7(1) LATA6(1) PORTA Data Latch Register (Read and Write to Data Latch) 52
TRISA TRISA7(1) TRISA6(1) PORTA Data Direction Control Register 52
ADCON1 — — VCFG1 VCFG0 PCFG3 PCFG2 PCFG1 PCFG0 51
CMCON C2OUT C1OUT C2INV C1INV CIS CM2 CM1 CM0 51
CVRCON CVREN CVROE CVRR CVRSS CVR3 CVR2 CVR1 CVR0 51
Legend: — = unimplemented, read as ‘0’. Shaded cells are not used by PORTA.
Note 1: RA7:RA6 and their associated latch and data direction bits are enabled as I/O pins based on oscillator
configuration; otherwise, they are read as ‘0’.
PIC18F2420/2520/4420/4520
DS39631B-page 108 Preliminary © 2007 Microchip Technology Inc.
10.2 PORTB, TRISB and LATB
Registers
PORTB is an 8-bit wide, bidirectional port. The corresponding
data direction register is TRISB. Setting a
TRISB bit (= 1) will make the corresponding PORTB
pin an input (i.e., put the corresponding output driver in
a high-impedance mode). Clearing a TRISB bit (= 0)
will make the corresponding PORTB pin an output (i.e.,
put the contents of the output latch on the selected pin).
The Data Latch register (LATB) is also memory
mapped. Read-modify-write operations on the LATB
register read and write the latched output value for
PORTB.
EXAMPLE 10-2: INITIALIZING PORTB
Each of the PORTB pins has a weak internal pull-up. A
single control bit can turn on all the pull-ups. This is performed
by clearing bit, RBPU (INTCON2<7>). The
weak pull-up is automatically turned off when the port
pin is configured as an output. The pull-ups are
disabled on a Power-on Reset.
Four of the PORTB pins (RB7:RB4) have an interrupton-
change feature. Only pins configured as inputs can
cause this interrupt to occur (i.e., any RB7:RB4 pin
configured as an output is excluded from the interrupton-
change comparison). The input pins (of RB7:RB4)
are compared with the old value latched on the last
read of PORTB. The “mismatch” outputs of RB7:RB4
are ORed together to generate the RB Port Change
Interrupt with Flag bit, RBIF (INTCON<0>).
This interrupt can wake the device from the Sleep
mode, or any of the Idle modes. The user, in the
Interrupt Service Routine, can clear the interrupt in the
following manner:
a) Any read or write of PORTB (except with the
MOVFF (ANY), PORTB instruction).
b) Clear flag bit, RBIF.
A mismatch condition will continue to set flag bit, RBIF.
Reading PORTB will end the mismatch condition and
allow flag bit, RBIF, to be cleared.
The interrupt-on-change feature is recommended for
wake-up on key depression operation and operations
where PORTB is only used for the interrupt-on-change
feature. Polling of PORTB is not recommended while
using the interrupt-on-change feature.
RB3 can be configured by the configuration bit,
CCP2MX, as the alternate peripheral pin for the CCP2
module (CCP2MX = 0).
Note: On a Power-on Reset, RB4:RB0 are
configured as analog inputs by default and
read as ‘0’; RB7:RB5 are configured as
digital inputs.
By programming the configuration bit,
PBADEN, RB4:RB0 will alternatively be
configured as digital inputs on POR.
CLRF PORTB ; Initialize PORTB by
; clearing output
; data latches
CLRF LATB ; Alternate method
; to clear output
; data latches
MOVLW 0Fh ; Set RB<4:0> as
MOVWF ADCON1 ; digital I/O pins
; (required if config bit
; PBADEN is set)
MOVLW 0CFh ; Value used to
; initialize data
; direction
MOVWF TRISB ; Set RB<3:0> as inputs
; RB<5:4> as outputs
; RB<7:6> as inputs
© 2007 Microchip Technology Inc. Preliminary DS39631B-page 109
PIC18F2420/2520/4420/4520
TABLE 10-3: PORTB I/O SUMMARY
Pin Function
TRIS
Setting
I/O
I/O
Type
Description
RB0/INT0/FLT0/
AN12
RB0 0 O DIG LATB<0> data output; not affected by analog input.
1 I TTL PORTB<0> data input; weak pull-up when RBPU bit is cleared.
Disabled when analog input enabled.(1)
INT0 1 I ST External interrupt 0 input.
FLT0 1 I ST Enhanced PWM Fault input (ECCP1 module); enabled in software.
AN12 1 I ANA A/D input channel 12.(1)
RB1/INT1/AN10 RB1 0 O DIG LATB<1> data output; not affected by analog input.
1 I TTL PORTB<1> data input; weak pull-up when RBPU bit is cleared.
Disabled when analog input enabled.(1)
INT1 1 I ST External Interrupt 1 input.
AN10 1 I ANA A/D input channel 10.(1)
RB2/INT2/AN8 RB2 0 O DIG LATB<2> data output; not affected by analog input.
1 I TTL PORTB<2> data input; weak pull-up when RBPU bit is cleared.
Disabled when analog input enabled.(1)
INT2 1 I ST External interrupt 2 input.
AN8 1 I ANA A/D input channel 8.(1)
RB3/AN9/CCP2 RB3 0 O DIG LATB<3> data output; not affected by analog input.
1 I TTL PORTB<3> data input; weak pull-up when RBPU bit is cleared.
Disabled when analog input enabled.(1)
AN9 1 I ANA A/D input channel 9.(1)
CCP2(2) 0 O DIG CCP2 compare and PWM output.
1 I ST CCP2 capture input
RB4/KBI0/AN11 RB4 0 O DIG LATB<4> data output; not affected by analog input.
1 I TTL PORTB<4> data input; weak pull-up when RBPU bit is cleared.
Disabled when analog input enabled.(1)
KBI0 1 I TTL Interrupt on pin change.
AN11 1 I ANA A/D input channel 11.(1)
RB5/KBI1/PGM RB5 0 O DIG LATB<5> data output.
1 I TTL PORTB<5> data input; weak pull-up when RBPU bit is cleared.
KBI1 1 I TTL Interrupt on pin change.
PGM x I ST Single-Supply Programming mode entry (ICSP™). Enabled by LVP
configuration bit; all other pin functions disabled.
RB6/KBI2/PGC RB6 0 O DIG LATB<6> data output.
1 I TTL PORTB<6> data input; weak pull-up when RBPU bit is cleared.
KBI2 1 I TTL Interrupt on pin change.
PGC x I ST Serial execution (ICSP) clock input for ICSP and ICD operation.(3)
RB7/KBI3/PGD RB7 0 O DIG LATB<7> data output.
1 I TTL PORTB<7> data input; weak pull-up when RBPU bit is cleared.
KBI3 1 I TTL Interrupt on pin change.
PGD x O DIG Serial execution data output for ICSP and ICD operation.(3)
x I ST Serial execution data input for ICSP and ICD operation.(3)
Legend: DIG = Digital level output; TTL = TTL input buffer; ST = Schmitt Trigger input buffer; ANA = Analog level input/output;
x = Don’t care (TRIS bit does not affect port direction or is overridden for this option).
Note 1: Configuration on POR is determined by the PBADEN configuration bit. Pins are configured as analog inputs by default
when PBADEN is set and digital inputs when PBADEN is cleared.
2: Alternate assignment for CCP2 when the CCP2MX configuration bit is ‘0’. Default assignment is RC1.
3: All other pin functions are disabled when ICSP or ICD are enabled.
PIC18F2420/2520/4420/4520
DS39631B-page 110 Preliminary © 2007 Microchip Technology Inc.
TABLE 10-4: SUMMARY OF REGISTERS ASSOCIATED WITH PORTB
Name Bit 7 Bit 6 Bit 5 Bit 4 Bit 3 Bit 2 Bit 1 Bit 0
Reset
Values
on page
PORTB RB7 RB6 RB5 RB4 RB3 RB2 RB1 RB0 52
LATB PORTB Data Latch Register (Read and Write to Data Latch) 52
TRISB PORTB Data Direction Control Register 52
INTCON GIE/GIEH PEIE/GIEL TMR0IE INT0IE RBIE TMR0IF INT0IF RBIF 49
INTCON2 RBPU INTEDG0 INTEDG1 INTEDG2 — TMR0IP — RBIP 49
INTCON3 INT2IP INT1IP — INT2IE INT1IE — INT2IF INT1IF 49
ADCON1 — — VCFG1 VCFG0 PCFG3 PCFG2 PCFG1 PCFG0 51
Legend: — = unimplemented, read as ‘0’. Shaded cells are not used by PORTB.
© 2007 Microchip Technology Inc. Preliminary DS39631B-page 111
PIC18F2420/2520/4420/4520
10.3 PORTC, TRISC and LATC
Registers
PORTC is an 8-bit wide, bidirectional port. The corresponding
data direction register is TRISC. Setting a
TRISC bit (= 1) will make the corresponding PORTC
pin an input (i.e., put the corresponding output driver in
a high-impedance mode). Clearing a TRISC bit (= 0)
will make the corresponding PORTC pin an output (i.e.,
put the contents of the output latch on the selected pin).
The Data Latch register (LATC) is also memory
mapped. Read-modify-write operations on the LATC
register read and write the latched output value for
PORTC.
PORTC is multiplexed with several peripheral functions
(Table 10-5). The pins have Schmitt Trigger input buffers.
RC1 is normally configured by configuration bit,
CCP2MX, as the default peripheral pin of the CCP2
module (default/erased state, CCP2MX = 1).
When enabling peripheral functions, care should be
taken in defining TRIS bits for each PORTC pin. Some
peripherals override the TRIS bit to make a pin an output,
while other peripherals override the TRIS bit to make a
pin an input. The user should refer to the corresponding
peripheral section for additional information.
The contents of the TRISC register are affected by
peripheral overrides. Reading TRISC always returns
the current contents, even though a peripheral device
may be overriding one or more of the pins.
EXAMPLE 10-3: INITIALIZING PORTC
Note: On a Power-on Reset, these pins are
configured as digital inputs.
CLRF PORTC ; Initialize PORTC by
; clearing output
; data latches
CLRF LATC ; Alternate method
; to clear output
; data latches
MOVLW 0CFh ; Value used to
; initialize data
; direction
MOVWF TRISC ; Set RC<3:0> as inputs
; RC<5:4> as outputs
; RC<7:6> as inputs
PIC18F2420/2520/4420/4520
DS39631B-page 112 Preliminary © 2007 Microchip Technology Inc.
TABLE 10-5: PORTC I/O SUMMARY
Pin Function
TRIS
Setting
I/O
I/O
Type
Description
RC0/T1OSO/
T13CKI
RC0 0 O DIG LATC<0> data output.
1 I ST PORTC<0> data input.
T1OSO x O ANA Timer1 oscillator output; enabled when Timer1 oscillator enabled.
Disables digital I/O.
T13CKI 1 I ST Timer1/Timer3 counter input.
RC1/T1OSI/CCP2 RC1 0 O DIG LATC<1> data output.
1 I ST PORTC<1> data input.
T1OSI x I ANA Timer1 oscillator input; enabled when Timer1 oscillator enabled.
Disables digital I/O.
CCP2(1) 0 O DIG CCP2 compare and PWM output; takes priority over port data.
1 I ST CCP2 capture input.
RC2/CCP1/P1A RC2 0 O DIG LATC<2> data output.
1 I ST PORTC<2> data input.
CCP1 0 O DIG ECCP1 compare or PWM output; takes priority over port data.
1 I ST ECCP1 capture input.
P1A(2) 0 O DIG ECCP1 Enhanced PWM output, channel A. May be configured for
tri-state during Enhanced PWM shutdown events. Takes priority over
port data.
RC3/SCK/SCL RC3 0 O DIG LATC<3> data output.
1 I ST PORTC<3> data input.
SCK 0 O DIG SPI™ clock output (MSSP module); takes priority over port data.
1 I ST SPI clock input (MSSP module).
SCL 0 O DIG I2 C™ clock output (MSSP module); takes priority over port data.
1 I I2C/SMB I2C clock input (MSSP module); input type depends on module setting.
RC4/SDI/SDA RC4 0 O DIG LATC<4> data output.
1 I ST PORTC<4> data input.
SDI 1 I ST SPI data input (MSSP module).
SDA 1 O DIG I2 C data output (MSSP module); takes priority over port data.
1 I I2C/SMB I2C data input (MSSP module); input type depends on module setting.
RC5/SDO RC5 0 O DIG LATC<5> data output.
1 I ST PORTC<5> data input.
SDO 0 O DIG SPI data output (MSSP module); takes priority over port data.
RC6/TX/CK RC6 0 O DIG LATC<6> data output.
1 I ST PORTC<6> data input.
TX 1 O DIG Asynchronous serial transmit data output (USART module);
takes priority over port data. User must configure as output.
CK 1 O DIG Synchronous serial clock output (USART module); takes priority
over port data.
1 I ST Synchronous serial clock input (USART module).
RC7/RX/DT RC7 0 O DIG LATC<7> data output.
1 I ST PORTC<7> data input.
RX 1 I ST Asynchronous serial receive data input (USART module).
DT 1 O DIG Synchronous serial data output (USART module); takes priority over
port data.
1 I ST Synchronous serial data input (USART module). User must
configure as an input.
Legend: DIG = Digital level output; TTL = TTL input buffer; ST = Schmitt Trigger input buffer; ANA = Analog level input/output;
I2C/SMB = I2C/SMBus input buffer; x = Don’t care (TRIS bit does not affect port direction or is overridden for this option).
Note 1: Default assignment for CCP2 when the CCP2MX configuration bit is set. Alternate assignment is RB3.
2: Enhanced PWM output is available only on PIC18F4520 devices.
© 2007 Microchip Technology Inc. Preliminary DS39631B-page 113
PIC18F2420/2520/4420/4520
TABLE 10-6: SUMMARY OF REGISTERS ASSOCIATED WITH PORTC
Name Bit 7 Bit 6 Bit 5 Bit 4 Bit 3 Bit 2 Bit 1 Bit 0
Reset
Values
on page
PORTC RC7 RC6 RC5 RC4 RC3 RC2 RC1 RC0 52
LATC PORTC Data Latch Register (Read and Write to Data Latch) 52
TRISC PORTC Data Direction Control Register 52
PIC18F2420/2520/4420/4520
DS39631B-page 114 Preliminary © 2007 Microchip Technology Inc.
10.4 PORTD, TRISD and LATD
Registers
PORTD is an 8-bit wide, bidirectional port. The corresponding
data direction register is TRISD. Setting a
TRISD bit (= 1) will make the corresponding PORTD
pin an input (i.e., put the corresponding output driver in
a high-impedance mode). Clearing a TRISD bit (= 0)
will make the corresponding PORTD pin an output (i.e.,
put the contents of the output latch on the selected pin).
The Data Latch register (LATD) is also memory
mapped. Read-modify-write operations on the LATD
register read and write the latched output value for
PORTD.
All pins on PORTD are implemented with Schmitt Trigger
input buffers. Each pin is individually configurable
as an input or output.
Three of the PORTD pins are multiplexed with outputs
P1B, P1C and P1D of the enhanced CCP module. The
operation of these additional PWM output pins is
covered in greater detail in Section 16.0 “Enhanced
Capture/Compare/PWM (ECCP) Module”.
PORTD can also be configured as an 8-bit wide microprocessor
port (Parallel Slave Port) by setting control
bit, PSPMODE (TRISE<4>). In this mode, the input
buffers are TTL. See Section 10.6 “Parallel Slave
Port” for additional information on the Parallel Slave
Port (PSP).
EXAMPLE 10-4: INITIALIZING PORTD
Note: PORTD is only available on 40/44-pin
devices.
Note: On a Power-on Reset, these pins are
configured as digital inputs.
Note: When the enhanced PWM mode is used
with either dual or quad outputs, the PSP
functions of PORTD are automatically
disabled.
CLRF PORTD ; Initialize PORTD by
; clearing output
; data latches
CLRF LATD ; Alternate method
; to clear output
; data latches
MOVLW 0CFh ; Value used to
; initialize data
; direction
MOVWF TRISD ; Set RD<3:0> as inputs
; RD<5:4> as outputs
; RD<7:6> as inputs
© 2007 Microchip Technology Inc. Preliminary DS39631B-page 115
PIC18F2420/2520/4420/4520
TABLE 10-7: PORTD I/O SUMMARY
Pin Function
TRIS
Setting
I/O
I/O
Type
Description
RD0/PSP0 RD0 0 O DIG LATD<0> data output.
1 I ST PORTD<0> data input.
PSP0 x O DIG PSP read data output (LATD<0>); takes priority over port data.
x I TTL PSP write data input.
RD1/PSP1 RD1 0 O DIG LATD<1> data output.
1 I ST PORTD<1> data input.
PSP1 x O DIG PSP read data output (LATD<1>); takes priority over port data.
x I TTL PSP write data input.
RD2/PSP2 RD2 0 O DIG LATD<2> data output.
1 I ST PORTD<2> data input.
PSP2 x O DIG PSP read data output (LATD<2>); takes priority over port data.
x I TTL PSP write data input.
RD3/PSP3 RD3 0 O DIG LATD<3> data output.
1 I ST PORTD<3> data input.
PSP3 x O DIG PSP read data output (LATD<3>); takes priority over port data.
x I TTL PSP write data input.
RD4/PSP4 RD4 0 O DIG LATD<4> data output.
1 I ST PORTD<4> data input.
PSP4 x O DIG PSP read data output (LATD<4>); takes priority over port data.
x I TTL PSP write data input.
RD5/PSP5/P1B RD5 0 O DIG LATD<5> data output.
1 I ST PORTD<5> data input.
PSP5 x O DIG PSP read data output (LATD<5>); takes priority over port data.
x I TTL PSP write data input.
P1B 0 O DIG ECCP1 Enhanced PWM output, channel B; takes priority over port and
PSP data. May be configured for tri-state during Enhanced PWM
shutdown events.
RD6/PSP6/P1C RD6 0 O DIG LATD<6> data output.
1 I ST PORTD<6> data input.
PSP6 x O DIG PSP read data output (LATD<6>); takes priority over port data.
x I TTL PSP write data input.
P1C 0 O DIG ECCP1 Enhanced PWM output, channel C; takes priority over port and
PSP data. May be configured for tri-state during Enhanced PWM
shutdown events.
RD7/PSP7/P1D RD7 0 O DIG LATD<7> data output.
1 I ST PORTD<7> data input.
PSP7 x O DIG PSP read data output (LATD<7>); takes priority over port data.
x I TTL PSP write data input.
P1D 0 O DIG ECCP1 Enhanced PWM output, channel D; takes priority over port and
PSP data. May be configured for tri-state during Enhanced PWM
shutdown events.
Legend: DIG = Digital level output; TTL = TTL input buffer; ST = Schmitt Trigger input buffer; x = Don’t care
(TRIS bit does not affect port direction or is overridden for this option).
PIC18F2420/2520/4420/4520
DS39631B-page 116 Preliminary © 2007 Microchip Technology Inc.
TABLE 10-8: SUMMARY OF REGISTERS ASSOCIATED WITH PORTD
Name Bit 7 Bit 6 Bit 5 Bit 4 Bit 3 Bit 2 Bit 1 Bit 0
Reset
Values
on page
PORTD RD7 RD6 RD5 RD4 RD3 RD2 RD1 RD0 52
LATD PORTD Data Latch Register (Read and Write to Data Latch) 52
TRISD PORTD Data Direction Control Register 52
TRISE IBF OBF IBOV PSPMODE — TRISE2 TRISE1 TRISE0 52
CCP1CON P1M1 P1M0 DC1B1 DC1B0 CCP1M3 CCP1M2 CCP1M1 CCP1M0 51
Legend: — = unimplemented, read as ‘0’. Shaded cells are not used by PORTD.
© 2007 Microchip Technology Inc. Preliminary DS39631B-page 117
PIC18F2420/2520/4420/4520
10.5 PORTE, TRISE and LATE
Registers
Depending on the particular PIC18F2420/2520/4420/
4520 device selected, PORTE is implemented in two
different ways.
For 40/44-pin devices, PORTE is a 4-bit wide port.
Three pins (RE0/RD/AN5, RE1/WR/AN6 and RE2/CS/
AN7) are individually configurable as inputs or outputs.
These pins have Schmitt Trigger input buffers. When
selected as an analog input, these pins will read as ‘0’s.
The corresponding data direction register is TRISE.
Setting a TRISE bit (= 1) will make the corresponding
PORTE pin an input (i.e., put the corresponding output
driver in a high-impedance mode). Clearing a TRISE bit
(= 0) will make the corresponding PORTE pin an output
(i.e., put the contents of the output latch on the selected
pin).
TRISE controls the direction of the RE pins, even when
they are being used as analog inputs. The user must
make sure to keep the pins configured as inputs when
using them as analog inputs.
The upper four bits of the TRISE register also control
the operation of the Parallel Slave Port. Their operation
is explained in Register 10-1.
The Data Latch register (LATE) is also memory
mapped. Read-modify-write operations on the LATE
register, read and write the latched output value for
PORTE.
The fourth pin of PORTE (MCLR/VPP/RE3) is an input
only pin. Its operation is controlled by the MCLRE configuration
bit. When selected as a port pin (MCLRE = 0),
it functions as a digital input only pin; as such, it does not
have TRIS or LAT bits associated with its operation.
Otherwise, it functions as the device’s Master Clear
input. In either configuration, RE3 also functions as the
programming voltage input during programming.
EXAMPLE 10-5: INITIALIZING PORTE
10.5.1 PORTE IN 28-PIN DEVICES
For 28-pin devices, PORTE is only available when
Master Clear functionality is disabled (MCLRE = 0). In
these cases, PORTE is a single bit, input only port comprised
of RE3 only. The pin operates as previously
described.
Note: On a Power-on Reset, RE2:RE0 are
configured as analog inputs.
Note: On a Power-on Reset, RE3 is enabled as
a digital input only if Master Clear
functionality is disabled.
CLRF PORTE ; Initialize PORTE by
; clearing output
; data latches
CLRF LATE ; Alternate method
; to clear output
; data latches
MOVLW 0Ah ; Configure A/D
MOVWF ADCON1 ; for digital inputs
MOVLW 03h ; Value used to
; initialize data
; direction
MOVWF TRISE ; Set RE<0> as inputs
; RE<1> as outputs
; RE<2> as inputs
PIC18F2420/2520/4420/4520
DS39631B-page 118 Preliminary © 2007 Microchip Technology Inc.
REGISTER 10-1: TRISE REGISTER (40/44-PIN DEVICES ONLY)
R-0 R-0 R/W-0 R/W-0 U-0 R/W-1 R/W-1 R/W-1
IBF OBF IBOV PSPMODE — TRISE2 TRISE1 TRISE0
bit 7 bit 0
bit 7 IBF: Input Buffer Full Status bit
1 = A word has been received and waiting to be read by the CPU
0 = No word has been received
bit 6 OBF: Output Buffer Full Status bit
1 = The output buffer still holds a previously written word
0 = The output buffer has been read
bit 5 IBOV: Input Buffer Overflow Detect bit (in Microprocessor mode)
1 = A write occurred when a previously input word has not been read (must be cleared in software)
0 = No overflow occurred
bit 4 PSPMODE: Parallel Slave Port Mode Select bit
1 = Parallel Slave Port mode
0 = General purpose I/O mode
bit 3 Unimplemented: Read as ‘0’
bit 2 TRISE2: RE2 Direction Control bit
1 = Input
0 = Output
bit 1 TRISE1: RE1 Direction Control bit
1 = Input
0 = Output
bit 0 TRISE0: RE0 Direction Control bit
1 = Input
0 = Output
Legend:
R = Readable bit W = Writable bit U = Unimplemented bit, read as ‘0’
-n = Value at POR ‘1’ = Bit is set ‘0’ = Bit is cleared x = Bit is unknown
© 2007 Microchip Technology Inc. Preliminary DS39631B-page 119
PIC18F2420/2520/4420/4520
TABLE 10-9: PORTE I/O SUMMARY
TABLE 10-10: SUMMARY OF REGISTERS ASSOCIATED WITH PORTE
Pin Function
TRIS
Setting
I/O
I/O
Type
Description
RE0/RD/AN5 RE0 0 O DIG LATE<0> data output; not affected by analog input.
1 I ST PORTE<0> data input; disabled when analog input enabled.
RD 1 I TTL PSP read enable input (PSP enabled).
AN5 1 I ANA A/D input channel 5; default input configuration on POR.
RE1/WR/AN6 RE1 0 O DIG LATE<1> data output; not affected by analog input.
1 I ST PORTE<1> data input; disabled when analog input enabled.
WR 1 I TTL PSP write enable input (PSP enabled).
AN6 1 I ANA A/D input channel 6; default input configuration on POR.
RE2/CS/AN7 RE2 0 O DIG LATE<2> data output; not affected by analog input.
1 I ST PORTE<2> data input; disabled when analog input enabled.
CS 1 I TTL PSP write enable input (PSP enabled).
AN7 1 I ANA A/D input channel 7; default input configuration on POR.
MCLR/VPP/RE3(1) MCLR — I ST External Master Clear input; enabled when MCLRE configuration bit is
set.
VPP — I ANA High-voltage detection; used for ICSP™ mode entry detection. Always
available, regardless of pin mode.
RE3 —(2) I ST PORTE<3> data input; enabled when MCLRE configuration bit is clear.
Legend: DIG = Digital level output; TTL = TTL input buffer; ST = Schmitt Trigger input buffer; ANA = Analog level input/output;
x = Don’t care (TRIS bit does not affect port direction or is overridden for this option).
Note 1: RE3 is available on both 28-pin and 40/44-pin devices. All other PORTE pins are only implemented on 40/44-pin
devices.
2: RE3 does not have a corresponding TRIS bit to control data direction.
Name Bit 7 Bit 6 Bit 5 Bit 4 Bit 3 Bit 2 Bit 1 Bit 0
Reset
Values
on page
PORTE — — — — RE3(1,2) RE2 RE1 RE0 52
LATE(2) — — — — — LATE Data Output Register 52
TRISE IBF OBF IBOV PSPMODE — TRISE2 TRISE1 TRISE0 52
ADCON1 — — VCFG1 VCFG0 PCFG3 PCFG2 PCFG1 PCFG0 51
Legend: — = unimplemented, read as ‘0’. Shaded cells are not used by PORTE.
Note 1: Implemented only when Master Clear functionality is disabled (MCLRE configuration bit = 0).
2: RE3 is the only PORTE bit implemented on both 28-pin and 40/44-pin devices. All other bits are
implemented only when PORTE is implemented (i.e., 40/44-pin devices).
PIC18F2420/2520/4420/4520
DS39631B-page 120 Preliminary © 2007 Microchip Technology Inc.
10.6 Parallel Slave Port
In addition to its function as a general I/O port, PORTD
can also operate as an 8-bit wide Parallel Slave Port
(PSP) or microprocessor port. PSP operation is controlled
by the 4 upper bits of the TRISE register
(Register 10-1). Setting control bit, PSPMODE
(TRISE<4>), enables PSP operation as long as the
enhanced CCP module is not operating in dual output
or quad output PWM mode. In Slave mode, the port is
asynchronously readable and writable by the external
world.
The PSP can directly interface to an 8-bit microprocessor
data bus. The external microprocessor can
read or write the PORTD latch as an 8-bit latch. Setting
the control bit, PSPMODE, enables the PORTE I/O
pins to become control inputs for the microprocessor
port. When set, port pin RE0 is the RD input, RE1 is the
WR input and RE2 is the CS (Chip Select) input. For
this functionality, the corresponding data direction bits
of the TRISE register (TRISE<2:0>) must be configured
as inputs (set). The A/D port configuration bits,
PFCG3:PFCG0 (ADCON1<3:0>), must also be set to a
value in the range of ‘1010’ through ‘1111’.
A write to the PSP occurs when both the CS and WR
lines are first detected low and ends when either are
detected high. The PSPIF and IBF flag bits are both set
when the write ends.
A read from the PSP occurs when both the CS and RD
lines are first detected low. The data in PORTD is read
out and the OBF bit is clear. If the user writes new data
to PORTD to set OBF, the data is immediately read out;
however, the OBF bit is not set.
When either the CS or RD lines are detected high, the
PORTD pins return to the input state and the PSPIF bit
is set. User applications should wait for PSPIF to be set
before servicing the PSP; when this happens, the IBF
and OBF bits can be polled and the appropriate action
taken.
The timing for the control signals in Write and Read
modes is shown in Figure 10-3 and Figure 10-4,
respectively.
FIGURE 10-2: PORTD AND PORTE
BLOCK DIAGRAM
(PARALLEL SLAVE PORT)
Note: The Parallel Slave Port is only available on
40/44-pin devices.
Data Bus
WR LATD
RDx pin
D Q
CK
EN
Q D
RD PORTD EN
One bit of PORTD
Set Interrupt Flag
PSPIF (PIR1<7>)
Read
Chip Select
Write
RD
CS
WR
TTL
TTL
TTL
TTL
or
WR PORTD
RD LATD
Data Latch
Note: I/O pins have diode protection to VDD and VSS.
PORTE Pins
© 2007 Microchip Technology Inc. Preliminary DS39631B-page 121
PIC18F2420/2520/4420/4520
FIGURE 10-3: PARALLEL SLAVE PORT WRITE WAVEFORMS
FIGURE 10-4: PARALLEL SLAVE PORT READ WAVEFORMS
TABLE 10-11: REGISTERS ASSOCIATED WITH PARALLEL SLAVE PORT
Name Bit 7 Bit 6 Bit 5 Bit 4 Bit 3 Bit 2 Bit 1 Bit 0
Reset
Values
on page
PORTD RD7 RD6 RD5 RD4 RD3 RD2 RD1 RD0 52
LATD PORTD Data Latch Register (Read and Write to Data Latch) 52
TRISD PORTD Data Direction Control Register 52
PORTE — — — — RE3 RE2 RE1 RE0 52
LATE — — — — — LATE Data Output bits 52
TRISE IBF OBF IBOV PSPMODE — TRISE2 TRISE1 TRISE0 52
INTCON GIE/GIEH PEIE/GIEL TMR0IF INT0IE RBIE TMR0IF INT0IF RBIF 49
PIR1 PSPIF ADIF RCIF TXIF SSPIF CCP1IF TMR2IF TMR1IF 52
PIE1 PSPIE ADIE RCIE TXIE SSPIE CCP1IE TMR2IE TMR1IE 52
IPR1 PSPIP ADIP RCIP TXIP SSPIP CCP1IP TMR2IP TMR1IP 52
ADCON1 — — VCFG1 VCFG0 PCFG3 PCFG2 PCFG1 PCFG0 51
Legend: — = unimplemented, read as ‘0’. Shaded cells are not used by the Parallel Slave Port.
Q1 Q2 Q3 Q4
CS
Q1 Q2 Q3 Q4 Q1 Q2 Q3 Q4
WR
RD
IBF
OBF
PSPIF
PORTD<7:0>
Q1 Q2 Q3 Q4
CS
Q1 Q2 Q3 Q4 Q1 Q2 Q3 Q4
WR
IBF
PSPIF
RD
OBF
PORTD<7:0>
PIC18F2420/2520/4420/4520
DS39631B-page 122 Preliminary © 2007 Microchip Technology Inc.
NOTES:
© 2007 Microchip Technology Inc. Preliminary DS39631B-page 123
PIC18F2420/2520/4420/4520
11.0 TIMER0 MODULE
The Timer0 module incorporates the following features:
• Software selectable operation as a timer or
counter in both 8-bit or 16-bit modes
• Readable and writable registers
• Dedicated 8-bit, software programmable
prescaler
• Selectable clock source (internal or external)
• Edge select for external clock
• Interrupt-on-overflow
The T0CON register (Register 11-1) controls all
aspects of the module’s operation, including the
prescale selection. It is both readable and writable.
A simplified block diagram of the Timer0 module in 8-bit
mode is shown in Figure 11-1. Figure 11-2 shows a
simplified block diagram of the Timer0 module in 16-bit
mode.
REGISTER 11-1: T0CON: TIMER0 CONTROL REGISTER
R/W-1 R/W-1 R/W-1 R/W-1 R/W-1 R/W-1 R/W-1 R/W-1
TMR0ON T08BIT T0CS T0SE PSA T0PS2 T0PS1 T0PS0
bit 7 bit 0
bit 7 TMR0ON: Timer0 On/Off Control bit
1 = Enables Timer0
0 = Stops Timer0
bit 6 T08BIT: Timer0 8-bit/16-bit Control bit
1 = Timer0 is configured as an 8-bit timer/counter
0 = Timer0 is configured as a 16-bit timer/counter
bit 5 T0CS: Timer0 Clock Source Select bit
1 = Transition on T0CKI pin
0 = Internal instruction cycle clock (CLKO)
bit 4 T0SE: Timer0 Source Edge Select bit
1 = Increment on high-to-low transition on T0CKI pin
0 = Increment on low-to-high transition on T0CKI pin
bit 3 PSA: Timer0 Prescaler Assignment bit
1 = TImer0 prescaler is NOT assigned. Timer0 clock input bypasses prescaler.
0 = Timer0 prescaler is assigned. Timer0 clock input comes from prescaler output.
bit 2-0 T0PS2:T0PS0: Timer0 Prescaler Select bits
111 = 1:256 prescale value
110 = 1:128 prescale value
101 = 1:64 prescale value
100 = 1:32 prescale value
011 = 1:16 prescale value
010 = 1:8 prescale value
001 = 1:4 prescale value
000 = 1:2 prescale value
Legend:
R = Readable bit W = Writable bit U = Unimplemented bit, read as ‘0’
-n = Value at POR ‘1’ = Bit is set ‘0’ = Bit is cleared x = Bit is unknown
PIC18F2420/2520/4420/4520
DS39631B-page 124 Preliminary © 2007 Microchip Technology Inc.
11.1 Timer0 Operation
Timer0 can operate as either a timer or a counter; the
mode is selected with the T0CS bit (T0CON<5>). In
Timer mode (T0CS = 0), the module increments on
every clock by default unless a different prescaler value
is selected (see Section 11.3 “Prescaler”). If the
TMR0 register is written to, the increment is inhibited
for the following two instruction cycles. The user can
work around this by writing an adjusted value to the
TMR0 register.
The Counter mode is selected by setting the T0CS bit
(= 1). In this mode, Timer0 increments either on every
rising or falling edge of pin RA4/T0CKI. The incrementing
edge is determined by the Timer0 Source Edge
Select bit, T0SE (T0CON<4>); clearing this bit selects
the rising edge. Restrictions on the external clock input
are discussed below.
An external clock source can be used to drive Timer0;
however, it must meet certain requirements to ensure
that the external clock can be synchronized with the
internal phase clock (TOSC). There is a delay between
synchronization and the onset of incrementing the
timer/counter.
11.2 Timer0 Reads and Writes in
16-Bit Mode
TMR0H is not the actual high byte of Timer0 in 16-bit
mode; it is actually a buffered version of the real high
byte of Timer0 which is not directly readable nor writable
(refer to Figure 11-2). TMR0H is updated with the
contents of the high byte of Timer0 during a read of
TMR0L. This provides the ability to read all 16 bits of
Timer0 without having to verify that the read of the high
and low byte were valid, due to a rollover between
successive reads of the high and low byte.
Similarly, a write to the high byte of Timer0 must also
take place through the TMR0H Buffer register. The high
byte is updated with the contents of TMR0H when a
write occurs to TMR0L. This allows all 16 bits of Timer0
to be updated at once.
FIGURE 11-1: TIMER0 BLOCK DIAGRAM (8-BIT MODE)
FIGURE 11-2: TIMER0 BLOCK DIAGRAM (16-BIT MODE)
Note: Upon Reset, Timer0 is enabled in 8-bit mode with clock input from T0CKI max. prescale.
T0CKI pin
T0SE
0
1
0
1
T0CS
FOSC/4
Programmable
Prescaler
Sync with
Internal
Clocks
TMR0L
(2 TCY Delay)
PSA Internal Data Bus
T0PS2:T0PS0
Set
TMR0IF
on Overflow
3 8
8
Note: Upon Reset, Timer0 is enabled in 8-bit mode with clock input from T0CKI max. prescale.
T0CKI pin
T0SE
0
1
0
1
T0CS
FOSC/4
Programmable
Prescaler
Sync with
Internal
Clocks
TMR0L
(2 TCY Delay)
Internal Data Bus
8
PSA
T0PS2:T0PS0
Set
TMR0IF
on Overflow
3
TMR0
TMR0H
High Byte
8
8
8
Read TMR0L
Write TMR0L
8
© 2007 Microchip Technology Inc. Preliminary DS39631B-page 125
PIC18F2420/2520/4420/4520
11.3 Prescaler
An 8-bit counter is available as a prescaler for the Timer0
module. The prescaler is not directly readable or writable;
its value is set by the PSA and T0PS2:T0PS0 bits
(T0CON<3:0>) which determine the prescaler
assignment and prescale ratio.
Clearing the PSA bit assigns the prescaler to the
Timer0 module. When it is assigned, prescale values
from 1:2 through 1:256 in power-of-2 increments are
selectable.
When assigned to the Timer0 module, all instructions
writing to the TMR0 register (e.g., CLRF TMR0, MOVWF
TMR0, BSF TMR0, etc.) clear the prescaler count.
11.3.1 SWITCHING PRESCALER
ASSIGNMENT
The prescaler assignment is fully under software
control and can be changed “on-the-fly” during program
execution.
11.4 Timer0 Interrupt
The TMR0 interrupt is generated when the TMR0 register
overflows from FFh to 00h in 8-bit mode, or from
FFFFh to 0000h in 16-bit mode. This overflow sets the
TMR0IF flag bit. The interrupt can be masked by clearing
the TMR0IE bit (INTCON<5>). Before re-enabling
the interrupt, the TMR0IF bit must be cleared in
software by the Interrupt Service Routine.
Since Timer0 is shut down in Sleep mode, the TMR0
interrupt cannot awaken the processor from Sleep.
TABLE 11-1: REGISTERS ASSOCIATED WITH TIMER0
Note: Writing to TMR0 when the prescaler is
assigned to Timer0 will clear the prescaler
count but will not change the prescaler
assignment.
Name Bit 7 Bit 6 Bit 5 Bit 4 Bit 3 Bit 2 Bit 1 Bit 0
Reset
Values
on page
TMR0L Timer0 Register, Low Byte 50
TMR0H Timer0 Register, High Byte 50
INTCON GIE/GIEH PEIE/GIEL TMR0IE INT0IE RBIE TMR0IF INT0IF RBIF 49
T0CON TMR0ON T08BIT T0CS T0SE PSA T0PS2 T0PS1 T0PS0 50
TRISA RA7(1) RA6(1) RA5 RA4 RA3 RA2 RA1 RA0 52
Legend: Shaded cells are not used by Timer0.
Note 1: PORTA<7:6> and their direction bits are individually configured as port pins based on various primary
oscillator modes. When disabled, these bits read as ‘0’.
PIC18F2420/2520/4420/4520
DS39631B-page 126 Preliminary © 2007 Microchip Technology Inc.
NOTES:
© 2007 Microchip Technology Inc. Preliminary DS39631B-page 127
PIC18F2420/2520/4420/4520
12.0 TIMER1 MODULE
The Timer1 timer/counter module incorporates these
features:
• Software selectable operation as a 16-bit timer or
counter
• Readable and writable 8-bit registers (TMR1H
and TMR1L)
• Selectable clock source (internal or external) with
device clock or Timer1 oscillator internal options
• Interrupt-on-overflow
• Reset on CCP Special Event Trigger
• Device clock status flag (T1RUN)
A simplified block diagram of the Timer1 module is
shown in Figure 12-1. A block diagram of the module’s
operation in Read/Write mode is shown in Figure 12-2.
The module incorporates its own low-power oscillator
to provide an additional clocking option. The Timer1
oscillator can also be used as a low-power clock source
for the microcontroller in power managed operation.
Timer1 can also be used to provide Real-Time Clock
(RTC) functionality to applications with only a minimal
addition of external components and code overhead.
Timer1 is controlled through the T1CON Control
register (Register 12-1). It also contains the Timer1
Oscillator Enable bit (T1OSCEN). Timer1 can be
enabled or disabled by setting or clearing control bit,
TMR1ON (T1CON<0>).
REGISTER 12-1: T1CON: TIMER1 CONTROL REGISTER
R/W-0 R-0 R/W-0 R/W-0 R/W-0 R/W-0 R/W-0 R/W-0
RD16 T1RUN T1CKPS1 T1CKPS0 T1OSCEN T1SYNC TMR1CS TMR1ON
bit 7 bit 0
bit 7 RD16: 16-bit Read/Write Mode Enable bit
1 = Enables register read/write of TImer1 in one 16-bit operation
0 = Enables register read/write of Timer1 in two 8-bit operations
bit 6 T1RUN: Timer1 System Clock Status bit
1 = Device clock is derived from Timer1 oscillator
0 = Device clock is derived from another source
bit 5-4 T1CKPS1:T1CKPS0: Timer1 Input Clock Prescale Select bits
11 = 1:8 Prescale value
10 = 1:4 Prescale value
01 = 1:2 Prescale value
00 = 1:1 Prescale value
bit 3 T1OSCEN: Timer1 Oscillator Enable bit
1 = Timer1 oscillator is enabled
0 = Timer1 oscillator is shut off
The oscillator inverter and feedback resistor are turned off to eliminate power drain.
bit 2 T1SYNC: Timer1 External Clock Input Synchronization Select bit
When TMR1CS = 1:
1 = Do not synchronize external clock input
0 = Synchronize external clock input
When TMR1CS = 0:
This bit is ignored. Timer1 uses the internal clock when TMR1CS = 0.
bit 1 TMR1CS: Timer1 Clock Source Select bit
1 = External clock from pin RC0/T1OSO/T13CKI (on the rising edge)
0 = Internal clock (FOSC/4)
bit 0 TMR1ON: Timer1 On bit
1 = Enables Timer1
0 = Stops Timer1
Legend:
R = Readable bit W = Writable bit U = Unimplemented bit, read as ‘0’
-n = Value at POR ‘1’ = Bit is set ‘0’ = Bit is cleared x = Bit is unknown
PIC18F2420/2520/4420/4520
DS39631B-page 128 Preliminary © 2007 Microchip Technology Inc.
12.1 Timer1 Operation
Timer1 can operate in one of these modes:
• Timer
• Synchronous Counter
• Asynchronous Counter
The operating mode is determined by the clock select
bit, TMR1CS (T1CON<1>). When TMR3CS is cleared
(= 0), Timer1 increments on every internal instruction
cycle (Fosc/4). When the bit is set, Timer1 increments
on every rising edge of the Timer1 external clock input
or the Timer1 oscillator, if enabled.
When Timer1 is enabled, the RC1/T1OSI and RC0/
T1OSO/T13CKI pins become inputs. This means the
values of TRISC<1:0> are ignored and the pins are
read as ‘0’.
FIGURE 12-1: TIMER1 BLOCK DIAGRAM
FIGURE 12-2: TIMER1 BLOCK DIAGRAM (16-BIT READ/WRITE MODE)
T1SYNC
TMR1CS
T1CKPS1:T1CKPS0
Sleep Input
T1OSCEN(1)
FOSC/4
Internal
Clock
On/Off
Prescaler
1, 2, 4, 8
Synchronize
Detect
1
0
2
T1OSO/T13CKI
T1OSI
1
0
TMR1ON
TMR1L
Set
TMR1IF
on Overflow
TMR1
Clear TMR1 High Byte
(CCP Special Event Trigger)
Timer1 Oscillator
Note 1: When enable bit, T1OSCEN, is cleared, the inverter and feedback resistor are turned off to eliminate power drain.
On/Off
Timer1
Timer1 Clock Input
T1SYNC
TMR1CS
T1CKPS1:T1CKPS0
Sleep Input
T1OSCEN(1)
FOSC/4
Internal
Clock
Prescaler
1, 2, 4, 8
Synchronize
Detect
1
0
2
T1OSO/T13CKI
T1OSI
Note 1: When enable bit, T1OSCEN, is cleared, the inverter and feedback resistor are turned off to eliminate power drain.
1
0
TMR1L
Internal Data Bus
8
Set
TMR1IF
on Overflow
TMR1
TMR1H
High Byte
8
8
8
Read TMR1L
Write TMR1L
8
TMR1ON
Clear TMR1
(CCP Special Event Trigger)
Timer1 Oscillator
On/Off
Timer1
Timer1 Clock Input
© 2007 Microchip Technology Inc. Preliminary DS39631B-page 129
PIC18F2420/2520/4420/4520
12.2 Timer1 16-Bit Read/Write Mode
Timer1 can be configured for 16-bit reads and writes
(see Figure 12-2). When the RD16 control bit
(T1CON<7>) is set, the address for TMR1H is mapped
to a buffer register for the high byte of Timer1. A read
from TMR1L will load the contents of the high byte of
Timer1 into the Timer1 high byte buffer. This provides
the user with the ability to accurately read all 16 bits of
Timer1 without having to determine whether a read of
the high byte, followed by a read of the low byte, has
become invalid due to a rollover between reads.
A write to the high byte of Timer1 must also take place
through the TMR1H Buffer register. The Timer1 high
byte is updated with the contents of TMR1H when a
write occurs to TMR1L. This allows a user to write all
16 bits to both the high and low bytes of Timer1 at once.
The high byte of Timer1 is not directly readable or
writable in this mode. All reads and writes must take
place through the Timer1 High Byte Buffer register.
Writes to TMR1H do not clear the Timer1 prescaler.
The prescaler is only cleared on writes to TMR1L.
12.3 Timer1 Oscillator
An on-chip crystal oscillator circuit is incorporated
between pins T1OSI (input) and T1OSO (amplifier output).
It is enabled by setting the Timer1 Oscillator Enable
bit, T1OSCEN (T1CON<3>). The oscillator is a lowpower
circuit rated for 32 kHz crystals. It will continue to
run during all power managed modes. The circuit for a
typical LP oscillator is shown in Figure 12-3. Table 12-1
shows the capacitor selection for the Timer1 oscillator.
The user must provide a software time delay to ensure
proper start-up of the Timer1 oscillator.
FIGURE 12-3: EXTERNAL
COMPONENTS FOR THE
TIMER1 LP OSCILLATOR
TABLE 12-1: CAPACITOR SELECTION FOR
THE TIMER OSCILLATOR
12.3.1 USING TIMER1 AS A
CLOCK SOURCE
The Timer1 oscillator is also available as a clock source
in power managed modes. By setting the clock select
bits, SCS1:SCS0 (OSCCON<1:0>), to ‘01’, the device
switches to SEC_RUN mode; both the CPU and
peripherals are clocked from the Timer1 oscillator. If the
IDLEN bit (OSCCON<7>) is cleared and a SLEEP
instruction is executed, the device enters SEC_IDLE
mode. Additional details are available in Section 3.0
“Power Managed Modes”.
Whenever the Timer1 oscillator is providing the clock
source, the Timer1 system clock status flag, T1RUN
(T1CON<6>), is set. This can be used to determine the
controller’s current clocking mode. It can also indicate
the clock source being currently used by the Fail-Safe
Clock Monitor. If the Clock Monitor is enabled and the
Timer1 oscillator fails while providing the clock, polling
the T1RUN bit will indicate whether the clock is being
provided by the Timer1 oscillator or another source.
12.3.2 LOW-POWER TIMER1 OPTION
The Timer1 oscillator can operate at two distinct levels
of power consumption based on device configuration.
When the LPT1OSC configuration bit is set, the Timer1
oscillator operates in a low-power mode. When
LPT1OSC is not set, Timer1 operates at a higher power
level. Power consumption for a particular mode is relatively
constant, regardless of the device’s operating
mode. The default Timer1 configuration is the higher
power mode.
As the low-power Timer1 mode tends to be more sensitive
to interference, high noise environments may
cause some oscillator instability. The low-power option
is, therefore, best suited for low noise applications
where power conservation is an important design
consideration.
Note: See the Notes with Table 12-1 for additional
information about capacitor selection.
C1
C2
XTAL
PIC18FXXXX
T1OSI
T1OSO
32.768 kHz
27 pF
27 pF
Osc Type Freq C1 C2
LP 32 kHz 27 pF(1) 27 pF(1)
Note 1: Microchip suggests these values as a
starting point in validating the oscillator
circuit.
2: Higher capacitance increases the stability
of the oscillator but also increases the
start-up time.
3: Since each resonator/crystal has its own
characteristics, the user should consult
the resonator/crystal manufacturer for
appropriate values of external
components.
4: Capacitor values are for design guidance
only.
PIC18F2420/2520/4420/4520
DS39631B-page 130 Preliminary © 2007 Microchip Technology Inc.
12.3.3 TIMER1 OSCILLATOR LAYOUT
CONSIDERATIONS
The Timer1 oscillator circuit draws very little power
during operation. Due to the low-power nature of the
oscillator, it may also be sensitive to rapidly changing
signals in close proximity.
The oscillator circuit, shown in Figure 12-3, should be
located as close as possible to the microcontroller.
There should be no circuits passing within the oscillator
circuit boundaries other than VSS or VDD.
If a high-speed circuit must be located near the oscillator
(such as the CCP1 pin in Output Compare or PWM
mode, or the primary oscillator using the OSC2 pin), a
grounded guard ring around the oscillator circuit, as
shown in Figure 12-4, may be helpful when used on a
single-sided PCB or in addition to a ground plane.
FIGURE 12-4: OSCILLATOR CIRCUIT
WITH GROUNDED
GUARD RING
12.4 Timer1 Interrupt
The TMR1 register pair (TMR1H:TMR1L) increments
from 0000h to FFFFh and rolls over to 0000h. The
Timer1 interrupt, if enabled, is generated on overflow,
which is latched in interrupt flag bit, TMR1IF
(PIR1<0>). This interrupt can be enabled or disabled
by setting or clearing the Timer1 Interrupt Enable bit,
TMR1IE (PIE1<0>).
12.5 Resetting Timer1 Using the CCP
Special Event Trigger
If either of the CCP modules is configured to use Timer1
and generate a Special Event Trigger in Compare mode
(CCP1M3:CCP1M0 or CCP2M3:CCP2M0 = 1011), this
signal will reset Timer1. The trigger from CCP2 will also
start an A/D conversion if the A/D module is enabled
(see Section 15.3.4 “Special Event Trigger” for more
information).
The module must be configured as either a timer or a
synchronous counter to take advantage of this feature.
When used this way, the CCPRH:CCPRL register pair
effectively becomes a period register for Timer1.
If Timer1 is running in Asynchronous Counter mode,
this Reset operation may not work.
In the event that a write to Timer1 coincides with a
special Event Trigger, the write operation will take
precedence.
12.6 Using Timer1 as a Real-Time Clock
Adding an external LP oscillator to Timer1 (such as the
one described in Section 12.3 “Timer1 Oscillator”
above) gives users the option to include RTC functionality
to their applications. This is accomplished with an
inexpensive watch crystal to provide an accurate time
base and several lines of application code to calculate
the time. When operating in Sleep mode and using a
battery or supercapacitor as a power source, it can
completely eliminate the need for a separate RTC
device and battery backup.
The application code routine, RTCisr, shown in
Example 12-1, demonstrates a simple method to
increment a counter at one-second intervals using an
Interrupt Service Routine. Incrementing the TMR1 register
pair to overflow triggers the interrupt and calls the
routine, which increments the seconds counter by one;
additional counters for minutes and hours are
incremented as the previous counter overflow.
Since the register pair is 16 bits wide, counting up to
overflow the register directly from a 32.768 kHz clock
would take 2 seconds. To force the overflow at the
required one-second intervals, it is necessary to preload
it; the simplest method is to set the MSb of TMR1H
with a BSF instruction. Note that the TMR1L register is
never preloaded or altered; doing so may introduce
cumulative error over many cycles.
For this method to be accurate, Timer1 must operate in
Asynchronous mode and the Timer1 overflow interrupt
must be enabled (PIE1<0> = 1), as shown in the
routine, RTCinit. The Timer1 oscillator must also be
enabled and running at all times.
VDD
OSC1
VSS
OSC2
RC0
RC1
RC2
Note: Not drawn to scale.
Note: The Special Event Triggers from the
CCP2 module will not set the TMR1IF
interrupt flag bit (PIR1<0>).
© 2007 Microchip Technology Inc. Preliminary DS39631B-page 131
PIC18F2420/2520/4420/4520
EXAMPLE 12-1: IMPLEMENTING A REAL-TIME CLOCK USING A TIMER1 INTERRUPT SERVICE
TABLE 12-2: REGISTERS ASSOCIATED WITH TIMER1 AS A TIMER/COUNTER
RTCinit
MOVLW 80h ; Preload TMR1 register pair
MOVWF TMR1H ; for 1 second overflow
CLRF TMR1L
MOVLW b’00001111’ ; Configure for external clock,
MOVWF T1CON ; Asynchronous operation, external oscillator
CLRF secs ; Initialize timekeeping registers
CLRF mins ;
MOVLW .12
MOVWF hours
BSF PIE1, TMR1IE ; Enable Timer1 interrupt
RETURN
RTCisr
BSF TMR1H, 7 ; Preload for 1 sec overflow
BCF PIR1, TMR1IF ; Clear interrupt flag
INCF secs, F ; Increment seconds
MOVLW .59 ; 60 seconds elapsed?
CPFSGT secs
RETURN ; No, done
CLRF secs ; Clear seconds
INCF mins, F ; Increment minutes
MOVLW .59 ; 60 minutes elapsed?
CPFSGT mins
RETURN ; No, done
CLRF mins ; clear minutes
INCF hours, F ; Increment hours
MOVLW .23 ; 24 hours elapsed?
CPFSGT hours
RETURN ; No, done
CLRF hours ; Reset hours
RETURN ; Done
Name Bit 7 Bit 6 Bit 5 Bit 4 Bit 3 Bit 2 Bit 1 Bit 0
Reset
Values
on page
INTCON GIE/GIEH PEIE/GIEL TMR0IE INT0IE RBIE TMR0IF INT0IF RBIF 49
PIR1 PSPIF(1) ADIF RCIF TXIF SSPIF CCP1IF TMR2IF TMR1IF 52
PIE1 PSPIE(1) ADIE RCIE TXIE SSPIE CCP1IE TMR2IE TMR1IE 52
IPR1 PSPIP(1) ADIP RCIP TXIP SSPIP CCP1IP TMR2IP TMR1IP 52
TMR1L Timer1 Register, Low Byte 50
TMR1H Timer1 Register, High Byte 50
T1CON RD16 T1RUN T1CKPS1 T1CKPS0 T1OSCEN T1SYNC TMR1CS TMR1ON 50
Legend: Shaded cells are not used by the Timer1 module.
Note 1: These bits are unimplemented on 28-pin devices; always maintain these bits clear.
PIC18F2420/2520/4420/4520
DS39631B-page 132 Preliminary © 2007 Microchip Technology Inc.
NOTES:
© 2007 Microchip Technology Inc. Preliminary DS39631B-page 133
PIC18F2420/2520/4420/4520
13.0 TIMER2 MODULE
The Timer2 module timer incorporates the following
features:
• 8-bit timer and period registers (TMR2 and PR2,
respectively)
• Readable and writable (both registers)
• Software programmable prescaler (1:1, 1:4 and
1:16)
• Software programmable postscaler (1:1 through
1:16)
• Interrupt on TMR2-to-PR2 match
• Optional use as the shift clock for the MSSP
module
The module is controlled through the T2CON register
(Register 13-1), which enables or disables the timer
and configures the prescaler and postscaler. Timer2
can be shut off by clearing control bit, TMR2ON
(T2CON<2>), to minimize power consumption.
A simplified block diagram of the module is shown in
Figure 13-1.
13.1 Timer2 Operation
In normal operation, TMR2 is incremented from 00h on
each clock (FOSC/4). A 4-bit counter/prescaler on the
clock input gives direct input, divide-by-4 and divide-by-
16 prescale options; these are selected by the prescaler
control bits, T2CKPS1:T2CKPS0 (T2CON<1:0>). The
value of TMR2 is compared to that of the period register,
PR2, on each clock cycle. When the two values match,
the comparator generates a match signal as the timer
output. This signal also resets the value of TMR2 to 00h
on the next cycle and drives the output counter/
postscaler (see Section 13.2 “Timer2 Interrupt”).
The TMR2 and PR2 registers are both directly readable
and writable. The TMR2 register is cleared on any
device Reset, while the PR2 register initializes at FFh.
Both the prescaler and postscaler counters are cleared
on the following events:
• a write to the TMR2 register
• a write to the T2CON register
• any device Reset (Power-on Reset, MCLR Reset,
Watchdog Timer Reset or Brown-out Reset)
TMR2 is not cleared when T2CON is written.
REGISTER 13-1: T2CON: TIMER2 CONTROL REGISTER
U-0 R/W-0 R/W-0 R/W-0 R/W-0 R/W-0 R/W-0 R/W-0
— T2OUTPS3 T2OUTPS2 T2OUTPS1 T2OUTPS0 TMR2ON T2CKPS1 T2CKPS0
bit 7 bit 0
bit 7 Unimplemented: Read as ‘0’
bit 6-3 T2OUTPS3:T2OUTPS0: Timer2 Output Postscale Select bits
0000 = 1:1 Postscale
0001 = 1:2 Postscale
•
•
•
1111 = 1:16 Postscale
bit 2 TMR2ON: Timer2 On bit
1 = Timer2 is on
0 = Timer2 is off
bit 1-0 T2CKPS1:T2CKPS0: Timer2 Clock Prescale Select bits
00 = Prescaler is 1
01 = Prescaler is 4
1x = Prescaler is 16
Legend:
R = Readable bit W = Writable bit U = Unimplemented bit, read as ‘0’
-n = Value at POR ‘1’ = Bit is set ‘0’ = Bit is cleared x = Bit is unknown
PIC18F2420/2520/4420/4520
DS39631B-page 134 Preliminary © 2007 Microchip Technology Inc.
13.2 Timer2 Interrupt
Timer2 also can generate an optional device interrupt.
The Timer2 output signal (TMR2-to-PR2 match) provides
the input for the 4-bit output counter/postscaler.
This counter generates the TMR2 match interrupt flag
which is latched in TMR2IF (PIR1<1>). The interrupt is
enabled by setting the TMR2 Match Interrupt Enable
bit, TMR2IE (PIE1<1>).
A range of 16 postscale options (from 1:1 through 1:16
inclusive) can be selected with the postscaler control
bits, T2OUTPS3:T2OUTPS0 (T2CON<6:3>).
13.3 Timer2 Output
The unscaled output of TMR2 is available primarily to
the CCP modules, where it is used as a time base for
operations in PWM mode.
Timer2 can be optionally used as the shift clock source
for the MSSP module operating in SPI mode. Additional
information is provided in Section 17.0 “Master
Synchronous Serial Port (MSSP) Module”.
FIGURE 13-1: TIMER2 BLOCK DIAGRAM
TABLE 13-1: REGISTERS ASSOCIATED WITH TIMER2 AS A TIMER/COUNTER
Name Bit 7 Bit 6 Bit 5 Bit 4 Bit 3 Bit 2 Bit 1 Bit 0
Reset
Values
on page
INTCON GIE/GIEH PEIE/GIEL TMR0IE INT0IE RBIE TMR0IF INT0IF RBIF 49
PIR1 PSPIF(1) ADIF RCIF TXIF SSPIF CCP1IF TMR2IF TMR1IF 52
PIE1 PSPIE(1) ADIE RCIE TXIE SSPIE CCP1IE TMR2IE TMR1IE 52
IPR1 PSPIP(1) ADIP RCIP TXIP SSPIP CCP1IP TMR2IP TMR1IP 52
TMR2 Timer2 Register 50
T2CON — T2OUTPS3 T2OUTPS2 T2OUTPS1 T2OUTPS0 TMR2ON T2CKPS1 T2CKPS0 50
PR2 Timer2 Period Register 50
Legend: — = unimplemented, read as ‘0’. Shaded cells are not used by the Timer2 module.
Note 1: These bits are unimplemented on 28-pin devices; always maintain these bits clear.
Comparator
TMR2 Output
TMR2
Postscaler
Prescaler
PR2
2
FOSC/4
1:1 to 1:16
1:1, 1:4, 1:16
4
T2OUTPS3:T2OUTPS0
T2CKPS1:T2CKPS0
Set TMR2IF
Internal Data Bus
8
Reset
TMR2/PR2
8 8
(to PWM or MSSP)
Match
© 2007 Microchip Technology Inc. Preliminary DS39631B-page 135
PIC18F2420/2520/4420/4520
14.0 TIMER3 MODULE
The Timer3 module timer/counter incorporates these
features:
• Software selectable operation as a 16-bit timer or
counter
• Readable and writable 8-bit registers (TMR3H
and TMR3L)
• Selectable clock source (internal or external) with
device clock or Timer1 oscillator internal options
• Interrupt-on-overflow
• Module Reset on CCP Special Event Trigger
A simplified block diagram of the Timer3 module is
shown in Figure 14-1. A block diagram of the module’s
operation in Read/Write mode is shown in Figure 14-2.
The Timer3 module is controlled through the T3CON
register (Register 14-1). It also selects the clock source
options for the CCP modules (see Section 15.1.1
“CCP Modules and Timer Resources” for more
information).
REGISTER 14-1: T3CON: TIMER3 CONTROL REGISTER
R/W-0 R/W-0 R/W-0 R/W-0 R/W-0 R/W-0 R/W-0 R/W-0
RD16 T3CCP2 T3CKPS1 T3CKPS0 T3CCP1 T3SYNC TMR3CS TMR3ON
bit 7 bit 0
bit 7 RD16: 16-bit Read/Write Mode Enable bit
1 = Enables register read/write of Timer3 in one 16-bit operation
0 = Enables register read/write of Timer3 in two 8-bit operations
bit 6,3 T3CCP2:T3CCP1: Timer3 and Timer1 to CCPx Enable bits
1x = Timer3 is the capture/compare clock source for the CCP modules
01 = Timer3 is the capture/compare clock source for CCP2;
Timer1 is the capture/compare clock source for CCP1
00 = Timer1 is the capture/compare clock source for the CCP modules
bit 5-4 T3CKPS1:T3CKPS0: Timer3 Input Clock Prescale Select bits
11 = 1:8 Prescale value
10 = 1:4 Prescale value
01 = 1:2 Prescale value
00 = 1:1 Prescale value
bit 2 T3SYNC: Timer3 External Clock Input Synchronization Control bit
(Not usable if the device clock comes from Timer1/Timer3.)
When TMR3CS = 1:
1 = Do not synchronize external clock input
0 = Synchronize external clock input
When TMR3CS = 0:
This bit is ignored. Timer3 uses the internal clock when TMR3CS = 0.
bit 1 TMR3CS: Timer3 Clock Source Select bit
1 = External clock input from Timer1 oscillator or T13CKI (on the rising edge after the first
falling edge)
0 = Internal clock (FOSC/4)
bit 0 TMR3ON: Timer3 On bit
1 = Enables Timer3
0 = Stops Timer3
Legend:
R = Readable bit W = Writable bit U = Unimplemented bit, read as ‘0’
-n = Value at POR ‘1’ = Bit is set ‘0’ = Bit is cleared x = Bit is unknown
PIC18F2420/2520/4420/4520
DS39631B-page 136 Preliminary © 2007 Microchip Technology Inc.
14.1 Timer3 Operation
Timer3 can operate in one of three modes:
• Timer
• Synchronous Counter
• Asynchronous Counter
The operating mode is determined by the clock select
bit, TMR3CS (T3CON<1>). When TMR3CS is cleared
(= 0), Timer3 increments on every internal instruction
cycle (FOSC/4). When the bit is set, Timer3 increments
on every rising edge of the Timer1 external clock input
or the Timer1 oscillator, if enabled.
As with Timer1, the RC1/T1OSI and RC0/T1OSO/
T13CKI pins become inputs when the Timer1 oscillator
is enabled. This means the values of TRISC<1:0> are
ignored and the pins are read as ‘0’.
FIGURE 14-1: TIMER3 BLOCK DIAGRAM
FIGURE 14-2: TIMER3 BLOCK DIAGRAM (16-BIT READ/WRITE MODE)
T3SYNC
TMR3CS
T3CKPS1:T3CKPS0
Sleep Input
T1OSCEN(1)
FOSC/4
Internal
Clock
Prescaler
1, 2, 4, 8
Synchronize
Detect
1
0
2
T1OSO/T13CKI
T1OSI
1
0
TMR3ON
TMR3L
Set
TMR3IF
on Overflow
TMR3
High Byte
Timer1 Oscillator
Note 1: When enable bit, T1OSCEN, is cleared, the inverter and feedback resistor are turned off to eliminate power drain.
On/Off
Timer3
CCP1/CCP2 Special Event Trigger
CCP1/CCP2 Select from T3CON<6,3>
Clear TMR3
Timer1 Clock Input
T3SYNC
TMR3CS
T3CKPS1:T3CKPS0
Sleep Input
T1OSCEN(1)
FOSC/4
Internal
Clock
Prescaler
1, 2, 4, 8
Synchronize
Detect
1
0
2
T13CKI/T1OSO
T1OSI
Note 1: When enable bit, T1OSCEN, is cleared, the inverter and feedback resistor are turned off to eliminate power drain.
1
0
TMR3L
Internal Data Bus
8
Set
TMR3IF
on Overflow
TMR3
TMR3H
High Byte
8
8
8
Read TMR1L
Write TMR1L
8
TMR3ON
CCP1/CCP2 Special Event Trigger
Timer1 Oscillator
On/Off
Timer3
Timer1 Clock Input
CCP1/CCP2 Select from T3CON<6,3>
Clear TMR3
© 2007 Microchip Technology Inc. Preliminary DS39631B-page 137
PIC18F2420/2520/4420/4520
14.2 Timer3 16-Bit Read/Write Mode
Timer3 can be configured for 16-bit reads and writes
(see Figure 14-2). When the RD16 control bit
(T3CON<7>) is set, the address for TMR3H is mapped
to a buffer register for the high byte of Timer3. A read
from TMR3L will load the contents of the high byte of
Timer3 into the Timer3 High Byte Buffer register. This
provides the user with the ability to accurately read all
16 bits of Timer1 without having to determine whether
a read of the high byte, followed by a read of the low
byte, has become invalid due to a rollover between
reads.
A write to the high byte of Timer3 must also take place
through the TMR3H Buffer register. The Timer3 high
byte is updated with the contents of TMR3H when a
write occurs to TMR3L. This allows a user to write all
16 bits to both the high and low bytes of Timer3 at once.
The high byte of Timer3 is not directly readable or
writable in this mode. All reads and writes must take
place through the Timer3 High Byte Buffer register.
Writes to TMR3H do not clear the Timer3 prescaler.
The prescaler is only cleared on writes to TMR3L.
14.3 Using the Timer1 Oscillator as the
Timer3 Clock Source
The Timer1 internal oscillator may be used as the clock
source for Timer3. The Timer1 oscillator is enabled by
setting the T1OSCEN (T1CON<3>) bit. To use it as the
Timer3 clock source, the TMR3CS bit must also be set.
As previously noted, this also configures Timer3 to
increment on every rising edge of the oscillator source.
The Timer1 oscillator is described in Section 12.0
“Timer1 Module”.
14.4 Timer3 Interrupt
The TMR3 register pair (TMR3H:TMR3L) increments
from 0000h to FFFFh and overflows to 0000h. The
Timer3 interrupt, if enabled, is generated on overflow
and is latched in interrupt flag bit, TMR3IF (PIR2<1>).
This interrupt can be enabled or disabled by setting or
clearing the Timer3 Interrupt Enable bit, TMR3IE
(PIE2<1>).
14.5 Resetting Timer3 Using the CCP
Special Event Trigger
If either of the CCP modules is configured to use
Timer3 and to generate a Special Event Trigger
in Compare mode (CCP1M3:CCP1M0 or
CCP2M3:CCP2M0 = 1011), this signal will reset
Timer3. It will also start an A/D conversion if the A/D
module is enabled (see Section 15.3.4 “Special
Event Trigger” for more information).
The module must be configured as either a timer or
synchronous counter to take advantage of this feature.
When used this way, the CCPR2H:CCPR2L register
pair effectively becomes a period register for Timer3.
If Timer3 is running in Asynchronous Counter mode,
the Reset operation may not work.
In the event that a write to Timer3 coincides with a
Special Event Trigger from a CCP module, the write will
take precedence.
TABLE 14-1: REGISTERS ASSOCIATED WITH TIMER3 AS A TIMER/COUNTER
Note: The Special Event Triggers from the
CCP2 module will not set the TMR3IF
interrupt flag bit (PIR1<0>).
Name Bit 7 Bit 6 Bit 5 Bit 4 Bit 3 Bit 2 Bit 1 Bit 0
Reset
Values
on page
INTCON GIE/GIEH PEIE/GIEL TMR0IE INT0IE RBIE TMR0IF INT0IF RBIF 49
PIR2 OSCFIF CMIF — EEIF BCLIF HLVDIF TMR3IF CCP2IF 52
PIE2 OSCFIE CMIE — EEIE BCLIE HLVDIE TMR3IE CCP2IE 52
IPR2 OSCFIP CMIP — EEIP BCLIP HLVDIP TMR3IP CCP2IP 52
TMR3L Timer3 Register, Low Byte 51
TMR3H Timer3 Register, High Byte 51
T1CON RD16 T1RUN T1CKPS1 T1CKPS0 T1OSCEN T1SYNC TMR1CS TMR1ON 50
T3CON RD16 T3CCP2 T3CKPS1 T3CKPS0 T3CCP1 T3SYNC TMR3CS TMR3ON 51
Legend: — = unimplemented, read as ‘0’. Shaded cells are not used by the Timer3 module.
PIC18F2420/2520/4420/4520
DS39631B-page 138 Preliminary © 2007 Microchip Technology Inc.
NOTES:
© 2007 Microchip Technology Inc. Preliminary DS39631B-page 139
PIC18F2420/2520/4420/4520
15.0 CAPTURE/COMPARE/PWM
(CCP) MODULES
PIC18F2420/2520/4420/4520 devices all have two
CCP (Capture/Compare/PWM) modules. Each module
contains a 16-bit register which can operate as a 16-bit
Capture register, a 16-bit Compare register or a PWM
Master/Slave Duty Cycle register.
In 28-pin devices, the two standard CCP modules (CCP1
and CCP2) operate as described in this chapter. In 40/
44-pin devices, CCP1 is implemented as an enhanced
CCP module with standard Capture and Compare
modes and enhanced PWM modes. The ECCP implementation
is discussed in Section 16.0 “Enhanced
Capture/Compare/PWM (ECCP) Module”.
The Capture and Compare operations described in this
chapter apply to all standard and enhanced CCP
modules.
REGISTER 15-1: CCPXCON REGISTER (CCP2 MODULE, CCP1 MODULE IN 28-PIN DEVICES)
Note: Throughout this section and Section 16.0
“Enhanced Capture/Compare/PWM (ECCP)
Module”, references to the register and bit
names for CCP modules are referred to generically
by the use of ‘x’ or ‘y’ in place of the
specific module number. Thus, “CCPxCON”
might refer to the control register for CCP1,
CCP2 or ECCP1. “CCPxCON” is used
throughout these sections to refer to the module
control register, regardless of whether the
CCP module is a standard or enhanced
implementation.
U-0 U-0 R/W-0 R/W-0 R/W-0 R/W-0 R/W-0 R/W-0
— — DCxB1 DCxB0 CCPxM3 CCPxM2 CCPxM1 CCPxM0
bit 7 bit 0
bit 7-6 Unimplemented: Read as ‘0’
bit 5-4 DCxB1:DCxB0: PWM Duty Cycle bit 1 and bit 0 for CCP Module x
Capture mode:
Unused.
Compare mode:
Unused.
PWM mode:
These bits are the two LSbs (bit 1 and bit 0) of the 10-bit PWM duty cycle. The eight MSbs
(DCx9:DCx2) of the duty cycle are found in CCPRxL.
bit 3-0 CCPxM3:CCPxM0: CCP Module x Mode Select bits
0000 = Capture/Compare/PWM disabled (resets CCP module)
0001 = Reserved
0010 = Compare mode, toggle output on match (CCPxIF bit is set)
0011 = Reserved
0100 = Capture mode, every falling edge
0101 = Capture mode, every rising edge
0110 = Capture mode, every 4th rising edge
0111 = Capture mode, every 16th rising edge
1000 = Compare mode: initialize CCP pin low; on compare match, force CCP pin high
(CCPIF bit is set)
1001 = Compare mode: initialize CCP pin high; on compare match, force CCP pin low
(CCPIF bit is set)
1010 = Compare mode: generate software interrupt on compare match (CCPxIF bit is set,
CCP pin reflects I/O state)
1011 = Compare mode: trigger special event, reset timer, start A/D conversion on
CCP2 match (CCPxIF bit is set)
11xx = PWM mode
Legend:
R = Readable bit W = Writable bit U = Unimplemented bit, read as ‘0’
-n = Value at POR ‘1’ = Bit is set ‘0’ = Bit is cleared x = Bit is unknown
PIC18F2420/2520/4420/4520
DS39631B-page 140 Preliminary © 2007 Microchip Technology Inc.
15.1 CCP Module Configuration
Each Capture/Compare/PWM module is associated
with a control register (generically, CCPxCON) and a
data register (CCPRx). The data register, in turn, is
comprised of two 8-bit registers: CCPRxL (low byte)
and CCPRxH (high byte). All registers are both
readable and writable.
15.1.1 CCP MODULES AND TIMER
RESOURCES
The CCP modules utilize Timers 1, 2 or 3, depending
on the mode selected. Timer1 and Timer3 are available
to modules in Capture or Compare modes, while
Timer2 is available for modules in PWM mode.
TABLE 15-1: CCP MODE – TIMER
RESOURCE
The assignment of a particular timer to a module is
determined by the Timer-to-CCP enable bits in the
T3CON register (Register 14-1). Both modules may be
active at any given time and may share the same timer
resource if they are configured to operate in the same
mode (Capture/Compare or PWM) at the same time. The
interactions between the two modules are summarized in
Figure 15-1 and Figure 15-2. In Timer1 in Asynchronous
Counter mode, the capture operation will not work.
15.1.2 CCP2 PIN ASSIGNMENT
The pin assignment for CCP2 (Capture input, Compare
and PWM output) can change, based on device configuration.
The CCP2MX configuration bit determines
which pin CCP2 is multiplexed to. By default, it is
assigned to RC1 (CCP2MX = 1). If the configuration bit
is cleared, CCP2 is multiplexed with RB3.
Changing the pin assignment of CCP2 does not automatically
change any requirements for configuring the
port pin. Users must always verify that the appropriate
TRIS register is configured correctly for CCP2
operation, regardless of where it is located.
TABLE 15-2: INTERACTIONS BETWEEN CCP1 AND CCP2 FOR TIMER RESOURCES
CCP/ECCP Mode Timer Resource
Capture
Compare
PWM
Timer1 or Timer3
Timer1 or Timer3
Timer2
CCP1 Mode CCP2 Mode Interaction
Capture Capture Each module can use TMR1 or TMR3 as the time base. The time base can be different
for each CCP.
Capture Compare CCP2 can be configured for the Special Event Trigger to reset TMR1 or TMR3
(depending upon which time base is used). Automatic A/D conversions on trigger event
can also be done. Operation of CCP1 could be affected if it is using the same timer as a
time base.
Compare Capture CCP1 can be configured for the Special Event Trigger to reset TMR1 or TMR3
(depending upon which time base is used). Operation of CCP2 could be affected if it is
using the same timer as a time base.
Compare Compare Either module can be configured for the Special Event Trigger to reset the time base.
Automatic A/D conversions on CCP2 trigger event can be done. Conflicts may occur if
both modules are using the same time base.
Capture PWM(1) None
Compare PWM(1) None
PWM(1) Capture None
PWM(1) Compare None
PWM(1) PWM Both PWMs will have the same frequency and update rate (TMR2 interrupt).
Note 1: Includes standard and enhanced PWM operation.
© 2007 Microchip Technology Inc. Preliminary DS39631B-page 141
PIC18F2420/2520/4420/4520
15.2 Capture Mode
In Capture mode, the CCPRxH:CCPRxL register pair
captures the 16-bit value of the TMR1 or TMR3
registers when an event occurs on the corresponding
CCPx pin. An event is defined as one of the following:
• every falling edge
• every rising edge
• every 4th rising edge
• every 16th rising edge
The event is selected by the mode select bits,
CCPxM3:CCPxM0 (CCPxCON<3:0>). When a capture
is made, the interrupt request flag bit, CCPxIF, is set; it
must be cleared in software. If another capture occurs
before the value in register CCPRx is read, the old
captured value is overwritten by the new captured value.
15.2.1 CCP PIN CONFIGURATION
In Capture mode, the appropriate CCPx pin should be
configured as an input by setting the corresponding
TRIS direction bit.
15.2.2 TIMER1/TIMER3 MODE SELECTION
The timers that are to be used with the capture feature
(Timer1 and/or Timer3) must be running in Timer mode or
Synchronized Counter mode. In Asynchronous Counter
mode, the capture operation will not work. The timer to be
used with each CCP module is selected in the T3CON
register (see Section 15.1.1 “CCP Modules and Timer
Resources”).
15.2.3 SOFTWARE INTERRUPT
When the Capture mode is changed, a false capture
interrupt may be generated. The user should keep the
CCPxIE interrupt enable bit clear to avoid false interrupts.
The interrupt flag bit, CCPxIF, should also be
cleared following any such change in operating mode.
15.2.4 CCP PRESCALER
There are four prescaler settings in Capture mode; they
are specified as part of the operating mode selected by
the mode select bits (CCPxM3:CCPxM0). Whenever
the CCP module is turned off or Capture mode is
disabled, the prescaler counter is cleared. This means
that any Reset will clear the prescaler counter.
Switching from one capture prescaler to another may
generate an interrupt. Also, the prescaler counter will
not be cleared; therefore, the first capture may be from
a non-zero prescaler. Example 15-1 shows the
recommended method for switching between capture
prescalers. This example also clears the prescaler
counter and will not generate the “false” interrupt.
EXAMPLE 15-1: CHANGING BETWEEN
CAPTURE PRESCALERS
(CCP2 SHOWN)
FIGURE 15-1: CAPTURE MODE OPERATION BLOCK DIAGRAM
Note: If RB3/CCP2 or RC1/CCP2 is configured
as an output, a write to the port can cause
a capture condition.
CLRF CCP2CON ; Turn CCP module off
MOVLW NEW_CAPT_PS ; Load WREG with the
; new prescaler mode
; value and CCP ON
MOVWF CCP2CON ; Load CCP2CON with
; this value
CCPR1H CCPR1L
TMR1H TMR1L
Set CCP1IF
TMR3
Enable
Q1:Q4
CCP1CON<3:0>
CCP1 pin
Prescaler
÷ 1, 4, 16
and
Edge Detect
TMR1
Enable
T3CCP2
T3CCP2
CCPR2H CCPR2L
TMR1H TMR1L
Set CCP2IF
TMR3
Enable
CCP2CON<3:0>
CCP2 pin
Prescaler
÷ 1, 4, 16
TMR3H TMR3L
TMR1
Enable
T3CCP2
T3CCP1
T3CCP2
T3CCP1
TMR3H TMR3L
and
Edge Detect
4
4
4
PIC18F2420/2520/4420/4520
DS39631B-page 142 Preliminary © 2007 Microchip Technology Inc.
15.3 Compare Mode
In Compare mode, the 16-bit CCPRx register value is
constantly compared against either the TMR1 or TMR3
register pair value. When a match occurs, the CCPx pin
can be:
• driven high
• driven low
• toggled (high-to-low or low-to-high)
• remain unchanged (that is, reflects the state of the
I/O latch)
The action on the pin is based on the value of the mode
select bits (CCPxM3:CCPxM0). At the same time, the
interrupt flag bit, CCPxIF, is set.
15.3.1 CCP PIN CONFIGURATION
The user must configure the CCPx pin as an output by
clearing the appropriate TRIS bit.
15.3.2 TIMER1/TIMER3 MODE SELECTION
Timer1 and/or Timer3 must be running in Timer mode
or Synchronized Counter mode if the CCP module is
using the compare feature. In Asynchronous Counter
mode, the compare operation may not work.
15.3.3 SOFTWARE INTERRUPT MODE
When the Generate Software Interrupt mode is chosen
(CCPxM3:CCPxM0 = 1010), the corresponding CCPx
pin is not affected. Only a CCP interrupt is generated,
if enabled and the CCPxIE bit is set.
15.3.4 SPECIAL EVENT TRIGGER
Both CCP modules are equipped with a Special Event
Trigger. This is an internal hardware signal generated
in Compare mode to trigger actions by other modules.
The Special Event Trigger is enabled by selecting
the Compare Special Event Trigger mode
(CCPxM3:CCPxM0 = 1011).
For either CCP module, the Special Event Trigger resets
the timer register pair for whichever timer resource is
currently assigned as the module’s time base. This
allows the CCPRx registers to serve as a programmable
period register for either timer.
The Special Event Trigger for CCP2 can also start an
A/D conversion. In order to do this, the A/D converter
must already be enabled.
FIGURE 15-2: COMPARE MODE OPERATION BLOCK DIAGRAM
Note: Clearing the CCP2CON register will force
the RB3 or RC1 compare output latch
(depending on device configuration) to the
default low level. This is not the PORTB or
PORTC I/O data latch.
CCPR1H CCPR1L
TMR1H TMR1L
Comparator
S Q
R
Output
Logic
Special Event Trigger
Set CCP1IF
CCP1 pin
TRIS
CCP1CON<3:0>
Output Enable
TMR3H TMR3L
CCPR2H CCPR2L
Comparator
1
0
T3CCP2
T3CCP1
Set CCP2IF
1
0
Compare
4
(Timer1/Timer3 Reset)
S Q
R
Output
Logic
Special Event Trigger
CCP2 pin
TRIS
CCP2CON<3:0>
4 Output Enable
(Timer1/Timer3 Reset, A/D Trigger)
Match
Compare
Match
© 2007 Microchip Technology Inc. Preliminary DS39631B-page 143
PIC18F2420/2520/4420/4520
TABLE 15-3: REGISTERS ASSOCIATED WITH CAPTURE, COMPARE, TIMER1 AND TIMER3
Name Bit 7 Bit 6 Bit 5 Bit 4 Bit 3 Bit 2 Bit 1 Bit 0
Reset
Values
on page
INTCON GIE/GIEH PEIE/GIEL TMR0IE INT0IE RBIE TMR0IF INT0IF RBIF 49
RCON IPEN SBOREN — RI TO PD POR BOR 48
PIR1 PSPIF(1) ADIF RCIF TXIF SSPIF CCP1IF TMR2IF TMR1IF 52
PIE1 PSPIE(1) ADIE RCIE TXIE SSPIE CCP1IE TMR2IE TMR1IE 52
IPR1 PSPIP(1) ADIP RCIP TXIP SSPIP CCP1IP TMR2IP TMR1IP 52
PIR2 OSCFIF CMIF — EEIF BCLIF HLVDIF TMR3IF CCP2IF 52
PIE2 OSCFIE CMIE — EEIE BCLIE HLVDIE TMR3IE CCP2IE 52
IPR2 OSCFIP CMIP — EEIP BCLIP HLVDIP TMR3IP CCP2IP 52
TRISB PORTB Data Direction Control Register 52
TRISC PORTC Data Direction Control Register 52
TMR1L Timer1 Register, Low Byte 50
TMR1H Timer1 Register, High Byte 50
T1CON RD16 T1RUN T1CKPS1 T1CKPS0 T1OSCEN T1SYNC TMR1CS TMR1ON 50
TMR3H Timer3 Register, High Byte 51
TMR3L Timer3 Register, Low Byte 51
T3CON RD16 T3CCP2 T3CKPS1 T3CKPS0 T3CCP1 T3SYNC TMR3CS TMR3ON 51
CCPR1L Capture/Compare/PWM Register 1, Low Byte 51
CCPR1H Capture/Compare/PWM Register 1, High Byte 51
CCP1CON P1M1(1) P1M0(1) DC1B1 DC1B0 CCP1M3 CCP1M2 CCP1M1 CCP1M0 51
CCPR2L Capture/Compare/PWM Register 2, Low Byte 51
CCPR2H Capture/Compare/PWM Register 2, High Byte 51
CCP2CON — — DC2B1 DC2B0 CCP2M3 CCP2M2 CCP2M1 CCP2M0 51
Legend: — = unimplemented, read as ‘0’. Shaded cells are not used by Capture/Compare, Timer1 or Timer3.
Note 1: These bits are unimplemented on 28-pin devices; always maintain these bits clear.
PIC18F2420/2520/4420/4520
DS39631B-page 144 Preliminary © 2007 Microchip Technology Inc.
15.4 PWM Mode
In Pulse Width Modulation (PWM) mode, the CCPx pin
produces up to a 10-bit resolution PWM output. Since
the CCP2 pin is multiplexed with a PORTB or PORTC
data latch, the appropriate TRIS bit must be cleared to
make the CCP2 pin an output.
Figure 15-3 shows a simplified block diagram of the
CCP module in PWM mode.
For a step-by-step procedure on how to set up the CCP
module for PWM operation, see Section 15.4.4
“Setup for PWM Operation”.
FIGURE 15-3: SIMPLIFIED PWM BLOCK
DIAGRAM
A PWM output (Figure 15-4) has a time base (period)
and a time that the output stays high (duty cycle).
The frequency of the PWM is the inverse of the
period (1/period).
FIGURE 15-4: PWM OUTPUT
15.4.1 PWM PERIOD
The PWM period is specified by writing to the PR2
register. The PWM period can be calculated using the
following formula:
EQUATION 15-1:
PWM frequency is defined as 1/[PWM period].
When TMR2 is equal to PR2, the following three events
occur on the next increment cycle:
• TMR2 is cleared
• The CCPx pin is set (exception: if PWM duty
cycle = 0%, the CCPx pin will not be set)
• The PWM duty cycle is latched from CCPRxL into
CCPRxH
15.4.2 PWM DUTY CYCLE
The PWM duty cycle is specified by writing to the
CCPRxL register and to the CCPxCON<5:4> bits. Up
to 10-bit resolution is available. The CCPRxL contains
the eight MSbs and the CCPxCON<5:4> contains the
two LSbs. This 10-bit value is represented by
CCPRxL:CCPxCON<5:4>. The following equation is
used to calculate the PWM duty cycle in time:
EQUATION 15-2:
CCPRxL and CCPxCON<5:4> can be written to at any
time, but the duty cycle value is not latched into
CCPRxH until after a match between PR2 and TMR2
occurs (i.e., the period is complete). In PWM mode,
CCPRxH is a read-only register.
Note: Clearing the CCP2CON register will force
the RB3 or RC1 output latch (depending on
device configuration) to the default low
level. This is not the PORTB or PORTC I/O
data latch.
CCPRxL
CCPRxH (Slave)
Comparator
TMR2
Comparator
PR2
(Note 1)
R Q
S
Duty Cycle Registers CCPxCON<5:4>
Clear Timer,
CCP1 pin and
latch D.C.
Note 1: The 8-bit TMR2 value is concatenated with the 2-bit
internal Q clock, or 2 bits of the prescaler, to create the
10-bit time base.
CCPx Output
Corresponding
TRIS bit
Period
Duty Cycle
TMR2 = PR2
TMR2 = Duty Cycle
TMR2 = PR2
Note: The Timer2 postscalers (see Section 13.0
“Timer2 Module”) are not used in the
determination of the PWM frequency. The
postscaler could be used to have a servo
update rate at a different frequency than
the PWM output.
PWM Period = [(PR2) + 1] • 4 • TOSC •
(TMR2 Prescale Value)
PWM Duty Cycle = (CCPRXL:CCPXCON<5:4>) •
TOSC • (TMR2 Prescale Value)
© 2007 Microchip Technology Inc. Preliminary DS39631B-page 145
PIC18F2420/2520/4420/4520
The CCPRxH register and a 2-bit internal latch are
used to double-buffer the PWM duty cycle. This
double-buffering is essential for glitchless PWM
operation.
When the CCPRxH and 2-bit latch match TMR2,
concatenated with an internal 2-bit Q clock or 2 bits of
the TMR2 prescaler, the CCPx pin is cleared.
The maximum PWM resolution (bits) for a given PWM
frequency is given by the equation:
EQUATION 15-3:
TABLE 15-4: EXAMPLE PWM FREQUENCIES AND RESOLUTIONS AT 40 MHz
15.4.3 PWM AUTO-SHUTDOWN
(CCP1 ONLY)
The PWM auto-shutdown features of the enhanced CCP
module are also available to CCP1 in 28-pin devices.
The operation of this feature is discussed in detail in
Section 16.4.7 “Enhanced PWM Auto-Shutdown”.
Auto-shutdown features are not available for CCP2.
15.4.4 SETUP FOR PWM OPERATION
The following steps should be taken when configuring
the CCP module for PWM operation:
1. Set the PWM period by writing to the PR2
register.
2. Set the PWM duty cycle by writing to the
CCPRxL register and CCPxCON<5:4> bits.
3. Make the CCPx pin an output by clearing the
appropriate TRIS bit.
4. Set the TMR2 prescale value, then enable
Timer2 by writing to T2CON.
5. Configure the CCPx module for PWM operation.
Note: If the PWM duty cycle value is longer than
the PWM period, the CCP2 pin will not be
cleared.
FOSC
FPWM
⎝---------------⎠
log⎛ ⎞
log(2)
PWM Resolution (max) = -----------------------------bits
PWM Frequency 2.44 kHz 9.77 kHz 39.06 kHz 156.25 kHz 312.50 kHz 416.67 kHz
Timer Prescaler (1, 4, 16) 16 4 1 1 1 1
PR2 Value FFh FFh FFh 3Fh 1Fh 17h
Maximum Resolution (bits) 10 10 10 8 7 6.58
PIC18F2420/2520/4420/4520
DS39631B-page 146 Preliminary © 2007 Microchip Technology Inc.
TABLE 15-5: REGISTERS ASSOCIATED WITH PWM AND TIMER2
Name Bit 7 Bit 6 Bit 5 Bit 4 Bit 3 Bit 2 Bit 1 Bit 0
Reset
Values
on page
INTCON GIE/GIEH PEIE/GIEL TMR0IE INT0IE RBIE TMR0IF INT0IF RBIF 49
RCON IPEN SBOREN — RI TO PD POR BOR 48
PIR1 PSPIF(1) ADIF RCIF TXIF SSPIF CCP1IF TMR2IF TMR1IF 52
PIE1 PSPIE(1) ADIE RCIE TXIE SSPIE CCP1IE TMR2IE TMR1IE 52
IPR1 PSPIP(1) ADIP RCIP TXIP SSPIP CCP1IP TMR2IP TMR1IP 52
TRISB PORTB Data Direction Control Register 52
TRISC PORTC Data Direction Control Register 52
TMR2 Timer2 Register 50
PR2 Timer2 Period Register 50
T2CON — T2OUTPS3 T2OUTPS2 T2OUTPS1 T2OUTPS0 TMR2ON T2CKPS1 T2CKPS0 50
CCPR1L Capture/Compare/PWM Register 1, Low Byte 51
CCPR1H Capture/Compare/PWM Register 1, High Byte 51
CCP1CON P1M1(1) P1M0(1) DC1B1 DC1B0 CCP1M3 CCP1M2 CCP1M1 CCP1M0 51
CCPR2L Capture/Compare/PWM Register 2, Low Byte 51
CCPR2H Capture/Compare/PWM Register 2, High Byte 51
CCP2CON — — DC2B1 DC2B0 CCP2M3 CCP2M2 CCP2M1 CCP2M0 51
ECCP1AS ECCPASE ECCPAS2 ECCPAS1 ECCPAS0 PSSAC1 PSSAC0 PSSBD1(1) PSSBD0(1) 51
PWM1CON PRSEN PDC6(1) PDC5(1) PDC4(1) PDC3(1) PDC2(1) PDC1(1) PDC0(1) 51
Legend: — = unimplemented, read as ‘0’. Shaded cells are not used by PWM or Timer2.
Note 1: These bits are unimplemented on 28-pin devices; always maintain these bits clear.
© 2007 Microchip Technology Inc. Preliminary DS39631B-page 147
PIC18F2420/2520/4420/4520
16.0 ENHANCED CAPTURE/
COMPARE/PWM (ECCP)
MODULE
In PIC18F4420/4520 devices, CCP1 is implemented
as a standard CCP module with enhanced PWM
capabilities. These include the provision for 2 or 4
output channels, user selectable polarity, dead-band
control and automatic shutdown and restart. The
enhanced features are discussed in detail in
Section 16.4 “Enhanced PWM Mode”. Capture,
Compare and single-output PWM functions of the
ECCP module are the same as described for the
standard CCP module.
The control register for the enhanced CCP module is
shown in Register 16-1. It differs from the CCPxCON
registers in PIC18F2420/2520 devices in that the two
Most Significant bits are implemented to control PWM
functionality.
REGISTER 16-1: CCP1CON REGISTER (ECCP1 MODULE, 40/44-PIN DEVICES)
Note: The ECCP module is implemented only in
40/44-pin devices.
R/W-0 R/W-0 R/W-0 R/W-0 R/W-0 R/W-0 R/W-0 R/W-0
P1M1 P1M0 DC1B1 DC1B0 CCP1M3 CCP1M2 CCP1M1 CCP1M0
bit 7 bit 0
bit 7-6 P1M1:P1M0: Enhanced PWM Output Configuration bits
If CCP1M3:CCP1M2 = 00, 01, 10:
xx = P1A assigned as Capture/Compare input/output; P1B, P1C, P1D assigned as port pins
If CCP1M3:CCP1M2 = 11:
00 = Single output: P1A modulated; P1B, P1C, P1D assigned as port pins
01 = Full-bridge output forward: P1D modulated; P1A active; P1B, P1C inactive
10 = Half-bridge output: P1A, P1B modulated with dead-band control; P1C, P1D assigned
as port pins
11 = Full-bridge output reverse: P1B modulated; P1C active; P1A, P1D inactive
bit 5-4 DC1B1:DC1B0: PWM Duty Cycle bit 1 and bit 0
Capture mode:
Unused.
Compare mode:
Unused.
PWM mode:
These bits are the two LSbs of the 10-bit PWM duty cycle. The eight MSbs of the duty cycle are
found in CCPR1L.
bit 3-0 CCP1M3:CCP1M0: Enhanced CCP Mode Select bits
0000 = Capture/Compare/PWM off (resets ECCP module)
0001 = Reserved
0010 = Compare mode, toggle output on match
0011 = Capture mode
0100 = Capture mode, every falling edge
0101 = Capture mode, every rising edge
0110 = Capture mode, every 4th rising edge
0111 = Capture mode, every 16th rising edge
1000 = Compare mode, initialize CCP1 pin low, set output on compare match (set CCP1IF)
1001 = Compare mode, initialize CCP1 pin high, clear output on compare match (set CCP1IF)
1010 = Compare mode, generate software interrupt only, CCP1 pin reverts to I/O state
1011 = Compare mode, trigger special event (ECCP resets TMR1 or TMR3, sets CC1IF bit)
1100 = PWM mode; P1A, P1C active-high; P1B, P1D active-high
1101 = PWM mode; P1A, P1C active-high; P1B, P1D active-low
1110 = PWM mode; P1A, P1C active-low; P1B, P1D active-high
1111 = PWM mode; P1A, P1C active-low; P1B, P1D active-low
Legend:
R = Readable bit W = Writable bit U = Unimplemented bit, read as ‘0’
-n = Value at POR ‘1’ = Bit is set ‘0’ = Bit is cleared x = Bit is unknown
PIC18F2420/2520/4420/4520
DS39631B-page 148 Preliminary © 2007 Microchip Technology Inc.
In addition to the expanded range of modes available
through the CCP1CON register and ECCP1AS
register, the ECCP module has an additional register
associated with Enhanced PWM operation and
auto-shutdown features. It is:
• PWM1CON (Dead-band delay)
16.1 ECCP Outputs and Configuration
The enhanced CCP module may have up to four PWM
outputs, depending on the selected operating mode.
These outputs, designated P1A through P1D, are
multiplexed with I/O pins on PORTC and PORTD. The
outputs that are active depend on the CCP operating
mode selected. The pin assignments are summarized
in Table 16-1.
To configure the I/O pins as PWM outputs, the proper
PWM mode must be selected by setting the
P1M1:P1M0 and CCP1M3:CCP1M0 bits. The
appropriate TRISC and TRISD direction bits for the port
pins must also be set as outputs.
16.1.1 ECCP MODULES AND TIMER
RESOURCES
Like the standard CCP modules, the ECCP module can
utilize Timers 1, 2 or 3, depending on the mode
selected. Timer1 and Timer3 are available for modules
in Capture or Compare modes, while Timer2 is available
for modules in PWM mode. Interactions between
the standard and enhanced CCP modules are identical
to those described for standard CCP modules.
Additional details on timer resources are provided in
Section 15.1.1 “CCP Modules and Timer
Resources”.
16.2 Capture and Compare Modes
Except for the operation of the Special Event Trigger
discussed below, the Capture and Compare modes of
the ECCP module are identical in operation to that of
CCP2. These are discussed in detail in Section 15.2
“Capture Mode” and Section 15.3 “Compare
Mode”. No changes are required when moving
between 28-pin and 40/44-pin devices.
16.2.1 SPECIAL EVENT TRIGGER
The Special Event Trigger output of ECCP1 resets the
TMR1 or TMR3 register pair, depending on which timer
resource is currently selected. This allows the CCPR1
register to effectively be a 16-bit programmable period
register for Timer1 or Timer3.
16.3 Standard PWM Mode
When configured in Single Output mode, the ECCP
module functions identically to the standard CCP
module in PWM mode, as described in Section 15.4
“PWM Mode”. This is also sometimes referred to as
“Compatible CCP” mode, as in Table 16-1.
TABLE 16-1: PIN ASSIGNMENTS FOR VARIOUS ECCP1 MODES
Note: When setting up single output PWM operations,
users are free to use either of the processes
described in Section 15.4.4 “Setup
for PWM Operation” or Section 16.4.9
“Setup for PWM Operation”. The latter is
more generic and will work for either single
or multi-output PWM.
ECCP Mode
CCP1CON
Configuration
RC2 RD5 RD6 RD7
All 40/44-pin devices:
Compatible CCP 00xx 11xx CCP1 RD5/PSP5 RD6/PSP6 RD7/PSP7
Dual PWM 10xx 11xx P1A P1B RD6/PSP6 RD7/PSP7
Quad PWM x1xx 11xx P1A P1B P1C P1D
Legend: x = Don’t care. Shaded cells indicate pin assignments not used by ECCP1 in a given mode.
© 2007 Microchip Technology Inc. Preliminary DS39631B-page 149
PIC18F2420/2520/4420/4520
16.4 Enhanced PWM Mode
The Enhanced PWM mode provides additional PWM
output options for a broader range of control applications.
The module is a backward compatible version of
the standard CCP module and offers up to four outputs,
designated P1A through P1D. Users are also able to
select the polarity of the signal (either active-high or
active-low). The module’s output mode and polarity are
configured by setting the P1M1:P1M0 and
CCP1M3:CCP1M0 bits of the CCP1CON register.
Figure 16-1 shows a simplified block diagram of PWM
operation. All control registers are double-buffered and
are loaded at the beginning of a new PWM cycle (the
period boundary when Timer2 resets) in order to prevent
glitches on any of the outputs. The exception is the
PWM Delay register, PWM1CON, which is loaded at
either the duty cycle boundary or the period boundary
(whichever comes first). Because of the buffering, the
module waits until the assigned timer resets, instead of
starting immediately. This means that enhanced PWM
waveforms do not exactly match the standard PWM
waveforms, but are instead offset by one full instruction
cycle (4 TOSC).
As before, the user must manually configure the
appropriate TRIS bits for output.
16.4.1 PWM PERIOD
The PWM period is specified by writing to the PR2
register. The PWM period can be calculated using the
following equation.
EQUATION 16-1:
PWM frequency is defined as 1/[PWM period]. When
TMR2 is equal to PR2, the following three events occur
on the next increment cycle:
• TMR2 is cleared
• The CCP1 pin is set (if PWM duty cycle = 0%, the
CCP1 pin will not be set)
• The PWM duty cycle is copied from CCPR1L into
CCPR1H
FIGURE 16-1: SIMPLIFIED BLOCK DIAGRAM OF THE ENHANCED PWM MODULE
Note: The Timer2 postscaler (see Section 13.0
“Timer2 Module”) is not used in the
determination of the PWM frequency. The
postscaler could be used to have a servo
update rate at a different frequency than
the PWM output.
PWM Period = [(PR2) + 1] • 4 • TOSC •
(TMR2 Prescale Value)
CCPR1L
CCPR1H (Slave)
Comparator
TMR2
Comparator
PR2
(Note 1)
R Q
S
Duty Cycle Registers
CCP1CON<5:4>
Clear Timer,
set CCP1 pin and
latch D.C.
Note: The 8-bit TMR2 register is concatenated with the 2-bit internal Q clock, or 2 bits of the prescaler, to create the 10-bit
time base.
TRISx
CCP1/P1A
TRISx
P1B
TRISx
TRISx
P1D
Output
Controller
P1M1<1:0>
2
CCP1M<3:0>
4
PWM1CON
CCP1/P1A
P1B
P1C
P1D
P1C
PIC18F2420/2520/4420/4520
DS39631B-page 150 Preliminary © 2007 Microchip Technology Inc.
16.4.2 PWM DUTY CYCLE
The PWM duty cycle is specified by writing to the
CCPR1L register and to the CCP1CON<5:4> bits. Up
to 10-bit resolution is available. The CCPR1L contains
the eight MSbs and the CCP1CON<5:4> contains the
two LSbs. This 10-bit value is represented by
CCPR1L:CCP1CON<5:4>. The PWM duty cycle is
calculated by the following equation.
EQUATION 16-2:
CCPR1L and CCP1CON<5:4> can be written to at any
time, but the duty cycle value is not copied into
CCPR1H until a match between PR2 and TMR2 occurs
(i.e., the period is complete). In PWM mode, CCPR1H
is a read-only register.
The CCPR1H register and a 2-bit internal latch are
used to double-buffer the PWM duty cycle. This
double-buffering is essential for glitchless PWM operation.
When the CCPR1H and 2-bit latch match TMR2,
concatenated with an internal 2-bit Q clock or two bits
of the TMR2 prescaler, the CCP1 pin is cleared. The
maximum PWM resolution (bits) for a given PWM
frequency is given by the following equation.
EQUATION 16-3:
16.4.3 PWM OUTPUT CONFIGURATIONS
The P1M1:P1M0 bits in the CCP1CON register allow
one of four configurations:
• Single Output
• Half-Bridge Output
• Full-Bridge Output, Forward mode
• Full-Bridge Output, Reverse mode
The Single Output mode is the standard PWM mode
discussed in Section 16.4 “Enhanced PWM Mode”.
The Half-Bridge and Full-Bridge Output modes are
covered in detail in the sections that follow.
The general relationship of the outputs in all
configurations is summarized in Figure 16-2.
TABLE 16-2: EXAMPLE PWM FREQUENCIES AND RESOLUTIONS AT 40 MHz
PWM Duty Cycle = (CCPR1L:CCP1CON<5:4>) •
TOSC • (TMR2 Prescale Value)
Note: If the PWM duty cycle value is longer than
the PWM period, the CCP1 pin will not be
cleared.
( )
PWM Resolution (max) =
FOSC
FPWM
log
log(2)
bits
PWM Frequency 2.44 kHz 9.77 kHz 39.06 kHz 156.25 kHz 312.50 kHz 416.67 kHz
Timer Prescaler (1, 4, 16) 16 4 1 1 1 1
PR2 Value FFh FFh FFh 3Fh 1Fh 17h
Maximum Resolution (bits) 10 10 10 8 7 6.58
© 2007 Microchip Technology Inc. Preliminary DS39631B-page 151
PIC18F2420/2520/4420/4520
FIGURE 16-2: PWM OUTPUT RELATIONSHIPS (ACTIVE-HIGH STATE)
FIGURE 16-3: PWM OUTPUT RELATIONSHIPS (ACTIVE-LOW STATE)
0
Period
00
10
01
11
SIGNAL
PR2 + 1
CCP1CON
<7:6>
P1A Modulated
P1A Modulated
P1B Modulated
P1A Active
P1B Inactive
P1C Inactive
P1D Modulated
P1A Inactive
P1B Modulated
P1C Active
P1D Inactive
Duty
Cycle
(Single Output)
(Half-Bridge)
(Full-Bridge,
Forward)
(Full-Bridge,
Reverse)
Delay(1) Delay(1)
0
Period
00
10
01
11
SIGNAL
PR2 + 1
CCP1CON
<7:6>
P1A Modulated
P1A Modulated
P1B Modulated
P1A Active
P1B Inactive
P1C Inactive
P1D Modulated
P1A Inactive
P1B Modulated
P1C Active
P1D Inactive
Duty
Cycle
(Single Output)
(Half-Bridge)
(Full-Bridge,
Forward)
(Full-Bridge,
Reverse)
Delay(1) Delay(1)
Relationships:
• Period = 4 * TOSC * (PR2 + 1) * (TMR2 Prescale Value)
• Duty Cycle = TOSC * (CCPR1L<7:0>:CCP1CON<5:4>) * (TMR2 Prescale Value)
• Delay = 4 * TOSC * (PWM1CON<6:0>)
Note 1: Dead-band delay is programmed using the PWM1CON register (see Section 16.4.6 “Programmable
Dead-Band Delay”).
PIC18F2420/2520/4420/4520
DS39631B-page 152 Preliminary © 2007 Microchip Technology Inc.
16.4.4 HALF-BRIDGE MODE
In the Half-Bridge Output mode, two pins are used as
outputs to drive push-pull loads. The PWM output signal
is output on the P1A pin, while the complementary PWM
output signal is output on the P1B pin (Figure 16-4). This
mode can be used for half-bridge applications, as shown
in Figure 16-5, or for full-bridge applications where four
power switches are being modulated with two PWM
signals.
In Half-Bridge Output mode, the programmable deadband
delay can be used to prevent shoot-through
current in half-bridge power devices. The value of bits,
PDC6:PDC0, sets the number of instruction cycles
before the output is driven active. If the value is greater
than the duty cycle, the corresponding output remains
inactive during the entire cycle. See Section 16.4.6
“Programmable Dead-Band Delay” for more details
of the dead-band delay operations.
Since the P1A and P1B outputs are multiplexed with
the PORTC<2> and PORTD<5> data latches, the
TRISC<2> and TRISD<5> bits must be cleared to
configure P1A and P1B as outputs.
FIGURE 16-4: HALF-BRIDGE PWM
OUTPUT
FIGURE 16-5: EXAMPLES OF HALF-BRIDGE OUTPUT MODE APPLICATIONS
Period
Duty Cycle
td
td
(1)
P1A(2)
P1B(2)
td = Dead-Band Delay
Period
(1) (1)
Note 1: At this time, the TMR2 register is equal to the
PR2 register.
2: Output signals are shown as active-high.
PIC18F4X2X
P1A
P1B
FET
Driver
FET
Driver
V+
VLoad
+
V-
+
VFET
Driver
FET
Driver
V+
VLoad
FET
Driver
FET
Driver
PIC18F4X2X
P1A
P1B
Standard Half-Bridge Circuit (“Push-Pull”)
Half-Bridge Output Driving a Full-Bridge Circuit
© 2007 Microchip Technology Inc. Preliminary DS39631B-page 153
PIC18F2420/2520/4420/4520
16.4.5 FULL-BRIDGE MODE
In Full-Bridge Output mode, four pins are used as
outputs; however, only two outputs are active at a time.
In the Forward mode, pin P1A is continuously active
and pin P1D is modulated. In the Reverse mode, pin
P1C is continuously active and pin P1B is modulated.
These are illustrated in Figure 16-6.
P1A, P1B, P1C and P1D outputs are multiplexed with
the PORTC<2> and PORTD<7:5> data latches. The
TRISC<2> and TRISD<7:5> bits must be cleared to
make the P1A, P1B, P1C and P1D pins outputs.
FIGURE 16-6: FULL-BRIDGE PWM OUTPUT
Period
Duty Cycle
P1A(2)
P1B(2)
P1C(2)
P1D(2)
Forward Mode
(1)
Period
Duty Cycle
P1A(2)
P1C(2)
P1D(2)
P1B(2)
Reverse Mode
(1)
(1) (1)
Note 1: At this time, the TMR2 register is equal to the PR2 register.
Note 2: Output signal is shown as active-high.
PIC18F2420/2520/4420/4520
DS39631B-page 154 Preliminary © 2007 Microchip Technology Inc.
FIGURE 16-7: EXAMPLE OF FULL-BRIDGE APPLICATION
16.4.5.1 Direction Change in Full-Bridge Mode
In the Full-Bridge Output mode, the P1M1 bit in the
CCP1CON register allows user to control the forward/
reverse direction. When the application firmware
changes this direction control bit, the module will
assume the new direction on the next PWM cycle.
Just before the end of the current PWM period, the
modulated outputs (P1B and P1D) are placed in their
inactive state, while the unmodulated outputs (P1A and
P1C) are switched to drive in the opposite direction.
This occurs in a time interval of 4 TOSC * (Timer2
Prescale Value) before the next PWM period begins.
The Timer2 prescaler will be either 1, 4 or 16, depending
on the value of the T2CKPS1:T2CKPS0 bits
(T2CON<1:0>). During the interval from the switch of
the unmodulated outputs to the beginning of the next
period, the modulated outputs (P1B and P1D) remain
inactive. This relationship is shown in Figure 16-8.
Note that in the Full-Bridge Output mode, the CCP1
module does not provide any dead-band delay. In general,
since only one output is modulated at all times,
dead-band delay is not required. However, there is a
situation where a dead-band delay might be required.
This situation occurs when both of the following
conditions are true:
1. The direction of the PWM output changes when
the duty cycle of the output is at or near 100%.
2. The turn-off time of the power switch, including
the power device and driver circuit, is greater
than the turn-on time.
Figure 16-9 shows an example where the PWM
direction changes from forward to reverse at a near
100% duty cycle. At time t1, the outputs P1A and P1D
become inactive, while output P1C becomes active. In
this example, since the turn-off time of the power
devices is longer than the turn-on time, a shoot-through
current may flow through power devices, QC and QD
(see Figure 16-7), for the duration of ‘t’. The same
phenomenon will occur to power devices, QA and QB,
for PWM direction change from reverse to forward.
If changing PWM direction at high duty cycle is required
for an application, one of the following requirements
must be met:
1. Reduce PWM for a PWM period before
changing directions.
2. Use switch drivers that can drive the switches off
faster than they can drive them on.
Other options to prevent shoot-through current may
exist.
P1A
P1C
FET
Driver
FET
Driver
V+
VLoad
FET
Driver
FET
Driver
P1B
P1D
QA
QB QD
PIC18F4X2X QC
© 2007 Microchip Technology Inc. Preliminary DS39631B-page 155
PIC18F2420/2520/4420/4520
FIGURE 16-8: PWM DIRECTION CHANGE
FIGURE 16-9: PWM DIRECTION CHANGE AT NEAR 100% DUTY CYCLE
DC
Period(1)
SIGNAL
Note 1: The direction bit in the CCP1 Control register (CCP1CON<7>) is written any time during the PWM cycle.
2: When changing directions, the P1A and P1C signals switch before the end of the current PWM cycle at intervals
of 4 TOSC, 16 TOSC or 64 TOSC, depending on the Timer2 prescaler value. The modulated P1B and P1D signals
are inactive at this time.
Period
(Note 2)
P1A (Active-High)
P1B (Active-High)
P1C (Active-High)
P1D (Active-High)
DC
Forward Period Reverse Period
P1A(1)
tON
(2)
tOFF
(3)
t = tOFF – tON
(2,3)
P1B(1)
P1C(1)
P1D(1)
External Switch D(1)
Potential
Shoot-Through
Current(1)
Note 1: All signals are shown as active-high.
2: tON is the turn-on delay of power switch QC and its driver.
3: tOFF is the turn-off delay of power switch QD and its driver.
External Switch C(1)
t1
DC
DC
PIC18F2420/2520/4420/4520
DS39631B-page 156 Preliminary © 2007 Microchip Technology Inc.
16.4.6 PROGRAMMABLE DEAD-BAND
DELAY
In half-bridge applications where all power switches are
modulated at the PWM frequency at all times, the
power switches normally require more time to turn off
than to turn on. If both the upper and lower power
switches are switched at the same time (one turned on
and the other turned off), both switches may be on for
a short period of time until one switch completely turns
off. During this brief interval, a very high current (shootthrough
current) may flow through both power
switches, shorting the bridge supply. To avoid this
potentially destructive shoot-through current from flowing
during switching, turning on either of the power
switches is normally delayed to allow the other switch
to completely turn off.
In the Half-Bridge Output mode, a digitally programmable
dead-band delay is available to avoid shoot-through
current from destroying the bridge power switches. The
delay occurs at the signal transition from the nonactive
state to the active state. See Figure 16-4 for illustration.
Bits PDC6:PDC0 of the PWM1CON register
(Register 16-2) set the delay period in terms of microcontroller
instruction cycles (TCY or 4 TOSC). These bits
are not available on 28-pin devices as the standard
CCP module does not support half-bridge operation.
16.4.7 ENHANCED PWM AUTO-SHUTDOWN
When the CCP1 is programmed for any of the enhanced
PWM modes, the active output pins may be configured
for auto-shutdown. Auto-shutdown immediately places
the enhanced PWM output pins into a defined shutdown
state when a shutdown event occurs.
A shutdown event can be caused by either of the
comparator modules, a low level on the Fault input pin
(FLT0) or any combination of these three sources. The
comparators may be used to monitor a voltage input
proportional to a current being monitored in the bridge
circuit. If the voltage exceeds a threshold, the
comparator switches state and triggers a shutdown.
Alternatively, a low digital signal on FLT0 can also trigger
a shutdown. The auto-shutdown feature can be disabled
by not selecting any auto-shutdown sources. The autoshutdown
sources to be used are selected using the
ECCPAS2:ECCPAS0 bits (bits<6:4> of the ECCP1AS
register).
When a shutdown occurs, the output pins are asynchronously
placed in their shutdown states, specified
by the PSSAC1:PSSAC0 and PSSBD1:PSSBD0 bits
(ECCPAS2:ECCPAS0). Each pin pair (P1A/P1C and
P1B/P1D) may be set to drive high, drive low or be tristated
(not driving). The ECCPASE bit (ECCP1AS<7>)
is also set to hold the enhanced PWM outputs in their
shutdown states.
The ECCPASE bit is set by hardware when a shutdown
event occurs. If automatic restarts are not enabled, the
ECCPASE bit is cleared by firmware when the cause of
the shutdown clears. If automatic restarts are enabled,
the ECCPASE bit is automatically cleared when the
cause of the auto-shutdown has cleared.
If the ECCPASE bit is set when a PWM period begins,
the PWM outputs remain in their shutdown state for that
entire PWM period. When the ECCPASE bit is cleared,
the PWM outputs will return to normal operation at the
beginning of the next PWM period.
REGISTER 16-2: PWM1CON: PWM CONFIGURATION REGISTER
Note: Programmable dead-band delay is not
implemented in 28-pin devices with
standard CCP modules.
Note: Writing to the ECCPASE bit is disabled
while a shutdown condition is active.
R/W-0 R/W-0 R/W-0 R/W-0 R/W-0 R/W-0 R/W-0 R/W-0
PRSEN PDC6(1) PDC5(1) PDC4(1) PDC3(1) PDC2(1) PDC1(1) PDC0(1)
bit 7 bit 0
bit 7 PRSEN: PWM Restart Enable bit
1 = Upon auto-shutdown, the ECCPASE bit clears automatically once the shutdown event
goes away; the PWM restarts automatically
0 = Upon auto-shutdown, ECCPASE must be cleared in software to restart the PWM
bit 6-0 PDC6:PDC0: PWM Delay Count bits(1)
Delay time, in number of FOSC/4 (4 * TOSC) cycles, between the scheduled and actual time for
a PWM signal to transition to active.
Note 1: Reserved on 28-pin devices; maintain these bits clear.
Legend:
R = Readable bit W = Writable bit U = Unimplemented bit, read as ‘0’
-n = Value at POR ‘1’ = Bit is set ‘0’ = Bit is cleared x = Bit is unknown
© 2007 Microchip Technology Inc. Preliminary DS39631B-page 157
PIC18F2420/2520/4420/4520
REGISTER 16-3: ECCP1AS: ENHANCED CAPTURE/COMPARE/PWM AUTO-SHUTDOWN
CONTROL REGISTER
R/W-0 R/W-0 R/W-0 R/W-0 R/W-0 R/W-0 R/W-0 R/W-0
ECCPASE ECCPAS2 ECCPAS1 ECCPAS0 PSSAC1 PSSAC0 PSSBD1(1) PSSBD0(1)
bit 7 bit 0
bit 7 ECCPASE: ECCP Auto-Shutdown Event Status bit
1 = A shutdown event has occurred; ECCP outputs are in shutdown state
0 = ECCP outputs are operating
bit 6-4 ECCPAS2:ECCPAS0: ECCP Auto-Shutdown Source Select bits
111 = FLT0 or Comparator 1 or Comparator 2
110 = FLT0 or Comparator 2
101 = FLT0 or Comparator 1
100 = FLT0
011 = Either Comparator 1 or 2
010 = Comparator 2 output
001 = Comparator 1 output
000 = Auto-shutdown is disabled
bit 3-2 PSSAC1:PSSAC0: Pins A and C Shutdown State Control bits
1x = Pins A and C are tri-state (40/44-pin devices);
PWM output is tri-state (28-pin devices)
01 = Drive Pins A and C to ‘1’
00 = Drive Pins A and C to ‘0’
bit 1-0 PSSBD1:PSSBD0: Pins B and D Shutdown State Control bits(1)
1x = Pins B and D tri-state
01 = Drive Pins B and D to ‘1’
00 = Drive Pins B and D to ‘0’
Note 1: Reserved on 28-pin devices; maintain these bits clear.
Legend:
R = Readable bit W = Writable bit U = Unimplemented bit, read as ‘0’
-n = Value at POR ‘1’ = Bit is set ‘0’ = Bit is cleared x = Bit is unknown
PIC18F2420/2520/4420/4520
DS39631B-page 158 Preliminary © 2007 Microchip Technology Inc.
16.4.7.1 Auto-Shutdown and Automatic
Restart
The auto-shutdown feature can be configured to allow
automatic restarts of the module following a shutdown
event. This is enabled by setting the PRSEN bit of the
PWM1CON register (PWM1CON<7>).
In Shutdown mode with PRSEN = 1 (Figure 16-10), the
ECCPASE bit will remain set for as long as the cause
of the shutdown continues. When the shutdown condition
clears, the ECCP1ASE bit is cleared. If PRSEN = 0
(Figure 16-11), once a shutdown condition occurs, the
ECCPASE bit will remain set until it is cleared by firmware.
Once ECCPASE is cleared, the enhanced PWM
will resume at the beginning of the next PWM period.
Independent of the PRSEN bit setting, if the autoshutdown
source is one of the comparators, the
shutdown condition is a level. The ECCPASE bit
cannot be cleared as long as the cause of the shutdown
persists.
The Auto-Shutdown mode can be forced by writing a ‘1’
to the ECCPASE bit.
16.4.8 START-UP CONSIDERATIONS
When the ECCP module is used in the PWM mode, the
application hardware must use the proper external pullup
and/or pull-down resistors on the PWM output pins.
When the microcontroller is released from Reset, all of
the I/O pins are in the high-impedance state. The external
circuits must keep the power switch devices in the
off state until the microcontroller drives the I/O pins with
the proper signal levels, or activates the PWM
output(s).
The CCP1M1:CCP1M0 bits (CCP1CON<1:0>) allow
the user to choose whether the PWM output signals are
active-high or active-low for each pair of PWM output
pins (P1A/P1C and P1B/P1D). The PWM output
polarities must be selected before the PWM pins are
configured as outputs. Changing the polarity configuration
while the PWM pins are configured as outputs is
not recommended, since it may result in damage to the
application circuits.
The P1A, P1B, P1C and P1D output latches may not be
in the proper states when the PWM module is initialized.
Enabling the PWM pins for output at the same time as
the ECCP module may cause damage to the application
circuit. The ECCP module must be enabled in the
proper output mode and complete a full PWM cycle
before configuring the PWM pins as outputs. The completion
of a full PWM cycle is indicated by the TMR2IF
bit being set as the second PWM period begins.
FIGURE 16-10: PWM AUTO-SHUTDOWN (PRSEN = 1, AUTO-RESTART ENABLED)
FIGURE 16-11: PWM AUTO-SHUTDOWN (PRSEN = 0, AUTO-RESTART DISABLED)
Note: Writing to the ECCPASE bit is disabled
while a shutdown condition is active.
Shutdown
PWM
ECCPASE bit
Activity
Event
Shutdown
Event Occurs
Shutdown
Event Clears
PWM
Resumes
Normal PWM
Start of
PWM Period
PWM Period
Shutdown
PWM
ECCPASE bit
Activity
Event
Shutdown
Event Occurs
Shutdown
Event Clears
PWM
Resumes
Normal PWM
Start of
PWM Period
ECCPASE
Cleared by
Firmware
PWM Period
© 2007 Microchip Technology Inc. Preliminary DS39631B-page 159
PIC18F2420/2520/4420/4520
16.4.9 SETUP FOR PWM OPERATION
The following steps should be taken when configuring
the ECCP module for PWM operation:
1. Configure the PWM pins, P1A and P1B (and
P1C and P1D, if used), as inputs by setting the
corresponding TRIS bits.
2. Set the PWM period by loading the PR2 register.
3. If auto-shutdown is required:
• Disable auto-shutdown (ECCP1AS = 0)
• Configure source (FLT0, Comparator 1 or
Comparator 2)
• Wait for non-shutdown condition
4. Configure the ECCP module for the desired
PWM mode and configuration by loading the
CCP1CON register with the appropriate values:
• Select one of the available output
configurations and direction with the
P1M1:P1M0 bits.
• Select the polarities of the PWM output
signals with the CCP1M3:CCP1M0 bits.
5. Set the PWM duty cycle by loading the CCPR1L
register and CCP1CON<5:4> bits.
6. For Half-Bridge Output mode, set the deadband
delay by loading PWM1CON<6:0> with
the appropriate value.
7. If auto-shutdown operation is required, load the
ECCP1AS register:
• Select the auto-shutdown sources using the
ECCPAS2:ECCPAS0 bits.
• Select the shutdown states of the PWM
output pins using the PSSAC1:PSSAC0 and
PSSBD1:PSSBD0 bits.
• Set the ECCPASE bit (ECCP1AS<7>).
• Configure the comparators using the CMCON
register.
• Configure the comparator inputs as analog
inputs.
8. If auto-restart operation is required, set the
PRSEN bit (PWM1CON<7>).
9. Configure and start TMR2:
• Clear the TMR2 interrupt flag bit by clearing
the TMR2IF bit (PIR1<1>).
• Set the TMR2 prescale value by loading the
T2CKPS bits (T2CON<1:0>).
• Enable Timer2 by setting the TMR2ON bit
(T2CON<2>).
10. Enable PWM outputs after a new PWM cycle
has started:
• Wait until TMRn overflows (TMRnIF bit is set).
• Enable the CCP1/P1A, P1B, P1C and/or P1D
pin outputs by clearing the respective TRIS
bits.
• Clear the ECCPASE bit (ECCP1AS<7>).
16.4.10 OPERATION IN POWER MANAGED
MODES
In Sleep mode, all clock sources are disabled. Timer2
will not increment and the state of the module will not
change. If the ECCP pin is driving a value, it will continue
to drive that value. When the device wakes up, it
will continue from this state. If Two-Speed Start-ups are
enabled, the initial start-up frequency from INTOSC
and the postscaler may not be stable immediately.
In PRI_IDLE mode, the primary clock will continue to
clock the ECCP module without change. In all other
power managed modes, the selected power managed
mode clock will clock Timer2. Other power managed
mode clocks will most likely be different than the
primary clock frequency.
16.4.10.1 Operation with Fail-Safe
Clock Monitor
If the Fail-Safe Clock Monitor is enabled, a clock failure
will force the device into the RC_RUN Power Managed
mode and the OSCFIF bit (PIR2<7>) will be set. The
ECCP will then be clocked from the internal oscillator
clock source, which may have a different clock
frequency than the primary clock.
See the previous section for additional details.
16.4.11 EFFECTS OF A RESET
Both Power-on Reset and subsequent Resets will force
all ports to Input mode and the CCP registers to their
Reset states.
This forces the enhanced CCP module to reset to a
state compatible with the standard CCP module.
PIC18F2420/2520/4420/4520
DS39631B-page 160 Preliminary © 2007 Microchip Technology Inc.
TABLE 16-3: REGISTERS ASSOCIATED WITH ECCP1 MODULE AND TIMER1 TO TIMER3
Name Bit 7 Bit 6 Bit 5 Bit 4 Bit 3 Bit 2 Bit 1 Bit 0
Reset
Values
on page
INTCON GIE/GIEH PEIE/GIEL TMR0IE INT0IE RBIE TMR0IF INT0IF RBIF 49
RCON IPEN SBOREN — RI TO PD POR BOR 48
PIR1 PSPIF ADIF RCIF TXIF SSPIF CCP1IF TMR2IF TMR1IF 52
PIE1 PSPIE ADIE RCIE TXIE SSPIE CCP1IE TMR2IE TMR1IE 52
IPR1 PSPIP ADIP RCIP TXIP SSPIP CCP1IP TMR2IP TMR1IP 52
PIR2 OSCFIF CMIF — EEIF BCLIF HLVDIF TMR3IF CCP2IF 52
PIE2 OSCFIE CMIE — EEIE BCLIE HLVDIE TMR3IE CCP2IE 52
IPR2 OSCFIP CMIP — EEIP BCLIP HLVDIP TMR3IP CCP2IP 52
TRISB PORTB Data Direction Control Register 52
TRISC PORTC Data Direction Control Register 52
TRISD PORTD Data Direction Control Register 52
TMR1L Timer1 Register, Low Byte 50
TMR1H Timer1 Register, High Byte 50
T1CON RD16 T1RUN T1CKPS1 T1CKPS0 T1OSCEN T1SYNC TMR1CS TMR1ON 50
TMR2 Timer2 Register 50
T2CON — T2OUTPS3 T2OUTPS2 T2OUTPS1 T2OUTPS0 TMR2ON T2CKPS1 T2CKPS0 50
PR2 Timer2 Period Register 50
TMR3L Timer3 Register, Low Byte 51
TMR3H Timer3 Register, High Byte 51
T3CON RD16 T3CCP2 T3CKPS1 T3CKPS0 T3CCP1 T3SYNC TMR3CS TMR3ON 51
CCPR1L Capture/Compare/PWM Register 1, Low Byte 51
CCPR1H Capture/Compare/PWM Register 1, High Byte 51
CCP1CON P1M1(1) P1M0(1) DC1B1 DC1B0 CCP1M3 CCP1M2 CCP1M1 CCP1M0 51
ECCP1AS ECCPASE ECCPAS2 ECCPAS1 ECCPAS0 PSSAC1 PSSAC0 PSSBD1(1) PSSBD0(1) 51
PWM1CON PRSEN PDC6(1) PDC5(1) PDC4(1) PDC3(1) PDC2(1) PDC1(1) PDC0(1) 51
Legend: — = unimplemented, read as ‘0’. Shaded cells are not used during ECCP operation.
Note 1: These bits are unimplemented on 28-pin devices; always maintain these bits clear.
© 2007 Microchip Technology Inc. Preliminary DS39631B-page 161
PIC18F2420/2520/4420/4520
17.0 MASTER SYNCHRONOUS
SERIAL PORT (MSSP)
MODULE
17.1 Master SSP (MSSP) Module
Overview
The Master Synchronous Serial Port (MSSP) module is
a serial interface, useful for communicating with other
peripheral or microcontroller devices. These peripheral
devices may be serial EEPROMs, shift registers, display
drivers, A/D converters, etc. The MSSP module
can operate in one of two modes:
• Serial Peripheral Interface (SPI)
• Inter-Integrated Circuit (I2C)
- Full Master mode
- Slave mode (with general address call)
The I2C interface supports the following modes in
hardware:
• Master mode
• Multi-Master mode
• Slave mode
17.2 Control Registers
The MSSP module has three associated registers.
These include a status register (SSPSTAT) and two
control registers (SSPCON1 and SSPCON2). The use
of these registers and their individual configuration bits
differ significantly depending on whether the MSSP
module is operated in SPI or I2C mode.
Additional details are provided under the individual
sections.
17.3 SPI Mode
The SPI mode allows 8 bits of data to be synchronously
transmitted and received simultaneously. All four
modes of SPI are supported. To accomplish
communication, typically three pins are used:
• Serial Data Out (SDO) – RC5/SDO
• Serial Data In (SDI) – RC4/SDI/SDA
• Serial Clock (SCK) – RC3/SCK/SCL
Additionally, a fourth pin may be used when in a Slave
mode of operation:
• Slave Select (SS) – RA5/SS
Figure 17-1 shows the block diagram of the MSSP
module when operating in SPI mode.
FIGURE 17-1: MSSP BLOCK DIAGRAM
(SPI MODE)
( )
Read Write
Internal
Data Bus
SSPSR reg
SSPM3:SSPM0
bit 0 Shift
Clock
SS Control
Enable
Edge
Select
Clock Select
TMR2 Output
Prescaler TOSC
4, 16, 64
2
Edge
Select
2
4
Data to TX/RX in SSPSR
TRIS bit
2
SMP:CKE
RC5/SDO
SSPBUF reg
RC4/SDI/SDA
RA5/AN4/SS/
RC3/SCK/
SCL
HLVDIN/C2OUT
PIC18F2420/2520/4420/4520
DS39631B-page 162 Preliminary © 2007 Microchip Technology Inc.
17.3.1 REGISTERS
The MSSP module has four registers for SPI mode
operation. These are:
• MSSP Control Register 1 (SSPCON1)
• MSSP Status Register (SSPSTAT)
• Serial Receive/Transmit Buffer Register
(SSPBUF)
• MSSP Shift Register (SSPSR) – Not directly
accessible
SSPCON1 and SSPSTAT are the control and status
registers in SPI mode operation. The SSPCON1 register
is readable and writable. The lower 6 bits of the
SSPSTAT are read-only. The upper two bits of the
SSPSTAT are read/write.
SSPSR is the shift register used for shifting data in or
out. SSPBUF is the buffer register to which data bytes
are written to or read from.
In receive operations, SSPSR and SSPBUF together
create a double-buffered receiver. When SSPSR
receives a complete byte, it is transferred to SSPBUF
and the SSPIF interrupt is set.
During transmission, the SSPBUF is not doublebuffered.
A write to SSPBUF will write to both SSPBUF
and SSPSR.
REGISTER 17-1: SSPSTAT: MSSP STATUS REGISTER (SPI MODE)
R/W-0 R/W-0 R-0 R-0 R-0 R-0 R-0 R-0
SMP CKE D/A P S R/W UA BF
bit 7 bit 0
bit 7 SMP: Sample bit
SPI Master mode:
1 = Input data sampled at end of data output time
0 = Input data sampled at middle of data output time
SPI Slave mode:
SMP must be cleared when SPI is used in Slave mode.
bit 6 CKE: SPI Clock Select bit
1 = Transmit occurs on transition from active to Idle clock state
0 = Transmit occurs on transition from Idle to active clock state
Note: Polarity of clock state is set by the CKP bit (SSPCON1<4>).
bit 5 D/A: Data/Address bit
Used in I2C mode only.
bit 4 P: Stop bit
Used in I2C mode only. This bit is cleared when the MSSP module is disabled, SSPEN is
cleared.
bit 3 S: Start bit
Used in I2C mode only.
bit 2 R/W: Read/Write Information bit
Used in I2C mode only.
bit 1 UA: Update Address bit
Used in I2C mode only.
bit 0 BF: Buffer Full Status bit (Receive mode only)
1 = Receive complete, SSPBUF is full
0 = Receive not complete, SSPBUF is empty
Legend:
R = Readable bit W = Writable bit U = Unimplemented bit, read as ‘0’
-n = Value at POR ‘1’ = Bit is set ‘0’ = Bit is cleared x = Bit is unknown
© 2007 Microchip Technology Inc. Preliminary DS39631B-page 163
PIC18F2420/2520/4420/4520
REGISTER 17-2: SSPCON1: MSSP CONTROL REGISTER 1 (SPI MODE)
R/W-0 R/W-0 R/W-0 R/W-0 R/W-0 R/W-0 R/W-0 R/W-0
WCOL SSPOV SSPEN CKP SSPM3 SSPM2 SSPM1 SSPM0
bit 7 bit 0
bit 7 WCOL: Write Collision Detect bit (Transmit mode only)
1 = The SSPBUF register is written while it is still transmitting the previous word
(must be cleared in software)
0 = No collision
bit 6 SSPOV: Receive Overflow Indicator bit
SPI Slave mode:
1 = A new byte is received while the SSPBUF register is still holding the previous data. In case
of overflow, the data in SSPSR is lost. Overflow can only occur in Slave mode. The user
must read the SSPBUF, even if only transmitting data, to avoid setting overflow (must be
cleared in software).
0 = No overflow
Note: In Master mode, the overflow bit is not set since each new reception (and
transmission) is initiated by writing to the SSPBUF register.
bit 5 SSPEN: Synchronous Serial Port Enable bit
1 = Enables serial port and configures SCK, SDO, SDI and SS as serial port pins
0 = Disables serial port and configures these pins as I/O port pins
Note: When enabled, these pins must be properly configured as input or output.
bit 4 CKP: Clock Polarity Select bit
1 = Idle state for clock is a high level
0 = Idle state for clock is a low level
bit 3-0 SSPM3:SSPM0: Synchronous Serial Port Mode Select bits
0101 = SPI Slave mode, clock = SCK pin, SS pin control disabled, SS can be used as I/O pin
0100 = SPI Slave mode, clock = SCK pin, SS pin control enabled
0011 = SPI Master mode, clock = TMR2 output/2
0010 = SPI Master mode, clock = FOSC/64
0001 = SPI Master mode, clock = FOSC/16
0000 = SPI Master mode, clock = FOSC/4
Note: Bit combinations not specifically listed here are either reserved or implemented in
I2C mode only.
Legend:
R = Readable bit W = Writable bit U = Unimplemented bit, read as ‘0’
-n = Value at POR ‘1’ = Bit is set ‘0’ = Bit is cleared x = Bit is unknown
PIC18F2420/2520/4420/4520
DS39631B-page 164 Preliminary © 2007 Microchip Technology Inc.
17.3.2 OPERATION
When initializing the SPI, several options need to be
specified. This is done by programming the appropriate
control bits (SSPCON1<5:0> and SSPSTAT<7:6>).
These control bits allow the following to be specified:
• Master mode (SCK is the clock output)
• Slave mode (SCK is the clock input)
• Clock Polarity (Idle state of SCK)
• Data Input Sample Phase (middle or end of data
output time)
• Clock Edge (output data on rising/falling edge of
SCK)
• Clock Rate (Master mode only)
• Slave Select mode (Slave mode only)
The MSSP consists of a transmit/receive shift register
(SSPSR) and a buffer register (SSPBUF). The SSPSR
shifts the data in and out of the device, MSb first. The
SSPBUF holds the data that was written to the SSPSR
until the received data is ready. Once the 8 bits of data
have been received, that byte is moved to the SSPBUF
register. Then, the Buffer Full detect bit, BF
(SSPSTAT<0>) and the interrupt flag bit, SSPIF, are
set. This double-buffering of the received data
(SSPBUF) allows the next byte to start reception before
reading the data that was just received. Any write to the
SSPBUF register during transmission/reception of data
will be ignored and the write collision detect bit, WCOL
(SSPCON1<7>), will be set. User software must clear
the WCOL bit so that it can be determined if the following
write(s) to the SSPBUF register completed
successfully.
When the application software is expecting to receive
valid data, the SSPBUF should be read before the next
byte of data to transfer is written to the SSPBUF. The
Buffer Full bit, BF (SSPSTAT<0>), indicates when
SSPBUF has been loaded with the received data
(transmission is complete). When the SSPBUF is read,
the BF bit is cleared. This data may be irrelevant if the
SPI is only a transmitter. Generally, the MSSP interrupt
is used to determine when the transmission/reception
has completed. The SSPBUF must be read and/or
written. If the interrupt method is not going to be used,
then software polling can be done to ensure that a write
collision does not occur. Example 17-1 shows the
loading of the SSPBUF (SSPSR) for data transmission.
The SSPSR is not directly readable or writable and can
only be accessed by addressing the SSPBUF register.
Additionally, the MSSP status register (SSPSTAT)
indicates the various status conditions.
EXAMPLE 17-1: LOADING THE SSPBUF (SSPSR) REGISTER
LOOP BTFSS SSPSTAT, BF ;Has data been received (transmit complete)?
BRA LOOP ;No
MOVF SSPBUF, W ;WREG reg = contents of SSPBUF
MOVWF RXDATA ;Save in user RAM, if data is meaningful
MOVF TXDATA, W ;W reg = contents of TXDATA
MOVWF SSPBUF ;New data to xmit
© 2007 Microchip Technology Inc. Preliminary DS39631B-page 165
PIC18F2420/2520/4420/4520
17.3.3 ENABLING SPI I/O
To enable the serial port, SSP Enable bit, SSPEN
(SSPCON1<5>), must be set. To reset or reconfigure
SPI mode, clear the SSPEN bit, reinitialize the
SSPCON registers and then set the SSPEN bit. This
configures the SDI, SDO, SCK and SS pins as serial
port pins. For the pins to behave as the serial port function,
some must have their data direction bits (in the
TRIS register) appropriately programmed as follows:
• SDI is automatically controlled by the SPI module
• SDO must have TRISC<5> bit cleared
• SCK (Master mode) must have TRISC<3> bit
cleared
• SCK (Slave mode) must have TRISC<3> bit set
• SS must have TRISA<5> bit set
Any serial port function that is not desired may be
overridden by programming the corresponding data
direction (TRIS) register to the opposite value.
17.3.4 TYPICAL CONNECTION
Figure 17-2 shows a typical connection between two
microcontrollers. The master controller (Processor 1)
initiates the data transfer by sending the SCK signal.
Data is shifted out of both shift registers on their programmed
clock edge and latched on the opposite edge
of the clock. Both processors should be programmed to
the same Clock Polarity (CKP), then both controllers
would send and receive data at the same time.
Whether the data is meaningful (or dummy data)
depends on the application software. This leads to
three scenarios for data transmission:
• Master sends data – Slave sends dummy data
• Master sends data – Slave sends data
• Master sends dummy data – Slave sends data
FIGURE 17-2: SPI MASTER/SLAVE CONNECTION
Serial Input Buffer
(SSPBUF)
Shift Register
(SSPSR)
MSb LSb
SDO
SDI
PROCESSOR 1
SCK
SPI Master SSPM3:SSPM0 = 00xxb
Serial Input Buffer
(SSPBUF)
Shift Register
(SSPSR)
MSb LSb
SDI
SDO
PROCESSOR 2
SCK
SPI Slave SSPM3:SSPM0 = 010xb
Serial Clock
PIC18F2420/2520/4420/4520
DS39631B-page 166 Preliminary © 2007 Microchip Technology Inc.
17.3.5 MASTER MODE
The master can initiate the data transfer at any time
because it controls the SCK. The master determines
when the slave (Processor 2, Figure 17-2) is to
broadcast data by the software protocol.
In Master mode, the data is transmitted/received as
soon as the SSPBUF register is written to. If the SPI is
only going to receive, the SDO output could be disabled
(programmed as an input). The SSPSR register
will continue to shift in the signal present on the SDI pin
at the programmed clock rate. As each byte is
received, it will be loaded into the SSPBUF register as
if a normal received byte (interrupts and status bits
appropriately set). This could be useful in receiver
applications as a “Line Activity Monitor” mode.
The clock polarity is selected by appropriately
programming the CKP bit (SSPCON1<4>). This then,
would give waveforms for SPI communication as
shown in Figure 17-3, Figure 17-5 and Figure 17-6,
where the MSB is transmitted first. In Master mode, the
SPI clock rate (bit rate) is user programmable to be one
of the following:
• FOSC/4 (or TCY)
• FOSC/16 (or 4 • TCY)
• FOSC/64 (or 16 • TCY)
• Timer2 output/2
This allows a maximum data rate (at 40 MHz) of
10.00 Mbps.
Figure 17-3 shows the waveforms for Master mode.
When the CKE bit is set, the SDO data is valid before
there is a clock edge on SCK. The change of the input
sample is shown based on the state of the SMP bit. The
time when the SSPBUF is loaded with the received
data is shown.
FIGURE 17-3: SPI MODE WAVEFORM (MASTER MODE)
SCK
(CKP = 0
SCK
(CKP = 1
SCK
(CKP = 0
SCK
(CKP = 1
4 Clock
Modes
Input
Sample
Input
Sample
SDI
bit 7 bit 0
SDO bit 7 bit 6 bit 5 bit 4 bit 3 bit 2 bit 1 bit 0
bit 7
SDI
SSPIF
(SMP = 1)
(SMP = 0)
(SMP = 1)
CKE = 1)
CKE = 0)
CKE = 1)
CKE = 0)
(SMP = 0)
Write to
SSPBUF
SSPSR to
SSPBUF
SDO bit 7 bit 6 bit 5 bit 4 bit 3 bit 2 bit 1 bit 0
(CKE = 0)
(CKE = 1)
Next Q4 Cycle
after Q2↓
bit 0
© 2007 Microchip Technology Inc. Preliminary DS39631B-page 167
PIC18F2420/2520/4420/4520
17.3.6 SLAVE MODE
In Slave mode, the data is transmitted and received as
the external clock pulses appear on SCK. When the
last bit is latched, the SSPIF interrupt flag bit is set.
Before enabling the module in SPI Slave mode, the
clock line must match the proper Idle state. The clock
line can be observed by reading the SCK pin. The Idle
state is determined by the CKP bit (SSPCON1<4>).
While in Slave mode, the external clock is supplied by
the external clock source on the SCK pin. This external
clock must meet the minimum high and low times as
specified in the electrical specifications.
While in Sleep mode, the slave can transmit/receive
data. When a byte is received, the device will wake-up
from Sleep.
17.3.7 SLAVE SELECT
SYNCHRONIZATION
The SS pin allows a Synchronous Slave mode. The
SPI must be in Slave mode with SS pin control enabled
(SSPCON1<3:0> = 04h). The pin must not be driven
low for the SS pin to function as an input. The data latch
must be high. When the SS pin is low, transmission and
reception are enabled and the SDO pin is driven. When
the SS pin goes high, the SDO pin is no longer driven,
even if in the middle of a transmitted byte and becomes
a floating output. External pull-up/pull-down resistors
may be desirable depending on the application.
When the SPI module resets, the bit counter is forced
to ‘0’. This can be done by either forcing the SS pin to
a high level or clearing the SSPEN bit.
To emulate two-wire communication, the SDO pin can
be connected to the SDI pin. When the SPI needs to
operate as a receiver, the SDO pin can be configured
as an input. This disables transmissions from the SDO.
The SDI can always be left as an input (SDI function)
since it cannot create a bus conflict.
FIGURE 17-4: SLAVE SYNCHRONIZATION WAVEFORM
Note 1: When the SPI is in Slave mode with SS pin
control enabled (SSPCON<3:0> = 0100),
the SPI module will reset if the SS pin is set
to VDD.
2: If the SPI is used in Slave mode with CKE
set, then the SS pin control must be
enabled.
SCK
(CKP = 1
SCK
(CKP = 0
Input
Sample
SDI
bit 7
SDO bit 7 bit 6 bit 7
SSPIF
Interrupt
(SMP = 0)
CKE = 0)
CKE = 0)
(SMP = 0)
Write to
SSPBUF
SSPSR to
SSPBUF
SS
Flag
bit 0
bit 7
bit 0
Next Q4 Cycle
after Q2↓
PIC18F2420/2520/4420/4520
DS39631B-page 168 Preliminary © 2007 Microchip Technology Inc.
FIGURE 17-5: SPI MODE WAVEFORM (SLAVE MODE WITH CKE = 0)
FIGURE 17-6: SPI MODE WAVEFORM (SLAVE MODE WITH CKE = 1)
SCK
(CKP = 1
SCK
(CKP = 0
Input
Sample
SDI
bit 7
SDO bit 7 bit 6 bit 5 bit 4 bit 3 bit 2 bit 1 bit 0
SSPIF
Interrupt
(SMP = 0)
CKE = 0)
CKE = 0)
(SMP = 0)
Write to
SSPBUF
SSPSR to
SSPBUF
SS
Flag
Optional
Next Q4 Cycle
after Q2↓
bit 0
SCK
(CKP = 1
SCK
(CKP = 0
Input
Sample
SDI
bit 7 bit 0
SDO bit 7 bit 6 bit 5 bit 4 bit 3 bit 2 bit 1 bit 0
SSPIF
Interrupt
(SMP = 0)
CKE = 1)
CKE = 1)
(SMP = 0)
Write to
SSPBUF
SSPSR to
SSPBUF
SS
Flag
Not Optional
Next Q4 Cycle
after Q2↓
© 2007 Microchip Technology Inc. Preliminary DS39631B-page 169
PIC18F2420/2520/4420/4520
17.3.8 OPERATION IN POWER MANAGED
MODES
In SPI Master mode, module clocks may be operating
at a different speed than when in full power mode; in
the case of the Sleep mode, all clocks are halted.
In most Idle modes, a clock is provided to the peripherals.
That clock should be from the primary clock
source, the secondary clock (Timer1 oscillator at
32.768 kHz) or the INTOSC source. See Section 2.7
“Clock Sources and Oscillator Switching” for
additional information.
In most cases, the speed that the master clocks SPI
data is not important; however, this should be
evaluated for each system.
If MSSP interrupts are enabled, they can wake the controller
from Sleep mode, or one of the Idle modes, when
the master completes sending data. If an exit from
Sleep or Idle mode is not desired, MSSP interrupts
should be disabled.
If the Sleep mode is selected, all module clocks are
halted and the transmission/reception will remain in
that state until the devices wakes. After the device
returns to Run mode, the module will resume
transmitting and receiving data.
In SPI Slave mode, the SPI Transmit/Receive Shift
register operates asynchronously to the device. This
allows the device to be placed in any power managed
mode and data to be shifted into the SPI Transmit/
Receive Shift register. When all 8 bits have been
received, the MSSP interrupt flag bit will be set and if
enabled, will wake the device.
17.3.9 EFFECTS OF A RESET
A Reset disables the MSSP module and terminates the
current transfer.
17.3.10 BUS MODE COMPATIBILITY
Table 17-1 shows the compatibility between the
standard SPI modes and the states of the CKP and
CKE control bits.
TABLE 17-1: SPI BUS MODES
There is also an SMP bit which controls when the data
is sampled.
TABLE 17-2: REGISTERS ASSOCIATED WITH SPI OPERATION
Standard SPI Mode
Terminology
Control Bits State
CKP CKE
0, 0 0 1
0, 1 0 0
1, 0 1 1
1, 1 1 0
Name Bit 7 Bit 6 Bit 5 Bit 4 Bit 3 Bit 2 Bit 1 Bit 0
Reset
Values
on page
INTCON GIE/GIEH PEIE/GIEL TMR0IE INT0IE RBIE TMR0IF INT0IF RBIF 49
PIR1 PSPIF(1) ADIF RCIF TXIF SSPIF CCP1IF TMR2IF TMR1IF 52
PIE1 PSPIE(1) ADIE RCIE TXIE SSPIE CCP1IE TMR2IE TMR1IE 52
IPR1 PSPIP(1) ADIP RCIP TXIP SSPIP CCP1IP TMR2IP TMR1IP 52
TRISA TRISA7(2) TRISA6(2) PORTA Data Direction Control Register 52
TRISC PORTC Data Direction Control Register 52
SSPBUF SSP Receive Buffer/Transmit Register 50
SSPCON1 WCOL SSPOV SSPEN CKP SSPM3 SSPM2 SSPM1 SSPM0 50
SSPSTAT SMP CKE D/A P S R/W UA BF 50
Legend: Shaded cells are not used by the MSSP in SPI mode.
Note 1: These bits are unimplemented in 28-pin devices; always maintain these bits clear.
2: PORTA<7:6> and their direction bits are individually configured as port pins based on various primary
oscillator modes. When disabled, these bits read as ‘0’.
PIC18F2420/2520/4420/4520
DS39631B-page 170 Preliminary © 2007 Microchip Technology Inc.
17.4 I2C Mode
The MSSP module in I2C mode fully implements all
master and slave functions (including general call
support) and provides interrupts on Start and Stop bits
in hardware to determine a free bus (multi-master
function). The MSSP module implements the standard
mode specifications as well as 7-bit and 10-bit
addressing.
Two pins are used for data transfer:
• Serial clock (SCL) – RC3/SCK/SCL
• Serial data (SDA) – RC4/SDI/SDA
The user must configure these pins as inputs or outputs
through the TRISC<4:3> bits.
FIGURE 17-7: MSSP BLOCK DIAGRAM
(I2C MODE)
17.4.1 REGISTERS
The MSSP module has six registers for I2C operation.
These are:
• MSSP Control Register 1 (SSPCON1)
• MSSP Control Register 2 (SSPCON2)
• MSSP Status Register (SSPSTAT)
• Serial Receive/Transmit Buffer Register
(SSPBUF)
• MSSP Shift Register (SSPSR) – Not directly
accessible
• MSSP Address Register (SSPADD)
SSPCON1, SSPCON2 and SSPSTAT are the control
and status registers in I2C mode operation. The
SSPCON1 and SSPCON2 registers are readable and
writable. The lower 6 bits of the SSPSTAT are read-only.
The upper two bits of the SSPSTAT are read/write.
SSPSR is the shift register used for shifting data in or
out. SSPBUF is the buffer register to which data bytes
are written to or read from.
SSPADD register holds the slave device address when
the SSP is configured in I2C Slave mode. When the
SSP is configured in Master mode, the lower seven bits
of SSPADD act as the Baud Rate Generator reload
value.
In receive operations, SSPSR and SSPBUF together
create a double-buffered receiver. When SSPSR
receives a complete byte, it is transferred to SSPBUF
and the SSPIF interrupt is set.
During transmission, the SSPBUF is not doublebuffered.
A write to SSPBUF will write to both SSPBUF
and SSPSR.
Read Write
SSPSR reg
Match Detect
SSPADD reg
Start and
Stop bit Detect
SSPBUF reg
Internal
Data Bus
Addr Match
Set, Reset
S, P bits
(SSPSTAT reg)
RC3/SCK/SCL
RC4/SDI/
Shift
Clock
MSb
SDA
LSb
© 2007 Microchip Technology Inc. Preliminary DS39631B-page 171
PIC18F2420/2520/4420/4520
REGISTER 17-3: SSPSTAT: MSSP STATUS REGISTER (I2C MODE)
R/W-0 R/W-0 R-0 R-0 R-0 R-0 R-0 R-0
SMP CKE D/A P S R/W UA BF
bit 7 bit 0
bit 7 SMP: Slew Rate Control bit
In Master or Slave mode:
1 = Slew rate control disabled for standard speed mode (100 kHz and 1 MHz)
0 = Slew rate control enabled for high-speed mode (400 kHz)
bit 6 CKE: SMBus Select bit
In Master or Slave mode:
1 = Enable SMBus specific inputs
0 = Disable SMBus specific inputs
bit 5 D/A: Data/Address bit
In Master mode:
Reserved.
In Slave mode:
1 = Indicates that the last byte received or transmitted was data
0 = Indicates that the last byte received or transmitted was address
bit 4 P: Stop bit
1 = Indicates that a Stop bit has been detected last
0 = Stop bit was not detected last
Note: This bit is cleared on Reset and when SSPEN is cleared.
bit 3 S: Start bit
1 = Indicates that a Start bit has been detected last
0 = Start bit was not detected last
Note: This bit is cleared on Reset and when SSPEN is cleared.
bit 2 R/W: Read/Write Information bit (I2C mode only)
In Slave mode:
1 = Read
0 = Write
Note: This bit holds the R/W bit information following the last address match. This bit is
only valid from the address match to the next Start bit, Stop bit or not ACK bit.
In Master mode:
1 = Transmit is in progress
0 = Transmit is not in progress
Note: ORing this bit with SEN, RSEN, PEN, RCEN or ACKEN will indicate if the MSSP is
in Active mode.
bit 1 UA: Update Address bit (10-bit Slave mode only)
1 = Indicates that the user needs to update the address in the SSPADD register
0 = Address does not need to be updated
bit 0 BF: Buffer Full Status bit
In Transmit mode:
1 = SSPBUF is full
0 = SSPBUF is empty
In Receive mode:
1 = SSPBUF is full (does not include the ACK and Stop bits)
0 = SSPBUF is empty (does not include the ACK and Stop bits)
Legend:
R = Readable bit W = Writable bit U = Unimplemented bit, read as ‘0’
-n = Value at POR ‘1’ = Bit is set ‘0’ = Bit is cleared x = Bit is unknown
PIC18F2420/2520/4420/4520
DS39631B-page 172 Preliminary © 2007 Microchip Technology Inc.
REGISTER 17-4: SSPCON1: MSSP CONTROL REGISTER 1 (I2C MODE)
R/W-0 R/W-0 R/W-0 R/W-0 R/W-0 R/W-0 R/W-0 R/W-0
WCOL SSPOV SSPEN CKP SSPM3 SSPM2 SSPM1 SSPM0
bit 7 bit 0
bit 7 WCOL: Write Collision Detect bit
In Master Transmit mode:
1 = A write to the SSPBUF register was attempted while the I2C conditions were not valid for a
transmission to be started (must be cleared in software)
0 = No collision
In Slave Transmit mode:
1 = The SSPBUF register is written while it is still transmitting the previous word (must be
cleared in software)
0 = No collision
In Receive mode (Master or Slave modes):
This is a “don’t care” bit.
bit 6 SSPOV: Receive Overflow Indicator bit
In Receive mode:
1 = A byte is received while the SSPBUF register is still holding the previous byte (must be
cleared in software)
0 = No overflow
In Transmit mode:
This is a “don’t care” bit in Transmit mode.
bit 5 SSPEN: Synchronous Serial Port Enable bit
1 = Enables the serial port and configures the SDA and SCL pins as the serial port pins
0 = Disables serial port and configures these pins as I/O port pins
Note: When enabled, the SDA and SCL pins must be properly configured as input or
output.
bit 4 CKP: SCK Release Control bit
In Slave mode:
1 = Release clock
0 = Holds clock low (clock stretch), used to ensure data setup time
In Master mode:
Unused in this mode.
bit 3-0 SSPM3:SSPM0: Synchronous Serial Port Mode Select bits
1111 = I2C Slave mode, 10-bit address with Start and Stop bit interrupts enabled
1110 = I2C Slave mode, 7-bit address with Start and Stop bit interrupts enabled
1011 = I2C Firmware Controlled Master mode (Slave Idle)
1000 = I2C Master mode, clock = FOSC/(4 * (SSPADD + 1))
0111 = I2C Slave mode, 10-bit address
0110 = I2C Slave mode, 7-bit address
Bit combinations not specifically listed here are either reserved or implemented in SPI mode only.
Legend:
R = Readable bit W = Writable bit U = Unimplemented bit, read as ‘0’
-n = Value at POR ‘1’ = Bit is set ‘0’ = Bit is cleared x = Bit is unknown
© 2007 Microchip Technology Inc. Preliminary DS39631B-page 173
PIC18F2420/2520/4420/4520
REGISTER 17-5: SSPCON2: MSSP CONTROL REGISTER 2 (I2C MODE)
R/W-0 R/W-0 R/W-0 R/W-0 R/W-0 R/W-0 R/W-0 R/W-0
GCEN ACKSTAT ACKDT ACKEN(1) RCEN(1) PEN(1) RSEN(1) SEN(1)
bit 7 bit 0
bit 7 GCEN: General Call Enable bit (Slave mode only)
1 = Enable interrupt when a general call address (0000h) is received in the SSPSR
0 = General call address disabled
bit 6 ACKSTAT: Acknowledge Status bit (Master Transmit mode only)
1 = Acknowledge was not received from slave
0 = Acknowledge was received from slave
bit 5 ACKDT: Acknowledge Data bit (Master Receive mode only)
1 = Not Acknowledge
0 = Acknowledge
Note: Value that will be transmitted when the user initiates an Acknowledge sequence at
the end of a receive.
bit 4 ACKEN: Acknowledge Sequence Enable bit (Master Receive mode only)(1)
1 = Initiate Acknowledge sequence on SDA and SCL pins and transmit ACKDT data bit.
Automatically cleared by hardware.
0 = Acknowledge sequence Idle
bit 3 RCEN: Receive Enable bit (Master mode only)(1)
1 = Enables Receive mode for I2C
0 = Receive Idle
bit 2 PEN: Stop Condition Enable bit (Master mode only)(1)
1 = Initiate Stop condition on SDA and SCL pins. Automatically cleared by hardware.
0 = Stop condition Idle
bit 1 RSEN: Repeated Start Condition Enable bit (Master mode only)(1)
1 = Initiate Repeated Start condition on SDA and SCL pins. Automatically cleared by hardware.
0 = Repeated Start condition Idle
bit 0 SEN: Start Condition Enable/Stretch Enable bit(1)
In Master mode:
1 = Initiate Start condition on SDA and SCL pins. Automatically cleared by hardware.
0 = Start condition Idle
In Slave mode:
1 = Clock stretching is enabled for both slave transmit and slave receive (stretch enabled)
0 = Clock stretching is disabled
Note 1: For bits ACKEN, RCEN, PEN, RSEN, SEN: If the I2C module is not in the Idle mode,
these bits may not be set (no spooling) and the SSPBUF may not be written (or
writes to the SSPBUF are disabled).
Legend:
R = Readable bit W = Writable bit U = Unimplemented bit, read as ‘0’
-n = Value at POR ‘1’ = Bit is set ‘0’ = Bit is cleared x = Bit is unknown
PIC18F2420/2520/4420/4520
DS39631B-page 174 Preliminary © 2007 Microchip Technology Inc.
17.4.2 OPERATION
The MSSP module functions are enabled by setting
MSSP Enable bit, SSPEN (SSPCON<5>).
The SSPCON1 register allows control of the I2C
operation. Four mode selection bits (SSPCON<3:0>)
allow one of the following I2C modes to be selected:
• I2C Master mode, clock = (FOSC/4) x (SSPADD + 1)
• I2C Slave mode (7-bit address)
• I2C Slave mode (10-bit address)
• I2C Slave mode (7-bit address) with Start and
Stop bit interrupts enabled
• I2C Slave mode (10-bit address) with Start and
Stop bit interrupts enabled
• I2C Firmware Controlled Master mode, slave is
Idle
Selection of any I2C mode with the SSPEN bit set,
forces the SCL and SDA pins to be open-drain,
provided these pins are programmed to inputs by
setting the appropriate TRISC bits. To ensure proper
operation of the module, pull-up resistors must be
provided externally to the SCL and SDA pins.
17.4.3 SLAVE MODE
In Slave mode, the SCL and SDA pins must be configured
as inputs (TRISC<4:3> set). The MSSP module
will override the input state with the output data when
required (slave-transmitter).
The I2C Slave mode hardware will always generate an
interrupt on an address match. Through the mode
select bits, the user can also choose to interrupt on
Start and Stop bits
When an address is matched, or the data transfer after
an address match is received, the hardware automatically
will generate the Acknowledge (ACK) pulse and
load the SSPBUF register with the received value
currently in the SSPSR register.
Any combination of the following conditions will cause
the MSSP module not to give this ACK pulse:
• The Buffer Full bit, BF (SSPSTAT<0>), was set
before the transfer was received.
• The overflow bit, SSPOV (SSPCON<6>), was set
before the transfer was received.
In this case, the SSPSR register value is not loaded
into the SSPBUF, but bit SSPIF (PIR1<3>) is set. The
BF bit is cleared by reading the SSPBUF register, while
bit SSPOV is cleared through software.
The SCL clock input must have a minimum high and
low for proper operation. The high and low times of the
I2C specification, as well as the requirement of the
MSSP module, are shown in timing parameter 100 and
parameter 101.
17.4.3.1 Addressing
Once the MSSP module has been enabled, it waits for
a Start condition to occur. Following the Start condition,
the 8 bits are shifted into the SSPSR register. All
incoming bits are sampled with the rising edge of the
clock (SCL) line. The value of register SSPSR<7:1> is
compared to the value of the SSPADD register. The
address is compared on the falling edge of the eighth
clock (SCL) pulse. If the addresses match and the BF
and SSPOV bits are clear, the following events occur:
1. The SSPSR register value is loaded into the
SSPBUF register.
2. The Buffer Full bit, BF, is set.
3. An ACK pulse is generated.
4. MSSP Interrupt Flag bit, SSPIF (PIR1<3>), is
set (interrupt is generated, if enabled) on the
falling edge of the ninth SCL pulse.
In 10-bit Address mode, two address bytes need to be
received by the slave. The five Most Significant bits
(MSbs) of the first address byte specify if this is a 10-bit
address. Bit R/W (SSPSTAT<2>) must specify a write so
the slave device will receive the second address byte.
For a 10-bit address, the first byte would equal ‘11110
A9 A8 0’, where ‘A9’ and ‘A8’ are the two MSbs of the
address. The sequence of events for 10-bit address is as
follows, with steps 7 through 9 for the slave-transmitter:
1. Receive first (high) byte of address (bits SSPIF,
BF and UA (SSPSTAT<1>) are set).
2. Update the SSPADD register with second (low)
byte of address (clears bit UA and releases the
SCL line).
3. Read the SSPBUF register (clears bit BF) and
clear flag bit, SSPIF.
4. Receive second (low) byte of address (bits
SSPIF, BF and UA are set).
5. Update the SSPADD register with the first (high)
byte of address. If match releases SCL line, this
will clear bit UA.
6. Read the SSPBUF register (clears bit BF) and
clear flag bit, SSPIF.
7. Receive Repeated Start condition.
8. Receive first (high) byte of address (bits SSPIF
and BF are set).
9. Read the SSPBUF register (clears bit BF) and
clear flag bit, SSPIF.
© 2007 Microchip Technology Inc. Preliminary DS39631B-page 175
PIC18F2420/2520/4420/4520
17.4.3.2 Reception
When the R/W bit of the address byte is clear and an
address match occurs, the R/W bit of the SSPSTAT
register is cleared. The received address is loaded into
the SSPBUF register and the SDA line is held low
(ACK).
When the address byte overflow condition exists, then
the no Acknowledge (ACK) pulse is given. An overflow
condition is defined as either bit BF (SSPSTAT<0>) is
set, or bit SSPOV (SSPCON1<6>) is set.
An MSSP interrupt is generated for each data transfer
byte. Flag bit, SSPIF (PIR1<3>), must be cleared in
software. The SSPSTAT register is used to determine
the status of the byte.
If SEN is enabled (SSPCON2<0> = 1), RC3/SCK/SCL
will be held low (clock stretch) following each data
transfer. The clock must be released by setting bit,
CKP (SSPCON<4>). See Section 17.4.4 “Clock
Stretching” for more detail.
17.4.3.3 Transmission
When the R/W bit of the incoming address byte is set
and an address match occurs, the R/W bit of the
SSPSTAT register is set. The received address is
loaded into the SSPBUF register. The ACK pulse will
be sent on the ninth bit and pin RC3/SCK/SCL is held
low regardless of SEN (see Section 17.4.4 “Clock
Stretching” for more detail). By stretching the clock,
the master will be unable to assert another clock pulse
until the slave is done preparing the transmit data. The
transmit data must be loaded into the SSPBUF register
which also loads the SSPSR register. Then pin RC3/
SCK/SCL should be enabled by setting bit, CKP
(SSPCON1<4>). The eight data bits are shifted out on
the falling edge of the SCL input. This ensures that the
SDA signal is valid during the SCL high time
(Figure 17-9).
The ACK pulse from the master-receiver is latched on
the rising edge of the ninth SCL input pulse. If the SDA
line is high (not ACK), then the data transfer is complete.
In this case, when the ACK is latched by the
slave, the slave logic is reset (resets SSPSTAT register)
and the slave monitors for another occurrence of
the Start bit. If the SDA line was low (ACK), the next
transmit data must be loaded into the SSPBUF register.
Again, pin RC3/SCK/SCL must be enabled by setting
bit CKP.
An MSSP interrupt is generated for each data transfer
byte. The SSPIF bit must be cleared in software and
the SSPSTAT register is used to determine the status
of the byte. The SSPIF bit is set on the falling edge of
the ninth clock pulse.
PIC18F2420/2520/4420/4520
DS39631B-page 176 Preliminary © 2007 Microchip Technology Inc.
FIGURE 17-8: I2C™ SLAVE MODE TIMING WITH SEN = 0 (RECEPTION, 7-BIT ADDRESS)
SDA
SCL
SSPIF
BF (SSPSTAT<0>)
SSPOV (SSPCON1<6>)
S 1 2 3 4 5 6 7 8 9 1 2 3 4 5 6 7 8 9 1 2 3 4 5 7 8 9 P
A7 A6 A5 A4 A3 A2 A1 D7 D6 D5 D4 D3 D2 D1 D0 D7 D6 D5 D4 D3 D1 D0
R/W = 0 Receiving Data ACK Receiving Data ACK
ACK
Receiving Address
Cleared in software
SSPBUF is read
Bus master
terminates
transfer
SSPOV is set
because SSPBUF is
still full. ACK is not sent.
D2
6
(PIR1<3>)
CKP (CKP does not reset to ‘0’ when SEN = 0)
© 2007 Microchip Technology Inc. Preliminary DS39631B-page 177
PIC18F2420/2520/4420/4520
FIGURE 17-9: I2C™ SLAVE MODE TIMING (TRANSMISSION, 7-BIT ADDRESS)
SDA
SCL
SSPIF (PIR1<3>)
BF (SSPSTAT<0>)
A6 A5 A4 A3 A2 A1 D6 D5 D4 D3 D2 D1 D0
1 2 3 4 5 6 7 8 2 3 4 5 6 7 8 9
SSPBUF is written in software
Cleared in software
From SSPIF ISR
Data in
sampled
S
ACK
R/W = 0 Transmitting Data
ACK
Receiving Address
A7 D7
9 1
D6 D5 D4 D3 D2 D1 D0
2 3 4 5 6 7 8 9
SSPBUF is written in software
Cleared in software
From SSPIF ISR
Transmitting Data
D7
1
CKP
P
ACK
CKP is set in software CKP is set in software
SCL held low
while CPU
responds to SSPIF
PIC18F2420/2520/4420/4520
DS39631B-page 178 Preliminary © 2007 Microchip Technology Inc.
FIGURE 17-10: I2C™ SLAVE MODE TIMING WITH SEN = 0 (RECEPTION, 10-BIT ADDRESS)
SDA
SCL
SSPIF
BF (SSPSTAT<0>)
S 1 2 3 4 5 6 7 8 9 1 2 3 4 5 6 7 8 9 1 2 3 4 5 7 8 9 P
1 1 1 1 0 A9 A8 A7 A6 A5 A4 A3 A2 A1 A0 D7 D6 D5 D4 D3 D1 D0
Receive Data Byte
ACK
R/W = 0
ACK
Receive First Byte of Address
Cleared in software
D2
6
(PIR1<3>)
Cleared in software
Receive Second Byte of Address
Cleared by hardware
when SSPADD is updated
with low byte of address
UA (SSPSTAT<1>)
Clock is held low until
update of SSPADD has
taken place
UA is set indicating that
the SSPADD needs to be
updated
UA is set indicating that
SSPADD needs to be
updated
Cleared by hardware when
SSPADD is updated with high
byte of address
SSPBUF is written with
contents of SSPSR
Dummy read of SSPBUF
to clear BF flag
ACK
CKP
1 2 3 4 5 7 8 9
D7 D6 D5 D4 D3 D1 D0
Receive Data Byte
Bus master
terminates
transfer
D2
6
ACK
Cleared in software Cleared in software
SSPOV (SSPCON1<6>)
SSPOV is set
because SSPBUF is
still full. ACK is not sent.
(CKP does not reset to ‘0’ when SEN = 0)
Clock is held low until
update of SSPADD has
taken place
© 2007 Microchip Technology Inc. Preliminary DS39631B-page 179
PIC18F2420/2520/4420/4520
FIGURE 17-11: I2C™ SLAVE MODE TIMING (TRANSMISSION, 10-BIT ADDRESS)
SDA
SCL
SSPIF
BF (SSPSTAT<0>)
S 1 2 3 4 5 6 7 8 9 1 2 3 4 5 6 7 8 9 1 2 3 4 5 7 8 9 P
1 1 1 1 0 A9 A8 A7 A6 A5 A4 A3 A2 A1 A0 1 1 1 1 0 A8
R/W=1
ACK ACK
R/W = 0
ACK
Receive First Byte of Address
Cleared in software
Bus master
terminates
transfer
A9
6
(PIR1<3>)
Receive Second Byte of Address
Cleared by hardware when
SSPADD is updated with low
byte of address
UA (SSPSTAT<1>)
Clock is held low until
update of SSPADD has
taken place
UA is set indicating that
the SSPADD needs to be
updated
UA is set indicating that
SSPADD needs to be
updated
Cleared by hardware when
SSPADD is updated with high
byte of address.
SSPBUF is written with
contents of SSPSR
Dummy read of SSPBUF
to clear BF flag
Receive First Byte of Address
1 2 3 4 5 7 8 9
D7 D6 D5 D4 D3 D1
ACK
D2
6
Transmitting Data Byte
D0
Dummy read of SSPBUF
to clear BF flag
Sr
Cleared in software
Write of SSPBUF
initiates transmit
Cleared in software
Completion of
clears BF flag
CKP (SSPCON1<4>)
CKP is set in software
CKP is automatically cleared in hardware, holding SCL low
Clock is held low until
update of SSPADD has
taken place
data transmission
Clock is held low until
CKP is set to ‘1’
third address sequence
BF flag is clear
at the end of the
PIC18F2420/2520/4420/4520
DS39631B-page 180 Preliminary © 2007 Microchip Technology Inc.
17.4.4 CLOCK STRETCHING
Both 7-bit and 10-bit Slave modes implement
automatic clock stretching during a transmit sequence.
The SEN bit (SSPCON2<0>) allows clock stretching to
be enabled during receives. Setting SEN will cause
the SCL pin to be held low at the end of each data
receive sequence.
17.4.4.1 Clock Stretching for 7-bit Slave
Receive Mode (SEN = 1)
In 7-bit Slave Receive mode, on the falling edge of the
ninth clock at the end of the ACK sequence if the BF
bit is set, the CKP bit in the SSPCON1 register is
automatically cleared, forcing the SCL output to be
held low. The CKP being cleared to ‘0’ will assert the
SCL line low. The CKP bit must be set in the user’s
ISR before reception is allowed to continue. By holding
the SCL line low, the user has time to service the ISR
and read the contents of the SSPBUF before the
master device can initiate another receive sequence.
This will prevent buffer overruns from occurring (see
Figure 17-13).
17.4.4.2 Clock Stretching for 10-bit Slave
Receive Mode (SEN = 1)
In 10-bit Slave Receive mode during the address
sequence, clock stretching automatically takes place
but CKP is not cleared. During this time, if the UA bit is
set after the ninth clock, clock stretching is initiated.
The UA bit is set after receiving the upper byte of the
10-bit address and following the receive of the second
byte of the 10-bit address with the R/W bit cleared to
‘0’. The release of the clock line occurs upon updating
SSPADD. Clock stretching will occur on each data
receive sequence as described in 7-bit mode.
17.4.4.3 Clock Stretching for 7-bit Slave
Transmit Mode
7-bit Slave Transmit mode implements clock stretching
by clearing the CKP bit after the falling edge of the
ninth clock if the BF bit is clear. This occurs regardless
of the state of the SEN bit.
The user’s ISR must set the CKP bit before transmission
is allowed to continue. By holding the SCL line
low, the user has time to service the ISR and load the
contents of the SSPBUF before the master device can
initiate another transmit sequence (see Figure 17-9).
17.4.4.4 Clock Stretching for 10-bit Slave
Transmit Mode
In 10-bit Slave Transmit mode, clock stretching is controlled
during the first two address sequences by the
state of the UA bit, just as it is in 10-bit Slave Receive
mode. The first two addresses are followed by a third
address sequence which contains the high-order bits
of the 10-bit address and the R/W bit set to ‘1’. After
the third address sequence is performed, the UA bit is
not set, the module is now configured in Transmit
mode and clock stretching is controlled by the BF flag
as in 7-bit Slave Transmit mode (see Figure 17-11).
Note 1: If the user reads the contents of the
SSPBUF before the falling edge of the
ninth clock, thus clearing the BF bit, the
CKP bit will not be cleared and clock
stretching will not occur.
2: The CKP bit can be set in software
regardless of the state of the BF bit. The
user should be careful to clear the BF bit
in the ISR before the next receive
sequence in order to prevent an overflow
condition.
Note: If the user polls the UA bit and clears it by
updating the SSPADD register before the
falling edge of the ninth clock occurs and if
the user hasn’t cleared the BF bit by reading
the SSPBUF register before that time,
then the CKP bit will still NOT be asserted
low. Clock stretching on the basis of the
state of the BF bit only occurs during a
data sequence, not an address sequence.
Note 1: If the user loads the contents of SSPBUF,
setting the BF bit before the falling edge of
the ninth clock, the CKP bit will not be
cleared and clock stretching will not occur.
2: The CKP bit can be set in software
regardless of the state of the BF bit.
© 2007 Microchip Technology Inc. Preliminary DS39631B-page 181
PIC18F2420/2520/4420/4520
17.4.4.5 Clock Synchronization and
the CKP bit
When the CKP bit is cleared, the SCL output is forced
to ‘0’. However, clearing the CKP bit will not assert the
SCL output low until the SCL output is already sampled
low. Therefore, the CKP bit will not assert the
SCL line until an external I2C master device has
already asserted the SCL line. The SCL output will
remain low until the CKP bit is set and all other
devices on the I2C bus have deasserted SCL. This
ensures that a write to the CKP bit will not violate the
minimum high time requirement for SCL (see
Figure 17-12).
FIGURE 17-12: CLOCK SYNCHRONIZATION TIMING
SDA
SCL
DX DX – 1
WR
Q1 Q2 Q3 Q4 Q1 Q2 Q3 Q4 Q1 Q2 Q3 Q4 Q1 Q2 Q3 Q4 Q1 Q2 Q3 Q4 Q1 Q2 Q3 Q4 Q1 Q2 Q3 Q4
SSPCON
CKP
Master device
deasserts clock
Master device
asserts clock
PIC18F2420/2520/4420/4520
DS39631B-page 182 Preliminary © 2007 Microchip Technology Inc.
FIGURE 17-13: I2C™ SLAVE MODE TIMING WITH SEN = 1 (RECEPTION, 7-BIT ADDRESS)
SDA
SCL
SSPIF
BF (SSPSTAT<0>)
SSPOV (SSPCON1<6>)
S 1 2 3 4 5 6 7 8 9 1 2 3 4 5 6 7 8 9 1 2 3 4 5 7 8 9 P
A7 A6 A5 A4 A3 A2 A1 D7 D6 D5 D4 D3 D2 D1 D0 D7 D6 D5 D4 D3 D1 D0
R/W = 0 Receiving Data ACK Receiving Data ACK
ACK
Receiving Address
Cleared in software
SSPBUF is read
Bus master
terminates
transfer
SSPOV is set
because SSPBUF is
still full. ACK is not sent.
D2
6
(PIR1<3>)
CKP
CKP
written
to ‘1’ in
If BF is cleared
prior to the falling
edge of the 9th clock,
CKP will not be reset
to ‘0’ and no clock
stretching will occur
software
Clock is held low until
CKP is set to ‘1’
Clock is not held low
because buffer full bit is
clear prior to falling edge
of 9th clock
Clock is not held low
because ACK = 1
BF is set after falling
edge of the 9th clock,
CKP is reset to ‘0’ and
clock stretching occurs
© 2007 Microchip Technology Inc. Preliminary DS39631B-page 183
PIC18F2420/2520/4420/4520
FIGURE 17-14: I2C™ SLAVE MODE TIMING WITH SEN = 1 (RECEPTION, 10-BIT ADDRESS)
SDA
SCL
SSPIF
BF (SSPSTAT<0>)
S 1 2 3 4 5 6 7 8 9 1 2 3 4 5 6 7 8 9 1 2 3 4 5 7 8 9 P
1 1 1 1 0 A9 A8 A7 A6 A5 A4 A3 A2 A1 A0 D7 D6 D5 D4 D3 D1 D0
Receive Data Byte
ACK
R/W = 0
ACK
Receive First Byte of Address
Cleared in software
D2
6
(PIR1<3>)
Cleared in software
Receive Second Byte of Address
Cleared by hardware when
SSPADD is updated with low
byte of address after falling edge
UA (SSPSTAT<1>)
Clock is held low until
update of SSPADD has
taken place
UA is set indicating that
the SSPADD needs to be
updated
UA is set indicating that
SSPADD needs to be
updated
Cleared by hardware when
SSPADD is updated with high
byte of address after falling edge
SSPBUF is written with
contents of SSPSR
Dummy read of SSPBUF
to clear BF flag
ACK
CKP
1 2 3 4 5 7 8 9
D7 D6 D5 D4 D3 D1 D0
Receive Data Byte
Bus master
terminates
transfer
D2
6
ACK
Cleared in software Cleared in software
SSPOV (SSPCON1<6>)
CKP written to ‘1’
Note: An update of the SSPADD register before
the falling edge of the ninth clock will have
no effect on UA and UA will remain set.
Note: An update of the SSPADD
register before the falling
edge of the ninth clock will
have no effect on UA and
UA will remain set.
in software
Clock is held low until
update of SSPADD has
taken place
of ninth clock of ninth clock
SSPOV is set
because SSPBUF is
still full. ACK is not sent.
Dummy read of SSPBUF
to clear BF flag
Clock is held low until
CKP is set to ‘1’
Clock is not held low
because ACK = 1
PIC18F2420/2520/4420/4520
DS39631B-page 184 Preliminary © 2007 Microchip Technology Inc.
17.4.5 GENERAL CALL ADDRESS
SUPPORT
The addressing procedure for the I2C bus is such that
the first byte after the Start condition usually
determines which device will be the slave addressed by
the master. The exception is the general call address
which can address all devices. When this address is
used, all devices should, in theory, respond with an
Acknowledge.
The general call address is one of eight addresses
reserved for specific purposes by the I2C protocol. It
consists of all ‘0’s with R/W = 0.
The general call address is recognized when the General
Call Enable bit, GCEN, is enabled (SSPCON2<7>
is set). Following a Start bit detect, 8 bits are shifted into
the SSPSR and the address is compared against the
SSPADD. It is also compared to the general call
address and fixed in hardware.
If the general call address matches, the SSPSR is
transferred to the SSPBUF, the BF flag bit is set (eighth
bit) and on the falling edge of the ninth bit (ACK bit), the
SSPIF interrupt flag bit is set.
When the interrupt is serviced, the source for the
interrupt can be checked by reading the contents of the
SSPBUF. The value can be used to determine if the
address was device specific or a general call address.
In 10-bit mode, the SSPADD is required to be updated
for the second half of the address to match and the UA
bit is set (SSPSTAT<1>). If the general call address is
sampled when the GCEN bit is set, while the slave is
configured in 10-bit Address mode, then the second
half of the address is not necessary, the UA bit will not
be set and the slave will begin receiving data after the
Acknowledge (Figure 17-15).
FIGURE 17-15: SLAVE MODE GENERAL CALL ADDRESS SEQUENCE
(7 OR 10-BIT ADDRESS MODE)
SDA
SCL
S
SSPIF
BF (SSPSTAT<0>)
SSPOV (SSPCON1<6>)
Cleared in software
SSPBUF is read
R/W = 0
General Call Address ACK
Address is compared to General Call Address
GCEN (SSPCON2<7>)
Receiving Data ACK
1 2 3 4 5 6 7 8 9 1 2 3 4 5 6 7 8 9
D7 D6 D5 D4 D3 D2 D1 D0
after ACK, set interrupt
‘0’
‘1’
© 2007 Microchip Technology Inc. Preliminary DS39631B-page 185
PIC18F2420/2520/4420/4520
17.4.6 MASTER MODE
Master mode is enabled by setting and clearing the
appropriate SSPM bits in SSPCON1 and by setting the
SSPEN bit. In Master mode, the SCL and SDA lines
are manipulated by the MSSP hardware.
Master mode of operation is supported by interrupt
generation on the detection of the Start and Stop conditions.
The Stop (P) and Start (S) bits are cleared from
a Reset or when the MSSP module is disabled. Control
of the I2C bus may be taken when the P bit is set, or the
bus is Idle, with both the S and P bits clear.
In Firmware Controlled Master mode, user code
conducts all I2C bus operations based on Start and
Stop bit conditions.
Once Master mode is enabled, the user has six
options.
1. Assert a Start condition on SDA and SCL.
2. Assert a Repeated Start condition on SDA and
SCL.
3. Write to the SSPBUF register initiating
transmission of data/address.
4. Configure the I2C port to receive data.
5. Generate an Acknowledge condition at the end
of a received byte of data.
6. Generate a Stop condition on SDA and SCL.
The following events will cause the SSP Interrupt Flag
bit, SSPIF, to be set (SSP interrupt, if enabled):
• Start condition
• Stop condition
• Data transfer byte transmitted/received
• Acknowledge transmit
• Repeated Start
FIGURE 17-16: MSSP BLOCK DIAGRAM (I2C MASTER MODE)
Note: The MSSP module, when configured in
I2C Master mode, does not allow queueing
of events. For instance, the user is not
allowed to initiate a Start condition and
immediately write the SSPBUF register to
initiate transmission before the Start condition
is complete. In this case, the SSPBUF
will not be written to and the WCOL bit will
be set, indicating that a write to the
SSPBUF did not occur.
Read Write
SSPSR
Start bit, Stop bit,
SSPBUF
Internal
Data Bus
Set/Reset, S, P, WCOL (SSPSTAT)
Shift
Clock
MSb LSb
SDA
Acknowledge
Generate
Stop bit Detect
Write Collision Detect
Clock Arbitration
State Counter for
end of XMIT/RCV
SCL
SCL In
Bus Collision
SDA In
Receive Enable
Clock Cntl
Clock Arbitrate/WCOL Detect
(hold off clock source)
SSPADD<6:0>
Baud
Set SSPIF, BCLIF
Reset ACKSTAT, PEN (SSPCON2)
Rate
Generator
SSPM3:SSPM0
Start bit Detect
PIC18F2420/2520/4420/4520
DS39631B-page 186 Preliminary © 2007 Microchip Technology Inc.
17.4.6.1 I2C Master Mode Operation
The master device generates all of the serial clock
pulses and the Start and Stop conditions. A transfer is
ended with a Stop condition or with a Repeated Start
condition. Since the Repeated Start condition is also
the beginning of the next serial transfer, the I2C bus will
not be released.
In Master Transmitter mode, serial data is output
through SDA, while SCL outputs the serial clock. The
first byte transmitted contains the slave address of the
receiving device (7 bits) and the Read/Write (R/W) bit.
In this case, the R/W bit will be logic ‘0’. Serial data is
transmitted 8 bits at a time. After each byte is transmitted,
an Acknowledge bit is received. Start and Stop
conditions are output to indicate the beginning and the
end of a serial transfer.
In Master Receive mode, the first byte transmitted contains
the slave address of the transmitting device
(7 bits) and the R/W bit. In this case, the R/W bit will be
logic ‘1’. Thus, the first byte transmitted is a 7-bit slave
address followed by a ‘1’ to indicate the receive bit.
Serial data is received via SDA, while SCL outputs the
serial clock. Serial data is received 8 bits at a time. After
each byte is received, an Acknowledge bit is transmitted.
Start and Stop conditions indicate the beginning
and end of transmission.
The Baud Rate Generator used for the SPI mode
operation is used to set the SCL clock frequency for
either 100 kHz, 400 kHz or 1 MHz I2C operation. See
Section 17.4.7 “Baud Rate” for more detail.
A typical transmit sequence would go as follows:
1. The user generates a Start condition by setting
the Start Enable bit, SEN (SSPCON2<0>).
2. SSPIF is set. The MSSP module will wait the
required start time before any other operation
takes place.
3. The user loads the SSPBUF with the slave
address to transmit.
4. Address is shifted out the SDA pin until all 8 bits
are transmitted.
5. The MSSP module shifts in the ACK bit from the
slave device and writes its value into the
SSPCON2 register (SSPCON2<6>).
6. The MSSP module generates an interrupt at the
end of the ninth clock cycle by setting the SSPIF
bit.
7. The user loads the SSPBUF with eight bits of
data.
8. Data is shifted out the SDA pin until all 8 bits are
transmitted.
9. The MSSP module shifts in the ACK bit from the
slave device and writes its value into the
SSPCON2 register (SSPCON2<6>).
10. The MSSP module generates an interrupt at the
end of the ninth clock cycle by setting the SSPIF
bit.
11. The user generates a Stop condition by setting
the Stop Enable bit, PEN (SSPCON2<2>).
12. Interrupt is generated once the Stop condition is
complete.
© 2007 Microchip Technology Inc. Preliminary DS39631B-page 187
PIC18F2420/2520/4420/4520
17.4.7 BAUD RATE
In I2C Master mode, the Baud Rate Generator (BRG)
reload value is placed in the lower 7 bits of the
SSPADD register (Figure 17-17). When a write occurs
to SSPBUF, the Baud Rate Generator will automatically
begin counting. The BRG counts down to ‘0’ and stops
until another reload has taken place. The BRG count is
decremented twice per instruction cycle (TCY) on the
Q2 and Q4 clocks. In I2C Master mode, the BRG is
reloaded automatically.
Once the given operation is complete (i.e., transmission
of the last data bit is followed by ACK), the internal
clock will automatically stop counting and the SCL pin
will remain in its last state.
Table 17-3 demonstrates clock rates based on
instruction cycles and the BRG value loaded into
SSPADD.
FIGURE 17-17: BAUD RATE GENERATOR BLOCK DIAGRAM
TABLE 17-3: I2C CLOCK RATE W/BRG
SSPM3:SSPM0
CLKO BRG Down Counter FOSC/4
SSPADD<6:0>
SSPM3:SSPM0
SCL
Reload
Control
Reload
FCY FCY*2 BRG Value
FSCL
(2 Rollovers of BRG)
10 MHz 20 MHz 18h 400 kHz(1)
10 MHz 20 MHz 1Fh 312.5 kHz
10 MHz 20 MHz 63h 100 kHz
4 MHz 8 MHz 09h 400 kHz(1)
4 MHz 8 MHz 0Ch 308 kHz
4 MHz 8 MHz 27h 100 kHz
1 MHz 2 MHz 02h 333 kHz(1)
1 MHz 2 MHz 09h 100 kHz
1 MHz 2 MHz 00h 1 MHz(1)
Note 1: The I2C interface does not conform to the 400 kHz I2C specification (which applies to rates greater than
100 kHz) in all details, but may be used with care where higher rates are required by the application.
PIC18F2420/2520/4420/4520
DS39631B-page 188 Preliminary © 2007 Microchip Technology Inc.
17.4.7.1 Clock Arbitration
Clock arbitration occurs when the master, during any
receive, transmit or Repeated Start/Stop condition,
deasserts the SCL pin (SCL allowed to float high).
When the SCL pin is allowed to float high, the Baud
Rate Generator (BRG) is suspended from counting
until the SCL pin is actually sampled high. When the
SCL pin is sampled high, the Baud Rate Generator is
reloaded with the contents of SSPADD<6:0> and
begins counting. This ensures that the SCL high time
will always be at least one BRG rollover count in the
event that the clock is held low by an external device
(Figure 17-18).
FIGURE 17-18: BAUD RATE GENERATOR TIMING WITH CLOCK ARBITRATION
SDA
SCL
SCL deasserted but slave holds
DX DX – 1
BRG
SCL is sampled high, reload takes
place and BRG starts its count
03h 02h 01h 00h (hold off) 03h 02h
Reload
BRG
Value
SCL low (clock arbitration)
SCL allowed to transition high
BRG decrements on
Q2 and Q4 cycles
© 2007 Microchip Technology Inc. Preliminary DS39631B-page 189
PIC18F2420/2520/4420/4520
17.4.8 I2C MASTER MODE START
CONDITION TIMING
To initiate a Start condition, the user sets the Start
Enable bit, SEN (SSPCON2<0>). If the SDA and SCL
pins are sampled high, the Baud Rate Generator is
reloaded with the contents of SSPADD<6:0> and starts
its count. If SCL and SDA are both sampled high when
the Baud Rate Generator times out (TBRG), the SDA
pin is driven low. The action of the SDA being driven
low while SCL is high is the Start condition and causes
the S bit (SSPSTAT<3>) to be set. Following this, the
Baud Rate Generator is reloaded with the contents of
SSPADD<6:0> and resumes its count. When the Baud
Rate Generator times out (TBRG), the SEN bit
(SSPCON2<0>) will be automatically cleared by
hardware; the Baud Rate Generator is suspended,
leaving the SDA line held low and the Start condition is
complete.
17.4.8.1 WCOL Status Flag
If the user writes the SSPBUF when a Start sequence
is in progress, the WCOL is set and the contents of the
buffer are unchanged (the write doesn’t occur).
FIGURE 17-19: FIRST START BIT TIMING
Note: If at the beginning of the Start condition,
the SDA and SCL pins are already sampled
low, or if during the Start condition, the
SCL line is sampled low before the SDA
line is driven low, a bus collision occurs,
the Bus Collision Interrupt Flag, BCLIF, is
set, the Start condition is aborted and the
I2C module is reset into its Idle state.
Note: Because queueing of events is not
allowed, writing to the lower 5 bits of
SSPCON2 is disabled until the Start
condition is complete.
SDA
SCL
S
TBRG
1st bit 2nd bit
TBRG
SDA = 1,
SCL = At completion of Start bit, 1
TBRG Write to SSPBUF occurs here
hardware clears SEN bit
TBRG
Write to SEN bit occurs here
Set S bit (SSPSTAT<3>)
and sets SSPIF bit
PIC18F2420/2520/4420/4520
DS39631B-page 190 Preliminary © 2007 Microchip Technology Inc.
17.4.9 I2C MASTER MODE REPEATED
START CONDITION TIMING
A Repeated Start condition occurs when the RSEN bit
(SSPCON2<1>) is programmed high and the I2C logic
module is in the Idle state. When the RSEN bit is set,
the SCL pin is asserted low. When the SCL pin is sampled
low, the Baud Rate Generator is loaded with the
contents of SSPADD<5:0> and begins counting. The
SDA pin is released (brought high) for one Baud Rate
Generator count (TBRG). When the Baud Rate Generator
times out, if SDA is sampled high, the SCL pin will
be deasserted (brought high). When SCL is sampled
high, the Baud Rate Generator is reloaded with the
contents of SSPADD<6:0> and begins counting. SDA
and SCL must be sampled high for one TBRG. This
action is then followed by assertion of the SDA pin
(SDA = 0) for one TBRG while SCL is high. Following
this, the RSEN bit (SSPCON2<1>) will be automatically
cleared and the Baud Rate Generator will not be
reloaded, leaving the SDA pin held low. As soon as a
Start condition is detected on the SDA and SCL pins,
the S bit (SSPSTAT<3>) will be set. The SSPIF bit will
not be set until the Baud Rate Generator has timed out.
Immediately following the SSPIF bit getting set, the user
may write the SSPBUF with the 7-bit address in 7-bit
mode or the default first address in 10-bit mode. After the
first eight bits are transmitted and an ACK is received,
the user may then transmit an additional eight bits of
address (10-bit mode) or eight bits of data (7-bit mode).
17.4.9.1 WCOL Status Flag
If the user writes the SSPBUF when a Repeated Start
sequence is in progress, the WCOL is set and the
contents of the buffer are unchanged (the write doesn’t
occur).
FIGURE 17-20: REPEAT START CONDITION WAVEFORM
Note 1: If RSEN is programmed while any other
event is in progress, it will not take effect.
2: A bus collision during the Repeated Start
condition occurs if:
• SDA is sampled low when SCL goes
from low-to-high.
• SCL goes low before SDA is
asserted low. This may indicate that
another master is attempting to
transmit a data ‘1’.
Note: Because queueing of events is not
allowed, writing of the lower 5 bits of
SSPCON2 is disabled until the Repeated
Start condition is complete.
SDA
SCL
Sr = Repeated Start
Write to SSPCON2
on falling edge of ninth clock, Write to SSPBUF occurs here
end of Xmit
At completion of Start bit,
hardware clears RSEN bit
1st bit
S bit set by hardware
TBRG
TBRG
SDA = 1,
SDA = 1,
SCL (no change).
SCL = 1
occurs here.
TBRG TBRG TBRG
and sets SSPIF
RSEN bit set by hardware
© 2007 Microchip Technology Inc. Preliminary DS39631B-page 191
PIC18F2420/2520/4420/4520
17.4.10 I2C MASTER MODE
TRANSMISSION
Transmission of a data byte, a 7-bit address or the
other half of a 10-bit address is accomplished by simply
writing a value to the SSPBUF register. This action will
set the Buffer Full flag bit, BF and allow the Baud Rate
Generator to begin counting and start the next transmission.
Each bit of address/data will be shifted out
onto the SDA pin after the falling edge of SCL is
asserted (see data hold time specification
parameter 106). SCL is held low for one Baud Rate
Generator rollover count (TBRG). Data should be valid
before SCL is released high (see data setup time specification
parameter 107). When the SCL pin is released
high, it is held that way for TBRG. The data on the SDA
pin must remain stable for that duration and some hold
time after the next falling edge of SCL. After the eighth
bit is shifted out (the falling edge of the eighth clock),
the BF flag is cleared and the master releases SDA.
This allows the slave device being addressed to
respond with an ACK bit during the ninth bit time if an
address match occurred, or if data was received properly.
The status of ACK is written into the ACKDT bit on
the falling edge of the ninth clock. If the master receives
an Acknowledge, the Acknowledge Status bit,
ACKSTAT, is cleared. If not, the bit is set. After the ninth
clock, the SSPIF bit is set and the master clock (Baud
Rate Generator) is suspended until the next data byte
is loaded into the SSPBUF, leaving SCL low and SDA
unchanged (Figure 17-21).
After the write to the SSPBUF, each bit of the address
will be shifted out on the falling edge of SCL until all
seven address bits and the R/W bit are completed. On
the falling edge of the eighth clock, the master will
deassert the SDA pin, allowing the slave to respond
with an Acknowledge. On the falling edge of the ninth
clock, the master will sample the SDA pin to see if the
address was recognized by a slave. The status of the
ACK bit is loaded into the ACKSTAT status bit
(SSPCON2<6>). Following the falling edge of the ninth
clock transmission of the address, the SSPIF is set, the
BF flag is cleared and the Baud Rate Generator is
turned off until another write to the SSPBUF takes
place, holding SCL low and allowing SDA to float.
17.4.10.1 BF Status Flag
In Transmit mode, the BF bit (SSPSTAT<0>) is set
when the CPU writes to SSPBUF and is cleared when
all 8 bits are shifted out.
17.4.10.2 WCOL Status Flag
If the user writes the SSPBUF when a transmit is
already in progress (i.e., SSPSR is still shifting out a
data byte), the WCOL is set and the contents of the
buffer are unchanged (the write doesn’t occur).
WCOL must be cleared in software.
17.4.10.3 ACKSTAT Status Flag
In Transmit mode, the ACKSTAT bit (SSPCON2<6>) is
cleared when the slave has sent an Acknowledge
(ACK = 0) and is set when the slave does not Acknowledge
(ACK = 1). A slave sends an Acknowledge when
it has recognized its address (including a general call),
or when the slave has properly received its data.
17.4.11 I2C MASTER MODE RECEPTION
Master mode reception is enabled by programming the
Receive Enable bit, RCEN (SSPCON2<3>).
The Baud Rate Generator begins counting and on each
rollover, the state of the SCL pin changes (high-to-low/
low-to-high) and data is shifted into the SSPSR. After
the falling edge of the eighth clock, the receive enable
flag is automatically cleared, the contents of the
SSPSR are loaded into the SSPBUF, the BF flag bit is
set, the SSPIF flag bit is set and the Baud Rate Generator
is suspended from counting, holding SCL low. The
MSSP is now in Idle state awaiting the next command.
When the buffer is read by the CPU, the BF flag bit is
automatically cleared. The user can then send an
Acknowledge bit at the end of reception by setting the
Acknowledge Sequence Enable bit, ACKEN
(SSPCON2<4>).
17.4.11.1 BF Status Flag
In receive operation, the BF bit is set when an address
or data byte is loaded into SSPBUF from SSPSR. It is
cleared when the SSPBUF register is read.
17.4.11.2 SSPOV Status Flag
In receive operation, the SSPOV bit is set when 8 bits
are received into the SSPSR and the BF flag bit is
already set from a previous reception.
17.4.11.3 WCOL Status Flag
If the user writes the SSPBUF when a receive is
already in progress (i.e., SSPSR is still shifting in a data
byte), the WCOL bit is set and the contents of the buffer
are unchanged (the write doesn’t occur).
Note: The MSSP module must be in an Idle state
before the RCEN bit is set or the RCEN bit
will be disregarded.
PIC18F2420/2520/4420/4520
DS39631B-page 192 Preliminary © 2007 Microchip Technology Inc.
FIGURE 17-21: I2C MASTER MODE WAVEFORM (TRANSMISSION, 7 OR 10-BIT ADDRESS)
SDA
SCL
SSPIF
BF (SSPSTAT<0>)
SEN
A7 A6 A5 A4 A3 A2 A1 ACK = ‘0’ D7 D6 D5 D4 D3 D2 D1 D0
ACK
Transmitting Data or Second Half
Transmit Address to Slave R/W = 0
1 2 3 4 5 6 7 8 9 1 2 3 4 5 6 7 8 9 P
Cleared in software service routine
SSPBUF is written in software
from SSP interrupt
After Start condition, SEN cleared by hardware
S
SSPBUF written with 7-bit address and R/W
start transmit
SCL held low
while CPU
responds to SSPIF
SEN = 0
of 10-bit Address
Write SSPCON2<0> SEN = 1
Start condition begins
From slave, clear ACKSTAT bit SSPCON2<6>
ACKSTAT in
SSPCON2 = 1
Cleared in software
SSPBUF written
PEN
R/W
Cleared in software
© 2007 Microchip Technology Inc. Preliminary DS39631B-page 193
PIC18F2420/2520/4420/4520
FIGURE 17-22: I2C MASTER MODE WAVEFORM (RECEPTION, 7-BIT ADDRESS)
P
5 6 7 8 9
D7 D6 D5 D4 D3 D2 D1 D0
S
SDA A7 A6 A5 A4 A3 A2 A1
SCL 1 2 3 4 5 6 7 8 9 1 2 3 4 5 6 7 8 9 1 2 3 4
Bus master
terminates
transfer
ACK
Receiving Data from Slave Receiving Data from Slave
ACK D7 D6 D5 D4 D3 D2 D1 D0
Transmit Address to Slave R/W = 0
SSPIF
BF
ACK is not sent
Write to SSPCON2<0> (SEN = 1),
Write to SSPBUF occurs here,
ACK from Slave
Master configured as a receiver
by programming SSPCON2<3> (RCEN = 1)
PEN bit = 1
written here
Data shifted in on falling edge of CLK
Cleared in software
start XMIT
SEN = 0
SSPOV
SDA = 0, SCL = 1
while CPU
(SSPSTAT<0>)
ACK
Cleared in software Cleared in software
Set SSPIF interrupt
at end of receive
Set P bit
(SSPSTAT<4>)
and SSPIF
Cleared in
software
ACK from Master
Set SSPIF at end
Set SSPIF interrupt
at end of Acknowledge
sequence
Set SSPIF interrupt
at end of Acknowledge
sequence
of receive
Set ACKEN, start Acknowledge sequence
SSPOV is set because
SSPBUF is still full
SDA = ACKDT = 1
RCEN cleared
automatically
RCEN = 1, start
next receive
Write to SSPCON2<4>
to start Acknowledge sequence
SDA = ACKDT (SSPCON2<5>) = 0
RCEN cleared
automatically
responds to SSPIF
ACKEN
begin Start condition
Cleared in software
SDA = ACKDT = 0
Last bit is shifted into SSPSR and
contents are unloaded into SSPBUF
PIC18F2420/2520/4420/4520
DS39631B-page 194 Preliminary © 2007 Microchip Technology Inc.
17.4.12 ACKNOWLEDGE SEQUENCE
TIMING
An Acknowledge sequence is enabled by setting the
Acknowledge Sequence Enable bit, ACKEN
(SSPCON2<4>). When this bit is set, the SCL pin is
pulled low and the contents of the Acknowledge data bit
are presented on the SDA pin. If the user wishes to generate
an Acknowledge, then the ACKDT bit should be
cleared. If not, the user should set the ACKDT bit before
starting an Acknowledge sequence. The Baud Rate
Generator then counts for one rollover period (TBRG)
and the SCL pin is deasserted (pulled high). When the
SCL pin is sampled high (clock arbitration), the Baud
Rate Generator counts for TBRG. The SCL pin is then
pulled low. Following this, the ACKEN bit is automatically
cleared, the Baud Rate Generator is turned off and the
MSSP module then goes into Idle mode (Figure 17-23).
17.4.12.1 WCOL Status Flag
If the user writes the SSPBUF when an Acknowledge
sequence is in progress, then WCOL is set and the
contents of the buffer are unchanged (the write doesn’t
occur).
17.4.13 STOP CONDITION TIMING
A Stop bit is asserted on the SDA pin at the end of a
receive/transmit by setting the Stop Sequence Enable
bit, PEN (SSPCON2<2>). At the end of a receive/
transmit, the SCL line is held low after the falling edge
of the ninth clock. When the PEN bit is set, the master
will assert the SDA line low. When the SDA line is sampled
low, the Baud Rate Generator is reloaded and
counts down to ‘0’. When the Baud Rate Generator
times out, the SCL pin will be brought high and one
TBRG (Baud Rate Generator rollover count) later, the
SDA pin will be deasserted. When the SDA pin is sampled
high while SCL is high, the P bit (SSPSTAT<4>) is
set. A TBRG later, the PEN bit is cleared and the SSPIF
bit is set (Figure 17-24).
17.4.13.1 WCOL Status Flag
If the user writes the SSPBUF when a Stop sequence
is in progress, then the WCOL bit is set and the contents
of the buffer are unchanged (the write doesn’t
occur).
FIGURE 17-23: ACKNOWLEDGE SEQUENCE WAVEFORM
FIGURE 17-24: STOP CONDITION RECEIVE OR TRANSMIT MODE
Note: TBRG = one Baud Rate Generator period.
SDA
SCL
SSPIF set at
Acknowledge sequence starts here,
write to SSPCON2
ACKEN automatically cleared
Cleared in
TBRG TBRG
the end of receive
8
ACKEN = 1, ACKDT = 0
D0
9
SSPIF
software SSPIF set at the end
of Acknowledge sequence
Cleared in
software
ACK
SCL
SDA
SDA asserted low before rising edge of clock
Write to SSPCON2,
set PEN
Falling edge of
SCL = 1 for TBRG, followed by SDA = 1 for TBRG
9th clock
SCL brought high after TBRG
Note: TBRG = one Baud Rate Generator period.
TBRG TBRG
after SDA sampled high. P bit (SSPSTAT<4>) is set.
TBRG
to setup Stop condition
ACK
P
TBRG
PEN bit (SSPCON2<2>) is cleared by
hardware and the SSPIF bit is set
© 2007 Microchip Technology Inc. Preliminary DS39631B-page 195
PIC18F2420/2520/4420/4520
17.4.14 SLEEP OPERATION
While in Sleep mode, the I2C module can receive
addresses or data and when an address match or complete
byte transfer occurs, wake the processor from
Sleep (if the MSSP interrupt is enabled).
17.4.15 EFFECTS OF A RESET
A Reset disables the MSSP module and terminates the
current transfer.
17.4.16 MULTI-MASTER MODE
In Multi-Master mode, the interrupt generation on the
detection of the Start and Stop conditions allows the
determination of when the bus is free. The Stop (P) and
Start (S) bits are cleared from a Reset or when the
MSSP module is disabled. Control of the I2C bus may
be taken when the P bit (SSPSTAT<4>) is set, or the
bus is Idle, with both the S and P bits clear. When the
bus is busy, enabling the SSP interrupt will generate
the interrupt when the Stop condition occurs.
In multi-master operation, the SDA line must be
monitored for arbitration to see if the signal level is the
expected output level. This check is performed in
hardware with the result placed in the BCLIF bit.
The states where arbitration can be lost are:
• Address Transfer
• Data Transfer
• A Start Condition
• A Repeated Start Condition
• An Acknowledge Condition
17.4.17 MULTI -MASTER COMMUNICATION,
BUS COLLISION AND BUS
ARBITRATION
Multi-Master mode support is achieved by bus arbitration.
When the master outputs address/data bits onto
the SDA pin, arbitration takes place when the master
outputs a ‘1’ on SDA, by letting SDA float high and
another master asserts a ‘0’. When the SCL pin floats
high, data should be stable. If the expected data on
SDA is a ‘1’ and the data sampled on the SDA pin = 0,
then a bus collision has taken place. The master will set
the Bus Collision Interrupt Flag, BCLIF and reset the
I2C port to its Idle state (Figure 17-25).
If a transmit was in progress when the bus collision
occurred, the transmission is halted, the BF flag is
cleared, the SDA and SCL lines are deasserted and the
SSPBUF can be written to. When the user services the
bus collision Interrupt Service Routine and if the I2C
bus is free, the user can resume communication by
asserting a Start condition.
If a Start, Repeated Start, Stop or Acknowledge condition
was in progress when the bus collision occurred, the
condition is aborted, the SDA and SCL lines are deasserted
and the respective control bits in the SSPCON2
register are cleared. When the user services the bus collision
Interrupt Service Routine and if the I2C bus is free,
the user can resume communication by asserting a Start
condition.
The master will continue to monitor the SDA and SCL
pins. If a Stop condition occurs, the SSPIF bit will be set.
A write to the SSPBUF will start the transmission of
data at the first data bit, regardless of where the
transmitter left off when the bus collision occurred.
In Multi-Master mode, the interrupt generation on the
detection of Start and Stop conditions allows the determination
of when the bus is free. Control of the I2C bus
can be taken when the P bit is set in the SSPSTAT
register, or the bus is Idle and the S and P bits are
cleared.
FIGURE 17-25: BUS COLLISION TIMING FOR TRANSMIT AND ACKNOWLEDGE
SDA
SCL
BCLIF
SDA released
SDA line pulled low
by another source
Sample SDA. While SCL is high,
data doesn’t match what is driven
Bus collision has occurred.
Set bus collision
interrupt (BCLIF)
by the master.
by master
Data changes
while SCL = 0
PIC18F2420/2520/4420/4520
DS39631B-page 196 Preliminary © 2007 Microchip Technology Inc.
17.4.17.1 Bus Collision During a Start
Condition
During a Start condition, a bus collision occurs if:
a) SDA or SCL are sampled low at the beginning of
the Start condition (Figure 17-26).
b) SCL is sampled low before SDA is asserted low
(Figure 17-27).
During a Start condition, both the SDA and the SCL
pins are monitored.
If the SDA pin is already low, or the SCL pin is already
low, then all of the following occur:
• the Start condition is aborted,
• the BCLIF flag is set and
• the MSSP module is reset to its Idle state
(Figure 17-26).
The Start condition begins with the SDA and SCL pins
deasserted. When the SDA pin is sampled high, the
Baud Rate Generator is loaded from SSPADD<6:0>
and counts down to 0. If the SCL pin is sampled low
while SDA is high, a bus collision occurs because it is
assumed that another master is attempting to drive a
data ‘1’ during the Start condition.
If the SDA pin is sampled low during this count, the
BRG is reset and the SDA line is asserted early
(Figure 17-28). If, however, a ‘1’ is sampled on the SDA
pin, the SDA pin is asserted low at the end of the BRG
count. The Baud Rate Generator is then reloaded and
counts down to 0; if the SCL pin is sampled as ‘0’
during this time, a bus collision does not occur. At the
end of the BRG count, the SCL pin is asserted low.
FIGURE 17-26: BUS COLLISION DURING START CONDITION (SDA ONLY)
Note: The reason that bus collision is not a factor
during a Start condition is that no two bus
masters can assert a Start condition at the
exact same time. Therefore, one master
will always assert SDA before the other.
This condition does not cause a bus collision
because the two masters must be
allowed to arbitrate the first address following
the Start condition. If the address is
the same, arbitration must be allowed to
continue into the data portion, Repeated
Start or Stop conditions.
SDA
SCL
SEN
SDA sampled low before
SDA goes low before the SEN bit is set.
S bit and SSPIF set because
SSP module reset into Idle state.
SEN cleared automatically because of bus collision.
S bit and SSPIF set because
Set SEN, enable Start
condition if SDA = 1, SCL = 1
SDA = 0, SCL = 1.
BCLIF
S
SSPIF
SDA = 0, SCL = 1.
SSPIF and BCLIF are
cleared in software
SSPIF and BCLIF are
cleared in software
Set BCLIF,
Start condition. Set BCLIF.
© 2007 Microchip Technology Inc. Preliminary DS39631B-page 197
PIC18F2420/2520/4420/4520
FIGURE 17-27: BUS COLLISION DURING START CONDITION (SCL = 0)
FIGURE 17-28: BRG RESET DUE TO SDA ARBITRATION DURING START CONDITION
SDA
SCL
SEN
bus collision occurs. Set BCLIF.
SCL = 0 before SDA = 0,
Set SEN, enable Start
sequence if SDA = 1, SCL = 1
TBRG TBRG
SDA = 0, SCL = 1
BCLIF
S
SSPIF
Interrupt cleared
in software
bus collision occurs. Set BCLIF.
SCL = 0 before BRG time-out,
‘0’ ‘0’
‘0’ ‘0’
SDA
SCL
SEN
Set S
Less than TBRG
TBRG
SDA = 0, SCL = 1
BCLIF
S
SSPIF
S
Interrupts cleared
set SSPIF in software
SDA = 0, SCL = 1,
SCL pulled low after BRG
time-out
Set SSPIF
‘0’
SDA pulled low by other master.
Reset BRG and assert SDA.
Set SEN, enable START
sequence if SDA = 1, SCL = 1
PIC18F2420/2520/4420/4520
DS39631B-page 198 Preliminary © 2007 Microchip Technology Inc.
17.4.17.2 Bus Collision During a Repeated
Start Condition
During a Repeated Start condition, a bus collision
occurs if:
a) A low level is sampled on SDA when SCL goes
from low level to high level.
b) SCL goes low before SDA is asserted low,
indicating that another master is attempting to
transmit a data ‘1’.
When the user deasserts SDA and the pin is allowed to
float high, the BRG is loaded with SSPADD<6:0> and
counts down to 0. The SCL pin is then deasserted and
when sampled high, the SDA pin is sampled.
If SDA is low, a bus collision has occurred (i.e., another
master is attempting to transmit a data ‘0’, Figure 17-29).
If SDA is sampled high, the BRG is reloaded and begins
counting. If SDA goes from high-to-low before the BRG
times out, no bus collision occurs because no two
masters can assert SDA at exactly the same time.
If SCL goes from high-to-low before the BRG times out
and SDA has not already been asserted, a bus collision
occurs. In this case, another master is attempting to
transmit a data ‘1’ during the Repeated Start condition,
see Figure 17-30.
If, at the end of the BRG time-out, both SCL and SDA
are still high, the SDA pin is driven low and the BRG is
reloaded and begins counting. At the end of the count,
regardless of the status of the SCL pin, the SCL pin is
driven low and the Repeated Start condition is
complete.
FIGURE 17-29: BUS COLLISION DURING A REPEATED START CONDITION (CASE 1)
FIGURE 17-30: BUS COLLISION DURING REPEATED START CONDITION (CASE 2)
SDA
SCL
RSEN
BCLIF
S
SSPIF
Sample SDA when SCL goes high.
If SDA = 0, set BCLIF and release SDA and SCL.
Cleared in software
‘0’
‘0’
SDA
SCL
BCLIF
RSEN
S
SSPIF
Interrupt cleared
in software
SCL goes low before SDA,
set BCLIF. Release SDA and SCL.
TBRG TBRG
‘0’
© 2007 Microchip Technology Inc. Preliminary DS39631B-page 199
PIC18F2420/2520/4420/4520
17.4.17.3 Bus Collision During a Stop
Condition
Bus collision occurs during a Stop condition if:
a) After the SDA pin has been deasserted and
allowed to float high, SDA is sampled low after
the BRG has timed out.
b) After the SCL pin is deasserted, SCL is sampled
low before SDA goes high.
The Stop condition begins with SDA asserted low.
When SDA is sampled low, the SCL pin is allowed to
float. When the pin is sampled high (clock arbitration),
the Baud Rate Generator is loaded with SSPADD<6:0>
and counts down to 0. After the BRG times out, SDA is
sampled. If SDA is sampled low, a bus collision has
occurred. This is due to another master attempting to
drive a data ‘0’ (Figure 17-31). If the SCL pin is
sampled low before SDA is allowed to float high, a bus
collision occurs. This is another case of another master
attempting to drive a data ‘0’ (Figure 17-32).
FIGURE 17-31: BUS COLLISION DURING A STOP CONDITION (CASE 1)
FIGURE 17-32: BUS COLLISION DURING A STOP CONDITION (CASE 2)
SDA
SCL
BCLIF
PEN
P
SSPIF
TBRG TBRG TBRG
SDA asserted low
SDA sampled
low after TBRG,
set BCLIF
‘0’
‘0’
SDA
SCL
BCLIF
PEN
P
SSPIF
TBRG TBRG TBRG
Assert SDA SCL goes low before SDA goes high,
set BCLIF
‘0’
‘0’
PIC18F2420/2520/4420/4520
DS39631B-page 200 Preliminary © 2007 Microchip Technology Inc.
NOTES:
© 2007 Microchip Technology Inc. Preliminary DS39631B-page 201
PIC18F2420/2520/4420/4520
18.0 ENHANCED UNIVERSAL
SYNCHRONOUS RECEIVER
TRANSMITTER (EUSART)
The Enhanced Universal Synchronous Asynchronous
Receiver Transmitter (EUSART) module is one of the
two serial I/O modules. (Generically, the USART is also
known as a Serial Communications Interface or SCI.)
The EUSART can be configured as a full-duplex
asynchronous system that can communicate with
peripheral devices, such as CRT terminals and
personal computers. It can also be configured as a halfduplex
synchronous system that can communicate
with peripheral devices, such as A/D or D/A integrated
circuits, serial EEPROMs, etc.
The Enhanced USART module implements additional
features, including automatic baud rate detection and
calibration, automatic wake-up on Sync Break reception
and 12-bit Break character transmit. These make it
ideally suited for use in Local Interconnect Network bus
(LIN bus) systems.
The EUSART can be configured in the following
modes:
• Asynchronous (full duplex) with:
- Auto-wake-up on character reception
- Auto-baud calibration
- 12-bit Break character transmission
• Synchronous – Master (half duplex) with
selectable clock polarity
• Synchronous – Slave (half duplex) with selectable
clock polarity
The pins of the Enhanced USART are multiplexed
with PORTC. In order to configure RC6/TX/CK and
RC7/RX/DT as a USART:
• bit SPEN (RCSTA<7>) must be set (= 1)
• bit TRISC<7> must be set (= 1)
• bit TRISC<6> must be set (= 1)
The operation of the Enhanced USART module is
controlled through three registers:
• Transmit Status and Control (TXSTA)
• Receive Status and Control (RCSTA)
• Baud Rate Control (BAUDCON)
These are detailed on the following pages in
Register 18-1, Register 18-2 and Register 18-3,
respectively.
Note: The EUSART control will automatically
reconfigure the pin from input to output as
needed.
PIC18F2420/2520/4420/4520
DS39631B-page 202 Preliminary © 2007 Microchip Technology Inc.
REGISTER 18-1: TXSTA: TRANSMIT STATUS AND CONTROL REGISTER
R/W-0 R/W-0 R/W-0 R/W-0 R/W-0 R/W-0 R-1 R/W-0
CSRC TX9 TXEN SYNC SENDB BRGH TRMT TX9D
bit 7 bit 0
bit 7 CSRC: Clock Source Select bit
Asynchronous mode:
Don’t care.
Synchronous mode:
1 = Master mode (clock generated internally from BRG)
0 = Slave mode (clock from external source)
bit 6 TX9: 9-bit Transmit Enable bit
1 = Selects 9-bit transmission
0 = Selects 8-bit transmission
bit 5 TXEN: Transmit Enable bit
1 = Transmit enabled
0 = Transmit disabled
Note: SREN/CREN overrides TXEN in Sync mode.
bit 4 SYNC: EUSART Mode Select bit
1 = Synchronous mode
0 = Asynchronous mode
bit 3 SENDB: Send Break Character bit
Asynchronous mode:
1 = Send Sync Break on next transmission (cleared by hardware upon completion)
0 = Sync Break transmission completed
Synchronous mode:
Don’t care.
bit 2 BRGH: High Baud Rate Select bit
Asynchronous mode:
1 = High speed
0 = Low speed
Synchronous mode:
Unused in this mode.
bit 1 TRMT: Transmit Shift Register Status bit
1 = TSR empty
0 = TSR full
bit 0 TX9D: 9th bit of Transmit Data
Can be address/data bit or a parity bit.
Legend:
R = Readable bit W = Writable bit U = Unimplemented bit, read as ‘0’
-n = Value at POR ‘1’ = Bit is set ‘0’ = Bit is cleared x = Bit is unknown
© 2007 Microchip Technology Inc. Preliminary DS39631B-page 203
PIC18F2420/2520/4420/4520
REGISTER 18-2: RCSTA: RECEIVE STATUS AND CONTROL REGISTER
R/W-0 R/W-0 R/W-0 R/W-0 R/W-0 R-0 R-0 R-x
SPEN RX9 SREN CREN ADDEN FERR OERR RX9D
bit 7 bit 0
bit 7 SPEN: Serial Port Enable bit
1 = Serial port enabled (configures RX/DT and TX/CK pins as serial port pins)
0 = Serial port disabled (held in Reset)
bit 6 RX9: 9-bit Receive Enable bit
1 = Selects 9-bit reception
0 = Selects 8-bit reception
bit 5 SREN: Single Receive Enable bit
Asynchronous mode:
Don’t care.
Synchronous mode – Master:
1 = Enables single receive
0 = Disables single receive
This bit is cleared after reception is complete.
Synchronous mode – Slave:
Don’t care.
bit 4 CREN: Continuous Receive Enable bit
Asynchronous mode:
1 = Enables receiver
0 = Disables receiver
Synchronous mode:
1 = Enables continuous receive until enable bit CREN is cleared (CREN overrides SREN)
0 = Disables continuous receive
bit 3 ADDEN: Address Detect Enable bit
Asynchronous mode 9-bit (RX9 = 1):
1 = Enables address detection, enables interrupt and loads the receive buffer when RSR<8>
is set
0 = Disables address detection, all bytes are received and ninth bit can be used as parity bit
Asynchronous mode 9-bit (RX9 = 0):
Don’t care.
bit 2 FERR: Framing Error bit
1 = Framing error (can be updated by reading RCREG register and receiving next valid byte)
0 = No framing error
bit 1 OERR: Overrun Error bit
1 = Overrun error (can be cleared by clearing bit CREN)
0 = No overrun error
bit 0 RX9D: 9th bit of Received Data
This can be address/data bit or a parity bit and must be calculated by user firmware.
Legend:
R = Readable bit W = Writable bit U = Unimplemented bit, read as ‘0’
-n = Value at POR ‘1’ = Bit is set ‘0’ = Bit is cleared x = Bit is unknown
PIC18F2420/2520/4420/4520
DS39631B-page 204 Preliminary © 2007 Microchip Technology Inc.
REGISTER 18-3: BAUDCON: BAUD RATE CONTROL REGISTER
R/W-0 R-1 U-0 R/W-0 R/W-0 U-0 R/W-0 R/W-0
ABDOVF RCIDL — SCKP BRG16 — WUE ABDEN
bit 7 bit 0
bit 7 ABDOVF: Auto-Baud Acquisition Rollover Status bit
1 = A BRG rollover has occurred during Auto-Baud Rate Detect mode
(must be cleared in software)
0 = No BRG rollover has occurred
bit 6 RCIDL: Receive Operation Idle Status bit
1 = Receive operation is Idle
0 = Receive operation is active
bit 5 Unimplemented: Read as ‘0’
bit 4 SCKP: Synchronous Clock Polarity Select bit
Asynchronous mode:
Unused in this mode.
Synchronous mode:
1 = Idle state for clock (CK) is a high level
0 = Idle state for clock (CK) is a low level
bit 3 BRG16: 16-bit Baud Rate Register Enable bit
1 = 16-bit Baud Rate Generator – SPBRGH and SPBRG
0 = 8-bit Baud Rate Generator – SPBRG only (Compatible mode), SPBRGH value ignored
bit 2 Unimplemented: Read as ‘0’
bit 1 WUE: Wake-up Enable bit
Asynchronous mode:
1 = EUSART will continue to sample the RX pin – interrupt generated on falling edge; bit
cleared in hardware on following rising edge
0 = RX pin not monitored or rising edge detected
Synchronous mode:
Unused in this mode.
bit 0 ABDEN: Auto-Baud Detect Enable bit
Asynchronous mode:
1 = Enable baud rate measurement on the next character. Requires reception of a Sync field
(55h); cleared in hardware upon completion
0 = Baud rate measurement disabled or completed
Synchronous mode:
Unused in this mode.
Legend:
R = Readable bit W = Writable bit U = Unimplemented bit, read as ‘0’
-n = Value at POR ‘1’ = Bit is set ‘0’ = Bit is cleared x = Bit is unknown
© 2007 Microchip Technology Inc. Preliminary DS39631B-page 205
PIC18F2420/2520/4420/4520
18.1 Baud Rate Generator (BRG)
The BRG is a dedicated 8-bit or 16-bit generator that
supports both the Asynchronous and Synchronous
modes of the EUSART. By default, the BRG operates
in 8-bit mode; setting the BRG16 bit (BAUDCON<3>)
selects 16-bit mode.
The SPBRGH:SPBRG register pair controls the period
of a free running timer. In Asynchronous mode, bits
BRGH (TXSTA<2>) and BRG16 (BAUDCON<3>) also
control the baud rate. In Synchronous mode, BRGH is
ignored. Table 18-1 shows the formula for computation
of the baud rate for different EUSART modes which
only apply in Master mode (internally generated clock).
Given the desired baud rate and FOSC, the nearest
integer value for the SPBRGH:SPBRG registers can be
calculated using the formulas in Table 18-1. From this,
the error in baud rate can be determined. An example
calculation is shown in Example 18-1. Typical baud
rates and error values for the various Asynchronous
modes are shown in Table 18-2. It may be advantageous
to use the high baud rate (BRGH = 1) or the
16-bit BRG to reduce the baud rate error, or achieve a
slow baud rate for a fast oscillator frequency.
Writing a new value to the SPBRGH:SPBRG registers
causes the BRG timer to be reset (or cleared). This
ensures the BRG does not wait for a timer overflow
before outputting the new baud rate.
18.1.1 OPERATION IN POWER MANAGED
MODES
The device clock is used to generate the desired baud
rate. When one of the power managed modes is
entered, the new clock source may be operating at a
different frequency. This may require an adjustment to
the value in the SPBRG register pair.
18.1.2 SAMPLING
The data on the RX pin is sampled three times by a
majority detect circuit to determine if a high or a low
level is present at the RX pin.
TABLE 18-1: BAUD RATE FORMULAS
Configuration Bits
BRG/EUSART Mode Baud Rate Formula
SYNC BRG16 BRGH
0 0 0 8-bit/Asynchronous FOSC/[64 (n + 1)]
0 0 1 8-bit/Asynchronous
FOSC/[16 (n + 1)]
0 1 0 16-bit/Asynchronous
0 1 1 16-bit/Asynchronous
1 0 x 8-bit/Synchronous FOSC/[4 (n + 1)]
1 1 x 16-bit/Synchronous
Legend: x = Don’t care, n = value of SPBRGH:SPBRG register pair
PIC18F2420/2520/4420/4520
DS39631B-page 206 Preliminary © 2007 Microchip Technology Inc.
EXAMPLE 18-1: CALCULATING BAUD RATE ERROR
TABLE 18-2: REGISTERS ASSOCIATED WITH BAUD RATE GENERATOR
For a device with FOSC of 16 MHz, desired baud rate of 9600, Asynchronous mode, 8-bit BRG:
Desired Baud Rate = FOSC/(64 ([SPBRGH:SPBRG] + 1))
Solving for SPBRGH:SPBRG:
X = ((FOSC/Desired Baud Rate)/64) – 1
= ((16000000/9600)/64) – 1
= [25.042] = 25
Calculated Baud Rate = 16000000/(64 (25 + 1))
= 9615
Error = (Calculated Baud Rate – Desired Baud Rate)/Desired Baud Rate
= (9615 – 9600)/9600 = 0.16%
Name Bit 7 Bit 6 Bit 5 Bit 4 Bit 3 Bit 2 Bit 1 Bit 0
Reset Values
on page
TXSTA CSRC TX9 TXEN SYNC SENDB BRGH TRMT TX9D 51
RCSTA SPEN RX9 SREN CREN ADDEN FERR OERR RX9D 51
BAUDCON ABDOVF RCIDL — SCKP BRG16 — WUE ABDEN 51
SPBRGH EUSART Baud Rate Generator Register, High Byte 51
SPBRG EUSART Baud Rate Generator Register, Low Byte 51
Legend: — = unimplemented, read as ‘0’. Shaded cells are not used by the BRG.
© 2007 Microchip Technology Inc. Preliminary DS39631B-page 207
PIC18F2420/2520/4420/4520
TABLE 18-3: BAUD RATES FOR ASYNCHRONOUS MODES
BAUD
RATE
(K)
SYNC = 0, BRGH = 0, BRG16 = 0
FOSC = 40.000 MHz FOSC = 20.000 MHz FOSC = 10.000 MHz FOSC = 8.000 MHz
Actual
Rate
(K)
%
Error
SPBRG
value
(decimal)
Actual
Rate
(K)
%
Error
SPBRG
value
(decimal)
Actual
Rate
(K)
%
Error
SPBRG
value
(decimal)
Actual
Rate
(K)
%
Error
SPBRG
value
(decimal)
0.3 — — — — — — — — — — — —
1.2 — — — 1.221 1.73 255 1.202 0.16 129 1201 -0.16 103
2.4 2.441 1.73 255 2.404 0.16 129 2.404 0.16 64 2403 -0.16 51
9.6 9.615 0.16 64 9.766 1.73 31 9.766 1.73 15 9615 -0.16 12
19.2 19.531 1.73 31 19.531 1.73 15 19.531 1.73 7 — — —
57.6 56.818 -1.36 10 62.500 8.51 4 52.083 -9.58 2 — — —
115.2 125.000 8.51 4 104.167 -9.58 2 78.125 -32.18 1 — — —
BAUD
RATE
(K)
SYNC = 0, BRGH = 0, BRG16 = 0
FOSC = 4.000 MHz FOSC = 2.000 MHz FOSC = 1.000 MHz
Actual
Rate
(K)
%
Error
SPBRG
value
(decimal)
Actual
Rate
(K)
%
Error
SPBRG
value
(decimal)
Actual
Rate
(K)
%
Error
SPBRG
value
(decimal)
0.3 0.300 0.16 207 300 -0.16 103 300 -0.16 51
1.2 1.202 0.16 51 1201 -0.16 25 1201 -0.16 12
2.4 2.404 0.16 25 2403 -0.16 12 — — —
9.6 8.929 -6.99 6 — — — — — —
19.2 20.833 8.51 2 — — — — — —
57.6 62.500 8.51 0 — — — — — —
115.2 62.500 -45.75 0 — — — — — —
BAUD
RATE
(K)
SYNC = 0, BRGH = 1, BRG16 = 0
FOSC = 40.000 MHz FOSC = 20.000 MHz FOSC = 10.000 MHz FOSC = 8.000 MHz
Actual
Rate
(K)
%
Error
SPBRG
value
(decimal)
Actual
Rate
(K)
%
Error
SPBRG
value
(decimal)
Actual
Rate
(K)
%
Error
SPBRG
value
(decimal)
Actual
Rate
(K)
%
Error
SPBRG
value
(decimal)
0.3 — — — — — — — — — — — —
1.2 — — — — — — — — — — — —
2.4 — — — — — — 2.441 1.73 255 2403 -0.16 207
9.6 9.766 1.73 255 9.615 0.16 129 9.615 0.16 64 9615 -0.16 51
19.2 19.231 0.16 129 19.231 0.16 64 19.531 1.73 31 19230 -0.16 25
57.6 58.140 0.94 42 56.818 -1.36 21 56.818 -1.36 10 55555 3.55 8
115.2 113.636 -1.36 21 113.636 -1.36 10 125.000 8.51 4 — — —
BAUD
RATE
(K)
SYNC = 0, BRGH = 1, BRG16 = 0
FOSC = 4.000 MHz FOSC = 2.000 MHz FOSC = 1.000 MHz
Actual
Rate
(K)
%
Error
SPBRG
value
(decimal)
Actual
Rate
(K)
%
Error
SPBRG
value
(decimal)
Actual
Rate
(K)
%
Error
SPBRG
value
(decimal)
0.3 — — — — — — 300 -0.16 207
1.2 1.202 0.16 207 1201 -0.16 103 1201 -0.16 51
2.4 2.404 0.16 103 2403 -0.16 51 2403 -0.16 25
9.6 9.615 0.16 25 9615 -0.16 12 — — —
19.2 19.231 0.16 12 — — — — — —
57.6 62.500 8.51 3 — — — — — —
115.2 125.000 8.51 1 — — — — — —
PIC18F2420/2520/4420/4520
DS39631B-page 208 Preliminary © 2007 Microchip Technology Inc.
BAUD
RATE
(K)
SYNC = 0, BRGH = 0, BRG16 = 1
FOSC = 40.000 MHz FOSC = 20.000 MHz FOSC = 10.000 MHz FOSC = 8.000 MHz
Actual
Rate
(K)
%
Error
SPBRG
value
(decimal)
Actual
Rate
(K)
%
Error
SPBRG
value
(decimal)
Actual
Rate
(K)
%
Error
SPBRG
value
(decimal)
Actual
Rate
(K)
%
Error
SPBRG
value
(decimal)
0.3 0.300 0.00 8332 0.300 0.02 4165 0.300 0.02 2082 300 -0.04 1665
1.2 1.200 0.02 2082 1.200 -0.03 1041 1.200 -0.03 520 1201 -0.16 415
2.4 2.402 0.06 1040 2.399 -0.03 520 2.404 0.16 259 2403 -0.16 207
9.6 9.615 0.16 259 9.615 0.16 129 9.615 0.16 64 9615 -0.16 51
19.2 19.231 0.16 129 19.231 0.16 64 19.531 1.73 31 19230 -0.16 25
57.6 58.140 0.94 42 56.818 -1.36 21 56.818 -1.36 10 55555 3.55 8
115.2 113.636 -1.36 21 113.636 -1.36 10 125.000 8.51 4 — — —
BAUD
RATE
(K)
SYNC = 0, BRGH = 0, BRG16 = 1
FOSC = 4.000 MHz FOSC = 2.000 MHz FOSC = 1.000 MHz
Actual
Rate
(K)
%
Error
SPBRG
value
(decimal)
Actual
Rate
(K)
%
Error
SPBRG
value
(decimal)
Actual
Rate
(K)
%
Error
SPBRG
value
(decimal)
0.3 0.300 0.04 832 300 -0.16 415 300 -0.16 207
1.2 1.202 0.16 207 1201 -0.16 103 1201 -0.16 51
2.4 2.404 0.16 103 2403 -0.16 51 2403 -0.16 25
9.6 9.615 0.16 25 9615 -0.16 12 — — —
19.2 19.231 0.16 12 — — — — — —
57.6 62.500 8.51 3 — — — — — —
115.2 125.000 8.51 1 — — — — — —
BAUD
RATE
(K)
SYNC = 0, BRGH = 1, BRG16 = 1 or SYNC = 1, BRG16 = 1
FOSC = 40.000 MHz FOSC = 20.000 MHz FOSC = 10.000 MHz FOSC = 8.000 MHz
Actual
Rate
(K)
%
Error
SPBRG
value
(decimal)
Actual
Rate
(K)
%
Error
SPBRG
value
(decimal)
Actual
Rate
(K)
%
Error
SPBRG
value
(decimal)
Actual
Rate
(K)
%
Error
SPBRG
value
(decimal)
0.3 0.300 0.00 33332 0.300 0.00 16665 0.300 0.00 8332 300 -0.01 6665
1.2 1.200 0.00 8332 1.200 0.02 4165 1.200 0.02 2082 1200 -0.04 1665
2.4 2.400 0.02 4165 2.400 0.02 2082 2.402 0.06 1040 2400 -0.04 832
9.6 9.606 0.06 1040 9.596 -0.03 520 9.615 0.16 259 9615 -0.16 207
19.2 19.193 -0.03 520 19.231 0.16 259 19.231 0.16 129 19230 -0.16 103
57.6 57.803 0.35 172 57.471 -0.22 86 58.140 0.94 42 57142 0.79 34
115.2 114.943 -0.22 86 116.279 0.94 42 113.636 -1.36 21 117647 -2.12 16
BAUD
RATE
(K)
SYNC = 0, BRGH = 1, BRG16 = 1 or SYNC = 1, BRG16 = 1
FOSC = 4.000 MHz FOSC = 2.000 MHz FOSC = 1.000 MHz
Actual
Rate
(K)
%
Error
SPBRG
value
(decimal)
Actual
Rate
(K)
%
Error
SPBRG
value
(decimal)
Actual
Rate
(K)
%
Error
SPBRG
value
(decimal)
0.3 0.300 0.01 3332 300 -0.04 1665 300 -0.04 832
1.2 1.200 0.04 832 1201 -0.16 415 1201 -0.16 207
2.4 2.404 0.16 415 2403 -0.16 207 2403 -0.16 103
9.6 9.615 0.16 103 9615 -0.16 51 9615 -0.16 25
19.2 19.231 0.16 51 19230 -0.16 25 19230 -0.16 12
57.6 58.824 2.12 16 55555 3.55 8 — — —
115.2 111.111 -3.55 8 — — — — — —
TABLE 18-3: BAUD RATES FOR ASYNCHRONOUS MODES (CONTINUED)
© 2007 Microchip Technology Inc. Preliminary DS39631B-page 209
PIC18F2420/2520/4420/4520
18.1.3 AUTO-BAUD RATE DETECT
The enhanced USART module supports the automatic
detection and calibration of baud rate. This feature is
active only in Asynchronous mode and while the WUE
bit is clear.
The automatic baud rate measurement sequence
(Figure 18-1) begins whenever a Start bit is received
and the ABDEN bit is set. The calculation is
self-averaging.
In the Auto-Baud Rate Detect (ABD) mode, the clock to
the BRG is reversed. Rather than the BRG clocking the
incoming RX signal, the RX signal is timing the BRG. In
ABD mode, the internal Baud Rate Generator is used
as a counter to time the bit period of the incoming serial
byte stream.
Once the ABDEN bit is set, the state machine will clear
the BRG and look for a Start bit. The Auto-Baud Rate
Detect must receive a byte with the value 55h (ASCII
“U”, which is also the LIN bus Sync character) in order to
calculate the proper bit rate. The measurement is taken
over both a low and a high bit time in order to minimize
any effects caused by asymmetry of the incoming signal.
After a Start bit, the SPBRG begins counting up, using
the preselected clock source on the first rising edge of
RX. After eight bits on the RX pin or the fifth rising edge,
an accumulated value totalling the proper BRG period is
left in the SPBRGH:SPBRG register pair. Once the 5th
edge is seen (this should correspond to the Stop bit), the
ABDEN bit is automatically cleared.
If a rollover of the BRG occurs (an overflow from FFFFh
to 0000h), the event is trapped by the ABDOVF status
bit (BAUDCON<7>). It is set in hardware by BRG rollovers
and can be set or cleared by the user in software.
ABD mode remains active after rollover events and the
ABDEN bit remains set (Figure 18-2).
While calibrating the baud rate period, the BRG registers
are clocked at 1/8th the preconfigured clock rate.
Note that the BRG clock will be configured by the
BRG16 and BRGH bits. Independent of the BRG16 bit
setting, both the SPBRG and SPBRGH will be used as
a 16-bit counter. This allows the user to verify that no
carry occurred for 8-bit modes by checking for 00h in
the SPBRGH register. Refer to Table 18-4 for counter
clock rates to the BRG.
While the ABD sequence takes place, the EUSART
state machine is held in Idle. The RCIF interrupt is set
once the fifth rising edge on RX is detected. The value
in the RCREG needs to be read to clear the RCIF
interrupt. The contents of RCREG should be discarded.
TABLE 18-4: BRG COUNTER
CLOCK RATES
18.1.3.1 ABD and EUSART Transmission
Since the BRG clock is reversed during ABD acquisition,
the EUSART transmitter cannot be used during
ABD. This means that whenever the ABDEN bit is set,
TXREG cannot be written to. Users should also ensure
that ABDEN does not become set during a transmit
sequence. Failing to do this may result in unpredictable
EUSART operation.
Note 1: If the WUE bit is set with the ABDEN bit,
Auto-Baud Rate Detection will occur on
the byte following the Break character.
2: It is up to the user to determine that the
incoming character baud rate is within the
range of the selected BRG clock source.
Some combinations of oscillator frequency
and EUSART baud rates are not possible
due to bit error rates. Overall system timing
and communication baud rates must
be taken into consideration when using the
Auto-Baud Rate Detection feature.
BRG16 BRGH BRG Counter Clock
0 0 FOSC/512
0 1 FOSC/128
1 0 FOSC/128
1 1 FOSC/32
Note: During the ABD sequence, SPBRG and
SPBRGH are both used as a 16-bit counter,
independent of BRG16 setting.
PIC18F2420/2520/4420/4520
DS39631B-page 210 Preliminary © 2007 Microchip Technology Inc.
FIGURE 18-1: AUTOMATIC BAUD RATE CALCULATION
FIGURE 18-2: BRG OVERFLOW SEQUENCE
BRG Value
RX pin
ABDEN bit
RCIF bit
Bit 0 Bit 1
(Interrupt)
Read
RCREG
BRG Clock
Start
Set by User Auto-Cleared
XXXXh 0000h
Edge #1
Bit 2 Bit 3
Edge #2
Bit 4 Bit 5
Edge #3
Bit 6 Bit 7
Edge #4
Stop Bit
Edge #5
001Ch
Note: The ABD sequence requires the EUSART module to be configured in Asynchronous mode and WUE = 0.
SPBRG XXXXh 1Ch
SPBRGH XXXXh 00h
Start Bit 0
XXXXh 0000h 0000h
FFFFh
BRG Clock
ABDEN bit
RX pin
ABDOVF bit
BRG Value
© 2007 Microchip Technology Inc. Preliminary DS39631B-page 211
PIC18F2420/2520/4420/4520
18.2 EUSART Asynchronous Mode
The Asynchronous mode of operation is selected by
clearing the SYNC bit (TXSTA<4>). In this mode, the
EUSART uses standard Non-Return-to-Zero (NRZ) format
(one Start bit, eight or nine data bits and one Stop
bit). The most common data format is 8 bits. An on-chip
dedicated 8-bit/16-bit Baud Rate Generator can be used
to derive standard baud rate frequencies from the
oscillator.
The EUSART transmits and receives the LSb first. The
EUSART’s transmitter and receiver are functionally
independent but use the same data format and baud
rate. The Baud Rate Generator produces a clock, either
x16 or x64 of the bit shift rate depending on the BRGH
and BRG16 bits (TXSTA<2> and BAUDCON<3>). Parity
is not supported by the hardware but can be
implemented in software and stored as the 9th data bit.
When operating in Asynchronous mode, the EUSART
module consists of the following important elements:
• Baud Rate Generator
• Sampling Circuit
• Asynchronous Transmitter
• Asynchronous Receiver
• Auto-Wake-up on Sync Break Character
• 12-bit Break Character Transmit
• Auto-Baud Rate Detection
18.2.1 EUSART ASYNCHRONOUS
TRANSMITTER
The EUSART transmitter block diagram is shown in
Figure 18-3. The heart of the transmitter is the Transmit
(Serial) Shift Register (TSR). The Shift register obtains
its data from the Read/Write Transmit Buffer register,
TXREG. The TXREG register is loaded with data in
software. The TSR register is not loaded until the Stop
bit has been transmitted from the previous load. As
soon as the Stop bit is transmitted, the TSR is loaded
with new data from the TXREG register (if available).
Once the TXREG register transfers the data to the TSR
register (occurs in one TCY), the TXREG register is empty
and the TXIF flag bit (PIR1<4>) is set. This interrupt can
be enabled or disabled by setting or clearing the interrupt
enable bit, TXIE (PIE1<4>). TXIF will be set regardless of
the state of TXIE; it cannot be cleared in software. TXIF
is also not cleared immediately upon loading TXREG, but
becomes valid in the second instruction cycle following
the load instruction. Polling TXIF immediately following a
load of TXREG will return invalid results.
While TXIF indicates the status of the TXREG register,
another bit, TRMT (TXSTA<1>), shows the status of
the TSR register. TRMT is a read-only bit which is set
when the TSR register is empty. No interrupt logic is
tied to this bit so the user has to poll this bit in order to
determine if the TSR register is empty.
To set up an Asynchronous Transmission:
1. Initialize the SPBRGH:SPBRG registers for the
appropriate baud rate. Set or clear the BRGH
and BRG16 bits, as required, to achieve the
desired baud rate.
2. Enable the asynchronous serial port by clearing
bit SYNC and setting bit SPEN.
3. If interrupts are desired, set enable bit TXIE.
4. If 9-bit transmission is desired, set transmit bit
TX9. Can be used as address/data bit.
5. Enable the transmission by setting bit TXEN
which will also set bit TXIF.
6. If 9-bit transmission is selected, the ninth bit
should be loaded in bit TX9D.
7. Load data to the TXREG register (starts
transmission).
8. If using interrupts, ensure that the GIE and PEIE
bits in the INTCON register (INTCON<7:6>) are
set.
FIGURE 18-3: EUSART TRANSMIT BLOCK DIAGRAM
Note 1: The TSR register is not mapped in data
memory so it is not available to the user.
2: Flag bit TXIF is set when enable bit TXEN
is set.
TXIF
TXIE
Interrupt
TXEN Baud Rate CLK
SPBRG
Baud Rate Generator TX9D
MSb LSb
Data Bus
TXREG Register
TSR Register
(8) 0
TX9
TRMT SPEN
TX pin
Pin Buffer
and Control
8
• • •
BRG16 SPBRGH
PIC18F2420/2520/4420/4520
DS39631B-page 212 Preliminary © 2007 Microchip Technology Inc.
FIGURE 18-4: ASYNCHRONOUS TRANSMISSION
FIGURE 18-5: ASYNCHRONOUS TRANSMISSION (BACK TO BACK)
TABLE 18-5: REGISTERS ASSOCIATED WITH ASYNCHRONOUS TRANSMISSION
Word 1
Word 1
Transmit Shift Reg
Start bit bit 0 bit 1 bit 7/8
Write to TXREG
BRG Output
(Shift Clock)
TX (pin)
TXIF bit
(Transmit Buffer
Reg. Empty Flag)
TRMT bit
(Transmit Shift
Reg. Empty Flag)
1 TCY
Stop bit
Word 1
Transmit Shift Reg.
Write to TXREG
BRG Output
(Shift Clock)
TX (pin)
TXIF bit
(Interrupt Reg. Flag)
TRMT bit
(Transmit Shift
Reg. Empty Flag)
Word 1 Word 2
Word 1 Word 2
Stop bit Start bit
Transmit Shift Reg.
Word 1 Word 2
bit 0 bit 1 bit 7/8 bit 0
Note: This timing diagram shows two consecutive transmissions.
1 TCY
1 TCY
Start bit
Name Bit 7 Bit 6 Bit 5 Bit 4 Bit 3 Bit 2 Bit 1 Bit 0
Reset
Values
on page
INTCON GIE/GIEH PEIE/GIEL TMR0IE INT0IE RBIE TMR0IF INT0IF RBIF 49
PIR1 PSPIF(1) ADIF RCIF TXIF SSPIF CCP1IF TMR2IF TMR1IF 52
PIE1 PSPIE(1) ADIE RCIE TXIE SSPIE CCP1IE TMR2IE TMR1IE 52
IPR1 PSPIP(1) ADIP RCIP TXIP SSPIP CCP1IP TMR2IP TMR1IP 52
RCSTA SPEN RX9 SREN CREN ADDEN FERR OERR RX9D 51
TXREG EUSART Transmit Register 51
TXSTA CSRC TX9 TXEN SYNC SENDB BRGH TRMT TX9D 51
BAUDCON ABDOVF RCIDL — SCKP BRG16 — WUE ABDEN 51
SPBRGH EUSART Baud Rate Generator Register, High Byte 51
SPBRG EUSART Baud Rate Generator Register, Low Byte 51
Legend: — = unimplemented locations read as ‘0’. Shaded cells are not used for asynchronous transmission.
Note 1: Reserved in 28-pin devices; always maintain these bits clear.
© 2007 Microchip Technology Inc. Preliminary DS39631B-page 213
PIC18F2420/2520/4420/4520
18.2.2 EUSART ASYNCHRONOUS
RECEIVER
The receiver block diagram is shown in Figure 18-6.
The data is received on the RX pin and drives the data
recovery block. The data recovery block is actually a
high-speed shifter operating at x16 times the baud rate,
whereas the main receive serial shifter operates at the
bit rate or at FOSC. This mode would typically be used
in RS-232 systems.
To set up an Asynchronous Reception:
1. Initialize the SPBRGH:SPBRG registers for the
appropriate baud rate. Set or clear the BRGH
and BRG16 bits, as required, to achieve the
desired baud rate.
2. Enable the asynchronous serial port by clearing
bit SYNC and setting bit SPEN.
3. If interrupts are desired, set enable bit RCIE.
4. If 9-bit reception is desired, set bit RX9.
5. Enable the reception by setting bit CREN.
6. Flag bit, RCIF, will be set when reception is
complete and an interrupt will be generated if
enable bit, RCIE, was set.
7. Read the RCSTA register to get the 9th bit (if
enabled) and determine if any error occurred
during reception.
8. Read the 8-bit received data by reading the
RCREG register.
9. If any error occurred, clear the error by clearing
enable bit CREN.
10. If using interrupts, ensure that the GIE and PEIE
bits in the INTCON register (INTCON<7:6>) are
set.
18.2.3 SETTING UP 9-BIT MODE WITH
ADDRESS DETECT
This mode would typically be used in RS-485 systems.
To set up an Asynchronous Reception with Address
Detect Enable:
1. Initialize the SPBRGH:SPBRG registers for the
appropriate baud rate. Set or clear the BRGH
and BRG16 bits, as required, to achieve the
desired baud rate.
2. Enable the asynchronous serial port by clearing
the SYNC bit and setting the SPEN bit.
3. If interrupts are required, set the RCEN bit and
select the desired priority level with the RCIP bit.
4. Set the RX9 bit to enable 9-bit reception.
5. Set the ADDEN bit to enable address detect.
6. Enable reception by setting the CREN bit.
7. The RCIF bit will be set when reception is
complete. The interrupt will be Acknowledged if
the RCIE and GIE bits are set.
8. Read the RCSTA register to determine if any
error occurred during reception, as well as read
bit 9 of data (if applicable).
9. Read RCREG to determine if the device is being
addressed.
10. If any error occurred, clear the CREN bit.
11. If the device has been addressed, clear the
ADDEN bit to allow all received data into the
receive buffer and interrupt the CPU.
FIGURE 18-6: EUSART RECEIVE BLOCK DIAGRAM
x64 Baud Rate CLK
Baud Rate Generator
RX
Pin Buffer
and Control
SPEN
Data
Recovery
CREN OERR FERR
MSb RSR Register LSb
RX9D RCREG Register
FIFO
Interrupt RCIF
RCIE
Data Bus
8
÷ 64
÷ 16
or
Stop (8) 7 1 0 Start
RX9
• • •
BRG16 SPBRGH SPBRG
or
÷ 4
PIC18F2420/2520/4420/4520
DS39631B-page 214 Preliminary © 2007 Microchip Technology Inc.
FIGURE 18-7: ASYNCHRONOUS RECEPTION
TABLE 18-6: REGISTERS ASSOCIATED WITH ASYNCHRONOUS RECEPTION
18.2.4 AUTO-WAKE-UP ON SYNC BREAK
CHARACTER
During Sleep mode, all clocks to the EUSART are
suspended. Because of this, the Baud Rate Generator
is inactive and a proper byte reception cannot be performed.
The auto-wake-up feature allows the controller
to wake-up due to activity on the RX/DT line while the
EUSART is operating in Asynchronous mode.
The auto-wake-up feature is enabled by setting the
WUE bit (BAUDCON<1>). Once set, the typical receive
sequence on RX/DT is disabled and the EUSART
remains in an Idle state, monitoring for a wake-up event
independent of the CPU mode. A wake-up event consists
of a high-to-low transition on the RX/DT line. (This
coincides with the start of a Sync Break or a Wake-up
Signal character for the LIN protocol.)
Following a wake-up event, the module generates an
RCIF interrupt. The interrupt is generated synchronously
to the Q clocks in normal operating modes
(Figure 18-8) and asynchronously, if the device is in
Sleep mode (Figure 18-9). The interrupt condition is
cleared by reading the RCREG register.
The WUE bit is automatically cleared once a low-tohigh
transition is observed on the RX line following the
wake-up event. At this point, the EUSART module is in
Idle mode and returns to normal operation. This signals
to the user that the Sync Break event is over.
Name Bit 7 Bit 6 Bit 5 Bit 4 Bit 3 Bit 2 Bit 1 Bit 0
Reset
Values
on page
INTCON GIE/GIEH PEIE/GIEL TMR0IE INT0IE RBIE TMR0IF INT0IF RBIF 49
PIR1 PSPIF(1) ADIF RCIF TXIF SSPIF CCP1IF TMR2IF TMR1IF 52
PIE1 PSPIE(1) ADIE RCIE TXIE SSPIE CCP1IE TMR2IE TMR1IE 52
IPR1 PSPIP(1) ADIP RCIP TXIP SSPIP CCP1IP TMR2IP TMR1IP 52
RCSTA SPEN RX9 SREN CREN ADDEN FERR OERR RX9D 51
RCREG EUSART Receive Register 51
TXSTA CSRC TX9 TXEN SYNC SENDB BRGH TRMT TX9D 51
BAUDCON ABDOVF RCIDL — SCKP BRG16 — WUE ABDEN 51
SPBRGH EUSART Baud Rate Generator Register, High Byte 51
SPBRG EUSART Baud Rate Generator Register, Low Byte 51
Legend: — = unimplemented locations read as ‘0’. Shaded cells are not used for asynchronous reception.
Note 1: Reserved in 28-pin devices; always maintain these bits clear.
Start
bit bit 0 bit 1 bit 7/8 Stop bit 0 bit 7/8
bit
Start
bit
Start
bit 7/8 Stop bit
bit
RX (pin)
Rcv Buffer Reg
Rcv Shift Reg
Read Rcv
Buffer Reg
RCREG
RCIF
(Interrupt Flag)
OERR bit
CREN
Word 1
RCREG
Word 2
RCREG
Stop
bit
Note: This timing diagram shows three words appearing on the RX input. The RCREG (receive buffer) is read after the third word
causing the OERR (overrun) bit to be set.
© 2007 Microchip Technology Inc. Preliminary DS39631B-page 215
PIC18F2420/2520/4420/4520
18.2.4.1 Special Considerations Using
Auto-Wake-up
Since auto-wake-up functions by sensing rising edge
transitions on RX/DT, information with any state
changes before the Stop bit may signal a false end-ofcharacter
and cause data or framing errors. To work
properly, therefore, the initial character in the transmission
must be all ‘0’s. This can be 00h (8 bytes) for
standard RS-232 devices or 000h (12 bits) for LIN bus.
Oscillator start-up time must also be considered,
especially in applications using oscillators with longer
start-up intervals (i.e., XT or HS mode). The Sync
Break (or Wake-up Signal) character must be of
sufficient length and be followed by a sufficient interval
to allow enough time for the selected oscillator to start
and provide proper initialization of the EUSART.
18.2.4.2 Special Considerations Using
the WUE Bit
The timing of WUE and RCIF events may cause some
confusion when it comes to determining the validity of
received data. As noted, setting the WUE bit places the
EUSART in an Idle mode. The wake-up event causes a
receive interrupt by setting the RCIF bit. The WUE bit is
cleared after this when a rising edge is seen on RX/DT.
The interrupt condition is then cleared by reading the
RCREG register. Ordinarily, the data in RCREG will be
dummy data and should be discarded.
The fact that the WUE bit has been cleared (or is still
set) and the RCIF flag is set should not be used as an
indicator of the integrity of the data in RCREG. Users
should consider implementing a parallel method in
firmware to verify received data integrity.
To assure that no actual data is lost, check the RCIDL
bit to verify that a receive operation is not in process. If
a receive operation is not occurring, the WUE bit may
then be set just prior to entering the Sleep mode.
FIGURE 18-8: AUTO-WAKE-UP BIT (WUE) TIMINGS DURING NORMAL OPERATION
FIGURE 18-9: AUTO-WAKE-UP BIT (WUE) TIMINGS DURING SLEEP
Q1 Q2 Q3 Q4 Q1 Q2 Q3 Q4 Q1 Q2 Q3 Q4 Q1 Q2 Q3 Q4 Q1 Q2 Q3 Q4 Q1 Q2 Q3 Q4 Q1 Q2 Q3 Q4 Q1 Q2 Q3 Q4 Q1 Q2 Q3 Q4 Q1 Q2 Q3 Q4
OSC1
WUE bit(1)
RX/DT Line
RCIF
Note 1: The EUSART remains in Idle while the WUE bit is set.
Bit set by user
Cleared due to user read of RCREG
Auto-Cleared
Q1 Q2 Q3 Q4 Q1 Q2 Q3 Q4 Q1 Q2 Q3 Q4 Q1 Q2 Q3 Q4 Q1 Q2 Q3 Q4 Q1 Q2 Q3 Q4 Q1 Q2 Q3 Q4 Q1 Q2 Q3 Q4
OSC1
WUE bit(2)
RX/DT Line
RCIF
Bit set by user
Cleared due to user read of RCREG
Sleep Command Executed
Note 1: If the wake-up event requires long oscillator warm-up time, the auto-clear of the WUE bit can occur before the oscillator is ready. This
sequence should not depend on the presence of Q clocks.
2: The EUSART remains in Idle while the WUE bit is set.
Sleep Ends
Note 1
Auto-Cleared
PIC18F2420/2520/4420/4520
DS39631B-page 216 Preliminary © 2007 Microchip Technology Inc.
18.2.5 BREAK CHARACTER SEQUENCE
The EUSART module has the capability of sending the
special Break character sequences that are required by
the LIN bus standard. The Break character transmit
consists of a Start bit, followed by twelve ‘0’ bits and a
Stop bit. The frame Break character is sent whenever
the SENDB and TXEN bits (TXSTA<3> and
TXSTA<5>) are set while the Transmit Shift register is
loaded with data. Note that the value of data written to
TXREG will be ignored and all ‘0’s will be transmitted.
The SENDB bit is automatically reset by hardware after
the corresponding Stop bit is sent. This allows the user
to preload the transmit FIFO with the next transmit byte
following the Break character (typically, the Sync
character in the LIN specification).
Note that the data value written to the TXREG for the
Break character is ignored. The write simply serves the
purpose of initiating the proper sequence.
The TRMT bit indicates when the transmit operation is
active or Idle, just as it does during normal transmission.
See Figure 18-10 for the timing of the Break
character sequence.
18.2.5.1 Break and Sync Transmit Sequence
The following sequence will send a message frame
header made up of a Break, followed by an Auto-Baud
Sync byte. This sequence is typical of a LIN bus
master.
1. Configure the EUSART for the desired mode.
2. Set the TXEN and SENDB bits to set up the
Break character.
3. Load the TXREG with a dummy character to
initiate transmission (the value is ignored).
4. Write ‘55h’ to TXREG to load the Sync character
into the transmit FIFO buffer.
5. After the Break has been sent, the SENDB bit is
reset by hardware. The Sync character now
transmits in the preconfigured mode.
When the TXREG becomes empty, as indicated by the
TXIF, the next data byte can be written to TXREG.
18.2.6 RECEIVING A BREAK CHARACTER
The enhanced USART module can receive a Break
character in two ways.
The first method forces configuration of the baud rate
at a frequency of 9/13 the typical speed. This allows for
the Stop bit transition to be at the correct sampling location
(13 bits for Break versus Start bit and 8 data bits for
typical data).
The second method uses the auto-wake-up feature
described in Section 18.2.4 “Auto-Wake-up on Sync
Break Character”. By enabling this feature, the
EUSART will sample the next two transitions on RX/DT,
cause an RCIF interrupt and receive the next data byte
followed by another interrupt.
Note that following a Break character, the user will
typically want to enable the Auto-Baud Rate Detect
feature. For both methods, the user can set the ABD bit
once the TXIF interrupt is observed.
FIGURE 18-10: SEND BREAK CHARACTER SEQUENCE
Write to TXREG
BRG Output
(Shift Clock)
Start Bit Bit 0 Bit 1 Bit 11 Stop Bit
Break
TXIF bit
(Transmit Buffer
Reg. Empty Flag)
TX (pin)
TRMT bit
(Transmit Shift
Reg. Empty Flag)
SENDB
(Transmit Shift
Reg. Empty Flag)
SENDB sampled here Auto-Cleared
Dummy Write
© 2007 Microchip Technology Inc. Preliminary DS39631B-page 217
PIC18F2420/2520/4420/4520
18.3 EUSART Synchronous
Master Mode
The Synchronous Master mode is entered by setting
the CSRC bit (TXSTA<7>). In this mode, the data is
transmitted in a half-duplex manner (i.e., transmission
and reception do not occur at the same time). When
transmitting data, the reception is inhibited and vice
versa. Synchronous mode is entered by setting bit
SYNC (TXSTA<4>). In addition, enable bit SPEN
(RCSTA<7>) is set in order to configure the TX and RX
pins to CK (clock) and DT (data) lines, respectively.
The Master mode indicates that the processor transmits
the master clock on the CK line. Clock polarity is
selected with the SCKP bit (BAUDCON<4>); setting
SCKP sets the Idle state on CK as high, while clearing
the bit sets the Idle state as low. This option is provided
to support Microwire devices with this module.
18.3.1 EUSART SYNCHRONOUS MASTER
TRANSMISSION
The EUSART transmitter block diagram is shown in
Figure 18-3. The heart of the transmitter is the Transmit
(Serial) Shift Register (TSR). The Shift register obtains
its data from the Read/Write Transmit Buffer register,
TXREG. The TXREG register is loaded with data in
software. The TSR register is not loaded until the last
bit has been transmitted from the previous load. As
soon as the last bit is transmitted, the TSR is loaded
with new data from the TXREG (if available).
Once the TXREG register transfers the data to the TSR
register (occurs in one TCY), the TXREG is empty and
the TXIF flag bit (PIR1<4>) is set. The interrupt can be
enabled or disabled by setting or clearing the interrupt
enable bit, TXIE (PIE1<4>). TXIF is set regardless of
the state of enable bit TXIE; it cannot be cleared in
software. It will reset only when new data is loaded into
the TXREG register.
While flag bit TXIF indicates the status of the TXREG
register, another bit, TRMT (TXSTA<1>), shows the
status of the TSR register. TRMT is a read-only bit which
is set when the TSR is empty. No interrupt logic is tied to
this bit so the user has to poll this bit in order to determine
if the TSR register is empty. The TSR is not
mapped in data memory so it is not available to the user.
To set up a Synchronous Master Transmission:
1. Initialize the SPBRGH:SPBRG registers for the
appropriate baud rate. Set or clear the BRG16
bit, as required, to achieve the desired baud rate.
2. Enable the synchronous master serial port by
setting bits SYNC, SPEN and CSRC.
3. If interrupts are desired, set enable bit TXIE.
4. If 9-bit transmission is desired, set bit TX9.
5. Enable the transmission by setting bit TXEN.
6. If 9-bit transmission is selected, the ninth bit
should be loaded in bit TX9D.
7. Start transmission by loading data to the TXREG
register.
8. If using interrupts, ensure that the GIE and PEIE
bits in the INTCON register (INTCON<7:6>) are
set.
FIGURE 18-11: SYNCHRONOUS TRANSMISSION
bit 0 bit 1 bit 7
Word 1
Q1 Q2 Q3Q4 Q1 Q2 Q3Q4 Q1Q2 Q3 Q4 Q1Q2 Q3 Q4Q1 Q2 Q3 Q4 Q3Q4 Q1Q2 Q3Q4 Q1Q2 Q3Q4 Q1 Q2Q3Q4 Q1 Q2Q3 Q4Q1 Q2 Q3 Q4 Q1 Q2 Q3 Q4
bit 2 bit 0 bit 1 bit 7
RC7/RX/DT
RC6/TX/CK pin
Write to
TXREG Reg
TXIF bit
(Interrupt Flag)
TXEN bit ‘1’ ‘1’
Word 2
TRMT bit
Write Word 1 Write Word 2
Note: Sync Master mode, SPBRG = 0, continuous transmission of two 8-bit words.
RC6/TX/CK pin
(SCKP = 0)
(SCKP = 1)
PIC18F2420/2520/4420/4520
DS39631B-page 218 Preliminary © 2007 Microchip Technology Inc.
FIGURE 18-12: SYNCHRONOUS TRANSMISSION (THROUGH TXEN)
TABLE 18-7: REGISTERS ASSOCIATED WITH SYNCHRONOUS MASTER TRANSMISSION
RC7/RX/DT pin
RC6/TX/CK pin
Write to
TXREG reg
TXIF bit
TRMT bit
bit 0 bit 1 bit 2 bit 6 bit 7
TXEN bit
Name Bit 7 Bit 6 Bit 5 Bit 4 Bit 3 Bit 2 Bit 1 Bit 0
Reset
Values
on page
INTCON GIE/GIEH PEIE/GIEL TMR0IE INT0IE RBIE TMR0IF INT0IF RBIF 49
PIR1 PSPIF(1) ADIF RCIF TXIF SSPIF CCP1IF TMR2IF TMR1IF 52
PIE1 PSPIE(1) ADIE RCIE TXIE SSPIE CCP1IE TMR2IE TMR1IE 52
IPR1 PSPIP(1) ADIP RCIP TXIP SSPIP CCP1IP TMR2IP TMR1IP 52
RCSTA SPEN RX9 SREN CREN ADDEN FERR OERR RX9D 51
TXREG EUSART Transmit Register 51
TXSTA CSRC TX9 TXEN SYNC SENDB BRGH TRMT TX9D 51
BAUDCON ABDOVF RCIDL — SCKP BRG16 — WUE ABDEN 51
SPBRGH EUSART Baud Rate Generator Register, High Byte 51
SPBRG EUSART Baud Rate Generator Register, Low Byte 51
Legend: — = unimplemented, read as ‘0’. Shaded cells are not used for synchronous master transmission.
Note 1: Reserved in 28-pin devices; always maintain these bits clear.
© 2007 Microchip Technology Inc. Preliminary DS39631B-page 219
PIC18F2420/2520/4420/4520
18.3.2 EUSART SYNCHRONOUS
MASTER RECEPTION
Once Synchronous mode is selected, reception is
enabled by setting either the Single Receive Enable bit,
SREN (RCSTA<5>), or the Continuous Receive
Enable bit, CREN (RCSTA<4>). Data is sampled on the
RX pin on the falling edge of the clock.
If enable bit SREN is set, only a single word is received.
If enable bit CREN is set, the reception is continuous
until CREN is cleared. If both bits are set, then CREN
takes precedence.
To set up a Synchronous Master Reception:
1. Initialize the SPBRGH:SPBRG registers for the
appropriate baud rate. Set or clear the BRG16
bit, as required, to achieve the desired baud rate.
2. Enable the synchronous master serial port by
setting bits SYNC, SPEN and CSRC.
3. Ensure bits CREN and SREN are clear.
4. If interrupts are desired, set enable bit RCIE.
5. If 9-bit reception is desired, set bit RX9.
6. If a single reception is required, set bit SREN.
For continuous reception, set bit CREN.
7. Interrupt flag bit, RCIF, will be set when reception
is complete and an interrupt will be generated if
the enable bit, RCIE, was set.
8. Read the RCSTA register to get the 9th bit (if
enabled) and determine if any error occurred
during reception.
9. Read the 8-bit received data by reading the
RCREG register.
10. If any error occurred, clear the error by clearing
bit CREN.
11. If using interrupts, ensure that the GIE and PEIE bits
in the INTCON register (INTCON<7:6>) are set.
FIGURE 18-13: SYNCHRONOUS RECEPTION (MASTER MODE, SREN)
TABLE 18-8: REGISTERS ASSOCIATED WITH SYNCHRONOUS MASTER RECEPTION
Name Bit 7 Bit 6 Bit 5 Bit 4 Bit 3 Bit 2 Bit 1 Bit 0
Reset
Values
on page
INTCON GIE/GIEH PEIE/GIEL TMR0IE INT0IE RBIE TMR0IF INT0IF RBIF 49
PIR1 PSPIF(1) ADIF RCIF TXIF SSPIF CCP1IF TMR2IF TMR1IF 52
PIE1 PSPIE(1) ADIE RCIE TXIE SSPIE CCP1IE TMR2IE TMR1IE 52
IPR1 PSPIP(1) ADIP RCIP TXIP SSPIP CCP1IP TMR2IP TMR1IP 52
RCSTA SPEN RX9 SREN CREN ADDEN FERR OERR RX9D 51
RCREG EUSART Receive Register 51
TXSTA CSRC TX9 TXEN SYNC SENDB BRGH TRMT TX9D 51
BAUDCON ABDOVF RCIDL — SCKP BRG16 — WUE ABDEN 51
SPBRGH EUSART Baud Rate Generator Register, High Byte 51
SPBRG EUSART Baud Rate Generator Register, Low Byte 51
Legend: — = unimplemented, read as ‘0’. Shaded cells are not used for synchronous master reception.
Note 1: Reserved in 28-pin devices; always maintain these bits clear.
CREN bit
RC7/RX/DT
RC6/TX/CK pin
Write to
bit SREN
SREN bit
RCIF bit
(Interrupt)
Read
RXREG
Q2 Q3 Q4 Q1 Q2 Q3 Q4 Q1 Q2 Q3 Q4 Q1 Q2 Q3 Q4 Q1 Q2 Q3 Q4 Q1 Q2 Q3 Q4Q1 Q2 Q3 Q4 Q1Q2 Q3 Q4 Q1 Q2Q3 Q4 Q1 Q2 Q3 Q4
‘0’
bit 0 bit 1 bit 2 bit 3 bit 4 bit 5 bit 6 bit 7
‘0’
Q1 Q2 Q3 Q4
Note: Timing diagram demonstrates Sync Master mode with bit SREN = 1 and bit BRGH = 0.
RC6/TX/CK pin
pin
(SCKP = 0)
(SCKP = 1)
PIC18F2420/2520/4420/4520
DS39631B-page 220 Preliminary © 2007 Microchip Technology Inc.
18.4 EUSART Synchronous
Slave Mode
Synchronous Slave mode is entered by clearing bit,
CSRC (TXSTA<7>). This mode differs from the
Synchronous Master mode in that the shift clock is supplied
externally at the CK pin (instead of being supplied
internally in Master mode). This allows the device to
transfer or receive data while in any low-power mode.
18.4.1 EUSART SYNCHRONOUS
SLAVE TRANSMISSION
The operation of the Synchronous Master and Slave
modes are identical, except in the case of the Sleep
mode.
If two words are written to the TXREG and then the
SLEEP instruction is executed, the following will occur:
a) The first word will immediately transfer to the
TSR register and transmit.
b) The second word will remain in the TXREG
register.
c) Flag bit, TXIF, will not be set.
d) When the first word has been shifted out of TSR,
the TXREG register will transfer the second
word to the TSR and flag bit, TXIF, will now be
set.
e) If enable bit TXIE is set, the interrupt will wake the
chip from Sleep. If the global interrupt is enabled,
the program will branch to the interrupt vector.
To set up a Synchronous Slave Transmission:
1. Enable the synchronous slave serial port by
setting bits SYNC and SPEN and clearing bit
CSRC.
2. Clear bits CREN and SREN.
3. If interrupts are desired, set enable bit TXIE.
4. If 9-bit transmission is desired, set bit TX9.
5. Enable the transmission by setting enable bit
TXEN.
6. If 9-bit transmission is selected, the ninth bit
should be loaded in bit TX9D.
7. Start transmission by loading data to the
TXREGx register.
8. If using interrupts, ensure that the GIE and PEIE
bits in the INTCON register (INTCON<7:6>) are
set.
TABLE 18-9: REGISTERS ASSOCIATED WITH SYNCHRONOUS SLAVE TRANSMISSION
Name Bit 7 Bit 6 Bit 5 Bit 4 Bit 3 Bit 2 Bit 1 Bit 0
Reset
Values
on page
INTCON GIE/GIEH PEIE/GIEL TMR0IE INT0IE RBIE TMR0IF INT0IF RBIF 49
PIR1 PSPIF(1) ADIF RCIF TXIF SSPIF CCP1IF TMR2IF TMR1IF 52
PIE1 PSPIE(1) ADIE RCIE TXIE SSPIE CCP1IE TMR2IE TMR1IE 52
IPR1 PSPIP(1) ADIP RCIP TXIP SSPIP CCP1IP TMR2IP TMR1IP 52
RCSTA SPEN RX9 SREN CREN ADDEN FERR OERR RX9D 51
TXREG EUSART Transmit Register 51
TXSTA CSRC TX9 TXEN SYNC SENDB BRGH TRMT TX9D 51
BAUDCON ABDOVF RCIDL — SCKP BRG16 — WUE ABDEN 51
SPBRGH EUSART Baud Rate Generator Register, High Byte 51
SPBRG EUSART Baud Rate Generator Register, Low Byte 51
Legend: — = unimplemented, read as ‘0’. Shaded cells are not used for synchronous slave transmission.
Note 1: Reserved in 28-pin devices; always maintain these bits clear.
© 2007 Microchip Technology Inc. Preliminary DS39631B-page 221
PIC18F2420/2520/4420/4520
18.4.2 EUSART SYNCHRONOUS SLAVE
RECEPTION
The operation of the Synchronous Master and Slave
modes is identical, except in the case of Sleep, or any
Idle mode and bit SREN, which is a “don’t care” in
Slave mode.
If receive is enabled by setting the CREN bit prior to
entering Sleep or any Idle mode, then a word may be
received while in this low-power mode. Once the word
is received, the RSR register will transfer the data to the
RCREG register; if the RCIE enable bit is set, the interrupt
generated will wake the chip from the low-power
mode. If the global interrupt is enabled, the program will
branch to the interrupt vector.
To set up a Synchronous Slave Reception:
1. Enable the synchronous master serial port by
setting bits SYNC and SPEN and clearing bit
CSRC.
2. If interrupts are desired, set enable bit RCIE.
3. If 9-bit reception is desired, set bit RX9.
4. To enable reception, set enable bit CREN.
5. Flag bit, RCIF, will be set when reception is
complete. An interrupt will be generated if
enable bit, RCIE, was set.
6. Read the RCSTA register to get the 9th bit (if
enabled) and determine if any error occurred
during reception.
7. Read the 8-bit received data by reading the
RCREG register.
8. If any error occurred, clear the error by clearing
bit CREN.
9. If using interrupts, ensure that the GIE and PEIE
bits in the INTCON register (INTCON<7:6>) are
set.
TABLE 18-10: REGISTERS ASSOCIATED WITH SYNCHRONOUS SLAVE RECEPTION
Name Bit 7 Bit 6 Bit 5 Bit 4 Bit 3 Bit 2 Bit 1 Bit 0
Reset
Values
on page
INTCON GIE/GIEH PEIE/GIEL TMR0IE INT0IE RBIE TMR0IF INT0IF RBIF 49
PIR1 PSPIF(1) ADIF RCIF TXIF SSPIF CCP1IF TMR2IF TMR1IF 52
PIE1 PSPIE(1) ADIE RCIE TXIE SSPIE CCP1IE TMR2IE TMR1IE 52
IPR1 PSPIP(1) ADIP RCIP TXIP SSPIP CCP1IP TMR2IP TMR1IP 52
RCSTA SPEN RX9 SREN CREN ADDEN FERR OERR RX9D 51
RCREG EUSART Receive Register 51
TXSTA CSRC TX9 TXEN SYNC SENDB BRGH TRMT TX9D 51
BAUDCON ABDOVF RCIDL — SCKP BRG16 — WUE ABDEN 51
SPBRGH EUSART Baud Rate Generator Register, High Byte 51
SPBRG EUSART Baud Rate Generator Register, Low Byte 51
Legend: — = unimplemented, read as ‘0’. Shaded cells are not used for synchronous slave reception.
Note 1: Reserved in 28-pin devices; always maintain these bits clear.
PIC18F2420/2520/4420/4520
DS39631B-page 222 Preliminary © 2007 Microchip Technology Inc.
NOTES:
© 2007 Microchip Technology Inc. Preliminary DS39631B-page 223
PIC18F2420/2520/4420/4520
19.0 10-BIT ANALOG-TO-DIGITAL
CONVERTER (A/D) MODULE
The Analog-to-Digital (A/D) converter module has
10 inputs for the 28-pin devices and 13 for the 40/44-pin
devices. This module allows conversion of an analog
input signal to a corresponding 10-bit digital number.
The module has five registers:
• A/D Result High Register (ADRESH)
• A/D Result Low Register (ADRESL)
• A/D Control Register 0 (ADCON0)
• A/D Control Register 1 (ADCON1)
• A/D Control Register 2 (ADCON2)
The ADCON0 register, shown in Register 19-1,
controls the operation of the A/D module. The
ADCON1 register, shown in Register 19-2, configures
the functions of the port pins. The ADCON2 register,
shown in Register 19-3, configures the A/D clock
source, programmed acquisition time and justification.
REGISTER 19-1: ADCON0 REGISTER
U-0 U-0 R/W-0 R/W-0 R/W-0 R/W-0 R/W-0 R/W-0
— — CHS3 CHS2 CHS1 CHS0 GO/DONE ADON
bit 7 bit 0
bit 7-6 Unimplemented: Read as ‘0’
bit 5-2 CHS3:CHS0: Analog Channel Select bits
0000 = Channel 0 (AN0)
0001 = Channel 1 (AN1)
0010 = Channel 2 (AN2)
0011 = Channel 3 (AN3)
0100 = Channel 4 (AN4)
0101 = Channel 5 (AN5)(1,2)
0110 = Channel 6 (AN6)(1,2)
0111 = Channel 7 (AN7)(1,2)
1000 = Channel 8 (AN8)
1001 = Channel 9 (AN9)
1010 = Channel 10 (AN10)
1011 = Channel 11 (AN11)
1100 = Channel 12 (AN12
1101 = Unimplemented(2)
1110 = Unimplemented(2)
1111 = Unimplemented(2)
Note 1: These channels are not implemented on 28-pin devices.
2: Performing a conversion on unimplemented channels will return a floating input
measurement.
bit 1 GO/DONE: A/D Conversion Status bit
When ADON = 1:
1 = A/D conversion in progress
0 = A/D Idle
bit 0 ADON: A/D On bit
1 = A/D converter module is enabled
0 = A/D converter module is disabled
Legend:
R = Readable bit W = Writable bit U = Unimplemented bit, read as ‘0’
-n = Value at POR ‘1’ = Bit is set ‘0’ = Bit is cleared x = Bit is unknown
PIC18F2420/2520/4420/4520
DS39631B-page 224 Preliminary © 2007 Microchip Technology Inc.
REGISTER 19-2: ADCON1 REGISTER
U-0 U-0 R/W-0 R/W-0 R/W-0(1) R/W(1) R/W(1) R/W(1)
— — VCFG1 VCFG0 PCFG3 PCFG2 PCFG1 PCFG0
bit 7 bit 0
bit 7-6 Unimplemented: Read as ‘0’
bit 5 VCFG1: Voltage Reference Configuration bit (VREF- source)
1 = VREF- (AN2)
0 = VSS
bit 4 VCFG0: Voltage Reference Configuration bit (VREF+ source)
1 = VREF+ (AN3)
0 = VDD
bit 3-0 PCFG3:PCFG0: A/D Port Configuration Control bits:
Legend:
R = Readable bit W = Writable bit U = Unimplemented bit, read as ‘0’
-n = Value at POR ‘1’ = Bit is set ‘0’ = Bit is cleared x = Bit is unknown
A = Analog input D = Digital I/O
Note 1: The POR value of the PCFG bits depends on the value of the PBADEN configuration
bit. When PBADEN = 1, PCFG<3:0> = 0000; when PBADEN = 0,
PCFG<3:0> = 0111.
2: AN5 through AN7 are available only on 40/44-pin devices.
PCFG3:
PCFG0
AN12
AN11
AN10
AN9
AN8
AN7(2)
AN6(2)
AN5(2)
AN4
AN3
AN2
AN1
AN0
0000(1) A A A A A A A A A A A A A
0001 A A A A A A A A A A A A A
0010 A A A A A A A A A A A A A
0011 D A A A A A A A A A A A A
0100 D D A A A A A A A A A A A
0101 D D D A A A A A A A A A A
0110 D D D D A A A A A A A A A
0111(1) D D D D D A A A A A A A A
1000 D D D D D D A A A A A A A
1001 D D D D D D D A A A A A A
1010 D D D D D D D D A A A A A
1011 D D D D D D D D D A A A A
1100 D D D D D D D D D D A A A
1101 D D D D D D D D D D D A A
1110 D D D D D D D D D D D D A
1111 D D D D D D D D D D D D D
© 2007 Microchip Technology Inc. Preliminary DS39631B-page 225
PIC18F2420/2520/4420/4520
REGISTER 19-3: ADCON2 REGISTER
R/W-0 U-0 R/W-0 R/W-0 R/W-0 R/W-0 R/W-0 R/W-0
ADFM — ACQT2 ACQT1 ACQT0 ADCS2 ADCS1 ADCS0
bit 7 bit 0
bit 7 ADFM: A/D Result Format Select bit
1 = Right justified
0 = Left justified
bit 6 Unimplemented: Read as ‘0’
bit 5-3 ACQT2:ACQT0: A/D Acquisition Time Select bits
111 = 20 TAD
110 = 16 TAD
101 = 12 TAD
100 = 8 TAD
011 = 6 TAD
010 = 4 TAD
001 = 2 TAD
000 = 0 TAD(1)
bit 2-0 ADCS2:ADCS0: A/D Conversion Clock Select bits
111 = FRC (clock derived from A/D RC oscillator)(1)
110 = FOSC/64
101 = FOSC/16
100 = FOSC/4
011 = FRC (clock derived from A/D RC oscillator)(1)
010 = FOSC/32
001 = FOSC/8
000 = FOSC/2
Note 1: If the A/D FRC clock source is selected, a delay of one TCY (instruction cycle) is
added before the A/D clock starts. This allows the SLEEP instruction to be executed
before starting a conversion.
Legend:
R = Readable bit W = Writable bit U = Unimplemented bit, read as ‘0’
-n = Value at POR ‘1’ = Bit is set ‘0’ = Bit is cleared x = Bit is unknown
PIC18F2420/2520/4420/4520
DS39631B-page 226 Preliminary © 2007 Microchip Technology Inc.
The analog reference voltage is software selectable to
either the device’s positive and negative supply voltage
(VDD and VSS), or the voltage level on the RA3/AN3/
VREF+ and RA2/AN2/VREF-/CVREF pins.
The A/D converter has a unique feature of being able
to operate while the device is in Sleep mode. To operate
in Sleep, the A/D conversion clock must be derived
from the A/D’s internal RC oscillator.
The output of the sample and hold is the input into the
converter, which generates the result via successive
approximation.
A device Reset forces all registers to their Reset state.
This forces the A/D module to be turned off and any
conversion in progress is aborted.
Each port pin associated with the A/D converter can be
configured as an analog input, or as a digital I/O. The
ADRESH and ADRESL registers contain the result of
the A/D conversion. When the A/D conversion is complete,
the result is loaded into the ADRESH:ADRESL
register pair, the GO/DONE bit (ADCON0 register) is
cleared and A/D Interrupt Flag bit, ADIF, is set. The block
diagram of the A/D module is shown in Figure 19-1.
FIGURE 19-1: A/D BLOCK DIAGRAM
(Input Voltage)
VAIN
VREF+
Reference
Voltage
VDD
VCFG1:VCFG0
CHS3:CHS0
AN7(1)
AN6(1)
AN5(1)
AN4
AN3
AN2
AN1
AN0
0111
0110
0101
0100
0011
0010
0001
0000
10-Bit
Converter
VREFVSS
A/D
AN12
AN11
AN10
AN9
AN8
1100
1011
1010
1001
1000
Note 1: Channels AN5 through AN7 are not available on 28-pin devices.
2: I/O pins have diode protection to VDD and VSS.
0X
1X
X1
X0
© 2007 Microchip Technology Inc. Preliminary DS39631B-page 227
PIC18F2420/2520/4420/4520
The value in the ADRESH:ADRESL registers is not
modified for a Power-on Reset. The ADRESH:ADRESL
registers will contain unknown data after a Power-on
Reset.
After the A/D module has been configured as desired,
the selected channel must be acquired before the
conversion is started. The analog input channels must
have their corresponding TRIS bits selected as an
input. To determine acquisition time, see Section 19.1
“A/D Acquisition Requirements”. After this acquisition
time has elapsed, the A/D conversion can be
started. An acquisition time can be programmed to
occur between setting the GO/DONE bit and the actual
start of the conversion.
The following steps should be followed to perform an A/D
conversion:
1. Configure the A/D module:
• Configure analog pins, voltage reference and
digital I/O (ADCON1)
• Select A/D input channel (ADCON0)
• Select A/D acquisition time (ADCON2)
• Select A/D conversion clock (ADCON2)
• Turn on A/D module (ADCON0)
2. Configure A/D interrupt (if desired):
• Clear ADIF bit
• Set ADIE bit
• Set GIE bit
3. Wait the required acquisition time (if required).
4. Start conversion:
• Set GO/DONE bit (ADCON0 register)
5. Wait for A/D conversion to complete, by either:
• Polling for the GO/DONE bit to be cleared
OR
• Waiting for the A/D interrupt
6. Read A/D Result registers (ADRESH:ADRESL);
clear bit ADIF, if required.
7. For next conversion, go to step 1 or step 2, as
required. The A/D conversion time per bit is
defined as TAD. A minimum wait of 2 TAD is
required before the next acquisition starts.
FIGURE 19-2: A/D TRANSFER FUNCTION
FIGURE 19-3: ANALOG INPUT MODEL
Digital Code Output
3FEh
003h
002h
001h
000h
0.5 LSB
1 LSB
1.5 LSB
2 LSB
2.5 LSB
1022 LSB
1022.5 LSB
3 LSB
Analog Input Voltage
3FFh
1023 LSB
1023.5 LSB
VAIN CPIN
Rs ANx
5 pF
VT = 0.6V
VT = 0.6V
ILEAKAGE
RIC ≤ 1k
Sampling
Switch
SS RSS
CHOLD = 25 pF
VSS
VDD
± 100 nA
Legend: CPIN
VT
ILEAKAGE
RIC
SS
CHOLD
= input capacitance
= threshold voltage
= leakage current at the pin due to
= interconnect resistance
= sampling switch
= sample/hold capacitance (from DAC)
various junctions
RSS = sampling switch resistance
VDD
6 V
Sampling Switch
5 V
4 V
3 V
2 V
1 2 3 4
(kΩ)
PIC18F2420/2520/4420/4520
DS39631B-page 228 Preliminary © 2007 Microchip Technology Inc.
19.1 A/D Acquisition Requirements
For the A/D converter to meet its specified accuracy,
the charge holding capacitor (CHOLD) must be allowed
to fully charge to the input channel voltage level. The
analog input model is shown in Figure 19-3. The
source impedance (RS) and the internal sampling
switch (RSS) impedance directly affect the time
required to charge the capacitor CHOLD. The sampling
switch (RSS) impedance varies over the device voltage
(VDD). The source impedance affects the offset voltage
at the analog input (due to pin leakage current). The
maximum recommended impedance for analog
sources is 2.5 kΩ. After the analog input channel is
selected (changed), the channel must be sampled for
at least the minimum acquisition time before starting a
conversion.
To calculate the minimum acquisition time,
Equation 19-1 may be used. This equation assumes
that 1/2 LSb error is used (1024 steps for the A/D). The
1/2 LSb error is the maximum error allowed for the A/D
to meet its specified resolution.
Example 19-3 shows the calculation of the minimum
required acquisition time TACQ. This calculation is
based on the following application system
assumptions:
CHOLD = 25 pF
Rs = 2.5 kΩ
Conversion Error ≤ 1/2 LSb
VDD = 5V → Rss = 2 kΩ
Temperature = 85°C (system max.)
EQUATION 19-1: ACQUISITION TIME
EQUATION 19-2: A/D MINIMUM CHARGING TIME
EQUATION 19-3: CALCULATING THE MINIMUM REQUIRED ACQUISITION TIME
Note: When the conversion is started, the
holding capacitor is disconnected from the
input pin.
TACQ = Amplifier Settling Time + Holding Capacitor Charging Time + Temperature Coefficient
= TAMP + TC + TCOFF
VHOLD = (VREF – (VREF/2048)) • (1 – e(-TC/CHOLD(RIC + RSS + RS)))
or
TC = -(CHOLD)(RIC + RSS + RS) ln(1/2048)
TACQ = TAMP + TC + TCOFF
TAMP = 0.2 μs
TCOFF = (Temp – 25°C)(0.02 μs/°C)
(85°C – 25°C)(0.02 μs/°C)
1.2 μs
Temperature coefficient is only required for temperatures > 25°C. Below 25°C, TCOFF = 0 ms.
TC = -(CHOLD)(RIC + RSS + RS) ln(1/2047) μs
-(25 pF) (1 kΩ + 2 kΩ + 2.5 kΩ) ln(0.0004883) μs
1.05 μs
TACQ = 0.2 μs + 1 μs + 1.2 μs
2.4 μs
© 2007 Microchip Technology Inc. Preliminary DS39631B-page 229
PIC18F2420/2520/4420/4520
19.2 Selecting and Configuring
Acquisition Time
The ADCON2 register allows the user to select an
acquisition time that occurs each time the GO/DONE
bit is set. It also gives users the option to use an
automatically determined acquisition time.
Acquisition time may be set with the ACQT2:ACQT0
bits (ADCON2<5:3>), which provides a range of 2 to
20 TAD. When the GO/DONE bit is set, the A/D module
continues to sample the input for the selected acquisition
time, then automatically begins a conversion.
Since the acquisition time is programmed, there may
be no need to wait for an acquisition time between
selecting a channel and setting the GO/DONE bit.
Manual acquisition is selected when
ACQT2:ACQT0 = 000. When the GO/DONE bit is set,
sampling is stopped and a conversion begins. The user
is responsible for ensuring the required acquisition time
has passed between selecting the desired input
channel and setting the GO/DONE bit. This option is
also the default Reset state of the ACQT2:ACQT0 bits
and is compatible with devices that do not offer
programmable acquisition times.
In either case, when the conversion is completed, the
GO/DONE bit is cleared, the ADIF flag is set and the
A/D begins sampling the currently selected channel
again. If an acquisition time is programmed, there is
nothing to indicate if the acquisition time has ended or
if the conversion has begun.
19.3 Selecting the A/D Conversion
Clock
The A/D conversion time per bit is defined as TAD. The
A/D conversion requires 11 TAD per 10-bit conversion.
The source of the A/D conversion clock is software
selectable. There are seven possible options for TAD:
• 2 TOSC
• 4 TOSC
• 8 TOSC
• 16 TOSC
• 32 TOSC
• 64 TOSC
• Internal RC Oscillator
For correct A/D conversions, the A/D conversion clock
(TAD) must be as short as possible, but greater than the
minimum TAD (see parameter 130 for more
information).
Table 19-1 shows the resultant TAD times derived from
the device operating frequencies and the A/D clock
source selected.
TABLE 19-1: TAD vs. DEVICE OPERATING FREQUENCIES
AD Clock Source (TAD) Maximum Device Frequency
Operation ADCS2:ADCS0 PIC18F2X20/4X20 PIC18LF2X20/4X20(4)
2 TOSC 000 2.86 MHz 1.43 kHz
4 TOSC 100 5.71 MHz 2.86 MHz
8 TOSC 001 11.43 MHz 5.72 MHz
16 TOSC 101 22.86 MHz 11.43 MHz
32 TOSC 010 40.0 MHz 22.86 MHz
64 TOSC 110 40.0 MHz 22.86 MHz
RC(3) x11 1.00 MHz(1) 1.00 MHz(2)
Note 1: The RC source has a typical TAD time of 1.2 μs.
2: The RC source has a typical TAD time of 2.5 μs.
3: For device frequencies above 1 MHz, the device must be in Sleep for the entire conversion or the A/D
accuracy may be out of specification.
4: Low-power (PIC18LFXXXX) devices only.
PIC18F2420/2520/4420/4520
DS39631B-page 230 Preliminary © 2007 Microchip Technology Inc.
19.4 Operation in Power Managed
Modes
The selection of the automatic acquisition time and A/D
conversion clock is determined in part by the clock
source and frequency while in a power managed mode.
If the A/D is expected to operate while the device is in
a power managed mode, the ACQT2:ACQT0 and
ADCS2:ADCS0 bits in ADCON2 should be updated in
accordance with the clock source to be used in that
mode. After entering the mode, an A/D acquisition or
conversion may be started. Once started, the device
should continue to be clocked by the same clock
source until the conversion has been completed.
If desired, the device may be placed into the
corresponding Idle mode during the conversion. If the
device clock frequency is less than 1 MHz, the A/D RC
clock source should be selected.
Operation in the Sleep mode requires the A/D FRC
clock to be selected. If bits ACQT2:ACQT0 are set to
‘000’ and a conversion is started, the conversion will be
delayed one instruction cycle to allow execution of the
SLEEP instruction and entry to Sleep mode. The IDLEN
bit (OSCCON<7>) must have already been cleared
prior to starting the conversion.
19.5 Configuring Analog Port Pins
The ADCON1, TRISA, TRISB and TRISE registers all
configure the A/D port pins. The port pins needed as
analog inputs must have their corresponding TRIS bits
set (input). If the TRIS bit is cleared (output), the digital
output level (VOH or VOL) will be converted.
The A/D operation is independent of the state of the
CHS3:CHS0 bits and the TRIS bits.
Note 1: When reading the Port register, all pins
configured as analog input channels will
read as cleared (a low level). Pins configured
as digital inputs will convert as
analog inputs. Analog levels on a digitally
configured input will be accurately
converted.
2: Analog levels on any pin defined as a digital
input may cause the digital input buffer
to consume current out of the device’s
specification limits.
3: The PBADEN bit in Configuration
Register 3H configures PORTB pins to
reset as analog or digital pins by controlling
how the PCFG0 bits in ADCON1 are
reset.
© 2007 Microchip Technology Inc. Preliminary DS39631B-page 231
PIC18F2420/2520/4420/4520
19.6 A/D Conversions
Figure 19-4 shows the operation of the A/D converter
after the GO bit has been set and the ACQT2:ACQT0
bits are cleared. A conversion is started after the following
instruction to allow entry into Sleep mode before the
conversion begins.
Figure 19-5 shows the operation of the A/D converter
after the GO bit has been set and the ACQT2:ACQT0
bits are set to ‘010’ and selecting a 4 TAD acquisition
time before the conversion starts.
Clearing the GO/DONE bit during a conversion will abort
the current conversion. The A/D Result register pair will
NOT be updated with the partially completed A/D
conversion sample. This means the ADRESH:ADRESL
registers will continue to contain the value of the last
completed conversion (or the last value written to the
ADRESH:ADRESL registers).
After the A/D conversion is completed or aborted, a
2 TAD wait is required before the next acquisition can
be started. After this wait, acquisition on the selected
channel is automatically started.
19.7 Discharge
The discharge phase is used to initialize the value of
the capacitor array. The array is discharged before
every sample. This feature helps to optimize the unitygain
amplifier, as the circuit always needs to charge the
capacitor array, rather than charge/discharge based on
previous measure values.
FIGURE 19-4: A/D CONVERSION TAD CYCLES (ACQT<2:0> = 000, TACQ = 0)
FIGURE 19-5: A/D CONVERSION TAD CYCLES (ACQT<2:0> = 010, TACQ = 4 TAD)
Note: The GO/DONE bit should NOT be set in
the same instruction that turns on the A/D.
TAD1 TAD2 TAD3 TAD4 TAD5 TAD6 TAD7 TAD8 TAD11
Set GO bit
Holding capacitor is disconnected from analog input (typically 100 ns)
TCY - TAD TAD9 TAD10
ADRESH:ADRESL is loaded, GO bit is cleared,
ADIF bit is set, holding capacitor is connected to analog input.
Conversion starts
b9 b8 b7 b6 b5 b4 b3 b2 b1 b0
On the following cycle:
TAD1
Discharge
1 2 3 4 5 6 7 8 11
Set GO bit
(Holding capacitor is disconnected)
9 10
Conversion starts
1 2 3 4
(Holding capacitor continues
acquiring input)
TACQT Cycles TAD Cycles
Automatic
Acquisition
Time
b9 b8 b7 b6 b5 b4 b3 b2 b1 b0
ADRESH:ADRESL is loaded, GO bit is cleared,
ADIF bit is set, holding capacitor is connected to analog input.
On the following cycle:
TAD1
Discharge
PIC18F2420/2520/4420/4520
DS39631B-page 232 Preliminary © 2007 Microchip Technology Inc.
19.8 Use of the CCP2 Trigger
An A/D conversion can be started by the Special Event
Trigger of the CCP2 module. This requires that the
CCP2M3:CCP2M0 bits (CCP2CON<3:0>) be
programmed as ‘1011’ and that the A/D module is
enabled (ADON bit is set). When the trigger occurs, the
GO/DONE bit will be set, starting the A/D acquisition
and conversion and the Timer1 (or Timer3) counter will
be reset to zero. Timer1 (or Timer3) is reset to automatically
repeat the A/D acquisition period with minimal
software overhead (moving ADRESH:ADRESL to the
desired location). The appropriate analog input channel
must be selected and the minimum acquisition
period is either timed by the user, or an appropriate
TACQ time selected before the Special Event Trigger
sets the GO/DONE bit (starts a conversion).
If the A/D module is not enabled (ADON is cleared), the
Special Event Trigger will be ignored by the A/D
module, but will still reset the Timer1 (or Timer3)
counter.
TABLE 19-2: REGISTERS ASSOCIATED WITH A/D OPERATION
Name Bit 7 Bit 6 Bit 5 Bit 4 Bit 3 Bit 2 Bit 1 Bit 0
Reset
Values
on page
INTCON GIE/GIEH PEIE/GIEL TMR0IE INT0IE RBIE TMR0IF INT0IF RBIF 49
PIR1 PSPIF(1) ADIF RCIF TXIF SSPIF CCP1IF TMR2IF TMR1IF 52
PIE1 PSPIE(1) ADIE RCIE TXIE SSPIE CCP1IE TMR2IE TMR1IE 52
IPR1 PSPIP(1) ADIP RCIP TXIP SSPIP CCP1IP TMR2IP TMR1IP 52
PIR2 OSCFIF CMIF — EEIF BCLIF HLVDIF TMR3IF CCP2IF 52
PIE2 OSCFIE CMIE — EEIE BCLIE HLVDIE TMR3IE CCP2IE 52
IPR2 OSCFIP CMIP — EEIP BCLIP HLVDIP TMR3IP CCP2IP 52
ADRESH A/D Result Register, High Byte 51
ADRESL A/D Result Register, Low Byte 51
ADCON0 — — CHS3 CHS2 CHS1 CHS0 GO/DONE ADON 51
ADCON1 — — VCFG1 VCFG0 PCFG3 PCFG2 PCFG1 PCFG0 51
ADCON2 ADFM — ACQT2 ACQT1 ACQT0 ADCS2 ADCS1 ADCS0 51
PORTA RA7(1) RA6(1) RA5 RA4 RA3 RA2 RA1 RA0 52
TRISA TRISA7(2) TRISA6(2) PORTA Data Direction Control Register 52
PORTB RB7 RB6 RB5 RB4 RB3 RB2 RB1 RB0 52
TRISB PORTB Data Direction Control Register 52
LATB PORTB Data Latch Register (Read and Write to Data Latch) 52
PORTE(4) — — — — RE3(3) RE2 RE1 RE0 52
TRISE(4) IBF OBF IBOV PSPMODE — TRISE2 TRISE1 TRISE0 52
LATE(4) — — — — — PORTE Data Latch Register 52
Legend: — = unimplemented, read as ‘0’. Shaded cells are not used for A/D conversion.
Note 1: These bits are unimplemented on 28-pin devices; always maintain these bits clear.
2: PORTA<7:6> and their direction bits are individually configured as port pins based on various primary
oscillator modes. When disabled, these bits read as ‘0’.
3: RE3 port bit is available only as an input pin when the MCLRE configuration bit is ‘0’.
4: These registers are not implemented on 28-pin devices.
© 2007 Microchip Technology Inc. Preliminary DS39631B-page 233
PIC18F2420/2520/4420/4520
20.0 COMPARATOR MODULE
The analog comparator module contains two
comparators that can be configured in a variety of
ways. The inputs can be selected from the analog
inputs multiplexed with pins RA0 through RA5, as well
as the on-chip voltage reference (see Section 21.0
“Comparator Voltage Reference Module”). The digital
outputs (normal or inverted) are available at the pin
level and can also be read through the control register.
The CMCON register (Register 20-1) selects the
comparator input and output configuration. Block
diagrams of the various comparator configurations are
shown in Figure 20-1.
REGISTER 20-1: CMCON REGISTER
R-0 R-0 R/W-0 R/W-0 R/W-0 R/W-1 R/W-1 R/W-1
C2OUT C1OUT C2INV C1INV CIS CM2 CM1 CM0
bit 7 bit 0
bit 7 C2OUT: Comparator 2 Output bit
When C2INV = 0:
1 = C2 VIN+ > C2 VIN-
0 = C2 VIN+ < C2 VINWhen
C2INV = 1:
1 = C2 VIN+ < C2 VIN-
0 = C2 VIN+ > C2 VINbit
6 C1OUT: Comparator 1 Output bit
When C1INV = 0:
1 = C1 VIN+ > C1 VIN-
0 = C1 VIN+ < C1 VINWhen
C1INV = 1:
1 = C1 VIN+ < C1 VIN-
0 = C1 VIN+ > C1 VINbit
5 C2INV: Comparator 2 Output Inversion bit
1 = C2 output inverted
0 = C2 output not inverted
bit 4 C1INV: Comparator 1 Output Inversion bit
1 = C1 output inverted
0 = C1 output not inverted
bit 3 CIS: Comparator Input Switch bit
When CM2:CM0 = 110:
1 = C1 VIN- connects to RA3/AN3/VREF+
C2 VIN- connects to RA2/AN2/VREF-/CVREF
0 = C1 VIN- connects to RA0/AN0
C2 VIN- connects to RA1/AN1
bit 2-0 CM2:CM0: Comparator Mode bits
Figure 20-1 shows the Comparator modes and the CM2:CM0 bit settings.
Legend:
R = Readable bit W = Writable bit U = Unimplemented bit, read as ‘0’
-n = Value at POR ‘1’ = Bit is set ‘0’ = Bit is cleared x = Bit is unknown
PIC18F2420/2520/4420/4520
DS39631B-page 234 Preliminary © 2007 Microchip Technology Inc.
20.1 Comparator Configuration
There are eight modes of operation for the comparators,
shown in Figure 20-1. Bits CM2:CM0 of the
CMCON register are used to select these modes. The
TRISA register controls the data direction of the comparator
pins for each mode. If the Comparator mode is
changed, the comparator output level may not be valid
for the specified mode change delay shown in
Section 26.0 “Electrical Characteristics”.
FIGURE 20-1: COMPARATOR I/O OPERATING MODES
Note: Comparator interrupts should be disabled
during a Comparator mode change;
otherwise, a false interrupt may occur.
C1
RA0/AN0 VINRA3/
AN3/ VIN+
Off (Read as ‘0’)
Comparators Reset
A
A
CM2:CM0 = 000
C2
RA1/AN1 VINRA2/
AN2/ VIN+
Off (Read as ‘0’)
A
A
C1
VINVIN+
C1OUT
Two Independent Comparators
A
A
CM2:CM0 = 010
C2
VINVIN+
C2OUT
A
A
C1
VINVIN+
C1OUT
Two Common Reference Comparators
A
A
CM2:CM0 = 100
C2
VINVIN+
C2OUT
A
D
C2
VINVIN+
Off (Read as ‘0’)
One Independent Comparator with Output
D
D
CM2:CM0 = 001
C1
VINVIN+
C1OUT
A
A
C1
VINVIN+
Off (Read as ‘0’)
Comparators Off (POR Default Value)
D
D
CM2:CM0 = 111
C2
VINVIN+
Off (Read as ‘0’)
D
D
C1
VINVIN+
C1OUT
Four Inputs Multiplexed to Two Comparators
A
A
CM2:CM0 = 110
C2
VINVIN+
C2OUT
A
A
From VREF Module
CIS = 0
CIS = 1
CIS = 0
CIS = 1
C1
VINVIN+
C1OUT
Two Common Reference Comparators with Outputs
A
A
CM2:CM0 = 101
C2
VINVIN+
C2OUT
A
D
A = Analog Input, port reads zeros always D = Digital Input CIS (CMCON<3>) is the Comparator Input Switch
CVREF
C1
VINVIN+
C1OUT
Two Independent Comparators with Outputs
A
A
CM2:CM0 = 011
C2
VINVIN+
C2OUT
A
A
RA5/AN4/SS/HLVDIN/C2OUT*
RA4/T0CKI/C1OUT*
VREF+
VREF-/CVREF
RA0/AN0
RA3/AN3/
RA1/AN1
RA2/AN2/
VREF+
VREF-/CVREF
RA0/AN0
RA3/AN3/
RA1/AN1
RA2/AN2/
VREF+
VREF-/CVREF
RA0/AN0
RA3/AN3/
RA1/AN1
RA2/AN2/
VREF+
VREF-/CVREF
RA0/AN0
RA3/AN3/
RA1/AN1
RA2/AN2/
VREF+
VREF-/CVREF
RA0/AN0
RA3/AN3/
RA1/AN1
RA2/AN2/
VREF+
VREF-/CVREF
RA0/AN0
RA3/AN3/
VREF+
RA1/AN1
RA2/AN2/
VREF-/CVREF
RA4/T0CKI/C1OUT*
RA5/AN4/SS/HLVDIN/C2OUT*
RA0/AN0
RA3/AN3/
VREF+
RA1/AN1
RA2/AN2/
VREF-/CVREF
RA4/T0CKI/C1OUT*
* Setting the TRISA<5:4> bits will disable the comparator outputs by configuring the pins as inputs.
© 2007 Microchip Technology Inc. Preliminary DS39631B-page 235
PIC18F2420/2520/4420/4520
20.2 Comparator Operation
A single comparator is shown in Figure 20-2, along with
the relationship between the analog input levels and
the digital output. When the analog input at VIN+ is less
than the analog input VIN-, the output of the comparator
is a digital low level. When the analog input at VIN+ is
greater than the analog input VIN-, the output of the
comparator is a digital high level. The shaded areas of
the output of the comparator in Figure 20-2 represent
the uncertainty, due to input offsets and response time.
20.3 Comparator Reference
Depending on the comparator operating mode, either
an external or internal voltage reference may be used.
The analog signal present at VIN- is compared to the
signal at VIN+ and the digital output of the comparator
is adjusted accordingly (Figure 20-2).
FIGURE 20-2: SINGLE COMPARATOR
20.3.1 EXTERNAL REFERENCE SIGNAL
When external voltage references are used, the
comparator module can be configured to have the comparators
operate from the same or different reference
sources. However, threshold detector applications may
require the same reference. The reference signal must
be between VSS and VDD and can be applied to either
pin of the comparator(s).
20.3.2 INTERNAL REFERENCE SIGNAL
The comparator module also allows the selection of an
internally generated voltage reference from the
comparator voltage reference module. This module is
described in more detail in Section 21.0 “Comparator
Voltage Reference Module”.
The internal reference is only available in the mode
where four inputs are multiplexed to two comparators
(CM2:CM0 = 110). In this mode, the internal voltage
reference is applied to the VIN+ pin of both
comparators.
20.4 Comparator Response Time
Response time is the minimum time, after selecting a
new reference voltage or input source, before the
comparator output has a valid level. If the internal reference
is changed, the maximum delay of the internal
voltage reference must be considered when using the
comparator outputs. Otherwise, the maximum delay of
the comparators should be used (see Section 26.0
“Electrical Characteristics”).
20.5 Comparator Outputs
The comparator outputs are read through the CMCON
register. These bits are read-only. The comparator
outputs may also be directly output to the RA4 and RA5
I/O pins. When enabled, multiplexors in the output path
of the RA4 and RA5 pins will switch and the output of
each pin will be the unsynchronized output of the
comparator. The uncertainty of each of the
comparators is related to the input offset voltage and
the response time given in the specifications.
Figure 20-3 shows the comparator output block
diagram.
The TRISA bits will still function as an output enable/
disable for the RA4 and RA5 pins while in this mode.
The polarity of the comparator outputs can be changed
using the C2INV and C1INV bits (CMCON<4:5>).
–
VIN+ +
VINOutput
Output
VINVIN+
Note 1: When reading the Port register, all pins
configured as analog inputs will read as a
‘0’. Pins configured as digital inputs will
convert an analog input according to the
Schmitt Trigger input specification.
2: Analog levels on any pin defined as a
digital input may cause the input buffer to
consume more current than is specified.
PIC18F2420/2520/4420/4520
DS39631B-page 236 Preliminary © 2007 Microchip Technology Inc.
FIGURE 20-3: COMPARATOR OUTPUT BLOCK DIAGRAM
20.6 Comparator Interrupts
The comparator interrupt flag is set whenever there is
a change in the output value of either comparator.
Software will need to maintain information about the
status of the output bits, as read from CMCON<7:6>, to
determine the actual change that occurred. The CMIF
bit (PIR2<6>) is the Comparator Interrupt Flag. The
CMIF bit must be reset by clearing it. Since it is also
possible to write a ‘1’ to this register, a simulated
interrupt may be initiated.
Both the CMIE bit (PIE2<6>) and the PEIE bit
(INTCON<6>) must be set to enable the interrupt. In
addition, the GIE bit (INTCON<7>) must also be set. If
any of these bits are clear, the interrupt is not enabled,
though the CMIF bit will still be set if an interrupt
condition occurs.
The user, in the Interrupt Service Routine, can clear the
interrupt in the following manner:
a) Any read or write of CMCON will end the
mismatch condition.
b) Clear flag bit CMIF.
A mismatch condition will continue to set flag bit CMIF.
Reading CMCON will end the mismatch condition and
allow flag bit CMIF to be cleared.
20.7 Comparator Operation
During Sleep
When a comparator is active and the device is placed
in Sleep mode, the comparator remains active and the
interrupt is functional if enabled. This interrupt will
wake-up the device from Sleep mode, when enabled.
Each operational comparator will consume additional
current, as shown in the comparator specifications. To
minimize power consumption while in Sleep mode, turn
off the comparators (CM2:CM0 = 111) before entering
Sleep. If the device wakes up from Sleep, the contents
of the CMCON register are not affected.
20.8 Effects of a Reset
A device Reset forces the CMCON register to its Reset
state, causing the comparator modules to be turned off
(CM2:CM0 = 111). However, the input pins (RA0
through RA3) are configured as analog inputs by
default on device Reset. The I/O configuration for these
pins is determined by the setting of the PCFG3:PCFG0
bits (ADCON1<3:0>). Therefore, device current is
minimized when analog inputs are present at Reset
time.
D Q
EN
To RA4 or
RA5 pin
Bus
Data
Set
MULTIPLEX
CMIF
bit
- +
Port pins
Read CMCON
Reset
From
other
Comparator
CxINV
D Q
EN CL
Note: If a change in the CMCON register
(C1OUT or C2OUT) should occur when a
read operation is being executed (start of
the Q2 cycle), then the CMIF (PIR
registers) interrupt flag may not get set.
© 2007 Microchip Technology Inc. Preliminary DS39631B-page 237
PIC18F2420/2520/4420/4520
20.9 Analog Input Connection
Considerations
A simplified circuit for an analog input is shown in
Figure 20-4. Since the analog pins are connected to a
digital output, they have reverse biased diodes to VDD
and VSS. The analog input, therefore, must be between
VSS and VDD. If the input voltage deviates from this
range by more than 0.6V in either direction, one of the
diodes is forward biased and a latch-up condition may
occur. A maximum source impedance of 10 kΩ is
recommended for the analog sources. Any external
component connected to an analog input pin, such as
a capacitor or a Zener diode, should have very little
leakage current.
FIGURE 20-4: COMPARATOR ANALOG INPUT MODEL
TABLE 20-1: REGISTERS ASSOCIATED WITH COMPARATOR MODULE
VA
RS < 10k
AIN
CPIN
5 pF
VDD
VT = 0.6V
VT = 0.6V
RIC
ILEAKAGE
±500 nA
VSS
Legend: CPIN = Input Capacitance
VT = Threshold Voltage
ILEAKAGE = Leakage Current at the pin due to various junctions
RIC = Interconnect Resistance
RS = Source Impedance
VA = Analog Voltage
Comparator
Input
Name Bit 7 Bit 6 Bit 5 Bit 4 Bit 3 Bit 2 Bit 1 Bit 0
Reset
Values
on page
CMCON C2OUT C1OUT C2INV C1INV CIS CM2 CM1 CM0 51
CVRCON CVREN CVROE CVRR CVRSS CVR3 CVR2 CVR1 CVR0 51
INTCON GIE/GIEH PEIE/GIEL TMR0IE INT0IE RBIE TMR0IF INT0IF RBIF 52
PIR2 OSCFIF CMIF — EEIF BCLIF HLVDIF TMR3IF CCP2IF 52
PIE2 OSCFIE CMIE — EEIE BCLIE HLVDIE TMR3IE CCP2IE 52
IPR2 OSCFIP CMIP — EEIP BCLIP HLVDIP TMR3IP CCP2IP 52
PORTA RA7(1) RA6(1) RA5 RA4 RA3 RA2 RA1 RA0 52
LATA LATA7(1) LATA6(1) PORTA Data Latch Register (Read and Write to Data Latch) 52
TRISA TRISA7(1) TRISA6(1) PORTA Data Direction Control Register 52
Legend: — = unimplemented, read as ‘0’. Shaded cells are unused by the comparator module.
Note 1: PORTA<7:6> and their direction and latch bits are individually configured as port pins based on various
primary oscillator modes. When disabled, these bits read as ‘0’.
PIC18F2420/2520/4420/4520
DS39631B-page 238 Preliminary © 2007 Microchip Technology Inc.
NOTES:
© 2007 Microchip Technology Inc. Preliminary DS39631B-page 239
PIC18F2420/2520/4420/4520
21.0 COMPARATOR VOLTAGE
REFERENCE MODULE
The comparator voltage reference is a 16-tap resistor
ladder network that provides a selectable reference
voltage. Although its primary purpose is to provide a
reference for the analog comparators, it may also be
used independently of them.
A block diagram of the module is shown in Figure 21-1.
The resistor ladder is segmented to provide two ranges
of CVREF values and has a power-down function to
conserve power when the reference is not being used.
The module’s supply reference can be provided from
either device VDD/VSS or an external voltage reference.
21.1 Configuring the Comparator
Voltage Reference
The voltage reference module is controlled through the
CVRCON register (Register 21-1). The comparator
voltage reference provides two ranges of output voltage,
each with 16 distinct levels. The range to be used
is selected by the CVRR bit (CVRCON<5>). The primary
difference between the ranges is the size of the
steps selected by the CVREF Selection bits
(CVR3:CVR0), with one range offering finer resolution.
The equations used to calculate the output of the
comparator voltage reference are as follows:
If CVRR = 1:
CVREF = ((CVR3:CVR0)/24) x CVRSRC
If CVRR = 0:
CVREF = (CVRSRC x 1/4) + (((CVR3:CVR0)/32) x
CVRSRC)
The comparator reference supply voltage can come
from either VDD and VSS, or the external VREF+ and
VREF- that are multiplexed with RA2 and RA3. The
voltage source is selected by the CVRSS bit
(CVRCON<4>).
The settling time of the comparator voltage reference
must be considered when changing the CVREF output
(see Table 26-3 in Section 26.0 “Electrical
Characteristics”).
REGISTER 21-1: CVRCON REGISTER
R/W-0 R/W-0 R/W-0 R/W-0 R/W-0 R/W-0 R/W-0 R/W-0
CVREN CVROE(1) CVRR CVRSS CVR3 CVR2 CVR1 CVR0
bit 7 bit 0
bit 7 CVREN: Comparator Voltage Reference Enable bit
1 = CVREF circuit powered on
0 = CVREF circuit powered down
bit 6 CVROE: Comparator VREF Output Enable bit(1)
1 = CVREF voltage level is also output on the RA2/AN2/VREF-/CVREF pin
0 = CVREF voltage is disconnected from the RA2/AN2/VREF-/CVREF pin
Note 1: CVROE overrides the TRISA<2> bit setting.
bit 5 CVRR: Comparator VREF Range Selection bit
1 = 0 to 0.667 CVRSRC, with CVRSRC/24 step size (low range)
0 = 0.25 CVRSRC to 0.75 CVRSRC, with CVRSRC/32 step size (high range)
bit 4 CVRSS: Comparator VREF Source Selection bit
1 = Comparator reference source, CVRSRC = (VREF+) – (VREF-)
0 = Comparator reference source, CVRSRC = VDD – VSS
bit 3-0 CVR3:CVR0: Comparator VREF Value Selection bits (0 ≤ (CVR3:CVR0) ≤ 15)
When CVRR = 1:
CVREF = ((CVR3:CVR0)/24) • (CVRSRC)
When CVRR = 0:
CVREF = (CVRSRC/4) + ((CVR3:CVR0)/32) • (CVRSRC)
Legend:
R = Readable bit W = Writable bit U = Unimplemented bit, read as ‘0’
-n = Value at POR ‘1’ = Bit is set ‘0’ = Bit is cleared x = Bit is unknown
PIC18F2420/2520/4420/4520
DS39631B-page 240 Preliminary © 2007 Microchip Technology Inc.
FIGURE 21-1: VOLTAGE REFERENCE BLOCK DIAGRAM
21.2 Voltage Reference Accuracy/Error
The full range of voltage reference cannot be realized
due to the construction of the module. The transistors
on the top and bottom of the resistor ladder network
(Figure 21-1) keep CVREF from approaching the reference
source rails. The voltage reference is derived
from the reference source; therefore, the CVREF output
changes with fluctuations in that source. The tested
absolute accuracy of the voltage reference can be
found in Section 26.0 “Electrical Characteristics”.
21.3 Operation During Sleep
When the device wakes up from Sleep through an
interrupt or a Watchdog Timer time-out, the contents of
the CVRCON register are not affected. To minimize
current consumption in Sleep mode, the voltage
reference should be disabled.
21.4 Effects of a Reset
A device Reset disables the voltage reference by
clearing bit, CVREN (CVRCON<7>). This Reset also
disconnects the reference from the RA2 pin by clearing
bit, CVROE (CVRCON<6>) and selects the high-voltage
range by clearing bit, CVRR (CVRCON<5>). The CVR
value select bits are also cleared.
21.5 Connection Considerations
The voltage reference module operates independently
of the comparator module. The output of the reference
generator may be connected to the RA2 pin if the
CVROE bit is set. Enabling the voltage reference output
onto RA2 when it is configured as a digital input will
increase current consumption. Connecting RA2 as a
digital output with CVRSS enabled will also increase
current consumption.
The RA2 pin can be used as a simple D/A output with
limited drive capability. Due to the limited current drive
capability, a buffer must be used on the voltage
reference output for external connections to VREF.
Figure 21-2 shows an example buffering technique.
16-to-1 MUX
CVR3:CVR0
8R
CVREN R
CVRSS = 0
VDD
VREF+
CVRSS = 1
8R
CVRSS = 0
VREFCVRSS
= 1
R
R
R
R
R
R
16 Steps
CVRR
CVREF
© 2007 Microchip Technology Inc. Preliminary DS39631B-page 241
PIC18F2420/2520/4420/4520
FIGURE 21-2: VOLTAGE REFERENCE OUTPUT BUFFER EXAMPLE
TABLE 21-1: REGISTERS ASSOCIATED WITH COMPARATOR VOLTAGE REFERENCE
CVREF Output
+
–
CVREF
Module
Voltage
Reference
Output
Impedance
R(1)
RA2
Note 1: R is dependent upon the voltage reference configuration bits, CVRCON<3:0> and CVRCON<5>.
PIC18FXXXX
Name Bit 7 Bit 6 Bit 5 Bit 4 Bit 3 Bit 2 Bit 1 Bit 0
Reset
Values
on page
CVRCON CVREN CVROE CVRR CVRSS CVR3 CVR2 CVR1 CVR0 51
CMCON C2OUT C1OUT C2INV C1INV CIS CM2 CM1 CM0 51
TRISA TRISA7(1) TRISA6(1) PORTA Data Direction Control Register 52
Legend: Shaded cells are not used with the comparator voltage reference.
Note 1: PORTA pins are enabled based on oscillator configuration.
PIC18F2420/2520/4420/4520
DS39631B-page 242 Preliminary © 2007 Microchip Technology Inc.
NOTES:
© 2007 Microchip Technology Inc. DS39631B-page 243
PIC18F2420/2520/4420/4520
22.0 HIGH/LOW-VOLTAGE
DETECT (HLVD)
PIC18F2420/2520/4420/4520 devices have a
High/Low-Voltage Detect module (HLVD). This is a programmable
circuit that allows the user to specify both a
device voltage trip point and the direction of change from
that point. If the device experiences an excursion past
the trip point in that direction, an interrupt flag is set. If the
interrupt is enabled, the program execution will branch to
the interrupt vector address and the software can then
respond to the interrupt.
The High/Low-Voltage Detect Control register
(Register 22-1) completely controls the operation of the
HLVD module. This allows the circuitry to be “turned
off” by the user under software control, which
minimizes the current consumption for the device.
The block diagram for the HLVD module is shown in
Figure 22-1.
REGISTER 22-1: HLVDCON REGISTER (HIGH/LOW-VOLTAGE DETECT CONTROL)
R/W-0 U-0 R-0 R/W-0 R/W-0 R/W-1 R/W-0 R/W-1
VDIRMAG — IRVST HLVDEN HLVDL3(1) HLVDL2(1) HLVDL1(1) HLVDL0(1)
bit 7 bit 0
bit 7 VDIRMAG: Voltage Direction Magnitude Select bit
1 = Event occurs when voltage equals or exceeds trip point (HLVDL3:HLDVL0)
0 = Event occurs when voltage equals or falls below trip point (HLVDL3:HLVDL0)
bit 6 Unimplemented: Read as ‘0’
bit 5 IRVST: Internal Reference Voltage Stable Flag bit
1 = Indicates that the voltage detect logic will generate the interrupt flag at the specified voltage
range
0 = Indicates that the voltage detect logic will not generate the interrupt flag at the specified
voltage range and the HLVD interrupt should not be enabled
bit 4 HLVDEN: High/Low-Voltage Detect Power Enable bit
1 = HLVD enabled
0 = HLVD disabled
bit 3-0 HLVDL3:HLVDL0: Voltage Detection Limit bits(1)
1111 = External analog input is used (input comes from the HLVDIN pin)
1110 = Maximum setting
.
.
.
0000 = Minimum setting
Note 1: See Table 26-4 for specifications.
Legend:
R = Readable bit W = Writable bit U = Unimplemented bit, read as ‘0’
-n = Value at POR ‘1’ = Bit is set ‘0’ = Bit is cleared x = Bit is unknown
PIC18F2420/2520/4420/4520
DS39631B-page 244 © 2007 Microchip Technology Inc.
The module is enabled by setting the HLVDEN bit.
Each time that the HLVD module is enabled, the circuitry
requires some time to stabilize. The IRVST bit is
a read-only bit and is used to indicate when the circuit
is stable. The module can only generate an interrupt
after the circuit is stable and IRVST is set.
The VDIRMAG bit determines the overall operation of
the module. When VDIRMAG is cleared, the module
monitors for drops in VDD below a predetermined set
point. When the bit is set, the module monitors for rises
in VDD above the set point.
22.1 Operation
When the HLVD module is enabled, a comparator uses
an internally generated reference voltage as the set
point. The set point is compared with the trip point,
where each node in the resistor divider represents a
trip point voltage. The “trip point” voltage is the voltage
level at which the device detects a high or low-voltage
event, depending on the configuration of the module.
When the supply voltage is equal to the trip point, the
voltage tapped off of the resistor array is equal to the
internal reference voltage generated by the voltage
reference module. The comparator then generates an
interrupt signal by setting the HLVDIF bit.
The trip point voltage is software programmable to any one
of 16 values. The trip point is selected by programming the
HLVDL3:HLVDL0 bits (HLVDCON<3:0>).
The HLVD module has an additional feature that allows
the user to supply the trip voltage to the module from an
external source. This mode is enabled when bits
HLVDL3:HLVDL0 are set to ‘1111’. In this state, the
comparator input is multiplexed from the external input
pin, HLVDIN. This gives users flexibility because it
allows them to configure the High/Low-Voltage Detect
interrupt to occur at any voltage in the valid operating
range.
FIGURE 22-1: HLVD MODULE BLOCK DIAGRAM (WITH EXTERNAL INPUT)
Set
VDD
16 to 1 MUX
HLVDEN
HLVDCON
HLVDIN
HLVDL3:HLVDL0
Register
HLVDIN
VDD
Externally Generated
Trip Point
HLVDIF
HLVDEN
BOREN
Internal Voltage
Reference
VDIRMAG
© 2007 Microchip Technology Inc. DS39631B-page 245
PIC18F2420/2520/4420/4520
22.2 HLVD Setup
The following steps are needed to set up the HLVD
module:
1. Write the value to the HLVDL3:HLVDL0 bits that
selects the desired HLVD trip point.
2. Set the VDIRMAG bit to detect high voltage
(VDIRMAG = 1) or low voltage (VDIRMAG = 0).
3. Enable the HLVD module by setting the
HLVDEN bit.
4. Clear the HLVD interrupt flag (PIR2<2>), which
may have been set from a previous interrupt.
5. Enable the HLVD interrupt if interrupts are
desired by setting the HLVDIE and GIE bits
(PIE2<2> and INTCON<7>). An interrupt will not
be generated until the IRVST bit is set.
22.3 Current Consumption
When the module is enabled, the HLVD comparator
and voltage divider are enabled and will consume static
current. The total current consumption, when enabled,
is specified in electrical specification parameter D022B.
Depending on the application, the HLVD module does
not need to be operating constantly. To decrease the
current requirements, the HLVD circuitry may only
need to be enabled for short periods where the voltage
is checked. After doing the check, the HLVD module
may be disabled.
22.4 HLVD Start-up Time
The internal reference voltage of the HLVD module,
specified in electrical specification parameter D420,
may be used by other internal circuitry, such as the
Programmable Brown-out Reset. If the HLVD or other
circuits using the voltage reference are disabled to
lower the device’s current consumption, the reference
voltage circuit will require time to become stable before
a low or high-voltage condition can be reliably
detected. This start-up time, TIRVST, is an interval that
is independent of device clock speed. It is specified in
electrical specification parameter 36.
The HLVD interrupt flag is not enabled until TIRVST has
expired and a stable reference voltage is reached. For
this reason, brief excursions beyond the set point may
not be detected during this interval. Refer to
Figure 22-2 or Figure 22-3.
FIGURE 22-2: LOW-VOLTAGE DETECT OPERATION (VDIRMAG = 0)
VLVD
VDD
HLVDIF
VLVD
VDD
Enable HLVD
TIVRST
HLVDIF may not be set
Enable HLVD
HLVDIF
HLVDIF cleared in software
HLVDIF cleared in software
HLVDIF cleared in software,
CASE 1:
CASE 2:
HLVDIF remains set since HLVD condition still exists
TIVRST
Internal Reference is stable
Internal Reference is stable
IRVST
IRVST
PIC18F2420/2520/4420/4520
DS39631B-page 246 © 2007 Microchip Technology Inc.
FIGURE 22-3: HIGH-VOLTAGE DETECT OPERATION (VDIRMAG = 1)
22.5 Applications
In many applications, the ability to detect a drop below
or rise above a particular threshold is desirable. For
example, the HLVD module could be periodically
enabled to detect Universal Serial Bus (USB) attach or
detach. This assumes the device is powered by a lower
voltage source than the USB when detached. An attach
would indicate a high-voltage detect from, for example,
3.3V to 5V (the voltage on USB) and vice versa for a
detach. This feature could save a design a few extra
components and an attach signal (input pin).
For general battery applications, Figure 22-4 shows a
possible voltage curve. Over time, the device voltage
decreases. When the device voltage reaches voltage
VA, the HLVD logic generates an interrupt at time TA.
The interrupt could cause the execution of an ISR,
which would allow the application to perform “housekeeping
tasks” and perform a controlled shutdown
before the device voltage exits the valid operating
range at TB. The HLVD, thus, would give the application
a time window, represented by the difference
between TA and TB, to safely exit.
FIGURE 22-4: TYPICAL LOW-VOLTAGE
DETECT APPLICATION
VLVD
VDD
HLVDIF
VLVD
VDD
Enable HLVD
TIVRST
HLVDIF may not be set
Enable HLVD
HLVDIF
HLVDIF cleared in software
HLVDIF cleared in software
HLVDIF cleared in software,
CASE 1:
CASE 2:
HLVDIF remains set since HLVD condition still exists
TIVRST
IRVST
Internal Reference is stable
Internal Reference is stable
IRVST
Time
Voltage
VA
VB
TA TB
VA = HLVD trip point
VB = Minimum valid device
operating voltage
Legend:
© 2007 Microchip Technology Inc. DS39631B-page 247
PIC18F2420/2520/4420/4520
22.6 Operation During Sleep
When enabled, the HLVD circuitry continues to operate
during Sleep. If the device voltage crosses the trip
point, the HLVDIF bit will be set and the device will
wake-up from Sleep. Device execution will continue
from the interrupt vector address if interrupts have
been globally enabled.
22.7 Effects of a Reset
A device Reset forces all registers to their Reset state.
This forces the HLVD module to be turned off.
TABLE 22-1: REGISTERS ASSOCIATED WITH HIGH/LOW-VOLTAGE DETECT MODULE
Name Bit 7 Bit 6 Bit 5 Bit 4 Bit 3 Bit 2 Bit 1 Bit 0
Reset
Values
on Page
HLVDCON VDIRMAG — IRVST HLVDEN HLVDL3 HLVDL2 HLVDL1 HLVDL0 50
INTCON GIE/GIEH PEIE/GIEL TMR0IE INT0IE RBIE TMR0IF INT0IF RBIF 49
PIR2 OSCFIF CMIF — EEIF BCLIF HLVDIF TMR3IF CCP2IF 52
PIE2 OCSFIE CMIE — EEIE BCLIE HLVDIE TMR3IE CCP2IE 52
IPR2 OSCFIP CMIP — EEIP BCLIP HLVDIP TMR3IP CCP2IP 52
Legend: — = unimplemented, read as ‘0’. Shaded cells are unused by the HLVD module.
PIC18F2420/2520/4420/4520
DS39631B-page 248 © 2007 Microchip Technology Inc.
NOTES:
© 2007 Microchip Technology Inc. Preliminary DS39631B-page 249
PIC18F2420/2520/4420/4520
23.0 SPECIAL FEATURES OF
THE CPU
PIC18F2420/2520/4420/4520 devices include several
features intended to maximize reliability and minimize
cost through elimination of external components. These
are:
• Oscillator Selection
• Resets:
- Power-on Reset (POR)
- Power-up Timer (PWRT)
- Oscillator Start-up Timer (OST)
- Brown-out Reset (BOR)
• Interrupts
• Watchdog Timer (WDT)
• Fail-Safe Clock Monitor
• Two-Speed Start-up
• Code Protection
• ID Locations
• In-Circuit Serial Programming
The oscillator can be configured for the application
depending on frequency, power, accuracy and cost. All
of the options are discussed in detail in Section 2.0
“Oscillator Configurations”.
A complete discussion of device Resets and interrupts
is available in previous sections of this data sheet.
In addition to their Power-up and Oscillator Start-up
Timers provided for Resets, PIC18F2420/2520/4420/
4520 devices have a Watchdog Timer, which is either
permanently enabled via the configuration bits or
software controlled (if configured as disabled).
The inclusion of an internal RC oscillator also provides
the additional benefits of a Fail-Safe Clock Monitor
(FSCM) and Two-Speed Start-up. FSCM provides for
background monitoring of the peripheral clock and
automatic switchover in the event of its failure. Two-
Speed Start-up enables code to be executed almost
immediately on start-up, while the primary clock source
completes its start-up delays.
All of these features are enabled and configured by
setting the appropriate configuration register bits.
23.1 Configuration Bits
The configuration bits can be programmed (read as ‘0’)
or left unprogrammed (read as ‘1’) to select various
device configurations. These bits are mapped starting
at program memory location 300000h.
The user will note that address 300000h is beyond the
user program memory space. In fact, it belongs to the
configuration memory space (300000h-3FFFFFh), which
can only be accessed using table reads and table writes.
Programming the configuration registers is done in a
manner similar to programming the Flash memory. The
WR bit in the EECON1 register starts a self-timed write
to the configuration register. In normal operation mode,
a TBLWT instruction with the TBLPTR pointing to the
configuration register sets up the address and the data
for the configuration register write. Setting the WR bit
starts a long write to the configuration register. The
configuration registers are written a byte at a time. To
write or erase a configuration cell, a TBLWT instruction
can write a ‘1’ or a ‘0’ into the cell. For additional details
on Flash programming, refer to Section 6.5 “Writing
to Flash Program Memory”.
TABLE 23-1: CONFIGURATION BITS AND DEVICE IDs
File Name Bit 7 Bit 6 Bit 5 Bit 4 Bit 3 Bit 2 Bit 1 Bit 0
Default/
Unprogrammed
Value
300001h CONFIG1H IESO FCMEN — — FOSC3 FOSC2 FOSC1 FOSC0 00-- 0111
300002h CONFIG2L — — — BORV1 BORV0 BOREN1 BOREN0 PWRTEN ---1 1111
300003h CONFIG2H — — — WDTPS3 WDTPS2 WDTPS1 WDTPS0 WDTEN ---1 1111
300005h CONFIG3H MCLRE — — — — LPT1OSC PBADEN CCP2MX 1--- -011
300006h CONFIG4L DEBUG XINST — — — LVP — STVREN 10-- -1-1
300008h CONFIG5L — — — — CP3(1) CP2(1) CP1 CP0 ---- 1111
300009h CONFIG5H CPD CPB — — — — — — 11-- ----
30000Ah CONFIG6L — — — — WRT3(1) WRT2(1) WRT1 WRT0 ---- 1111
30000Bh CONFIG6H WRTD WRTB WRTC — — — — — 111- ----
30000Ch CONFIG7L — — — — EBTR3(1) EBTR2(1) EBTR1 EBTR0 ---- 1111
30000Dh CONFIG7H — EBTRB — — — — — — -1-- ----
3FFFFEh DEVID1(1) DEV2 DEV1 DEV0 REV4 REV3 REV2 REV1 REV0 xxxx xxxx(2)
3FFFFFh DEVID2(1) DEV10 DEV9 DEV8 DEV7 DEV6 DEV5 DEV4 DEV3 0000 1100
Legend: x = unknown, u = unchanged, — = unimplemented, q = value depends on condition.
Shaded cells are unimplemented, read as ‘0’.
Note 1: Unimplemented in PIC18F2420/4420 devices; maintain this bit set.
2: See Register 23-14 for DEVID1 values. DEVID registers are read-only and cannot be programmed by the user.
PIC18F2420/2520/4420/4520
DS39631B-page 250 Preliminary © 2007 Microchip Technology Inc.
REGISTER 23-1: CONFIG1H: CONFIGURATION REGISTER 1 HIGH (BYTE ADDRESS 300001h)
R/P-0 R/P-0 U-0 U-0 R/P-0 R/P-1 R/P-1 R/P-1
IESO FCMEN — — FOSC3 FOSC2 FOSC1 FOSC0
bit 7 bit 0
bit 7 IESO: Internal/External Oscillator Switchover bit
1 = Oscillator Switchover mode enabled
0 = Oscillator Switchover mode disabled
bit 6 FCMEN: Fail-Safe Clock Monitor Enable bit
1 = Fail-Safe Clock Monitor enabled
0 = Fail-Safe Clock Monitor disabled
bit 5-4 Unimplemented: Read as ‘0’
bit 3-0 FOSC3:FOSC0: Oscillator Selection bits
11xx = External RC oscillator, CLKO function on RA6
101x = External RC oscillator, CLKO function on RA6
1001 = Internal oscillator block, CLKO function on RA6, port function on RA7
1000 = Internal oscillator block, port function on RA6 and RA7
0111 = External RC oscillator, port function on RA6
0110 = HS oscillator, PLL enabled (Clock Frequency = 4 x FOSC1)
0101 = EC oscillator, port function on RA6
0100 = EC oscillator, CLKO function on RA6
0011 = External RC oscillator, CLKO function on RA6
0010 = HS oscillator
0001 = XT oscillator
0000 = LP oscillator
Legend:
R = Readable bit P = Programmable bit U = Unimplemented bit, read as ‘0’
-n = Value when device is unprogrammed u = Unchanged from programmed state
© 2007 Microchip Technology Inc. Preliminary DS39631B-page 251
PIC18F2420/2520/4420/4520
REGISTER 23-2: CONFIG2L: CONFIGURATION REGISTER 2 LOW (BYTE ADDRESS 300002h)
U-0 U-0 U-0 R/P-1 R/P-1 R/P-1 R/P-1 R/P-1
— — — BORV1(1) BORV0(1) BOREN1(2) BOREN0(2) PWRTEN(2)
bit 7 bit 0
bit 7-5 Unimplemented: Read as ‘0’
bit 4-3 BORV1:BORV0: Brown-out Reset Voltage bits(1)
11 = Maximum setting
.
.
.
00 = Minimum setting
bit 2-1 BOREN1:BOREN0: Brown-out Reset Enable bits(2)
11 = Brown-out Reset enabled in hardware only (SBOREN is disabled)
10 = Brown-out Reset enabled in hardware only and disabled in Sleep mode
(SBOREN is disabled)
01 = Brown-out Reset enabled and controlled by software (SBOREN is enabled)
00 = Brown-out Reset disabled in hardware and software
bit 0 PWRTEN: Power-up Timer Enable bit(2)
1 = PWRT disabled
0 = PWRT enabled
Note 1: See Section 26.1 “DC Characteristics: Supply Voltage” for specifications.
2: The Power-up Timer is decoupled from Brown-out Reset, allowing these features to
be independently controlled.
Legend:
R = Readable bit P = Programmable bit U = Unimplemented bit, read as ‘0’
-n = Value when device is unprogrammed u = Unchanged from programmed state
PIC18F2420/2520/4420/4520
DS39631B-page 252 Preliminary © 2007 Microchip Technology Inc.
REGISTER 23-3: CONFIG2H: CONFIGURATION REGISTER 2 HIGH (BYTE ADDRESS 300003h)
U-0 U-0 U-0 R/P-1 R/P-1 R/P-1 R/P-1 R/P-1
— — — WDTPS3 WDTPS2 WDTPS1 WDTPS0 WDTEN
bit 7 bit 0
bit 7-5 Unimplemented: Read as ‘0’
bit 4-1 WDTPS3:WDTPS0: Watchdog Timer Postscale Select bits
1111 = 1:32,768
1110 = 1:16,384
1101 = 1:8,192
1100 = 1:4,096
1011 = 1:2,048
1010 = 1:1,024
1001 = 1:512
1000 = 1:256
0111 = 1:128
0110 = 1:64
0101 = 1:32
0100 = 1:16
0011 = 1:8
0010 = 1:4
0001 = 1:2
0000 = 1:1
bit 0 WDTEN: Watchdog Timer Enable bit
1 = WDT enabled
0 = WDT disabled (control is placed on the SWDTEN bit)
Legend:
R = Readable bit P = Programmable bit U = Unimplemented bit, read as ‘0’
-n = Value when device is unprogrammed u = Unchanged from programmed state
© 2007 Microchip Technology Inc. Preliminary DS39631B-page 253
PIC18F2420/2520/4420/4520
REGISTER 23-4: CONFIG3H: CONFIGURATION REGISTER 3 HIGH (BYTE ADDRESS 300005h)
REGISTER 23-5: CONFIG4L: CONFIGURATION REGISTER 4 LOW (BYTE ADDRESS 300006h)
R/P-1 U-0 U-0 U-0 U-0 R/P-0 R/P-1 R/P-1
MCLRE — — — — LPT1OSC PBADEN CCP2MX
bit 7 bit 0
bit 7 MCLRE: MCLR Pin Enable bit
1 = MCLR pin enabled; RE3 input pin disabled
0 = RE3 input pin enabled; MCLR disabled
bit 6-3 Unimplemented: Read as ‘0’
bit 2 LPT1OSC: Low-Power Timer1 Oscillator Enable bit
1 = Timer1 configured for low-power operation
0 = Timer1 configured for higher power operation
bit 1 PBADEN: PORTB A/D Enable bit
(Affects ADCON1 Reset state. ADCON1 controls PORTB<4:0> pin configuration.)
1 = PORTB<4:0> pins are configured as analog input channels on Reset
0 = PORTB<4:0> pins are configured as digital I/O on Reset
bit 0 CCP2MX: CCP2 Mux bit
1 = CCP2 input/output is multiplexed with RC1
0 = CCP2 input/output is multiplexed with RB3
Legend:
R = Readable bit P = Programmable bit U = Unimplemented bit, read as ‘0’
-n = Value when device is unprogrammed u = Unchanged from programmed state
R/P-1 R/P-0 U-0 U-0 U-0 R/P-1 U-0 R/P-1
DEBUG XINST — — — LVP — STVREN
bit 7 bit 0
bit 7 DEBUG: Background Debugger Enable bit
1 = Background debugger disabled, RB6 and RB7 configured as general purpose I/O pins
0 = Background debugger enabled, RB6 and RB7 are dedicated to In-Circuit Debug
bit 6 XINST: Extended Instruction Set Enable bit
1 = Instruction set extension and Indexed Addressing mode enabled
0 = Instruction set extension and Indexed Addressing mode disabled (Legacy mode)
bit 5-3 Unimplemented: Read as ‘0’
bit 2 LVP: Single-Supply ICSP Enable bit
1 = Single-Supply ICSP enabled
0 = Single-Supply ICSP disabled
bit 1 Unimplemented: Read as ‘0’
bit 0 STVREN: Stack Full/Underflow Reset Enable bit
1 = Stack full/underflow will cause Reset
0 = Stack full/underflow will not cause Reset
Legend:
R = Readable bit C = Clearable bit U = Unimplemented bit, read as ‘0’
-n = Value when device is unprogrammed u = Unchanged from programmed state
PIC18F2420/2520/4420/4520
DS39631B-page 254 Preliminary © 2007 Microchip Technology Inc.
REGISTER 23-6: CONFIG5L: CONFIGURATION REGISTER 5 LOW (BYTE ADDRESS 300008h)
REGISTER 23-7: CONFIG5H: CONFIGURATION REGISTER 5 HIGH (BYTE ADDRESS 300009h)
U-0 U-0 U-0 U-0 R/C-1 R/C-1 R/C-1 R/C-1
— — — — CP3(1,2) CP2(1) CP1 CP0
bit 7 bit 0
bit 7-4 Unimplemented: Read as ‘0’
bit 3 CP3: Code Protection bit(1,2)
1 = Block 3 (006000-007FFFh) not code-protected
0 = Block 3 (006000-007FFFh) code-protected
bit 2 CP2: Code Protection bit(1)
1 = Block 2 (004000-005FFFh) not code-protected
0 = Block 2 (004000-005FFFh) code-protected
bit 1 CP1: Code Protection bit
1 = Block 1 (002000-003FFFh) not code-protected
0 = Block 1 (002000-003FFFh) code-protected
bit 0 CP0: Code Protection bit
1 = Block 0 (000800-001FFFh) not code-protected
0 = Block 0 (000800-001FFFh) code-protected
Note 1: Unimplemented in PIC18F2420/4420 devices; maintain this bit set.
2: Unimplemented in PIC18F2425/4425 devices; maintain this bit set.
Legend:
R = Readable bit C = Clearable bit U = Unimplemented bit, read as ‘0’
-n = Value when device is unprogrammed u = Unchanged from programmed state
R/C-1 R/C-1 U-0 U-0 U-0 U-0 U-0 U-0
CPD CPB — — — — — —
bit 7 bit 0
bit 7 CPD: Data EEPROM Code Protection bit
1 = Data EEPROM not code-protected
0 = Data EEPROM code-protected
bit 6 CPB: Boot Block Code Protection bit
1 = Boot block (000000-0007FFh) not code-protected
0 = Boot block (000000-0007FFh) code-protected
bit 5-0 Unimplemented: Read as ‘0’
Legend:
R = Readable bit C = Clearable bit U = Unimplemented bit, read as ‘0’
-n = Value when device is unprogrammed u = Unchanged from programmed state
© 2007 Microchip Technology Inc. Preliminary DS39631B-page 255
PIC18F2420/2520/4420/4520
REGISTER 23-8: CONFIG6L: CONFIGURATION REGISTER 6 LOW (BYTE ADDRESS 30000Ah)
REGISTER 23-9: CONFIG6H: CONFIGURATION REGISTER 6 HIGH (BYTE ADDRESS 30000Bh)
U-0 U-0 U-0 U-0 R/C-1 R/C-1 R/C-1 R/C-1
— — — — WRT3(1,2) WRT2(1) WRT1 WRT0
bit 7 bit 0
bit 7-4 Unimplemented: Read as ‘0’
bit 3 WRT3: Write Protection bit(1,2)
1 = Block 3 (006000-007FFFh) not write-protected
0 = Block 3 (006000-007FFFh) write-protected
bit 2 WRT2: Write Protection bit(1)
1 = Block 2 (004000-005FFFh) not write-protected
0 = Block 2 (004000-005FFFh) write-protected
bit 1 WRT1: Write Protection bit
1 = Block 1 (002000-003FFFh) not write-protected
0 = Block 1 (002000-003FFFh) write-protected
bit 0 WRT0: Write Protection bit
1 = Block 0 (000800-001FFFh) not write-protected
0 = Block 0 (000800-001FFFh) write-protected
Note 1: Unimplemented in PIC18F2420/4420 devices; maintain this bit set.
2: Unimplemented in PIC18F2425/4425 devices; maintain this bit set.
Legend:
R = Readable bit C = Clearable bit U = Unimplemented bit, read as ‘0’
-n = Value when device is unprogrammed u = Unchanged from programmed state
R/C-1 R/C-1 R-1 U-0 U-0 U-0 U-0 U-0
WRTD WRTB WRTC(1) — — — — —
bit 7 bit 0
bit 7 WRTD: Data EEPROM Write Protection bit
1 = Data EEPROM not write-protected
0 = Data EEPROM write-protected
bit 6 WRTB: Boot Block Write Protection bit
1 = Boot block (000000-0007FFh) not write-protected
0 = Boot block (000000-0007FFh) write-protected
bit 5 WRTC: Configuration Register Write Protection bit(1)
1 = Configuration registers (300000-3000FFh) not write-protected
0 = Configuration registers (300000-3000FFh) write-protected
Note 1: This bit is read-only in normal execution mode; it can be written only in Program mode.
bit 4-0 Unimplemented: Read as ‘0’
Legend:
R = Readable bit C = Clearable bit U = Unimplemented bit, read as ‘0’
- n = Value when device is unprogrammed u = Unchanged from programmed state
PIC18F2420/2520/4420/4520
DS39631B-page 256 Preliminary © 2007 Microchip Technology Inc.
REGISTER 23-10: CONFIG7L: CONFIGURATION REGISTER 7 LOW (BYTE ADDRESS 30000Ch)
REGISTER 23-11: CONFIG7H: CONFIGURATION REGISTER 7 HIGH (BYTE ADDRESS 30000Dh)
U-0 U-0 U-0 U-0 R/C-1 R/C-1 R/C-1 R/C-1
— — — — EBTR3(1,2) EBTR2(1) EBTR1 EBTR0
bit 7 bit 0
bit 7-4 Unimplemented: Read as ‘0’
bit 3 EBTR3: Table Read Protection bit(1,2)
1 = Block 3 (006000-007FFFh) not protected from table reads executed in other blocks
0 = Block 3 (006000-007FFFh) protected from table reads executed in other blocks
bit 2 EBTR2: Table Read Protection bit(1)
1 = Block 2 (004000-005FFFh) not protected from table reads executed in other blocks
0 = Block 2 (004000-005FFFh) protected from table reads executed in other blocks
bit 1 EBTR1: Table Read Protection bit
1 = Block 1 (002000-003FFFh) not protected from table reads executed in other blocks
0 = Block 1 (002000-003FFFh) protected from table reads executed in other blocks
bit 0 EBTR0: Table Read Protection bit
1 = Block 0 (000800-001FFFh) not protected from table reads executed in other blocks
0 = Block 0 (000800-001FFFh) protected from table reads executed in other blocks
Note 1: Unimplemented in PIC18F2420/4420 devices; maintain this bit set.
2: Unimplemented in PIC18F2425/4425 devices; maintain this bit set.
Legend:
R = Readable bit C = Clearable bit U = Unimplemented bit, read as ‘0’
-n = Value when device is unprogrammed u = Unchanged from programmed state
U-0 R/C-1 U-0 U-0 U-0 U-0 U-0 U-0
— EBTRB — — — — — —
bit 7 bit 0
bit 7 Unimplemented: Read as ‘0’
bit 6 EBTRB: Boot Block Table Read Protection bit
1 = Boot block (000000-0007FFh) not protected from table reads executed in other blocks
0 = Boot block (000000-0007FFh) protected from table reads executed in other blocks
bit 5-0 Unimplemented: Read as ‘0’
Legend:
R = Readable bit C = Clearable bit U = Unimplemented bit, read as ‘0’
-n = Value when device is unprogrammed u = Unchanged from programmed state
© 2007 Microchip Technology Inc. Preliminary DS39631B-page 257
PIC18F2420/2520/4420/4520
REGISTER 23-12: DEVID1: DEVICE ID REGISTER 1 FOR PIC18F2420/2520/4420/4520
REGISTER 23-13: DEVID2: DEVICE ID REGISTER 2 FOR PIC18F2420/2520/4420/4520
R R R R R R R R
DEV2 DEV1 DEV0 REV4 REV3 REV2 REV1 REV0
bit 7 bit 0
bit 7-5 DEV2:DEV0: Device ID bits
000 = PIC18F4520
010 = PIC18F4420
100 = PIC18F2520
110 = PIC18F2420
bit 4-0 REV4:REV0: Revision ID bits
These bits are used to indicate the device revision.
Legend:
R = Read-only bit P = Programmable bit U = Unimplemented bit, read as ‘0’
-n = Value when device is unprogrammed u = Unchanged from programmed state
R R R R R R R R
DEV10 DEV9 DEV8 DEV7 DEV6 DEV5 DEV4 DEV3
bit 7 bit 0
bit 7-0 DEV10:DEV3: Device ID bits
These bits are used with the DEV2:DEV0 bits in the Device ID Register 1 to identify the
part number.
0000 1100 = PIC18F2420/2520/4420/4520 devices
Note: These values for DEV10:DEV3 may be shared with other devices. The specific
device is always identified by using the entire DEV10:DEV0 bit sequence.
Legend:
R = Read-only bit P = Programmable bit U = Unimplemented bit, read as ‘0’
-n = Value when device is unprogrammed u = Unchanged from programmed state
PIC18F2420/2520/4420/4520
DS39631B-page 258 Preliminary © 2007 Microchip Technology Inc.
23.2 Watchdog Timer (WDT)
For PIC18F2420/2520/4420/4520 devices, the WDT is
driven by the INTRC source. When the WDT is
enabled, the clock source is also enabled. The nominal
WDT period is 4 ms and has the same stability as the
INTRC oscillator.
The 4 ms period of the WDT is multiplied by a 16-bit
postscaler. Any output of the WDT postscaler is
selected by a multiplexer, controlled by bits in Configuration
Register 2H. Available periods range from 4 ms
to 131.072 seconds (2.18 minutes). The WDT and
postscaler are cleared when any of the following events
occur: a SLEEP or CLRWDT instruction is executed, the
IRCF bits (OSCCON<6:4>) are changed or a clock
failure has occurred.
23.2.1 CONTROL REGISTER
Register 23-14 shows the WDTCON register. This is a
readable and writable register which contains a control
bit that allows software to override the WDT enable
configuration bit, but only if the configuration bit has
disabled the WDT.
FIGURE 23-1: WDT BLOCK DIAGRAM
Note 1: The CLRWDT and SLEEP instructions
clear the WDT and postscaler counts
when executed.
2: Changing the setting of the IRCF bits
(OSCCON<6:4>) clears the WDT and
postscaler counts.
3: When a CLRWDT instruction is executed,
the postscaler count will be cleared.
INTRC Source
WDT
Wake-up
Reset
WDT Counter
Programmable Postscaler
1:1 to 1:32,768
Enable WDT
WDTPS<3:0>
SWDTEN
WDTEN
CLRWDT
4
from Power
Reset
All Device Resets
Sleep
÷128
Change on IRCF bits
Managed Modes
© 2007 Microchip Technology Inc. Preliminary DS39631B-page 259
PIC18F2420/2520/4420/4520
REGISTER 23-14: WDTCON REGISTER
TABLE 23-2: SUMMARY OF WATCHDOG TIMER REGISTERS
U-0 U-0 U-0 U-0 U-0 U-0 U-0 R/W-0
— — — — — — — SWDTEN(1)
bit 7 bit 0
bit 7-1 Unimplemented: Read as ‘0’
bit 0 SWDTEN: Software Controlled Watchdog Timer Enable bit(1)
1 = Watchdog Timer is on
0 = Watchdog Timer is off
Note 1: This bit has no effect if the configuration bit, WDTEN, is enabled.
Legend:
R = Readable bit W = Writable bit
U = Unimplemented bit, read as ‘0’ -n = Value at POR
Name Bit 7 Bit 6 Bit 5 Bit 4 Bit 3 Bit 2 Bit 1 Bit 0
Reset
Values
on page
RCON IPEN SBOREN — RI TO PD POR BOR 48
WDTCON — — — — — — — SWDTEN 50
Legend: — = unimplemented, read as ‘0’. Shaded cells are not used by the Watchdog Timer.
PIC18F2420/2520/4420/4520
DS39631B-page 260 Preliminary © 2007 Microchip Technology Inc.
23.3 Two-Speed Start-up
The Two-Speed Start-up feature helps to minimize the
latency period from oscillator start-up to code execution
by allowing the microcontroller to use the INTOSC
oscillator as a clock source until the primary clock
source is available. It is enabled by setting the IESO
configuration bit.
Two-Speed Start-up should be enabled only if the
primary oscillator mode is LP, XT, HS or HSPLL
(crystal-based modes). Other sources do not require
an OST start-up delay; for these, Two-Speed Start-up
should be disabled.
When enabled, Resets and wake-ups from Sleep mode
cause the device to configure itself to run from the
internal oscillator block as the clock source, following
the time-out of the Power-up Timer after a Power-on
Reset is enabled. This allows almost immediate code
execution while the primary oscillator starts and the
OST is running. Once the OST times out, the device
automatically switches to PRI_RUN mode.
To use a higher clock speed on wake-up, the INTOSC
or postscaler clock sources can be selected to provide
a higher clock speed by setting bits IRCF2:IRCF0
immediately after Reset. For wake-ups from Sleep, the
INTOSC or postscaler clock sources can be selected
by setting the IRCF2:IRCF0 bits prior to entering Sleep
mode.
In all other power managed modes, Two-Speed Startup
is not used. The device will be clocked by the
currently selected clock source until the primary clock
source becomes available. The setting of the IESO bit
is ignored.
23.3.1 SPECIAL CONSIDERATIONS FOR
USING TWO-SPEED START-UP
While using the INTOSC oscillator in Two-Speed Startup,
the device still obeys the normal command
sequences for entering power managed modes,
including multiple SLEEP instructions (refer to
Section 3.1.4 “Multiple Sleep Commands”). In
practice, this means that user code can change the
SCS1:SCS0 bit settings or issue SLEEP instructions
before the OST times out. This would allow an
application to briefly wake-up, perform routine
“housekeeping” tasks and return to Sleep before the
device starts to operate from the primary oscillator.
User code can also check if the primary clock source is
currently providing the device clocking by checking the
status of the OSTS bit (OSCCON<3>). If the bit is set,
the primary oscillator is providing the clock. Otherwise,
the internal oscillator block is providing the clock during
wake-up from Reset or Sleep mode.
FIGURE 23-2: TIMING TRANSITION FOR TWO-SPEED START-UP (INTOSC TO HSPLL)
Q1 Q3 Q4
OSC1
Peripheral
Program
PC PC + 2
INTOSC
PLL Clock
Q1
PC + 6
Q2
Output
Q3 Q4 Q1
CPU Clock
PC + 4
Clock
Counter
Q2 Q2 Q3
Note 1: TOST = 1024 TOSC; TPLL = 2 ms (approx). These intervals are not shown to scale.
2: Clock transition typically occurs within 2-4 TOSC.
Wake from Interrupt Event
TPLL(1)
1 2 n-1 n
Clock
OSTS bit Set
Transition(2)
Multiplexer
TOST(1)
© 2007 Microchip Technology Inc. Preliminary DS39631B-page 261
PIC18F2420/2520/4420/4520
23.4 Fail-Safe Clock Monitor
The Fail-Safe Clock Monitor (FSCM) allows the
microcontroller to continue operation in the event of an
external oscillator failure by automatically switching the
device clock to the internal oscillator block. The FSCM
function is enabled by setting the FCMEN configuration
bit.
When FSCM is enabled, the INTRC oscillator runs at
all times to monitor clocks to peripherals and provide a
backup clock in the event of a clock failure. Clock
monitoring (shown in Figure 23-3) is accomplished by
creating a sample clock signal, which is the INTRC output
divided by 64. This allows ample time between
FSCM sample clocks for a peripheral clock edge to
occur. The peripheral device clock and the sample
clock are presented as inputs to the Clock Monitor latch
(CM). The CM is set on the falling edge of the device
clock source, but cleared on the rising edge of the
sample clock.
FIGURE 23-3: FSCM BLOCK DIAGRAM
Clock failure is tested for on the falling edge of the sample
clock. If a sample clock falling edge occurs while
CM is still set, a clock failure has been detected
(Figure 23-4). This causes the following:
• the FSCM generates an oscillator fail interrupt by
setting bit, OSCFIF (PIR2<7>);
• the device clock source is switched to the internal
oscillator block (OSCCON is not updated to show
the current clock source – this is the fail-safe
condition) and
• the WDT is reset.
During switchover, the postscaler frequency from the
internal oscillator block may not be sufficiently stable for
timing sensitive applications. In these cases, it may be
desirable to select another clock configuration and enter
an alternate power managed mode. This can be done to
attempt a partial recovery or execute a controlled shutdown.
See Section 3.1.4 “Multiple Sleep Commands”
and Section 23.3.1 “Special Considerations for
Using Two-Speed Start-up” for more details.
To use a higher clock speed on wake-up, the INTOSC or
postscaler clock sources can be selected to provide a
higher clock speed by setting bits, IRCF2:IRCF0, immediately
after Reset. For wake-ups from Sleep, the INTOSC
or postscaler clock sources can be selected by setting the
IRCF2:IRCF0 bits prior to entering Sleep mode.
The FSCM will detect failures of the primary or secondary
clock sources only. If the internal oscillator block
fails, no failure would be detected, nor would any action
be possible.
23.4.1 FSCM AND THE WATCHDOG TIMER
Both the FSCM and the WDT are clocked by the
INTRC oscillator. Since the WDT operates with a separate
divider and counter, disabling the WDT has no
effect on the operation of the INTRC oscillator when the
FSCM is enabled.
As already noted, the clock source is switched to the
INTOSC clock when a clock failure is detected.
Depending on the frequency selected by the
IRCF2:IRCF0 bits, this may mean a substantial change
in the speed of code execution. If the WDT is enabled
with a small prescale value, a decrease in clock speed
allows a WDT time-out to occur and a subsequent
device Reset. For this reason, fail-safe clock events
also reset the WDT and postscaler, allowing it to start
timing from when execution speed was changed and
decreasing the likelihood of an erroneous time-out.
23.4.2 EXITING FAIL-SAFE OPERATION
The fail-safe condition is terminated by either a device
Reset or by entering a power managed mode. On
Reset, the controller starts the primary clock source
specified in Configuration Register 1H (with any
required start-up delays that are required for the oscillator
mode, such as OST or PLL timer). The INTOSC
multiplexer provides the device clock until the primary
clock source becomes ready (similar to a Two-Speed
Start-up). The clock source is then switched to the primary
clock (indicated by the OSTS bit in the OSCCON
register becoming set). The Fail-Safe Clock Monitor
then resumes monitoring the peripheral clock.
The primary clock source may never become ready during
start-up. In this case, operation is clocked by the
INTOSC multiplexer. The OSCCON register will remain
in its Reset state until a power managed mode is entered.
Peripheral
INTRC
÷ 64
S
C
Q
(32 μs) 488 Hz
(2.048 ms)
Clock Monitor
Latch (CM)
(edge-triggered)
Clock
Failure
Detected
Source
Clock
Q
PIC18F2420/2520/4420/4520
DS39631B-page 262 Preliminary © 2007 Microchip Technology Inc.
FIGURE 23-4: FSCM TIMING DIAGRAM
23.4.3 FSCM INTERRUPTS IN POWER
MANAGED MODES
By entering a power managed mode, the clock
multiplexer selects the clock source selected by the
OSCCON register. Fail-Safe Monitoring of the power
managed clock source resumes in the power managed
mode.
If an oscillator failure occurs during power managed
operation, the subsequent events depend on whether
or not the oscillator failure interrupt is enabled. If
enabled (OSCFIF = 1), code execution will be clocked
by the INTOSC multiplexer. An automatic transition
back to the failed clock source will not occur.
If the interrupt is disabled, subsequent interrupts while
in Idle mode will cause the CPU to begin executing
instructions while being clocked by the INTOSC
source.
23.4.4 POR OR WAKE FROM SLEEP
The FSCM is designed to detect oscillator failure at any
point after the device has exited Power-on Reset
(POR) or low-power Sleep mode. When the primary
device clock is EC, RC or INTRC modes, monitoring
can begin immediately following these events.
For oscillator modes involving a crystal or resonator
(HS, HSPLL, LP or XT), the situation is somewhat
different. Since the oscillator may require a start-up
time considerably longer than the FCSM sample clock
time, a false clock failure may be detected. To prevent
this, the internal oscillator block is automatically configured
as the device clock and functions until the primary
clock is stable (the OST and PLL timers have timed
out). This is identical to Two-Speed Start-up mode.
Once the primary clock is stable, the INTRC returns to
its role as the FSCM source.
As noted in Section 23.3.1 “Special Considerations
for Using Two-Speed Start-up”, it is also possible to
select another clock configuration and enter an
alternate power managed mode while waiting for the
primary clock to become stable. When the new power
managed mode is selected, the primary clock is
disabled.
OSCFIF
CM Output
Device
Clock
Output
Sample Clock
Failure
Detected
Oscillator
Failure
Note: The device clock is normally at a much higher frequency than the sample clock. The relative frequencies in this
example have been chosen for clarity.
(Q)
CM Test CM Test CM Test
Note: The same logic that prevents false oscillator
failure interrupts on POR, or wake from
Sleep, will also prevent the detection of
the oscillator’s failure to start at all following
these events. This can be avoided by
monitoring the OSTS bit and using a
timing routine to determine if the oscillator
is taking too long to start. Even so, no
oscillator failure interrupt will be flagged.
© 2007 Microchip Technology Inc. Preliminary DS39631B-page 263
PIC18F2420/2520/4420/4520
23.5 Program Verification and
Code Protection
The overall structure of the code protection on the
PIC18 Flash devices differs significantly from other
PIC® devices.
The user program memory is divided into five blocks.
One of these is a boot block of 2 Kbytes. The remainder
of the memory is divided into four blocks on binary
boundaries.
Each of the five blocks has three code protection bits
associated with them. They are:
• Code-Protect bit (CPn)
• Write-Protect bit (WRTn)
• External Block Table Read bit (EBTRn)
Figure 23-5 shows the program memory organization
for 16 and 32-Kbyte devices and the specific code protection
bit associated with each block. The actual
locations of the bits are summarized in Table 23-3.
FIGURE 23-5: CODE-PROTECTED PROGRAM MEMORY FOR
PIC18F2420/2520/4420/4520
TABLE 23-3: SUMMARY OF CODE PROTECTION REGISTERS
File Name Bit 7 Bit 6 Bit 5 Bit 4 Bit 3 Bit 2 Bit 1 Bit 0
300008h CONFIG5L — — — — CP3(1,2) CP2(1) CP1 CP0
300009h CONFIG5H CPD CPB — — — — — —
30000Ah CONFIG6L — — — — WRT3(1,2) WRT2(1) WRT1 WRT0
30000Bh CONFIG6H WRTD WRTB WRTC — — — — —
30000Ch CONFIG7L — — — — EBTR3(1,2) EBTR2(1) EBTR1 EBTR0
30000Dh CONFIG7H — EBTRB — — — — — —
Legend: Shaded cells are unimplemented.
Note 1: Unimplemented in PIC18F2420/4420 devices; maintain this bit set.
2: Unimplemented in PIC18F2425/4425 devices; maintain this bit set.
MEMORY SIZE/DEVICE
Block Code Protection
16 Kbytes Controlled By:
(PIC18F2420/4420)
32 Kbytes
(PIC18F2520/4520)
Address
Range
Boot Block Boot Block
000000h
0007FFh
CPB, WRTB, EBTRB
Block 0 Block 0
000800h
001FFFh
CP0, WRT0, EBTR0
Block 1 Block 1
002000h
003FFFh
CP1, WRT1, EBTR1
Unimplemented
Read ‘0’s
Block 2
004000h
005FFFh
CP2, WRT2, EBTR2
Block 3
006000h
007FFFh
CP3, WRT3, EBTR3
Unimplemented
Read ‘0’s
1FFFFFh
(Unimplemented Memory Space)
PIC18F2420/2520/4420/4520
DS39631B-page 264 Preliminary © 2007 Microchip Technology Inc.
23.5.1 PROGRAM MEMORY
CODE PROTECTION
The program memory may be read to or written from
any location using the table read and table write
instructions. The device ID may be read with table
reads. The configuration registers may be read and
written with the table read and table write instructions.
In normal execution mode, the CPn bits have no direct
effect. CPn bits inhibit external reads and writes. A block
of user memory may be protected from table writes if the
WRTn configuration bit is ‘0’. The EBTRn bits control
table reads. For a block of user memory with the EBTRn
bit set to ‘0’, a table read instruction that executes from
within that block is allowed to read. A table read instruction
that executes from a location outside of that block is
not allowed to read and will result in reading ‘0’s.
Figures 23-6 through 23-8 illustrate table write and table
read protection.
FIGURE 23-6: TABLE WRITE (WRTn) DISALLOWED
Note: Code protection bits may only be written to
a ‘0’ from a ‘1’ state. It is not possible to
write a ‘1’ to a bit in the ‘0’ state. Code protection
bits are only set to ‘1’ by a full chip
erase or block erase function. The full chip
erase and block erase functions can only
be initiated via ICSP or an external
programmer.
000000h
0007FFh
000800h
001FFFh
002000h
003FFFh
004000h
005FFFh
006000h
007FFFh
WRTB, EBTRB = 11
WRT0, EBTR0 = 01
WRT1, EBTR1 = 11
WRT2, EBTR2 = 11
WRT3, EBTR3 = 11
TBLWT*
TBLPTR = 0008FFh
PC = 001FFEh
PC = 005FFEh TBLWT*
Register Values Program Memory Configuration Bit Settings
Results: All table writes disabled to Blockn whenever WRTn = 0.
© 2007 Microchip Technology Inc. Preliminary DS39631B-page 265
PIC18F2420/2520/4420/4520
FIGURE 23-7: EXTERNAL BLOCK TABLE READ (EBTRn) DISALLOWED
FIGURE 23-8: EXTERNAL BLOCK TABLE READ (EBTRn) ALLOWED
WRTB, EBTRB = 11
WRT0, EBTR0 = 10
WRT1, EBTR1 = 11
WRT2, EBTR2 = 11
WRT3, EBTR3 = 11
TBLRD*
TBLPTR = 0008FFh
PC = 003FFEh
Results: All table reads from external blocks to Blockn are disabled whenever EBTRn = 0.
TABLAT register returns a value of ‘0’.
Register Values Program Memory Configuration Bit Settings
000000h
0007FFh
000800h
001FFFh
002000h
003FFFh
004000h
005FFFh
006000h
007FFFh
WRTB, EBTRB = 11
WRT0, EBTR0 = 10
WRT1, EBTR1 = 11
WRT2, EBTR2 = 11
WRT3, EBTR3 = 11
TBLRD*
TBLPTR = 0008FFh
PC = 001FFEh
Register Values Program Memory Configuration Bit Settings
Results: Table reads permitted within Blockn, even when EBTRBn = 0.
TABLAT register returns the value of the data at the location TBLPTR.
000000h
0007FFh
000800h
001FFFh
002000h
003FFFh
004000h
005FFFh
006000h
007FFFh
PIC18F2420/2520/4420/4520
DS39631B-page 266 Preliminary © 2007 Microchip Technology Inc.
23.5.2 DATA EEPROM
CODE PROTECTION
The entire data EEPROM is protected from external
reads and writes by two bits: CPD and WRTD. CPD
inhibits external reads and writes of data EEPROM.
WRTD inhibits internal and external writes to data
EEPROM. The CPU can always read data EEPROM
under normal operation, regardless of the protection bit
settings.
23.5.3 CONFIGURATION REGISTER
PROTECTION
The configuration registers can be write-protected. The
WRTC bit controls protection of the configuration
registers. In normal execution mode, the WRTC bit is
readable only. WRTC can only be written via ICSP or
an external programmer.
23.6 ID Locations
Eight memory locations (200000h-200007h) are
designated as ID locations, where the user can store
checksum or other code identification numbers. These
locations are both readable and writable during normal
execution through the TBLRD and TBLWT instructions
or during program/verify. The ID locations can be read
when the device is code-protected.
23.7 In-Circuit Serial Programming
PIC18F2420/2520/4420/4520 devices can be serially
programmed while in the end application circuit. This is
simply done with two lines for clock and data and three
other lines for power, ground and the programming
voltage. This allows customers to manufacture boards
with unprogrammed devices and then program the
microcontroller just before shipping the product. This
also allows the most recent firmware or a custom
firmware to be programmed.
23.8 In-Circuit Debugger
When the DEBUG configuration bit is programmed to a
‘0’, the In-Circuit Debugger functionality is enabled.
This function allows simple debugging functions when
used with MPLAB® IDE. When the microcontroller has
this feature enabled, some resources are not available
for general use. Table 23-4 shows which resources are
required by the background debugger.
TABLE 23-4: DEBUGGER RESOURCES
To use the In-Circuit Debugger function of the microcontroller,
the design must implement In-Circuit Serial
Programming connections to MCLR/VPP/RE3, VDD,
VSS, RB7 and RB6. This will interface to the In-Circuit
Debugger module available from Microchip or one of
the third party development tool companies.
23.9 Single-Supply ICSP Programming
The LVP configuration bit enables Single-Supply ICSP
Programming (formerly known as Low-Voltage ICSP
Programming or LVP). When Single-Supply Programming
is enabled, the microcontroller can be programmed
without requiring high voltage being applied to the
MCLR/VPP/RE3 pin, but the RB5/KBI1/PGM pin is then
dedicated to controlling Program mode entry and is not
available as a general purpose I/O pin.
While programming, using Single-Supply Programming
mode, VDD is applied to the MCLR/VPP/RE3 pin as in
normal execution mode. To enter Programming mode,
VDD is applied to the PGM pin.
If Single-Supply ICSP Programming mode will not be
used, the LVP bit can be cleared. RB5/KBI1/PGM then
becomes available as the digital I/O pin, RB5. The LVP
bit may be set or cleared only when using standard
high-voltage programming (VIHH applied to the MCLR/
VPP/RE3 pin). Once LVP has been disabled, only the
standard high-voltage programming is available and
must be used to program the device.
Memory that is not code-protected can be erased using
either a block erase, or erased row by row, then written
at any specified VDD. If code-protected memory is to be
erased, a block erase is required. If a block erase is to
be performed when using Low-Voltage Programming,
the device must be supplied with VDD of 4.5V to 5.5V.
I/O pins: RB6, RB7
Stack: 2 levels
Program Memory: 512 bytes
Data Memory: 10 bytes
Note 1: High-voltage programming is always
available, regardless of the state of the
LVP bit or the PGM pin, by applying VIHH
to the MCLR pin.
2: By default, Single-Supply ICSP is
enabled in unprogrammed devices (as
supplied from Microchip) and erased
devices.
3: When Single-Supply Programming is
enabled, the RB5 pin can no longer be
used as a general purpose I/O pin.
4: When LVP is enabled, externally pull the
PGM pin to VSS to allow normal program
execution.
© 2007 Microchip Technology Inc. Preliminary DS39631B-page 267
PIC18F2420/2520/4420/4520
24.0 INSTRUCTION SET SUMMARY
PIC18F2420/2520/4420/4520 devices incorporate the
standard set of 75 PIC18 core instructions, as well as an
extended set of 8 new instructions, for the optimization
of code that is recursive or that utilizes a software stack.
The extended set is discussed later in this section.
24.1 Standard Instruction Set
The standard PIC18 instruction set adds many
enhancements to the previous PIC® instruction sets,
while maintaining an easy migration from these PIC
instruction sets. Most instructions are a single program
memory word (16 bits), but there are four instructions
that require two program memory locations.
Each single-word instruction is a 16-bit word divided
into an opcode, which specifies the instruction type and
one or more operands, which further specify the
operation of the instruction.
The instruction set is highly orthogonal and is grouped
into four basic categories:
• Byte-oriented operations
• Bit-oriented operations
• Literal operations
• Control operations
The PIC18 instruction set summary in Table 24-2 lists
byte-oriented, bit-oriented, literal and control
operations. Table 24-1 shows the opcode field
descriptions.
Most byte-oriented instructions have three operands:
1. The file register (specified by ‘f’)
2. The destination of the result (specified by ‘d’)
3. The accessed memory (specified by ‘a’)
The file register designator ‘f’ specifies which file
register is to be used by the instruction. The destination
designator ‘d’ specifies where the result of the operation
is to be placed. If ‘d’ is zero, the result is placed in
the WREG register. If ‘d’ is one, the result is placed in
the file register specified in the instruction.
All bit-oriented instructions have three operands:
1. The file register (specified by ‘f’)
2. The bit in the file register (specified by ‘b’)
3. The accessed memory (specified by ‘a’)
The bit field designator ‘b’ selects the number of the bit
affected by the operation, while the file register
designator ‘f’ represents the number of the file in which
the bit is located.
The literal instructions may use some of the following
operands:
• A literal value to be loaded into a file register
(specified by ‘k’)
• The desired FSR register to load the literal value
into (specified by ‘f’)
• No operand required
(specified by ‘—’)
The control instructions may use some of the following
operands:
• A program memory address (specified by ‘n’)
• The mode of the CALL or RETURN instructions
(specified by ‘s’)
• The mode of the table read and table write
instructions (specified by ‘m’)
• No operand required
(specified by ‘—’)
All instructions are a single word, except for four
double-word instructions. These instructions were
made double-word to contain the required information
in 32 bits. In the second word, the 4 MSbs are ‘1’s. If
this second word is executed as an instruction (by
itself), it will execute as a NOP.
All single-word instructions are executed in a single
instruction cycle, unless a conditional test is true or the
program counter is changed as a result of the instruction.
In these cases, the execution takes two instruction
cycles, with the additional instruction cycle(s) executed
as a NOP.
The double-word instructions execute in two instruction
cycles.
One instruction cycle consists of four oscillator periods.
Thus, for an oscillator frequency of 4 MHz, the normal
instruction execution time is 1 μs. If a conditional test is
true, or the program counter is changed as a result of
an instruction, the instruction execution time is 2 μs.
Two-word branch instructions (if true) would take 3 μs.
Figure 24-1 shows the general formats that the instructions
can have. All examples use the convention ‘nnh’
to represent a hexadecimal number.
The Instruction Set Summary, shown in Table 24-2,
lists the standard instructions recognized by the
Microchip Assembler (MPASMTM).
Section 24.1.1 “Standard Instruction Set” provides
a description of each instruction.
PIC18F2420/2520/4420/4520
DS39631B-page 268 Preliminary © 2007 Microchip Technology Inc.
TABLE 24-1: OPCODE FIELD DESCRIPTIONS
Field Description
a RAM access bit
a = 0: RAM location in Access RAM (BSR register is ignored)
a = 1: RAM bank is specified by BSR register
bbb Bit address within an 8-bit file register (0 to 7).
BSR Bank Select Register. Used to select the current RAM bank.
C, DC, Z, OV, N ALU Status bits: Carry, Digit Carry, Zero, Overflow, Negative.
d Destination select bit
d = 0: store result in WREG
d = 1: store result in file register f
dest Destination: either the WREG register or the specified register file location.
f 8-bit Register file address (00h to FFh) or 2-bit FSR designator (0h to 3h).
fs 12-bit Register file address (000h to FFFh). This is the source address.
fd 12-bit Register file address (000h to FFFh). This is the destination address.
GIE Global Interrupt Enable bit.
k Literal field, constant data or label (may be either an 8-bit, 12-bit or a 20-bit value).
label Label name.
mm The mode of the TBLPTR register for the table read and table write instructions.
Only used with table read and table write instructions:
* No change to register (such as TBLPTR with table reads and writes)
*+ Post-Increment register (such as TBLPTR with table reads and writes)
*- Post-Decrement register (such as TBLPTR with table reads and writes)
+* Pre-Increment register (such as TBLPTR with table reads and writes)
n The relative address (2’s complement number) for relative branch instructions or the direct address for
Call/Branch and Return instructions.
PC Program Counter.
PCL Program Counter Low Byte.
PCH Program Counter High Byte.
PCLATH Program Counter High Byte Latch.
PCLATU Program Counter Upper Byte Latch.
PD Power-down bit.
PRODH Product of Multiply High Byte.
PRODL Product of Multiply Low Byte.
s Fast Call/Return mode select bit
s = 0: do not update into/from shadow registers
s = 1: certain registers loaded into/from shadow registers (Fast mode)
TBLPTR 21-bit Table Pointer (points to a Program Memory location).
TABLAT 8-bit Table Latch.
TO Time-out bit.
TOS Top-of-Stack.
u Unused or unchanged.
WDT Watchdog Timer.
WREG Working register (accumulator).
x Don’t care (‘0’ or ‘1’). The assembler will generate code with x = 0. It is the recommended form of use for
compatibility with all Microchip software tools.
zs 7-bit offset value for indirect addressing of register files (source).
zd 7-bit offset value for indirect addressing of register files (destination).
{ } Optional argument.
[text] Indicates an indexed address.
(text) The contents of text.
[expr] Specifies bit n of the register indicated by the pointer expr.
→ Assigned to.
< > Register bit field.
∈ In the set of.
italics User defined term (font is Courier).
© 2007 Microchip Technology Inc. Preliminary DS39631B-page 269
PIC18F2420/2520/4420/4520
FIGURE 24-1: GENERAL FORMAT FOR INSTRUCTIONS
Byte-oriented file register operations
15 10 9 8 7 0
d = 0 for result destination to be WREG register
OPCODE d a f (FILE #)
d = 1 for result destination to be file register (f)
a = 0 to force Access Bank
Bit-oriented file register operations
15 12 11 9 8 7 0
OPCODE b (BIT #) a f (FILE #)
b = 3-bit position of bit in file register (f)
Literal operations
15 8 7 0
OPCODE k (literal)
k = 8-bit immediate value
Byte to Byte move operations (2-word)
15 12 11 0
OPCODE f (Source FILE #)
CALL, GOTO and Branch operations
15 8 7 0
OPCODE n<7:0> (literal)
n = 20-bit immediate value
a = 1 for BSR to select bank
f = 8-bit file register address
a = 0 to force Access Bank
a = 1 for BSR to select bank
f = 8-bit file register address
15 12 11 0
1111 n<19:8> (literal)
15 12 11 0
1111 f (Destination FILE #)
f = 12-bit file register address
Control operations
Example Instruction
ADDWF MYREG, W, B
MOVFF MYREG1, MYREG2
BSF MYREG, bit, B
MOVLW 7Fh
GOTO Label
15 8 7 0
OPCODE n<7:0> (literal)
15 12 11 0
1111 n<19:8> (literal)
CALL MYFUNC
15 11 10 0
OPCODE n<10:0> (literal)
S = Fast bit
BRA MYFUNC
15 8 7 0
OPCODE n<7:0> (literal) BC MYFUNC
S
PIC18F2420/2520/4420/4520
DS39631B-page 270 Preliminary © 2007 Microchip Technology Inc.
TABLE 24-2: PIC18FXXXX INSTRUCTION SET
Mnemonic,
Operands
Description Cycles
16-Bit Instruction Word Status
Affected
Notes
MSb LSb
BYTE-ORIENTED OPERATIONS
ADDWF
ADDWFC
ANDWF
CLRF
COMF
CPFSEQ
CPFSGT
CPFSLT
DECF
DECFSZ
DCFSNZ
INCF
INCFSZ
INFSNZ
IORWF
MOVF
MOVFF
MOVWF
MULWF
NEGF
RLCF
RLNCF
RRCF
RRNCF
SETF
SUBFWB
SUBWF
SUBWFB
SWAPF
TSTFSZ
XORWF
f, d, a
f, d, a
f, d, a
f, a
f, d, a
f, a
f, a
f, a
f, d, a
f, d, a
f, d, a
f, d, a
f, d, a
f, d, a
f, d, a
f, d, a
fs, fd
f, a
f, a
f, a
f, d, a
f, d, a
f, d, a
f, d, a
f, a
f, d, a
f, d, a
f, d, a
f, d, a
f, a
f, d, a
Add WREG and f
Add WREG and CARRY bit to f
AND WREG with f
Clear f
Complement f
Compare f with WREG, skip =
Compare f with WREG, skip >
Compare f with WREG, skip <
Decrement f
Decrement f, Skip if 0
Decrement f, Skip if Not 0
Increment f
Increment f, Skip if 0
Increment f, Skip if Not 0
Inclusive OR WREG with f
Move f
Move fs (source) to 1st word
fd (destination) 2nd word
Move WREG to f
Multiply WREG with f
Negate f
Rotate Left f through Carry
Rotate Left f (No Carry)
Rotate Right f through Carry
Rotate Right f (No Carry)
Set f
Subtract f from WREG with
borrow
Subtract WREG from f
Subtract WREG from f with
borrow
Swap nibbles in f
Test f, skip if 0
Exclusive OR WREG with f
1
1
1
1
1
1 (2 or 3)
1 (2 or 3)
1 (2 or 3)
1
1 (2 or 3)
1 (2 or 3)
1
1 (2 or 3)
1 (2 or 3)
1
1
2
1
1
1
1
1
1
1
1
1
1
1
1
1 (2 or 3)
1
0010
0010
0001
0110
0001
0110
0110
0110
0000
0010
0100
0010
0011
0100
0001
0101
1100
1111
0110
0000
0110
0011
0100
0011
0100
0110
0101
0101
0101
0011
0110
0001
01da0
0da
01da
101a
11da
001a
010a
000a
01da
11da
11da
10da
11da
10da
00da
00da
ffff
ffff
111a
001a
110a
01da
01da
00da
00da
100a
01da
11da
10da
10da
011a
10da
ffff
ffff
ffff
ffff
ffff
ffff
ffff
ffff
ffff
ffff
ffff
ffff
ffff
ffff
ffff
ffff
ffff
ffff
ffff
ffff
ffff
ffff
ffff
ffff
ffff
ffff
ffff
ffff
ffff
ffff
ffff
ffff
ffff
ffff
ffff
ffff
ffff
ffff
ffff
ffff
ffff
ffff
ffff
ffff
ffff
ffff
ffff
ffff
ffff
ffff
ffff
ffff
ffff
ffff
ffff
ffff
ffff
ffff
ffff
ffff
ffff
ffff
ffff
ffff
C, DC, Z, OV, N
C, DC, Z, OV, N
Z, N
Z
Z, N
None
None
None
C, DC, Z, OV, N
None
None
C, DC, Z, OV, N
None
None
Z, N
Z, N
None
None
None
C, DC, Z, OV, N
C, Z, N
Z, N
C, Z, N
Z, N
None
C, DC, Z, OV, N
C, DC, Z, OV, N
C, DC, Z, OV, N
None
None
Z, N
1, 2
1, 2
1,2
2
1, 2
4
4
1, 2
1, 2, 3, 4
1, 2, 3, 4
1, 2
1, 2, 3, 4
4
1, 2
1, 2
1
1, 2
1, 2
1, 2
1, 2
4
1, 2
Note 1: When a Port register is modified as a function of itself (e.g., MOVF PORTB, 1, 0), the value used will be that value
present on the pins themselves. For example, if the data latch is ‘1’ for a pin configured as input and is driven low by an
external device, the data will be written back with a ‘0’.
2: If this instruction is executed on the TMR0 register (and where applicable, ‘d’ = 1), the prescaler will be cleared if
assigned.
3: If Program Counter (PC) is modified or a conditional test is true, the instruction requires two cycles. The second cycle is
executed as a NOP.
4: Some instructions are two-word instructions. The second word of these instructions will be executed as a NOP unless the
first word of the instruction retrieves the information embedded in these 16 bits. This ensures that all program memory
locations have a valid instruction.
© 2007 Microchip Technology Inc. Preliminary DS39631B-page 271
PIC18F2420/2520/4420/4520
BIT-ORIENTED OPERATIONS
BCF
BSF
BTFSC
BTFSS
BTG
f, b, a
f, b, a
f, b, a
f, b, a
f, d, a
Bit Clear f
Bit Set f
Bit Test f, Skip if Clear
Bit Test f, Skip if Set
Bit Toggle f
1
1
1 (2 or 3)
1 (2 or 3)
1
1001
1000
1011
1010
0111
bbba
bbba
bbba
bbba
bbba
ffff
ffff
ffff
ffff
ffff
ffff
ffff
ffff
ffff
ffff
None
None
None
None
None
1, 2
1, 2
3, 4
3, 4
1, 2
CONTROL OPERATIONS
BC
BN
BNC
BNN
BNOV
BNZ
BOV
BRA
BZ
CALL
CLRWDT
DAW
GOTO
NOP
NOP
POP
PUSH
RCALL
RESET
RETFIE
RETLW
RETURN
SLEEP
n
n
n
n
n
n
n
n
n
n, s
—
—
n
—
—
—
—
n
s
k
s
—
Branch if Carry
Branch if Negative
Branch if Not Carry
Branch if Not Negative
Branch if Not Overflow
Branch if Not Zero
Branch if Overflow
Branch Unconditionally
Branch if Zero
Call subroutine 1st word
2nd word
Clear Watchdog Timer
Decimal Adjust WREG
Go to address 1st word
2nd word
No Operation
No Operation
Pop top of return stack (TOS)
Push top of return stack (TOS)
Relative Call
Software device Reset
Return from interrupt enable
Return with literal in WREG
Return from Subroutine
Go into Standby mode
1 (2)
1 (2)
1 (2)
1 (2)
1 (2)
1 (2)
1 (2)
2
1 (2)
2
1
1
2
1
1
1
1
2
1
2
2
2
1
1110
1110
1110
1110
1110
1110
1110
1101
1110
1110
1111
0000
0000
1110
1111
0000
1111
0000
0000
1101
0000
0000
0000
0000
0000
0010
0110
0011
0111
0101
0001
0100
0nnn
0000
110s
kkkk
0000
0000
1111
kkkk
0000
xxxx
0000
0000
1nnn
0000
0000
1100
0000
0000
nnnn
nnnn
nnnn
nnnn
nnnn
nnnn
nnnn
nnnn
nnnn
kkkk
kkkk
0000
0000
kkkk
kkkk
0000
xxxx
0000
0000
nnnn
1111
0001
kkkk
0001
0000
nnnn
nnnn
nnnn
nnnn
nnnn
nnnn
nnnn
nnnn
nnnn
kkkk
kkkk
0100
0111
kkkk
kkkk
0000
xxxx
0110
0101
nnnn
1111
000s
kkkk
001s
0011
None
None
None
None
None
None
None
None
None
None
TO, PD
C
None
None
None
None
None
None
All
GIE/GIEH,
PEIE/GIEL
None
None
TO, PD
4
TABLE 24-2: PIC18FXXXX INSTRUCTION SET (CONTINUED)
Mnemonic,
Operands
Description Cycles
16-Bit Instruction Word Status
Affected
Notes
MSb LSb
Note 1: When a Port register is modified as a function of itself (e.g., MOVF PORTB, 1, 0), the value used will be that value
present on the pins themselves. For example, if the data latch is ‘1’ for a pin configured as input and is driven low by an
external device, the data will be written back with a ‘0’.
2: If this instruction is executed on the TMR0 register (and where applicable, ‘d’ = 1), the prescaler will be cleared if
assigned.
3: If Program Counter (PC) is modified or a conditional test is true, the instruction requires two cycles. The second cycle is
executed as a NOP.
4: Some instructions are two-word instructions. The second word of these instructions will be executed as a NOP unless the
first word of the instruction retrieves the information embedded in these 16 bits. This ensures that all program memory
locations have a valid instruction.
PIC18F2420/2520/4420/4520
DS39631B-page 272 Preliminary © 2007 Microchip Technology Inc.
LITERAL OPERATIONS
ADDLW
ANDLW
IORLW
LFSR
MOVLB
MOVLW
MULLW
RETLW
SUBLW
XORLW
k
k
k
f, k
k
k
k
k
k
k
Add literal and WREG
AND literal with WREG
Inclusive OR literal with WREG
Move literal (12-bit) 2nd word
to FSR(f) 1st word
Move literal to BSR<3:0>
Move literal to WREG
Multiply literal with WREG
Return with literal in WREG
Subtract WREG from literal
Exclusive OR literal with WREG
1
1
1
2
1
1
1
2
1
1
0000
0000
0000
1110
1111
0000
0000
0000
0000
0000
0000
1111
1011
1001
1110
0000
0001
1110
1101
1100
1000
1010
kkkk
kkkk
kkkk
00ff
kkkk
0000
kkkk
kkkk
kkkk
kkkk
kkkk
kkkk
kkkk
kkkk
kkkk
kkkk
kkkk
kkkk
kkkk
kkkk
kkkk
kkkk
C, DC, Z, OV, N
Z, N
Z, N
None
None
None
None
None
C, DC, Z, OV, N
Z, N
DATA MEMORY ↔ PROGRAM MEMORY OPERATIONS
TBLRD*
TBLRD*+
TBLRD*-
TBLRD+*
TBLWT*
TBLWT*+
TBLWT*-
TBLWT+*
Table Read
Table Read with post-increment
Table Read with post-decrement
Table Read with pre-increment
Table Write
Table Write with post-increment
Table Write with post-decrement
Table Write with pre-increment
2
2
0000
0000
0000
0000
0000
0000
0000
0000
0000
0000
0000
0000
0000
0000
0000
0000
0000
0000
0000
0000
0000
0000
0000
0000
1000
1001
1010
1011
1100
1101
1110
1111
None
None
None
None
None
None
None
None
TABLE 24-2: PIC18FXXXX INSTRUCTION SET (CONTINUED)
Mnemonic,
Operands
Description Cycles
16-Bit Instruction Word Status
Affected
Notes
MSb LSb
Note 1: When a Port register is modified as a function of itself (e.g., MOVF PORTB, 1, 0), the value used will be that value
present on the pins themselves. For example, if the data latch is ‘1’ for a pin configured as input and is driven low by an
external device, the data will be written back with a ‘0’.
2: If this instruction is executed on the TMR0 register (and where applicable, ‘d’ = 1), the prescaler will be cleared if
assigned.
3: If Program Counter (PC) is modified or a conditional test is true, the instruction requires two cycles. The second cycle is
executed as a NOP.
4: Some instructions are two-word instructions. The second word of these instructions will be executed as a NOP unless the
first word of the instruction retrieves the information embedded in these 16 bits. This ensures that all program memory
locations have a valid instruction.
© 2007 Microchip Technology Inc. Preliminary DS39631B-page 273
PIC18F2420/2520/4420/4520
24.1.1 STANDARD INSTRUCTION SET
ADDLW ADD literal to W
Syntax: ADDLW k
Operands: 0 ≤ k ≤ 255
Operation: (W) + k → W
Status Affected: N, OV, C, DC, Z
Encoding: 0000 1111 kkkk kkkk
Description: The contents of W are added to the
8-bit literal ‘k’ and the result is placed in
W.
Words: 1
Cycles: 1
Q Cycle Activity:
Q1 Q2 Q3 Q4
Decode Read
literal ‘k’
Process
Data
Write to W
Example: ADDLW 15h
Before Instruction
W = 10h
After Instruction
W = 25h
ADDWF ADD W to f
Syntax: ADDWF f {,d {,a}}
Operands: 0 ≤ f ≤ 255
d ∈ [0,1]
a ∈ [0,1]
Operation: (W) + (f) → dest
Status Affected: N, OV, C, DC, Z
Encoding: 0010 01da ffff ffff
Description: Add W to register ‘f’. If ‘d’ is ‘0’, the
result is stored in W. If ‘d’ is ‘1’, the
result is stored back in register ‘f’
(default).
If ‘a’ is ‘0’, the Access Bank is selected.
If ‘a’ is ‘1’, the BSR is used to select the
GPR bank (default).
If ‘a’ is ‘0’ and the extended instruction
set is enabled, this instruction operates
in Indexed Literal Offset Addressing
mode whenever f ≤ 95 (5Fh). See
Section 24.2.3 “Byte-Oriented and
Bit-Oriented Instructions in Indexed
Literal Offset Mode” for details.
Words: 1
Cycles: 1
Q Cycle Activity:
Q1 Q2 Q3 Q4
Decode Read
register ‘f’
Process
Data
Write to
destination
Example: ADDWF REG, 0, 0
Before Instruction
W = 17h
REG = 0C2h
After Instruction
W = 0D9h
REG = 0C2h
Note: All PIC18 instructions may take an optional label argument preceding the instruction mnemonic for use in
symbolic addressing. If a label is used, the instruction format then becomes: {label} instruction argument(s).
PIC18F2420/2520/4420/4520
DS39631B-page 274 Preliminary © 2007 Microchip Technology Inc.
ADDWFC ADD W and CARRY bit to f
Syntax: ADDWFC f {,d {,a}}
Operands: 0 ≤ f ≤ 255
d ∈ [0,1]
a ∈ [0,1]
Operation: (W) + (f) + (C) → dest
Status Affected: N,OV, C, DC, Z
Encoding: 0010 00da ffff ffff
Description: Add W, the CARRY flag and data memory
location ‘f’. If ‘d’ is ‘0’, the result is
placed in W. If ‘d’ is ‘1’, the result is
placed in data memory location ‘f’.
If ‘a’ is ‘0’, the Access Bank is selected.
If ‘a’ is ‘1’, the BSR is used to select the
GPR bank (default).
If ‘a’ is ‘0’ and the extended instruction
set is enabled, this instruction operates
in Indexed Literal Offset Addressing
mode whenever f ≤ 95 (5Fh). See
Section 24.2.3 “Byte-Oriented and
Bit-Oriented Instructions in Indexed
Literal Offset Mode” for details.
Words: 1
Cycles: 1
Q Cycle Activity:
Q1 Q2 Q3 Q4
Decode Read
register ‘f’
Process
Data
Write to
destination
Example: ADDWFC REG, 0, 1
Before Instruction
CARRY bit = 1
REG = 02h
W = 4Dh
After Instruction
CARRY bit = 0
REG = 02h
W = 50h
ANDLW AND literal with W
Syntax: ANDLW k
Operands: 0 ≤ k ≤ 255
Operation: (W) .AND. k → W
Status Affected: N, Z
Encoding: 0000 1011 kkkk kkkk
Description: The contents of W are AND’ed with the
8-bit literal ‘k’. The result is placed in W.
Words: 1
Cycles: 1
Q Cycle Activity:
Q1 Q2 Q3 Q4
Decode Read literal
‘k’
Process
Data
Write to W
Example: ANDLW 05Fh
Before Instruction
W = A3h
After Instruction
W = 03h
© 2007 Microchip Technology Inc. Preliminary DS39631B-page 275
PIC18F2420/2520/4420/4520
ANDWF AND W with f
Syntax: ANDWF f {,d {,a}}
Operands: 0 ≤ f ≤ 255
d ∈ [0,1]
a ∈ [0,1]
Operation: (W) .AND. (f) → dest
Status Affected: N, Z
Encoding: 0001 01da ffff ffff
Description: The contents of W are AND’ed with
register ‘f’. If ‘d’ is ‘0’, the result is stored
in W. If ‘d’ is ‘1’, the result is stored back
in register ‘f’ (default).
If ‘a’ is ‘0’, the Access Bank is selected.
If ‘a’ is ‘1’, the BSR is used to select the
GPR bank (default).
If ‘a’ is ‘0’ and the extended instruction
set is enabled, this instruction operates
in Indexed Literal Offset Addressing
mode whenever f ≤ 95 (5Fh). See
Section 24.2.3 “Byte-Oriented and
Bit-Oriented Instructions in Indexed
Literal Offset Mode” for details.
Words: 1
Cycles: 1
Q Cycle Activity:
Q1 Q2 Q3 Q4
Decode Read
register ‘f’
Process
Data
Write to
destination
Example: ANDWF REG, 0, 0
Before Instruction
W = 17h
REG = C2h
After Instruction
W = 02h
REG = C2h
BC Branch if Carry
Syntax: BC n
Operands: -128 ≤ n ≤ 127
Operation: if CARRY bit is ‘1’
(PC) + 2 + 2n → PC
Status Affected: None
Encoding: 1110 0010 nnnn nnnn
Description: If the CARRY bit is ‘1’, then the program
will branch.
The 2’s complement number ‘2n’ is
added to the PC. Since the PC will have
incremented to fetch the next
instruction, the new address will be
PC + 2 + 2n. This instruction is then a
two-cycle instruction.
Words: 1
Cycles: 1(2)
Q Cycle Activity:
If Jump:
Q1 Q2 Q3 Q4
Decode Read literal
‘n’
Process
Data
Write to PC
No
operation
No
operation
No
operation
No
operation
If No Jump:
Q1 Q2 Q3 Q4
Decode Read literal
‘n’
Process
Data
No
operation
Example: HERE BC 5
Before Instruction
PC = address (HERE)
After Instruction
If CARRY = 1;
PC = address (HERE + 12)
If CARRY = 0;
PC = address (HERE + 2)
PIC18F2420/2520/4420/4520
DS39631B-page 276 Preliminary © 2007 Microchip Technology Inc.
BCF Bit Clear f
Syntax: BCF f, b {,a}
Operands: 0 ≤ f ≤ 255
0 ≤ b ≤ 7
a ∈ [0,1]
Operation: 0 → f
Status Affected: None
Encoding: 1001 bbba ffff ffff
Description: Bit ‘b’ in register ‘f’ is cleared.
If ‘a’ is ‘0’, the Access Bank is selected.
If ‘a’ is ‘1’, the BSR is used to select the
GPR bank (default).
If ‘a’ is ‘0’ and the extended instruction
set is enabled, this instruction operates
in Indexed Literal Offset Addressing
mode whenever f ≤ 95 (5Fh). See
Section 24.2.3 “Byte-Oriented and
Bit-Oriented Instructions in Indexed
Literal Offset Mode” for details.
Words: 1
Cycles: 1
Q Cycle Activity:
Q1 Q2 Q3 Q4
Decode Read
register ‘f’
Process
Data
Write
register ‘f’
Example: BCF FLAG_REG, 7, 0
Before Instruction
FLAG_REG = C7h
After Instruction
FLAG_REG = 47h
BN Branch if Negative
Syntax: BN n
Operands: -128 ≤ n ≤ 127
Operation: if NEGATIVE bit is ‘1’
(PC) + 2 + 2n → PC
Status Affected: None
Encoding: 1110 0110 nnnn nnnn
Description: If the NEGATIVE bit is ‘1’, then the
program will branch.
The 2’s complement number ‘2n’ is
added to the PC. Since the PC will have
incremented to fetch the next
instruction, the new address will be
PC + 2 + 2n. This instruction is then a
two-cycle instruction.
Words: 1
Cycles: 1(2)
Q Cycle Activity:
If Jump:
Q1 Q2 Q3 Q4
Decode Read literal
‘n’
Process
Data
Write to PC
No
operation
No
operation
No
operation
No
operation
If No Jump:
Q1 Q2 Q3 Q4
Decode Read literal
‘n’
Process
Data
No
operation
Example: HERE BN Jump
Before Instruction
PC = address (HERE)
After Instruction
If NEGATIVE = 1;
PC = address (Jump)
If NEGATIVE = 0;
PC = address (HERE + 2)
© 2007 Microchip Technology Inc. Preliminary DS39631B-page 277
PIC18F2420/2520/4420/4520
BNC Branch if Not Carry
Syntax: BNC n
Operands: -128 ≤ n ≤ 127
Operation: if CARRY bit is ‘0’
(PC) + 2 + 2n → PC
Status Affected: None
Encoding: 1110 0011 nnnn nnnn
Description: If the CARRY bit is ‘0’, then the program
will branch.
The 2’s complement number ‘2n’ is
added to the PC. Since the PC will have
incremented to fetch the next
instruction, the new address will be
PC + 2 + 2n. This instruction is then a
two-cycle instruction.
Words: 1
Cycles: 1(2)
Q Cycle Activity:
If Jump:
Q1 Q2 Q3 Q4
Decode Read literal
‘n’
Process
Data
Write to PC
No
operation
No
operation
No
operation
No
operation
If No Jump:
Q1 Q2 Q3 Q4
Decode Read literal
‘n’
Process
Data
No
operation
Example: HERE BNC Jump
Before Instruction
PC = address (HERE)
After Instruction
If CARRY = 0;
PC = address (Jump)
If CARRY = 1;
PC = address (HERE + 2)
BNN Branch if Not Negative
Syntax: BNN n
Operands: -128 ≤ n ≤ 127
Operation: if NEGATIVE bit is ‘0’
(PC) + 2 + 2n → PC
Status Affected: None
Encoding: 1110 0111 nnnn nnnn
Description: If the NEGATIVE bit is ‘0’, then the
program will branch.
The 2’s complement number ‘2n’ is
added to the PC. Since the PC will have
incremented to fetch the next
instruction, the new address will be
PC + 2 + 2n. This instruction is then a
two-cycle instruction.
Words: 1
Cycles: 1(2)
Q Cycle Activity:
If Jump:
Q1 Q2 Q3 Q4
Decode Read literal
‘n’
Process
Data
Write to PC
No
operation
No
operation
No
operation
No
operation
If No Jump:
Q1 Q2 Q3 Q4
Decode Read literal
‘n’
Process
Data
No
operation
Example: HERE BNN Jump
Before Instruction
PC = address (HERE)
After Instruction
If NEGATIVE = 0;
PC = address (Jump)
If NEGATIVE = 1;
PC = address (HERE + 2)
PIC18F2420/2520/4420/4520
DS39631B-page 278 Preliminary © 2007 Microchip Technology Inc.
BNOV Branch if Not Overflow
Syntax: BNOV n
Operands: -128 ≤ n ≤ 127
Operation: if OVERFLOW bit is ‘0’
(PC) + 2 + 2n → PC
Status Affected: None
Encoding: 1110 0101 nnnn nnnn
Description: If the OVERFLOW bit is ‘0’, then the
program will branch.
The 2’s complement number ‘2n’ is
added to the PC. Since the PC will have
incremented to fetch the next
instruction, the new address will be
PC + 2 + 2n. This instruction is then a
two-cycle instruction.
Words: 1
Cycles: 1(2)
Q Cycle Activity:
If Jump:
Q1 Q2 Q3 Q4
Decode Read literal
‘n’
Process
Data
Write to PC
No
operation
No
operation
No
operation
No
operation
If No Jump:
Q1 Q2 Q3 Q4
Decode Read literal
‘n’
Process
Data
No
operation
Example: HERE BNOV Jump
Before Instruction
PC = address (HERE)
After Instruction
If OVERFLOW= 0;
PC = address (Jump)
If OVERFLOW= 1;
PC = address (HERE + 2)
BNZ Branch if Not Zero
Syntax: BNZ n
Operands: -128 ≤ n ≤ 127
Operation: if ZERO bit is ‘0’
(PC) + 2 + 2n → PC
Status Affected: None
Encoding: 1110 0001 nnnn nnnn
Description: If the ZERO bit is ‘0’, then the program
will branch.
The 2’s complement number ‘2n’ is
added to the PC. Since the PC will have
incremented to fetch the next
instruction, the new address will be
PC + 2 + 2n. This instruction is then a
two-cycle instruction.
Words: 1
Cycles: 1(2)
Q Cycle Activity:
If Jump:
Q1 Q2 Q3 Q4
Decode Read literal
‘n’
Process
Data
Write to PC
No
operation
No
operation
No
operation
No
operation
If No Jump:
Q1 Q2 Q3 Q4
Decode Read literal
‘n’
Process
Data
No
operation
Example: HERE BNZ Jump
Before Instruction
PC = address (HERE)
After Instruction
If ZERO = 0;
PC = address (Jump)
If ZERO = 1;
PC = address (HERE + 2)
© 2007 Microchip Technology Inc. Preliminary DS39631B-page 279
PIC18F2420/2520/4420/4520
BRA Unconditional Branch
Syntax: BRA n
Operands: -1024 ≤ n ≤ 1023
Operation: (PC) + 2 + 2n → PC
Status Affected: None
Encoding: 1101 0nnn nnnn nnnn
Description: Add the 2’s complement number ‘2n’ to
the PC. Since the PC will have incremented
to fetch the next instruction, the
new address will be PC + 2 + 2n. This
instruction is a two-cycle instruction.
Words: 1
Cycles: 2
Q Cycle Activity:
Q1 Q2 Q3 Q4
Decode Read literal
‘n’
Process
Data
Write to PC
No
operation
No
operation
No
operation
No
operation
Example: HERE BRA Jump
Before Instruction
PC = address (HERE)
After Instruction
PC = address (Jump)
BSF Bit Set f
Syntax: BSF f, b {,a}
Operands: 0 ≤ f ≤ 255
0 ≤ b ≤ 7
a ∈ [0,1]
Operation: 1 → f
Status Affected: None
Encoding: 1000 bbba ffff ffff
Description: Bit ‘b’ in register ‘f’ is set.
If ‘a’ is ‘0’, the Access Bank is selected.
If ‘a’ is ‘1’, the BSR is used to select the
GPR bank (default).
If ‘a’ is ‘0’ and the extended instruction
set is enabled, this instruction operates
in Indexed Literal Offset Addressing
mode whenever f ≤ 95 (5Fh). See
Section 24.2.3 “Byte-Oriented and
Bit-Oriented Instructions in Indexed
Literal Offset Mode” for details.
Words: 1
Cycles: 1
Q Cycle Activity:
Q1 Q2 Q3 Q4
Decode Read
register ‘f’
Process
Data
Write
register ‘f’
Example: BSF FLAG_REG, 7, 1
Before Instruction
FLAG_REG = 0Ah
After Instruction
FLAG_REG = 8Ah
PIC18F2420/2520/4420/4520
DS39631B-page 280 Preliminary © 2007 Microchip Technology Inc.
BTFSC Bit Test File, Skip if Clear
Syntax: BTFSC f, b {,a}
Operands: 0 ≤ f ≤ 255
0 ≤ b ≤ 7
a ∈ [0,1]
Operation: skip if (f) = 0
Status Affected: None
Encoding: 1011 bbba ffff ffff
Description: If bit ‘b’ in register ‘f’ is ‘0’, then the next
instruction is skipped. If bit ‘b’ is ‘0’, then
the next instruction fetched during the
current instruction execution is discarded
and a NOP is executed instead, making
this a two-cycle instruction.
If ‘a’ is ‘0’, the Access Bank is selected. If
‘a’ is ‘1’, the BSR is used to select the
GPR bank (default).
If ‘a’ is ‘0’ and the extended instruction
set is enabled, this instruction operates in
Indexed Literal Offset Addressing
mode whenever f ≤ 95 (5Fh).
See Section 24.2.3 “Byte-Oriented and
Bit-Oriented Instructions in Indexed
Literal Offset Mode” for details.
Words: 1
Cycles: 1(2)
Note: 3 cycles if skip and followed
by a 2-word instruction.
Q Cycle Activity:
Q1 Q2 Q3 Q4
Decode Read
register ‘f’
Process
Data
No
operation
If skip:
Q1 Q2 Q3 Q4
No
operation
No
operation
No
operation
No
operation
If skip and followed by 2-word instruction:
Q1 Q2 Q3 Q4
No
operation
No
operation
No
operation
No
operation
No
operation
No
operation
No
operation
No
operation
Example: HERE
FALSE
TRUE
BTFSC
:
:
FLAG, 1, 0
Before Instruction
PC = address (HERE)
After Instruction
If FLAG<1> = 0;
PC = address (TRUE)
If FLAG<1> = 1;
PC = address (FALSE)
BTFSS Bit Test File, Skip if Set
Syntax: BTFSS f, b {,a}
Operands: 0 ≤ f ≤ 255
0 ≤ b < 7
a ∈ [0,1]
Operation: skip if (f) = 1
Status Affected: None
Encoding: 1010 bbba ffff ffff
Description: If bit ‘b’ in register ‘f’ is ‘1’, then the next
instruction is skipped. If bit ‘b’ is ‘1’, then
the next instruction fetched during the
current instruction execution is discarded
and a NOP is executed instead, making
this a two-cycle instruction.
If ‘a’ is ‘0’, the Access Bank is selected. If
‘a’ is ‘1’, the BSR is used to select the
GPR bank (default).
If ‘a’ is ‘0’ and the extended instruction
set is enabled, this instruction operates
in Indexed Literal Offset Addressing
mode whenever f ≤ 95 (5Fh).
See Section 24.2.3 “Byte-Oriented and
Bit-Oriented Instructions in Indexed
Literal Offset Mode” for details.
Words: 1
Cycles: 1(2)
Note: 3 cycles if skip and followed
by a 2-word instruction.
Q Cycle Activity:
Q1 Q2 Q3 Q4
Decode Read
register ‘f’
Process
Data
No
operation
If skip:
Q1 Q2 Q3 Q4
No
operation
No
operation
No
operation
No
operation
If skip and followed by 2-word instruction:
Q1 Q2 Q3 Q4
No
operation
No
operation
No
operation
No
operation
No
operation
No
operation
No
operation
No
operation
Example: HERE
FALSE
TRUE
BTFSS
:
:
FLAG, 1, 0
Before Instruction
PC = address (HERE)
After Instruction
If FLAG<1> = 0;
PC = address (FALSE)
If FLAG<1> = 1;
PC = address (TRUE)
© 2007 Microchip Technology Inc. Preliminary DS39631B-page 281
PIC18F2420/2520/4420/4520
BTG Bit Toggle f
Syntax: BTG f, b {,a}
Operands: 0 ≤ f ≤ 255
0 ≤ b < 7
a ∈ [0,1]
Operation: (f) → f
Status Affected: None
Encoding: 0111 bbba ffff ffff
Description: Bit ‘b’ in data memory location ‘f’ is
inverted.
If ‘a’ is ‘0’, the Access Bank is selected.
If ‘a’ is ‘1’, the BSR is used to select the
GPR bank (default).
If ‘a’ is ‘0’ and the extended instruction
set is enabled, this instruction operates
in Indexed Literal Offset Addressing
mode whenever f ≤ 95 (5Fh). See
Section 24.2.3 “Byte-Oriented and
Bit-Oriented Instructions in Indexed
Literal Offset Mode” for details.
Words: 1
Cycles: 1
Q Cycle Activity:
Q1 Q2 Q3 Q4
Decode Read
register ‘f’
Process
Data
Write
register ‘f’
Example: BTG PORTC, 4, 0
Before Instruction:
PORTC = 0111 0101 [75h]
After Instruction:
PORTC = 0110 0101 [65h]
BOV Branch if Overflow
Syntax: BOV n
Operands: -128 ≤ n ≤ 127
Operation: if OVERFLOW bit is ‘1’
(PC) + 2 + 2n → PC
Status Affected: None
Encoding: 1110 0100 nnnn nnnn
Description: If the OVERFLOW bit is ‘1’, then the
program will branch.
The 2’s complement number ‘2n’ is
added to the PC. Since the PC will have
incremented to fetch the next
instruction, the new address will be
PC + 2 + 2n. This instruction is then a
two-cycle instruction.
Words: 1
Cycles: 1(2)
Q Cycle Activity:
If Jump:
Q1 Q2 Q3 Q4
Decode Read literal
‘n’
Process
Data
Write to PC
No
operation
No
operation
No
operation
No
operation
If No Jump:
Q1 Q2 Q3 Q4
Decode Read literal
‘n’
Process
Data
No
operation
Example: HERE BOV Jump
Before Instruction
PC = address (HERE)
After Instruction
If OVERFLOW= 1;
PC = address (Jump)
If OVERFLOW= 0;
PC = address (HERE + 2)
PIC18F2420/2520/4420/4520
DS39631B-page 282 Preliminary © 2007 Microchip Technology Inc.
BZ Branch if Zero
Syntax: BZ n
Operands: -128 ≤ n ≤ 127
Operation: if ZERO bit is ‘1’
(PC) + 2 + 2n → PC
Status Affected: None
Encoding: 1110 0000 nnnn nnnn
Description: If the ZERO bit is ‘1’, then the program
will branch.
The 2’s complement number ‘2n’ is
added to the PC. Since the PC will
have incremented to fetch the next
instruction, the new address will be
PC + 2 + 2n. This instruction is then a
two-cycle instruction.
Words: 1
Cycles: 1(2)
Q Cycle Activity:
If Jump:
Q1 Q2 Q3 Q4
Decode Read literal
‘n’
Process
Data
Write to PC
No
operation
No
operation
No
operation
No
operation
If No Jump:
Q1 Q2 Q3 Q4
Decode Read literal
‘n’
Process
Data
No
operation
Example: HERE BZ Jump
Before Instruction
PC = address (HERE)
After Instruction
If ZERO = 1;
PC = address (Jump)
If ZERO = 0;
PC = address (HERE + 2)
CALL Subroutine Call
Syntax: CALL k {,s}
Operands: 0 ≤ k ≤ 1048575
s ∈ [0,1]
Operation: (PC) + 4 → TOS,
k → PC<20:1>,
if s = 1
(W) → WS,
(Status) → STATUSS,
(BSR) → BSRS
Status Affected: None
Encoding:
1st word (k<7:0>)
2nd word(k<19:8>)
1110
1111
110s
k19kkk
k7kkk
kkkk
kkkk0
kkkk8
Description: Subroutine call of entire 2-Mbyte
memory range. First, return address
(PC + 4) is pushed onto the return
stack. If ‘s’ = 1, the W, Status and BSR
registers are also pushed into their
respective shadow registers, WS,
STATUSS and BSRS. If ‘s’ = 0, no
update occurs (default). Then, the
20-bit value ‘k’ is loaded into PC<20:1>.
CALL is a two-cycle instruction.
Words: 2
Cycles: 2
Q Cycle Activity:
Q1 Q2 Q3 Q4
Decode Read literal
‘k’<7:0>,
PUSH PC to
stack
Read literal
‘k’<19:8>,
Write to PC
No
operation
No
operation
No
operation
No
operation
Example: HERE CALL THERE, 1
Before Instruction
PC = address (HERE)
After Instruction
PC = address (THERE)
TOS = address (HERE + 4)
WS = W
BSRS = BSR
STATUSS= Status
© 2007 Microchip Technology Inc. Preliminary DS39631B-page 283
PIC18F2420/2520/4420/4520
CLRF Clear f
Syntax: CLRF f {,a}
Operands: 0 ≤ f ≤ 255
a ∈ [0,1]
Operation: 000h → f
1 → Z
Status Affected: Z
Encoding: 0110 101a ffff ffff
Description: Clears the contents of the specified
register.
If ‘a’ is ‘0’, the Access Bank is selected.
If ‘a’ is ‘1’, the BSR is used to select the
GPR bank (default).
If ‘a’ is ‘0’ and the extended instruction
set is enabled, this instruction operates
in Indexed Literal Offset Addressing
mode whenever f ≤ 95 (5Fh). See
Section 24.2.3 “Byte-Oriented and
Bit-Oriented Instructions in Indexed
Literal Offset Mode” for details.
Words: 1
Cycles: 1
Q Cycle Activity:
Q1 Q2 Q3 Q4
Decode Read
register ‘f’
Process
Data
Write
register ‘f’
Example: CLRF FLAG_REG, 1
Before Instruction
FLAG_REG = 5Ah
After Instruction
FLAG_REG = 00h
CLRWDT Clear Watchdog Timer
Syntax: CLRWDT
Operands: None
Operation: 000h → WDT,
000h → WDT postscaler,
1 → TO,
1 → PD
Status Affected: TO, PD
Encoding: 0000 0000 0000 0100
Description: CLRWDT instruction resets the
Watchdog Timer. It also resets the
postscaler of the WDT. Status bits, TO
and PD, are set.
Words: 1
Cycles: 1
Q Cycle Activity:
Q1 Q2 Q3 Q4
Decode No
operation
Process
Data
No
operation
Example: CLRWDT
Before Instruction
WDT Counter = ?
After Instruction
WDT Counter = 00h
WDT Postscaler = 0
TO = 1
PD = 1
PIC18F2420/2520/4420/4520
DS39631B-page 284 Preliminary © 2007 Microchip Technology Inc.
COMF Complement f
Syntax: COMF f {,d {,a}}
Operands: 0 ≤ f ≤ 255
d ∈ [0,1]
a ∈ [0,1]
Operation: (f) → dest
Status Affected: N, Z
Encoding: 0001 11da ffff ffff
Description: The contents of register ‘f’ are
complemented. If ‘d’ is ‘0’, the result is
stored in W. If ‘d’ is ‘1’, the result is
stored back in register ‘f’ (default).
If ‘a’ is ‘0’, the Access Bank is selected.
If ‘a’ is ‘1’, the BSR is used to select the
GPR bank (default).
If ‘a’ is ‘0’ and the extended instruction
set is enabled, this instruction operates
in Indexed Literal Offset Addressing
mode whenever f ≤ 95 (5Fh). See
Section 24.2.3 “Byte-Oriented and
Bit-Oriented Instructions in Indexed
Literal Offset Mode” for details.
Words: 1
Cycles: 1
Q Cycle Activity:
Q1 Q2 Q3 Q4
Decode Read
register ‘f’
Process
Data
Write to
destination
Example: COMF REG, 0, 0
Before Instruction
REG = 13h
After Instruction
REG = 13h
W = ECh
CPFSEQ Compare f with W, skip if f = W
Syntax: CPFSEQ f {,a}
Operands: 0 ≤ f ≤ 255
a ∈ [0,1]
Operation: (f) – (W),
skip if (f) = (W)
(unsigned comparison)
Status Affected: None
Encoding: 0110 001a ffff ffff
Description: Compares the contents of data memory
location ‘f’ to the contents of W by
performing an unsigned subtraction.
If ‘f’ = W, then the fetched instruction is
discarded and a NOP is executed
instead, making this a two-cycle
instruction.
If ‘a’ is ‘0’, the Access Bank is selected.
If ‘a’ is ‘1’, the BSR is used to select the
GPR bank (default).
If ‘a’ is ‘0’ and the extended instruction
set is enabled, this instruction operates
in Indexed Literal Offset Addressing
mode whenever f ≤ 95 (5Fh). See
Section 24.2.3 “Byte-Oriented and
Bit-Oriented Instructions in Indexed
Literal Offset Mode” for details.
Words: 1
Cycles: 1(2)
Note: 3 cycles if skip and followed
by a 2-word instruction.
Q Cycle Activity:
Q1 Q2 Q3 Q4
Decode Read
register ‘f’
Process
Data
No
operation
If skip:
Q1 Q2 Q3 Q4
No
operation
No
operation
No
operation
No
operation
If skip and followed by 2-word instruction:
Q1 Q2 Q3 Q4
No
operation
No
operation
No
operation
No
operation
No
operation
No
operation
No
operation
No
operation
Example: HERE CPFSEQ REG, 0
NEQUAL :
EQUAL :
Before Instruction
PC Address = HERE
W = ?
REG = ?
After Instruction
If REG = W;
PC = Address (EQUAL)
If REG ≠ W;
PC = Address (NEQUAL)
© 2007 Microchip Technology Inc. Preliminary DS39631B-page 285
PIC18F2420/2520/4420/4520
CPFSGT Compare f with W, skip if f > W
Syntax: CPFSGT f {,a}
Operands: 0 ≤ f ≤ 255
a ∈ [0,1]
Operation: (f) – (W),
skip if (f) > (W)
(unsigned comparison)
Status Affected: None
Encoding: 0110 010a ffff ffff
Description: Compares the contents of data memory
location ‘f’ to the contents of the W by
performing an unsigned subtraction.
If the contents of ‘f’ are greater than the
contents of WREG, then the fetched
instruction is discarded and a NOP is
executed instead, making this a
two-cycle instruction.
If ‘a’ is ‘0’, the Access Bank is selected.
If ‘a’ is ‘1’, the BSR is used to select the
GPR bank (default).
If ‘a’ is ‘0’ and the extended instruction
set is enabled, this instruction operates
in Indexed Literal Offset Addressing
mode whenever f ≤ 95 (5Fh). See
Section 24.2.3 “Byte-Oriented and
Bit-Oriented Instructions in Indexed
Literal Offset Mode” for details.
Words: 1
Cycles: 1(2)
Note: 3 cycles if skip and followed
by a 2-word instruction.
Q Cycle Activity:
Q1 Q2 Q3 Q4
Decode Read
register ‘f’
Process
Data
No
operation
If skip:
Q1 Q2 Q3 Q4
No
operation
No
operation
No
operation
No
operation
If skip and followed by 2-word instruction:
Q1 Q2 Q3 Q4
No
operation
No
operation
No
operation
No
operation
No
operation
No
operation
No
operation
No
operation
Example: HERE CPFSGT REG, 0
NGREATER :
GREATER :
Before Instruction
PC = Address (HERE)
W = ?
After Instruction
If REG > W;
PC = Address (GREATER)
If REG ≤ W;
PC = Address (NGREATER)
CPFSLT Compare f with W, skip if f < W
Syntax: CPFSLT f {,a}
Operands: 0 ≤ f ≤ 255
a ∈ [0,1]
Operation: (f) – (W),
skip if (f) < (W)
(unsigned comparison)
Status Affected: None
Encoding: 0110 000a ffff ffff
Description: Compares the contents of data memory
location ‘f’ to the contents of W by
performing an unsigned subtraction.
If the contents of ‘f’ are less than the
contents of W, then the fetched
instruction is discarded and a NOP is
executed instead, making this a
two-cycle instruction.
If ‘a’ is ‘0’, the Access Bank is selected.
If ‘a’ is ‘1’, the BSR is used to select the
GPR bank (default).
Words: 1
Cycles: 1(2)
Note: 3 cycles if skip and followed
by a 2-word instruction.
Q Cycle Activity:
Q1 Q2 Q3 Q4
Decode Read
register ‘f’
Process
Data
No
operation
If skip:
Q1 Q2 Q3 Q4
No
operation
No
operation
No
operation
No
operation
If skip and followed by 2-word instruction:
Q1 Q2 Q3 Q4
No
operation
No
operation
No
operation
No
operation
No
operation
No
operation
No
operation
No
operation
Example: HERE CPFSLT REG, 1
NLESS :
LESS :
Before Instruction
PC = Address (HERE)
W = ?
After Instruction
If REG < W;
PC = Address (LESS)
If REG ≥ W;
PC = Address (NLESS)
PIC18F2420/2520/4420/4520
DS39631B-page 286 Preliminary © 2007 Microchip Technology Inc.
DAW Decimal Adjust W Register
Syntax: DAW
Operands: None
Operation: If [W<3:0> > 9] or [DC = 1] then
(W<3:0>) + 6 → W<3:0>;
else
(W<3:0>) → W<3:0>;
If [W<7:4> + DC > 9] or [C = 1] then
(W<7:4>) + 6 + DC → W<7:4> ;
else
(W<7:4>) + DC → W<7:4>
Status Affected: C
Encoding: 0000 0000 0000 0111
Description: DAW adjusts the eight-bit value in W,
resulting from the earlier addition of two
variables (each in packed BCD format)
and produces a correct packed BCD
result.
Words: 1
Cycles: 1
Q Cycle Activity:
Q1 Q2 Q3 Q4
Decode Read
register W
Process
Data
Write
W
Example1:
DAW
Before Instruction
W = A5h
C = 0
DC = 0
After Instruction
W = 05h
C = 1
DC = 0
Example 2:
Before Instruction
W = CEh
C = 0
DC = 0
After Instruction
W = 34h
C = 1
DC = 0
DECF Decrement f
Syntax: DECF f {,d {,a}}
Operands: 0 ≤ f ≤ 255
d ∈ [0,1]
a ∈ [0,1]
Operation: (f) – 1 → dest
Status Affected: C, DC, N, OV, Z
Encoding: 0000 01da ffff ffff
Description: Decrement register ‘f’. If ‘d’ is ‘0’, the
result is stored in W. If ‘d’ is ‘1’, the
result is stored back in register ‘f’
(default).
If ‘a’ is ‘0’, the Access Bank is selected.
If ‘a’ is ‘1’, the BSR is used to select the
GPR bank (default).
If ‘a’ is ‘0’ and the extended instruction
set is enabled, this instruction operates
in Indexed Literal Offset Addressing
mode whenever f ≤ 95 (5Fh). See
Section 24.2.3 “Byte-Oriented and
Bit-Oriented Instructions in Indexed
Literal Offset Mode” for details.
Words: 1
Cycles: 1
Q Cycle Activity:
Q1 Q2 Q3 Q4
Decode Read
register ‘f’
Process
Data
Write to
destination
Example: DECF CNT, 1, 0
Before Instruction
CNT = 01h
Z = 0
After Instruction
CNT = 00h
Z = 1
© 2007 Microchip Technology Inc. Preliminary DS39631B-page 287
PIC18F2420/2520/4420/4520
DECFSZ Decrement f, skip if 0
Syntax: DECFSZ f {,d {,a}}
Operands: 0 ≤ f ≤ 255
d ∈ [0,1]
a ∈ [0,1]
Operation: (f) – 1 → dest,
skip if result = 0
Status Affected: None
Encoding: 0010 11da ffff ffff
Description: The contents of register ‘f’ are
decremented. If ‘d’ is ‘0’, the result is
placed in W. If ‘d’ is ‘1’, the result is
placed back in register ‘f’ (default).
If the result is ‘0’, the next instruction,
which is already fetched, is discarded
and a NOP is executed instead, making
it a two-cycle instruction.
If ‘a’ is ‘0’, the Access Bank is selected.
If ‘a’ is ‘1’, the BSR is used to select the
GPR bank (default).
If ‘a’ is ‘0’ and the extended instruction
set is enabled, this instruction operates
in Indexed Literal Offset Addressing
mode whenever f ≤ 95 (5Fh). See
Section 24.2.3 “Byte-Oriented and
Bit-Oriented Instructions in Indexed
Literal Offset Mode” for details.
Words: 1
Cycles: 1(2)
Note: 3 cycles if skip and followed
by a 2-word instruction.
Q Cycle Activity:
Q1 Q2 Q3 Q4
Decode Read
register ‘f’
Process
Data
Write to
destination
If skip:
Q1 Q2 Q3 Q4
No
operation
No
operation
No
operation
No
operation
If skip and followed by 2-word instruction:
Q1 Q2 Q3 Q4
No
operation
No
operation
No
operation
No
operation
No
operation
No
operation
No
operation
No
operation
Example: HERE DECFSZ CNT, 1, 1
GOTO LOOP
CONTINUE
Before Instruction
PC = Address (HERE)
After Instruction
CNT = CNT - 1
If CNT = 0;
PC = Address (CONTINUE)
If CNT ≠ 0;
PC = Address (HERE + 2)
DCFSNZ Decrement f, skip if not 0
Syntax: DCFSNZ f {,d {,a}}
Operands: 0 ≤ f ≤ 255
d ∈ [0,1]
a ∈ [0,1]
Operation: (f) – 1 → dest,
skip if result ≠ 0
Status Affected: None
Encoding: 0100 11da ffff ffff
Description: The contents of register ‘f’ are
decremented. If ‘d’ is ‘0’, the result is
placed in W. If ‘d’ is ‘1’, the result is
placed back in register ‘f’ (default).
If the result is not ‘0’, the next
instruction, which is already fetched, is
discarded and a NOP is executed
instead, making it a two-cycle
instruction.
If ‘a’ is ‘0’, the Access Bank is selected.
If ‘a’ is ‘1’, the BSR is used to select the
GPR bank (default).
If ‘a’ is ‘0’ and the extended instruction
set is enabled, this instruction operates
in Indexed Literal Offset Addressing
mode whenever f ≤ 95 (5Fh). See
Section 24.2.3 “Byte-Oriented and
Bit-Oriented Instructions in Indexed
Literal Offset Mode” for details.
Words: 1
Cycles: 1(2)
Note: 3 cycles if skip and followed
by a 2-word instruction.
Q Cycle Activity:
Q1 Q2 Q3 Q4
Decode Read
register ‘f’
Process
Data
Write to
destination
If skip:
Q1 Q2 Q3 Q4
No
operation
No
operation
No
operation
No
operation
If skip and followed by 2-word instruction:
Q1 Q2 Q3 Q4
No
operation
No
operation
No
operation
No
operation
No
operation
No
operation
No
operation
No
operation
Example: HERE DCFSNZ TEMP, 1, 0
ZERO :
NZERO :
Before Instruction
TEMP = ?
After Instruction
TEMP = TEMP – 1,
If TEMP = 0;
PC = Address (ZERO)
If TEMP ≠ 0;
PC = Address (NZERO)
PIC18F2420/2520/4420/4520
DS39631B-page 288 Preliminary © 2007 Microchip Technology Inc.
GOTO Unconditional Branch
Syntax: GOTO k
Operands: 0 ≤ k ≤ 1048575
Operation: k → PC<20:1>
Status Affected: None
Encoding:
1st word (k<7:0>)
2nd word(k<19:8>)
1110
1111
1111
k19kkk
k7kkk
kkkk
kkkk0
kkkk8
Description: GOTO allows an unconditional branch
anywhere within entire
2-Mbyte memory range. The 20-bit
value ‘k’ is loaded into PC<20:1>.
GOTO is always a two-cycle
instruction.
Words: 2
Cycles: 2
Q Cycle Activity:
Q1 Q2 Q3 Q4
Decode Read literal
‘k’<7:0>,
No
operation
Read literal
‘k’<19:8>,
Write to PC
No
operation
No
operation
No
operation
No
operation
Example: GOTO THERE
After Instruction
PC = Address (THERE)
INCF Increment f
Syntax: INCF f {,d {,a}}
Operands: 0 ≤ f ≤ 255
d ∈ [0,1]
a ∈ [0,1]
Operation: (f) + 1 → dest
Status Affected: C, DC, N, OV, Z
Encoding: 0010 10da ffff ffff
Description: The contents of register ‘f’ are
incremented. If ‘d’ is ‘0’, the result is
placed in W. If ‘d’ is ‘1’, the result is
placed back in register ‘f’ (default).
If ‘a’ is ‘0’, the Access Bank is selected.
If ‘a’ is ‘1’, the BSR is used to select the
GPR bank (default).
If ‘a’ is ‘0’ and the extended instruction
set is enabled, this instruction operates
in Indexed Literal Offset Addressing
mode whenever f ≤ 95 (5Fh). See
Section 24.2.3 “Byte-Oriented and
Bit-Oriented Instructions in Indexed
Literal Offset Mode” for details.
Words: 1
Cycles: 1
Q Cycle Activity:
Q1 Q2 Q3 Q4
Decode Read
register ‘f’
Process
Data
Write to
destination
Example: INCF CNT, 1, 0
Before Instruction
CNT = FFh
Z = 0
C = ?
DC = ?
After Instruction
CNT = 00h
Z = 1
C = 1
DC = 1
© 2007 Microchip Technology Inc. Preliminary DS39631B-page 289
PIC18F2420/2520/4420/4520
INCFSZ Increment f, skip if 0
Syntax: INCFSZ f {,d {,a}}
Operands: 0 ≤ f ≤ 255
d ∈ [0,1]
a ∈ [0,1]
Operation: (f) + 1 → dest,
skip if result = 0
Status Affected: None
Encoding: 0011 11da ffff ffff
Description: The contents of register ‘f’ are
incremented. If ‘d’ is ‘0’, the result is
placed in W. If ‘d’ is ‘1’, the result is
placed back in register ‘f’ (default).
If the result is ‘0’, the next instruction,
which is already fetched, is discarded
and a NOP is executed instead, making
it a two-cycle instruction.
If ‘a’ is ‘0’, the Access Bank is selected.
If ‘a’ is ‘1’, the BSR is used to select the
GPR bank (default).
If ‘a’ is ‘0’ and the extended instruction
set is enabled, this instruction operates
in Indexed Literal Offset Addressing
mode whenever f ≤ 95 (5Fh). See
Section 24.2.3 “Byte-Oriented and
Bit-Oriented Instructions in Indexed
Literal Offset Mode” for details.
Words: 1
Cycles: 1(2)
Note: 3 cycles if skip and followed
by a 2-word instruction.
Q Cycle Activity:
Q1 Q2 Q3 Q4
Decode Read
register ‘f’
Process
Data
Write to
destination
If skip:
Q1 Q2 Q3 Q4
No
operation
No
operation
No
operation
No
operation
If skip and followed by 2-word instruction:
Q1 Q2 Q3 Q4
No
operation
No
operation
No
operation
No
operation
No
operation
No
operation
No
operation
No
operation
Example: HERE INCFSZ CNT, 1, 0
NZERO :
ZERO :
Before Instruction
PC = Address (HERE)
After Instruction
CNT = CNT + 1
If CNT = 0;
PC = Address (ZERO)
If CNT ≠ 0;
PC = Address (NZERO)
INFSNZ Increment f, skip if not 0
Syntax: INFSNZ f {,d {,a}}
Operands: 0 ≤ f ≤ 255
d ∈ [0,1]
a ∈ [0,1]
Operation: (f) + 1 → dest,
skip if result ≠ 0
Status Affected: None
Encoding: 0100 10da ffff ffff
Description: The contents of register ‘f’ are
incremented. If ‘d’ is ‘0’, the result is
placed in W. If ‘d’ is ‘1’, the result is
placed back in register ‘f’ (default).
If the result is not ‘0’, the next
instruction, which is already fetched, is
discarded and a NOP is executed
instead, making it a two-cycle
instruction.
If ‘a’ is ‘0’, the Access Bank is selected.
If ‘a’ is ‘1’, the BSR is used to select the
GPR bank (default).
If ‘a’ is ‘0’ and the extended instruction
set is enabled, this instruction operates
in Indexed Literal Offset Addressing
mode whenever f ≤ 95 (5Fh). See
Section 24.2.3 “Byte-Oriented and
Bit-Oriented Instructions in Indexed
Literal Offset Mode” for details.
Words: 1
Cycles: 1(2)
Note: 3 cycles if skip and followed
by a 2-word instruction.
Q Cycle Activity:
Q1 Q2 Q3 Q4
Decode Read
register ‘f’
Process
Data
Write to
destination
If skip:
Q1 Q2 Q3 Q4
No
operation
No
operation
No
operation
No
operation
If skip and followed by 2-word instruction:
Q1 Q2 Q3 Q4
No
operation
No
operation
No
operation
No
operation
No
operation
No
operation
No
operation
No
operation
Example: HERE INFSNZ REG, 1, 0
ZERO
NZERO
Before Instruction
PC = Address (HERE)
After Instruction
REG = REG + 1
If REG ≠ 0;
PC = Address (NZERO)
If REG = 0;
PC = Address (ZERO)
PIC18F2420/2520/4420/4520
DS39631B-page 290 Preliminary © 2007 Microchip Technology Inc.
IORLW Inclusive OR literal with W
Syntax: IORLW k
Operands: 0 ≤ k ≤ 255
Operation: (W) .OR. k → W
Status Affected: N, Z
Encoding: 0000 1001 kkkk kkkk
Description: The contents of W are ORed with the
eight-bit literal ‘k’. The result is placed in
W.
Words: 1
Cycles: 1
Q Cycle Activity:
Q1 Q2 Q3 Q4
Decode Read
literal ‘k’
Process
Data
Write to W
Example: IORLW 35h
Before Instruction
W = 9Ah
After Instruction
W = BFh
IORWF Inclusive OR W with f
Syntax: IORWF f {,d {,a}}
Operands: 0 ≤ f ≤ 255
d ∈ [0,1]
a ∈ [0,1]
Operation: (W) .OR. (f) → dest
Status Affected: N, Z
Encoding: 0001 00da ffff ffff
Description: Inclusive OR W with register ‘f’. If ‘d’ is
‘0’, the result is placed in W. If ‘d’ is ‘1’,
the result is placed back in register ‘f’
(default).
If ‘a’ is ‘0’, the Access Bank is selected.
If ‘a’ is ‘1’, the BSR is used to select the
GPR bank (default).
If ‘a’ is ‘0’ and the extended instruction
set is enabled, this instruction operates
in Indexed Literal Offset Addressing
mode whenever f ≤ 95 (5Fh). See
Section 24.2.3 “Byte-Oriented and
Bit-Oriented Instructions in Indexed
Literal Offset Mode” for details.
Words: 1
Cycles: 1
Q Cycle Activity:
Q1 Q2 Q3 Q4
Decode Read
register ‘f’
Process
Data
Write to
destination
Example: IORWF RESULT, 0, 1
Before Instruction
RESULT = 13h
W = 91h
After Instruction
RESULT = 13h
W = 93h
© 2007 Microchip Technology Inc. Preliminary DS39631B-page 291
PIC18F2420/2520/4420/4520
LFSR Load FSR
Syntax: LFSR f, k
Operands: 0 ≤ f ≤ 2
0 ≤ k ≤ 4095
Operation: k → FSRf
Status Affected: None
Encoding: 1110
1111
1110
0000
00ff
k7kkk
k11kkk
kkkk
Description: The 12-bit literal ‘k’ is loaded into the
File Select Register pointed to by ‘f’.
Words: 2
Cycles: 2
Q Cycle Activity:
Q1 Q2 Q3 Q4
Decode Read literal
‘k’ MSB
Process
Data
Write
literal ‘k’
MSB to
FSRfH
Decode Read literal
‘k’ LSB
Process
Data
Write literal
‘k’ to FSRfL
Example: LFSR 2, 3ABh
After Instruction
FSR2H = 03h
FSR2L = ABh
MOVF Move f
Syntax: MOVF f {,d {,a}}
Operands: 0 ≤ f ≤ 255
d ∈ [0,1]
a ∈ [0,1]
Operation: f → dest
Status Affected: N, Z
Encoding: 0101 00da ffff ffff
Description: The contents of register ‘f’ are moved to
a destination dependent upon the
status of ‘d’. If ‘d’ is ‘0’, the result is
placed in W. If ‘d’ is ‘1’, the result is
placed back in register ‘f’ (default).
Location ‘f’ can be anywhere in the
256-byte bank.
If ‘a’ is ‘0’, the Access Bank is selected.
If ‘a’ is ‘1’, the BSR is used to select the
GPR bank (default).
If ‘a’ is ‘0’ and the extended instruction
set is enabled, this instruction operates
in Indexed Literal Offset Addressing
mode whenever f ≤ 95 (5Fh). See
Section 24.2.3 “Byte-Oriented and
Bit-Oriented Instructions in Indexed
Literal Offset Mode” for details.
Words: 1
Cycles: 1
Q Cycle Activity:
Q1 Q2 Q3 Q4
Decode Read
register ‘f’
Process
Data
Write W
Example: MOVF REG, 0, 0
Before Instruction
REG = 22h
W = FFh
After Instruction
REG = 22h
W = 22h
PIC18F2420/2520/4420/4520
DS39631B-page 292 Preliminary © 2007 Microchip Technology Inc.
MOVFF Move f to f
Syntax: MOVFF fs,fd
Operands: 0 ≤ fs ≤ 4095
0 ≤ fd ≤ 4095
Operation: (fs) → fd
Status Affected: None
Encoding:
1st word (source)
2nd word (destin.)
1100
1111
ffff
ffff
ffff
ffff
ffffs
ffffd
Description: The contents of source register ‘fs’ are
moved to destination register ‘fd’.
Location of source ‘fs’ can be anywhere
in the 4096-byte data space (000h to
FFFh) and location of destination ‘fd’
can also be anywhere from 000h to
FFFh.
Either source or destination can be W
(a useful special situation).
MOVFF is particularly useful for
transferring a data memory location to a
peripheral register (such as the transmit
buffer or an I/O port).
The MOVFF instruction cannot use the
PCL, TOSU, TOSH or TOSL as the
destination register.
Words: 2
Cycles: 2 (3)
Q Cycle Activity:
Q1 Q2 Q3 Q4
Decode Read
register ‘f’
(src)
Process
Data
No
operation
Decode No
operation
No dummy
read
No
operation
Write
register ‘f’
(dest)
Example: MOVFF REG1, REG2
Before Instruction
REG1 = 33h
REG2 = 11h
After Instruction
REG1 = 33h
REG2 = 33h
MOVLB Move literal to low nibble in BSR
Syntax: MOVLW k
Operands: 0 ≤ k ≤ 255
Operation: k → BSR
Status Affected: None
Encoding: 0000 0001 kkkk kkkk
Description: The eight-bit literal ‘k’ is loaded into the
Bank Select Register (BSR). The value
of BSR<7:4> always remains ‘0’,
regardless of the value of k7:k4.
Words: 1
Cycles: 1
Q Cycle Activity:
Q1 Q2 Q3 Q4
Decode Read
literal ‘k’
Process
Data
Write literal
‘k’ to BSR
Example: MOVLB 5
Before Instruction
BSR Register = 02h
After Instruction
BSR Register = 05h
© 2007 Microchip Technology Inc. Preliminary DS39631B-page 293
PIC18F2420/2520/4420/4520
MOVLW Move literal to W
Syntax: MOVLW k
Operands: 0 ≤ k ≤ 255
Operation: k → W
Status Affected: None
Encoding: 0000 1110 kkkk kkkk
Description: The eight-bit literal ‘k’ is loaded into W.
Words: 1
Cycles: 1
Q Cycle Activity:
Q1 Q2 Q3 Q4
Decode Read
literal ‘k’
Process
Data
Write to W
Example: MOVLW 5Ah
After Instruction
W = 5Ah
MOVWF Move W to f
Syntax: MOVWF f {,a}
Operands: 0 ≤ f ≤ 255
a ∈ [0,1]
Operation: (W) → f
Status Affected: None
Encoding: 0110 111a ffff ffff
Description: Move data from W to register ‘f’.
Location ‘f’ can be anywhere in the
256-byte bank.
If ‘a’ is ‘0’, the Access Bank is selected.
If ‘a’ is ‘1’, the BSR is used to select the
GPR bank (default).
If ‘a’ is ‘0’ and the extended instruction
set is enabled, this instruction operates
in Indexed Literal Offset Addressing
mode whenever f ≤ 95 (5Fh). See
Section 24.2.3 “Byte-Oriented and
Bit-Oriented Instructions in Indexed
Literal Offset Mode” for details.
Words: 1
Cycles: 1
Q Cycle Activity:
Q1 Q2 Q3 Q4
Decode Read
register ‘f’
Process
Data
Write
register ‘f’
Example: MOVWF REG, 0
Before Instruction
W = 4Fh
REG = FFh
After Instruction
W = 4Fh
REG = 4Fh
PIC18F2420/2520/4420/4520
DS39631B-page 294 Preliminary © 2007 Microchip Technology Inc.
MULLW Multiply literal with W
Syntax: MULLW k
Operands: 0 ≤ k ≤ 255
Operation: (W) x k → PRODH:PRODL
Status Affected: None
Encoding: 0000 1101 kkkk kkkk
Description: An unsigned multiplication is carried
out between the contents of W and the
8-bit literal ‘k’. The 16-bit result is
placed in the PRODH:PRODL register
pair. PRODH contains the high byte.
W is unchanged.
None of the Status flags are affected.
Note that neither overflow nor carry is
possible in this operation. A zero result
is possible but not detected.
Words: 1
Cycles: 1
Q Cycle Activity:
Q1 Q2 Q3 Q4
Decode Read
literal ‘k’
Process
Data
Write
registers
PRODH:
PRODL
Example: MULLW 0C4h
Before Instruction
W = E2h
PRODH = ?
PRODL = ?
After Instruction
W = E2h
PRODH = ADh
PRODL = 08h
MULWF Multiply W with f
Syntax: MULWF f {,a}
Operands: 0 ≤ f ≤ 255
a ∈ [0,1]
Operation: (W) x (f) → PRODH:PRODL
Status Affected: None
Encoding: 0000 001a ffff ffff
Description: An unsigned multiplication is carried
out between the contents of W and the
register file location ‘f’. The 16-bit
result is stored in the PRODH:PRODL
register pair. PRODH contains the
high byte. Both W and ‘f’ are
unchanged.
None of the Status flags are affected.
Note that neither overflow nor carry is
possible in this operation. A zero
result is possible but not detected.
If ‘a’ is ‘0’, the Access Bank is
selected. If ‘a’ is ‘1’, the BSR is used
to select the GPR bank (default).
If ‘a’ is ‘0’ and the extended instruction
set is enabled, this instruction
operates in Indexed Literal Offset
Addressing mode whenever
f ≤ 95 (5Fh). See Section 24.2.3
“Byte-Oriented and Bit-Oriented
Instructions in Indexed Literal Offset
Mode” for details.
Words: 1
Cycles: 1
Q Cycle Activity:
Q1 Q2 Q3 Q4
Decode Read
register ‘f’
Process
Data
Write
registers
PRODH:
PRODL
Example: MULWF REG, 1
Before Instruction
W = C4h
REG = B5h
PRODH = ?
PRODL = ?
After Instruction
W = C4h
REG = B5h
PRODH = 8Ah
PRODL = 94h
© 2007 Microchip Technology Inc. Preliminary DS39631B-page 295
PIC18F2420/2520/4420/4520
NEGF Negate f
Syntax: NEGF f {,a}
Operands: 0 ≤ f ≤ 255
a ∈ [0,1]
Operation: ( f ) + 1 → f
Status Affected: N, OV, C, DC, Z
Encoding: 0110 110a ffff ffff
Description: Location ‘f’ is negated using two’s
complement. The result is placed in the
data memory location ‘f’.
If ‘a’ is ‘0’, the Access Bank is selected.
If ‘a’ is ‘1’, the BSR is used to select the
GPR bank (default).
If ‘a’ is ‘0’ and the extended instruction
set is enabled, this instruction operates
in Indexed Literal Offset Addressing
mode whenever f ≤ 95 (5Fh). See
Section 24.2.3 “Byte-Oriented and
Bit-Oriented Instructions in Indexed
Literal Offset Mode” for details.
Words: 1
Cycles: 1
Q Cycle Activity:
Q1 Q2 Q3 Q4
Decode Read
register ‘f’
Process
Data
Write
register ‘f’
Example: NEGF REG, 1
Before Instruction
REG = 0011 1010 [3Ah]
After Instruction
REG = 1100 0110 [C6h]
NOP No Operation
Syntax: NOP
Operands: None
Operation: No operation
Status Affected: None
Encoding: 0000
1111
0000
xxxx
0000
xxxx
0000
xxxx
Description: No operation.
Words: 1
Cycles: 1
Q Cycle Activity:
Q1 Q2 Q3 Q4
Decode No
operation
No
operation
No
operation
Example:
None.
PIC18F2420/2520/4420/4520
DS39631B-page 296 Preliminary © 2007 Microchip Technology Inc.
POP Pop Top of Return Stack
Syntax: POP
Operands: None
Operation: (TOS) → bit bucket
Status Affected: None
Encoding: 0000 0000 0000 0110
Description: The TOS value is pulled off the return
stack and is discarded. The TOS value
then becomes the previous value that
was pushed onto the return stack.
This instruction is provided to enable
the user to properly manage the return
stack to incorporate a software stack.
Words: 1
Cycles: 1
Q Cycle Activity:
Q1 Q2 Q3 Q4
Decode No
operation
POP TOS
value
No
operation
Example: POP
GOTO NEW
Before Instruction
TOS = 0031A2h
Stack (1 level down) = 014332h
After Instruction
TOS = 014332h
PC = NEW
PUSH Push Top of Return Stack
Syntax: PUSH
Operands: None
Operation: (PC + 2) → TOS
Status Affected: None
Encoding: 0000 0000 0000 0101
Description: The PC + 2 is pushed onto the top of
the return stack. The previous TOS
value is pushed down on the stack.
This instruction allows implementing a
software stack by modifying TOS and
then pushing it onto the return stack.
Words: 1
Cycles: 1
Q Cycle Activity:
Q1 Q2 Q3 Q4
Decode PUSH
PC + 2 onto
return stack
No
operation
No
operation
Example: PUSH
Before Instruction
TOS = 345Ah
PC = 0124h
After Instruction
PC = 0126h
TOS = 0126h
Stack (1 level down) = 345Ah
© 2007 Microchip Technology Inc. Preliminary DS39631B-page 297
PIC18F2420/2520/4420/4520
RCALL Relative Call
Syntax: RCALL n
Operands: -1024 ≤ n ≤ 1023
Operation: (PC) + 2 → TOS,
(PC) + 2 + 2n → PC
Status Affected: None
Encoding: 1101 1nnn nnnn nnnn
Description: Subroutine call with a jump up to 1K
from the current location. First, return
address (PC + 2) is pushed onto the
stack. Then, add the 2’s complement
number ‘2n’ to the PC. Since the PC will
have incremented to fetch the next
instruction, the new address will be
PC + 2 + 2n. This instruction is a
two-cycle instruction.
Words: 1
Cycles: 2
Q Cycle Activity:
Q1 Q2 Q3 Q4
Decode Read literal
‘n’
PUSH PC to
stack
Process
Data
Write to PC
No
operation
No
operation
No
operation
No
operation
Example: HERE RCALL Jump
Before Instruction
PC = Address (HERE)
After Instruction
PC = Address (Jump)
TOS = Address (HERE + 2)
RESET Reset
Syntax: RESET
Operands: None
Operation: Reset all registers and flags that are
affected by a MCLR Reset.
Status Affected: All
Encoding: 0000 0000 1111 1111
Description: This instruction provides a way to
execute a MCLR Reset in software.
Words: 1
Cycles: 1
Q Cycle Activity:
Q1 Q2 Q3 Q4
Decode Start
Reset
No
operation
No
operation
Example: RESET
After Instruction
Registers = Reset Value
Flags* = Reset Value
PIC18F2420/2520/4420/4520
DS39631B-page 298 Preliminary © 2007 Microchip Technology Inc.
RETFIE Return from Interrupt
Syntax: RETFIE {s}
Operands: s ∈ [0,1]
Operation: (TOS) → PC,
1 → GIE/GIEH or PEIE/GIEL,
if s = 1
(WS) → W,
(STATUSS) → Status,
(BSRS) → BSR,
PCLATU, PCLATH are unchanged.
Status Affected: GIE/GIEH, PEIE/GIEL.
Encoding: 0000 0000 0001 000s
Description: Return from interrupt. Stack is popped
and Top-of-Stack (TOS) is loaded into
the PC. Interrupts are enabled by
setting either the high or low priority
global interrupt enable bit. If ‘s’ = 1, the
contents of the shadow registers, WS,
STATUSS and BSRS, are loaded into
their corresponding registers, W,
Status and BSR. If ‘s’ = 0, no update of
these registers occurs (default).
Words: 1
Cycles: 2
Q Cycle Activity:
Q1 Q2 Q3 Q4
Decode No
operation
No
operation
POP PC
from stack
Set GIEH or
GIEL
No
operation
No
operation
No
operation
No
operation
Example: RETFIE 1
After Interrupt
PC = TOS
W = WS
BSR = BSRS
Status = STATUSS
GIE/GIEH, PEIE/GIEL = 1
RETLW Return literal to W
Syntax: RETLW k
Operands: 0 ≤ k ≤ 255
Operation: k → W,
(TOS) → PC,
PCLATU, PCLATH are unchanged
Status Affected: None
Encoding: 0000 1100 kkkk kkkk
Description: W is loaded with the eight-bit literal ‘k’.
The program counter is loaded from the
top of the stack (the return address).
The high address latch (PCLATH)
remains unchanged.
Words: 1
Cycles: 2
Q Cycle Activity:
Q1 Q2 Q3 Q4
Decode Read
literal ‘k’
Process
Data
POP PC
from stack,
Write to W
No
operation
No
operation
No
operation
No
operation
Example:
CALL TABLE ; W contains table
; offset value
; W now has
; table value
:
TABLE
ADDWF PCL ; W = offset
RETLW k0 ; Begin table
RETLW k1 ;
:
:
RETLW kn ; End of table
Before Instruction
W = 07h
After Instruction
W = value of kn
© 2007 Microchip Technology Inc. Preliminary DS39631B-page 299
PIC18F2420/2520/4420/4520
RETURN Return from Subroutine
Syntax: RETURN {s}
Operands: s ∈ [0,1]
Operation: (TOS) → PC,
if s = 1
(WS) → W,
(STATUSS) → Status,
(BSRS) → BSR,
PCLATU, PCLATH are unchanged
Status Affected: None
Encoding: 0000 0000 0001 001s
Description: Return from subroutine. The stack is
popped and the top of the stack (TOS)
is loaded into the program counter. If
‘s’= 1, the contents of the shadow
registers, WS, STATUSS and BSRS,
are loaded into their corresponding
registers, W, Status and BSR. If
‘s’ = 0, no update of these registers
occurs (default).
Words: 1
Cycles: 2
Q Cycle Activity:
Q1 Q2 Q3 Q4
Decode No
operation
Process
Data
POP PC
from stack
No
operation
No
operation
No
operation
No
operation
Example: RETURN
After Instruction:
PC = TOS
RLCF Rotate Left f through Carry
Syntax: RLCF f {,d {,a}}
Operands: 0 ≤ f ≤ 255
d ∈ [0,1]
a ∈ [0,1]
Operation: (f) → dest,
(f<7>) → C,
(C) → dest<0>
Status Affected: C, N, Z
Encoding: 0011 01da ffff ffff
Description: The contents of register ‘f’ are rotated
one bit to the left through the CARRY
flag. If ‘d’ is ‘0’, the result is placed in
W. If ‘d’ is ‘1’, the result is stored back
in register ‘f’ (default).
If ‘a’ is ‘0’, the Access Bank is
selected. If ‘a’ is ‘1’, the BSR is used to
select the GPR bank (default).
If ‘a’ is ‘0’ and the extended instruction
set is enabled, this instruction
operates in Indexed Literal Offset
Addressing mode whenever
f ≤ 95 (5Fh). See Section 24.2.3
“Byte-Oriented and Bit-Oriented
Instructions in Indexed Literal Offset
Mode” for details.
Words: 1
Cycles: 1
Q Cycle Activity:
Q1 Q2 Q3 Q4
Decode Read
register ‘f’
Process
Data
Write to
destination
Example: RLCF REG, 0, 0
Before Instruction
REG = 1110 0110
C = 0
After Instruction
REG = 1110 0110
W = 1100 1100
C = 1
C register f
PIC18F2420/2520/4420/4520
DS39631B-page 300 Preliminary © 2007 Microchip Technology Inc.
RLNCF Rotate Left f (No Carry)
Syntax: RLNCF f {,d {,a}}
Operands: 0 ≤ f ≤ 255
d ∈ [0,1]
a ∈ [0,1]
Operation: (f) → dest,
(f<7>) → dest<0>
Status Affected: N, Z
Encoding: 0100 01da ffff ffff
Description: The contents of register ‘f’ are rotated
one bit to the left. If ‘d’ is ‘0’, the result
is placed in W. If ‘d’ is ‘1’, the result is
stored back in register ‘f’ (default).
If ‘a’ is ‘0’, the Access Bank is selected.
If ‘a’ is ‘1’, the BSR is used to select the
GPR bank (default).
If ‘a’ is ‘0’ and the extended instruction
set is enabled, this instruction operates
in Indexed Literal Offset Addressing
mode whenever f ≤ 95 (5Fh). See
Section 24.2.3 “Byte-Oriented and
Bit-Oriented Instructions in Indexed
Literal Offset Mode” for details.
Words: 1
Cycles: 1
Q Cycle Activity:
Q1 Q2 Q3 Q4
Decode Read
register ‘f’
Process
Data
Write to
destination
Example: RLNCF REG, 1, 0
Before Instruction
REG = 1010 1011
After Instruction
REG = 0101 0111
register f
RRCF Rotate Right f through Carry
Syntax: RRCF f {,d {,a}}
Operands: 0 ≤ f ≤ 255
d ∈ [0,1]
a ∈ [0,1]
Operation: (f) → dest,
(f<0>) → C,
(C) → dest<7>
Status Affected: C, N, Z
Encoding: 0011 00da ffff ffff
Description: The contents of register ‘f’ are rotated
one bit to the right through the CARRY
flag. If ‘d’ is ‘0’, the result is placed in W.
If ‘d’ is ‘1’, the result is placed back in
register ‘f’ (default).
If ‘a’ is ‘0’, the Access Bank is selected.
If ‘a’ is ‘1’, the BSR is used to select the
GPR bank (default).
If ‘a’ is ‘0’ and the extended instruction
set is enabled, this instruction operates
in Indexed Literal Offset Addressing
mode whenever f ≤ 95 (5Fh). See
Section 24.2.3 “Byte-Oriented and
Bit-Oriented Instructions in Indexed
Literal Offset Mode” for details.
Words: 1
Cycles: 1
Q Cycle Activity:
Q1 Q2 Q3 Q4
Decode Read
register ‘f’
Process
Data
Write to
destination
Example: RRCF REG, 0, 0
Before Instruction
REG = 1110 0110
C = 0
After Instruction
REG = 1110 0110
W = 0111 0011
C = 0
C register f
© 2007 Microchip Technology Inc. Preliminary DS39631B-page 301
PIC18F2420/2520/4420/4520
RRNCF Rotate Right f (No Carry)
Syntax: RRNCF f {,d {,a}}
Operands: 0 ≤ f ≤ 255
d ∈ [0,1]
a ∈ [0,1]
Operation: (f) → dest,
(f<0>) → dest<7>
Status Affected: N, Z
Encoding: 0100 00da ffff ffff
Description: The contents of register ‘f’ are rotated
one bit to the right. If ‘d’ is ‘0’, the result
is placed in W. If ‘d’ is ‘1’, the result is
placed back in register ‘f’ (default).
If ‘a’ is ‘0’, the Access Bank will be
selected, overriding the BSR value. If ‘a’
is ‘1’, then the bank will be selected as
per the BSR value (default).
If ‘a’ is ‘0’ and the extended instruction
set is enabled, this instruction operates
in Indexed Literal Offset Addressing
mode whenever f ≤ 95 (5Fh). See
Section 24.2.3 “Byte-Oriented and
Bit-Oriented Instructions in Indexed
Literal Offset Mode” for details.
Words: 1
Cycles: 1
Q Cycle Activity:
Q1 Q2 Q3 Q4
Decode Read
register ‘f’
Process
Data
Write to
destination
Example 1: RRNCF REG, 1, 0
Before Instruction
REG = 1101 0111
After Instruction
REG = 1110 1011
Example 2: RRNCF REG, 0, 0
Before Instruction
W = ?
REG = 1101 0111
After Instruction
W = 1110 1011
REG = 1101 0111
register f
SETF Set f
Syntax: SETF f {,a}
Operands: 0 ≤ f ≤ 255
a ∈ [0,1]
Operation: FFh → f
Status Affected: None
Encoding: 0110 100a ffff ffff
Description: The contents of the specified register
are set to FFh.
If ‘a’ is ‘0’, the Access Bank is selected.
If ‘a’ is ‘1’, the BSR is used to select the
GPR bank (default).
If ‘a’ is ‘0’ and the extended instruction
set is enabled, this instruction operates
in Indexed Literal Offset Addressing
mode whenever f ≤ 95 (5Fh). See
Section 24.2.3 “Byte-Oriented and
Bit-Oriented Instructions in Indexed
Literal Offset Mode” for details.
Words: 1
Cycles: 1
Q Cycle Activity:
Q1 Q2 Q3 Q4
Decode Read
register ‘f’
Process
Data
Write
register ‘f’
Example: SETF REG, 1
Before Instruction
REG = 5Ah
After Instruction
REG = FFh
PIC18F2420/2520/4420/4520
DS39631B-page 302 Preliminary © 2007 Microchip Technology Inc.
SLEEP Enter Sleep mode
Syntax: SLEEP
Operands: None
Operation: 00h → WDT,
0 → WDT postscaler,
1 → TO,
0 → PD
Status Affected: TO, PD
Encoding: 0000 0000 0000 0011
Description: The Power-down status bit (PD) is
cleared. The Time-out status bit (TO)
is set. Watchdog Timer and its
postscaler are cleared.
The processor is put into Sleep mode
with the oscillator stopped.
Words: 1
Cycles: 1
Q Cycle Activity:
Q1 Q2 Q3 Q4
Decode No
operation
Process
Data
Go to
Sleep
Example: SLEEP
Before Instruction
TO = ?
PD = ?
After Instruction
TO = 1 †
PD = 0
† If WDT causes wake-up, this bit is cleared.
SUBFWB Subtract f from W with borrow
Syntax: SUBFWB f {,d {,a}}
Operands: 0 ≤ f ≤ 255
d ∈ [0,1]
a ∈ [0,1]
Operation: (W) – (f) – (C) → dest
Status Affected: N, OV, C, DC, Z
Encoding: 0101 01da ffff ffff
Description: Subtract register ‘f’ and CARRY flag
(borrow) from W (2’s complement
method). If ‘d’ is ‘0’, the result is stored
in W. If ‘d’ is ‘1’, the result is stored in
register ‘f’ (default).
If ‘a’ is ‘0’, the Access Bank is
selected. If ‘a’ is ‘1’, the BSR is used
to select the GPR bank (default).
If ‘a’ is ‘0’ and the extended instruction
set is enabled, this instruction
operates in Indexed Literal Offset
Addressing mode whenever
f ≤ 95 (5Fh). See Section 24.2.3
“Byte-Oriented and Bit-Oriented
Instructions in Indexed Literal Offset
Mode” for details.
Words: 1
Cycles: 1
Q Cycle Activity:
Q1 Q2 Q3 Q4
Decode Read
register ‘f’
Process
Data
Write to
destination
Example 1: SUBFWB REG, 1, 0
Before Instruction
REG = 3
W = 2
C = 1
After Instruction
REG = FF
W = 2
C = 0
Z = 0
N = 1 ; result is negative
Example 2: SUBFWB REG, 0, 0
Before Instruction
REG = 2
W = 5
C = 1
After Instruction
REG = 2
W = 3
C = 1
Z = 0
N = 0 ; result is positive
Example 3: SUBFWB REG, 1, 0
Before Instruction
REG = 1
W = 2
C = 0
After Instruction
REG = 0
W = 2
C = 1
Z = 1 ; result is zero
N = 0
© 2007 Microchip Technology Inc. Preliminary DS39631B-page 303
PIC18F2420/2520/4420/4520
SUBLW Subtract W from literal
Syntax: SUBLW k
Operands: 0 ≤ k ≤ 255
Operation: k – (W) → W
Status Affected: N, OV, C, DC, Z
Encoding: 0000 1000 kkkk kkkk
Description W is subtracted from the eight-bit
literal ‘k’. The result is placed in W.
Words: 1
Cycles: 1
Q Cycle Activity:
Q1 Q2 Q3 Q4
Decode Read
literal ‘k’
Process
Data
Write to W
Example 1: SUBLW 02h
Before Instruction
W = 01h
C = ?
After Instruction
W = 01h
C = 1 ; result is positive
Z = 0
N = 0
Example 2: SUBLW 02h
Before Instruction
W = 02h
C = ?
After Instruction
W = 00h
C = 1 ; result is zero
Z = 1
N = 0
Example 3: SUBLW 02h
Before Instruction
W = 03h
C = ?
After Instruction
W = FFh ; (2’s complement)
C = 0 ; result is negative
Z = 0
N = 1
SUBWF Subtract W from f
Syntax: SUBWF f {,d {,a}}
Operands: 0 ≤ f ≤ 255
d ∈ [0,1]
a ∈ [0,1]
Operation: (f) – (W) → dest
Status Affected: N, OV, C, DC, Z
Encoding: 0101 11da ffff ffff
Description: Subtract W from register ‘f’ (2’s
complement method). If ‘d’ is ‘0’, the
result is stored in W. If ‘d’ is ‘1’, the
result is stored back in register ‘f’
(default).
If ‘a’ is ‘0’, the Access Bank is
selected. If ‘a’ is ‘1’, the BSR is used
to select the GPR bank (default).
If ‘a’ is ‘0’ and the extended instruction
set is enabled, this instruction
operates in Indexed Literal Offset
Addressing mode whenever
f ≤ 95 (5Fh). See Section 24.2.3
“Byte-Oriented and Bit-Oriented
Instructions in Indexed Literal Offset
Mode” for details.
Words: 1
Cycles: 1
Q Cycle Activity:
Q1 Q2 Q3 Q4
Decode Read
register ‘f’
Process
Data
Write to
destination
Example 1: SUBWF REG, 1, 0
Before Instruction
REG = 3
W = 2
C = ?
After Instruction
REG = 1
W = 2
C = 1 ; result is positive
Z = 0
N = 0
Example 2: SUBWF REG, 0, 0
Before Instruction
REG = 2
W = 2
C = ?
After Instruction
REG = 2
W = 0
C = 1 ; result is zero
Z = 1
N = 0
Example 3: SUBWF REG, 1, 0
Before Instruction
REG = 1
W = 2
C = ?
After Instruction
REG = FFh ;(2’s complement)
W = 2
C = 0 ; result is negative
Z = 0
N = 1
PIC18F2420/2520/4420/4520
DS39631B-page 304 Preliminary © 2007 Microchip Technology Inc.
SUBWFB Subtract W from f with Borrow
Syntax: SUBWFB f {,d {,a}}
Operands: 0 ≤ f ≤ 255
d ∈ [0,1]
a ∈ [0,1]
Operation: (f) – (W) – (C) → dest
Status Affected: N, OV, C, DC, Z
Encoding: 0101 10da ffff ffff
Description: Subtract W and the CARRY flag
(borrow) from register ‘f’ (2’s complement
method). If ‘d’ is ‘0’, the result is
stored in W. If ‘d’ is ‘1’, the result is
stored back in register ‘f’ (default).
If ‘a’ is ‘0’, the Access Bank is selected.
If ‘a’ is ‘1’, the BSR is used to select the
GPR bank (default).
If ‘a’ is ‘0’ and the extended instruction
set is enabled, this instruction operates
in Indexed Literal Offset Addressing
mode whenever f ≤ 95 (5Fh). See
Section 24.2.3 “Byte-Oriented and
Bit-Oriented Instructions in Indexed
Literal Offset Mode” for details.
Words: 1
Cycles: 1
Q Cycle Activity:
Q1 Q2 Q3 Q4
Decode Read
register ‘f’
Process
Data
Write to
destination
Example 1: SUBWFB REG, 1, 0
Before Instruction
REG = 19h (0001 1001)
W = 0Dh (0000 1101)
C = 1
After Instruction
REG = 0Ch (0000 1011)
W = 0Dh (0000 1101)
C = 1
Z = 0
N = 0 ; result is positive
Example 2: SUBWFB REG, 0, 0
Before Instruction
REG = 1Bh (0001 1011)
W = 1Ah (0001 1010)
C = 0
After Instruction
REG = 1Bh (0001 1011)
W = 00h
C = 1
Z = 1 ; result is zero
N = 0
Example 3: SUBWFB REG, 1, 0
Before Instruction
REG = 03h (0000 0011)
W = 0Eh (0000 1101)
C = 1
After Instruction
REG = F5h (1111 0100)
; [2’s comp]
W = 0Eh (0000 1101)
C = 0
Z = 0
N = 1 ; result is negative
SWAPF Swap f
Syntax: SWAPF f {,d {,a}}
Operands: 0 ≤ f ≤ 255
d ∈ [0,1]
a ∈ [0,1]
Operation: (f<3:0>) → dest<7:4>,
(f<7:4>) → dest<3:0>
Status Affected: None
Encoding: 0011 10da ffff ffff
Description: The upper and lower nibbles of register
‘f’ are exchanged. If ‘d’ is ‘0’, the result
is placed in W. If ‘d’ is ‘1’, the result is
placed in register ‘f’ (default).
If ‘a’ is ‘0’, the Access Bank is selected.
If ‘a’ is ‘1’, the BSR is used to select the
GPR bank (default).
If ‘a’ is ‘0’ and the extended instruction
set is enabled, this instruction operates
in Indexed Literal Offset Addressing
mode whenever f ≤ 95 (5Fh). See
Section 24.2.3 “Byte-Oriented and
Bit-Oriented Instructions in Indexed
Literal Offset Mode” for details.
Words: 1
Cycles: 1
Q Cycle Activity:
Q1 Q2 Q3 Q4
Decode Read
register ‘f’
Process
Data
Write to
destination
Example: SWAPF REG, 1, 0
Before Instruction
REG = 53h
After Instruction
REG = 35h
© 2007 Microchip Technology Inc. Preliminary DS39631B-page 305
PIC18F2420/2520/4420/4520
TBLRD Table Read
Syntax: TBLRD ( *; *+; *-; +*)
Operands: None
Operation: if TBLRD *,
(Prog Mem (TBLPTR)) → TABLAT;
TBLPTR – No Change;
if TBLRD *+,
(Prog Mem (TBLPTR)) → TABLAT;
(TBLPTR) + 1 → TBLPTR;
if TBLRD *-,
(Prog Mem (TBLPTR)) → TABLAT;
(TBLPTR) – 1 → TBLPTR;
if TBLRD +*,
(TBLPTR) + 1 → TBLPTR;
(Prog Mem (TBLPTR)) → TABLAT;
Status Affected: None
Encoding: 0000 0000 0000 10nn
nn=0 *
=1 *+
=2 *-
=3 +*
Description: This instruction is used to read the contents
of Program Memory (P.M.). To address the
program memory, a pointer called Table
Pointer (TBLPTR) is used.
The TBLPTR (a 21-bit pointer) points to
each byte in the program memory. TBLPTR
has a 2-Mbyte address range.
TBLPTR[0] = 0: Least Significant Byte
of Program Memory
Word
TBLPTR[0] = 1: Most Significant Byte
of Program Memory
Word
The TBLRD instruction can modify the value
of TBLPTR as follows:
• no change
• post-increment
• post-decrement
• pre-increment
Words: 1
Cycles: 2
Q Cycle Activity:
Q1 Q2 Q3 Q4
Decode No
operation
No
operation
No
operation
No
operation
No operation
(Read Program
Memory)
No
operation
No operation
(Write TABLAT)
TBLRD Table Read (Continued)
Example1: TBLRD *+ ;
Before Instruction
TABLAT = 55h
TBLPTR = 00A356h
MEMORY (00A356h) = 34h
After Instruction
TABLAT = 34h
TBLPTR = 00A357h
Example2: TBLRD +* ;
Before Instruction
TABLAT = AAh
TBLPTR = 01A357h
MEMORY (01A357h) = 12h
MEMORY (01A358h) = 34h
After Instruction
TABLAT = 34h
TBLPTR = 01A358h
PIC18F2420/2520/4420/4520
DS39631B-page 306 Preliminary © 2007 Microchip Technology Inc.
TBLWT Table Write
Syntax: TBLWT ( *; *+; *-; +*)
Operands: None
Operation: if TBLWT*,
(TABLAT) → Holding Register;
TBLPTR – No Change;
if TBLWT*+,
(TABLAT) → Holding Register;
(TBLPTR) + 1 → TBLPTR;
if TBLWT*-,
(TABLAT) → Holding Register;
(TBLPTR) – 1 → TBLPTR;
if TBLWT+*,
(TBLPTR) + 1 → TBLPTR;
(TABLAT) → Holding Register;
Status Affected: None
Encoding: 0000 0000 0000 11nn
nn=0 *
=1 *+
=2 *-
=3 +*
Description: This instruction uses the 3 LSBs of
TBLPTR to determine which of the
8 holding registers the TABLAT is written
to. The holding registers are used to
program the contents of Program
Memory (P.M.). (Refer to Section 6.0
“Flash Program Memory” for additional
details on programming Flash memory.)
The TBLPTR (a 21-bit pointer) points to
each byte in the program memory.
TBLPTR has a 2-MByte address range.
The LSb of the TBLPTR selects which
byte of the program memory location to
access.
TBLPTR[0] = 0: Least Significant
Byte of Program
Memory Word
TBLPTR[0] = 1: Most Significant
Byte of Program
Memory Word
The TBLWT instruction can modify the
value of TBLPTR as follows:
• no change
• post-increment
• post-decrement
• pre-increment
Words: 1
Cycles: 2
Q Cycle Activity:
Q1 Q2 Q3 Q4
Decode No
operation
No
operation
No
operation
No
operation
No
operation
(Read
TABLAT)
No
operation
No
operation
(Write to
Holding
Register )
TBLWT Table Write (Continued)
Example1: TBLWT *+;
Before Instruction
TABLAT = 55h
TBLPTR = 00A356h
HOLDING REGISTER
(00A356h) = FFh
After Instructions (table write completion)
TABLAT = 55h
TBLPTR = 00A357h
HOLDING REGISTER
(00A356h) = 55h
Example 2: TBLWT +*;
Before Instruction
TABLAT = 34h
TBLPTR = 01389Ah
HOLDING REGISTER
(01389Ah) = FFh
HOLDING REGISTER
(01389Bh) = FFh
After Instruction (table write completion)
TABLAT = 34h
TBLPTR = 01389Bh
HOLDING REGISTER
(01389Ah) = FFh
HOLDING REGISTER
(01389Bh) = 34h
© 2007 Microchip Technology Inc. Preliminary DS39631B-page 307
PIC18F2420/2520/4420/4520
TSTFSZ Test f, skip if 0
Syntax: TSTFSZ f {,a}
Operands: 0 ≤ f ≤ 255
a ∈ [0,1]
Operation: skip if f = 0
Status Affected: None
Encoding: 0110 011a ffff ffff
Description: If ‘f’ = 0, the next instruction fetched
during the current instruction execution
is discarded and a NOP is executed,
making this a two-cycle instruction.
If ‘a’ is ‘0’, the Access Bank is selected.
If ‘a’ is ‘1’, the BSR is used to select the
GPR bank (default).
If ‘a’ is ‘0’ and the extended instruction
set is enabled, this instruction operates
in Indexed Literal Offset Addressing
mode whenever f ≤ 95 (5Fh). See
Section 24.2.3 “Byte-Oriented and
Bit-Oriented Instructions in Indexed
Literal Offset Mode” for details.
Words: 1
Cycles: 1(2)
Note: 3 cycles if skip and followed
by a 2-word instruction.
Q Cycle Activity:
Q1 Q2 Q3 Q4
Decode Read
register ‘f’
Process
Data
No
operation
If skip:
Q1 Q2 Q3 Q4
No
operation
No
operation
No
operation
No
operation
If skip and followed by 2-word instruction:
Q1 Q2 Q3 Q4
No
operation
No
operation
No
operation
No
operation
No
operation
No
operation
No
operation
No
operation
Example: HERE TSTFSZ CNT, 1
NZERO :
ZERO :
Before Instruction
PC = Address (HERE)
After Instruction
If CNT = 00h,
PC = Address (ZERO)
If CNT ≠ 00h,
PC = Address (NZERO)
XORLW Exclusive OR literal with W
Syntax: XORLW k
Operands: 0 ≤ k ≤ 255
Operation: (W) .XOR. k → W
Status Affected: N, Z
Encoding: 0000 1010 kkkk kkkk
Description: The contents of W are XORed with
the 8-bit literal ‘k’. The result is placed
in W.
Words: 1
Cycles: 1
Q Cycle Activity:
Q1 Q2 Q3 Q4
Decode Read
literal ‘k’
Process
Data
Write to W
Example: XORLW 0AFh
Before Instruction
W = B5h
After Instruction
W = 1Ah
PIC18F2420/2520/4420/4520
DS39631B-page 308 Preliminary © 2007 Microchip Technology Inc.
XORWF Exclusive OR W with f
Syntax: XORWF f {,d {,a}}
Operands: 0 ≤ f ≤ 255
d ∈ [0,1]
a ∈ [0,1]
Operation: (W) .XOR. (f) → dest
Status Affected: N, Z
Encoding: 0001 10da ffff ffff
Description: Exclusive OR the contents of W with
register ‘f’. If ‘d’ is ‘0’, the result is stored
in W. If ‘d’ is ‘1’, the result is stored back
in the register ‘f’ (default).
If ‘a’ is ‘0’, the Access Bank is selected.
If ‘a’ is ‘1’, the BSR is used to select the
GPR bank (default).
If ‘a’ is ‘0’ and the extended instruction
set is enabled, this instruction operates
in Indexed Literal Offset Addressing
mode whenever f ≤ 95 (5Fh). See
Section 24.2.3 “Byte-Oriented and
Bit-Oriented Instructions in Indexed
Literal Offset Mode” for details.
Words: 1
Cycles: 1
Q Cycle Activity:
Q1 Q2 Q3 Q4
Decode Read
register ‘f’
Process
Data
Write to
destination
Example: XORWF REG, 1, 0
Before Instruction
REG = AFh
W = B5h
After Instruction
REG = 1Ah
W = B5h
© 2007 Microchip Technology Inc. Preliminary DS39631B-page 309
PIC18F2420/2520/4420/4520
24.2 Extended Instruction Set
In addition to the standard 75 instructions of the PIC18
instruction set, PIC18F2420/2520/4420/4520 devices
also provide an optional extension to the core CPU
functionality. The added features include eight additional
instructions that augment indirect and indexed
addressing operations and the implementation of
Indexed Literal Offset Addressing mode for many of the
standard PIC18 instructions.
The additional features of the extended instruction set
are disabled by default. To enable them, users must set
the XINST configuration bit.
The instructions in the extended set can all be
classified as literal operations, which either manipulate
the File Select Registers, or use them for indexed
addressing. Two of the instructions, ADDFSR and
SUBFSR, each have an additional special instantiation
for using FSR2. These versions (ADDULNK and
SUBULNK) allow for automatic return after execution.
The extended instructions are specifically implemented
to optimize re-entrant program code (that is, code that
is recursive or that uses a software stack) written in
high-level languages, particularly C. Among other
things, they allow users working in high-level
languages to perform certain operations on data
structures more efficiently. These include:
• dynamic allocation and deallocation of software
stack space when entering and leaving
subroutines
• function pointer invocation
• software stack pointer manipulation
• manipulation of variables located in a software
stack
A summary of the instructions in the extended instruction
set is provided in Table 24-3. Detailed descriptions
are provided in Section 24.2.2 “Extended Instruction
Set”. The opcode field descriptions in Table 24-1
(page 268) apply to both the standard and extended
PIC18 instruction sets.
24.2.1 EXTENDED INSTRUCTION SYNTAX
Most of the extended instructions use indexed arguments,
using one of the File Select Registers and some
offset to specify a source or destination register. When
an argument for an instruction serves as part of
indexed addressing, it is enclosed in square brackets
(“[ ]”). This is done to indicate that the argument is used
as an index or offset. MPASM™ Assembler will flag an
error if it determines that an index or offset value is not
bracketed.
When the extended instruction set is enabled, brackets
are also used to indicate index arguments in byteoriented
and bit-oriented instructions. This is in addition
to other changes in their syntax. For more details, see
Section 24.2.3.1 “Extended Instruction Syntax with
Standard PIC18 Commands”.
TABLE 24-3: EXTENSIONS TO THE PIC18 INSTRUCTION SET
Note: The instruction set extension and the
Indexed Literal Offset Addressing mode
were designed for optimizing applications
written in C; the user may likely never use
these instructions directly in assembler.
The syntax for these commands is provided
as a reference for users who may be
reviewing code that has been generated
by a compiler.
Note: In the past, square brackets have been
used to denote optional arguments in the
PIC18 and earlier instruction sets. In this
text and going forward, optional
arguments are denoted by braces (“{ }”).
Mnemonic,
Operands
Description Cycles
16-Bit Instruction Word Status
MSb LSb Affected
ADDFSR
ADDULNK
CALLW
MOVSF
MOVSS
PUSHL
SUBFSR
SUBULNK
f, k
k
zs, fd
zs, zd
k
f, k
k
Add literal to FSR
Add literal to FSR2 and return
Call subroutine using WREG
Move zs (source) to 1st word
fd (destination) 2nd word
Move zs (source) to 1st word
zd (destination) 2nd word
Store literal at FSR2,
decrement FSR2
Subtract literal from FSR
Subtract literal from FSR2 and
return
1
2
2
2
2
1
1
2
1110
1110
0000
1110
1111
1110
1111
1110
1110
1110
1000
1000
0000
1011
ffff
1011
xxxx
1010
1001
1001
ffkk
11kk
0001
0zzz
ffff
1zzz
xzzz
kkkk
ffkk
11kk
kkkk
kkkk
0100
zzzz
ffff
zzzz
zzzz
kkkk
kkkk
kkkk
None
None
None
None
None
None
None
None
PIC18F2420/2520/4420/4520
DS39631B-page 310 Preliminary © 2007 Microchip Technology Inc.
24.2.2 EXTENDED INSTRUCTION SET
ADDFSR Add Literal to FSR
Syntax: ADDFSR f, k
Operands: 0 ≤ k ≤ 63
f ∈ [ 0, 1, 2 ]
Operation: FSR(f) + k → FSR(f)
Status Affected: None
Encoding: 1110 1000 ffkk kkkk
Description: The 6-bit literal ‘k’ is added to the
contents of the FSR specified by ‘f’.
Words: 1
Cycles: 1
Q Cycle Activity:
Q1 Q2 Q3 Q4
Decode Read
literal ‘k’
Process
Data
Write to
FSR
Example: ADDFSR 2, 23h
Before Instruction
FSR2 = 03FFh
After Instruction
FSR2 = 0422h
ADDULNK Add Literal to FSR2 and Return
Syntax: ADDULNK k
Operands: 0 ≤ k ≤ 63
Operation: FSR2 + k → FSR2,
(TOS) → PC
Status Affected: None
Encoding: 1110 1000 11kk kkkk
Description: The 6-bit literal ‘k’ is added to the
contents of FSR2. A RETURN is then
executed by loading the PC with the
TOS.
The instruction takes two cycles to
execute; a NOP is performed during
the second cycle.
This may be thought of as a special
case of the ADDFSR instruction,
where f = 3 (binary ‘11’); it operates
only on FSR2.
Words: 1
Cycles: 2
Q Cycle Activity:
Q1 Q2 Q3 Q4
Decode Read
literal ‘k’
Process
Data
Write to
FSR
No
Operation
No
Operation
No
Operation
No
Operation
Example: ADDULNK 23h
Before Instruction
FSR2 = 03FFh
PC = 0100h
After Instruction
FSR2 = 0422h
PC = (TOS)
Note: All PIC18 instructions may take an optional label argument preceding the instruction mnemonic for use in
symbolic addressing. If a label is used, the instruction syntax then becomes: {label} instruction argument(s).
© 2007 Microchip Technology Inc. Preliminary DS39631B-page 311
PIC18F2420/2520/4420/4520
CALLW Subroutine Call Using WREG
Syntax: CALLW
Operands: None
Operation: (PC + 2) → TOS,
(W) → PCL,
(PCLATH) → PCH,
(PCLATU) → PCU
Status Affected: None
Encoding: 0000 0000 0001 0100
Description First, the return address (PC + 2) is
pushed onto the return stack. Next, the
contents of W are written to PCL; the
existing value is discarded. Then, the
contents of PCLATH and PCLATU are
latched into PCH and PCU,
respectively. The second cycle is
executed as a NOP instruction while the
new next instruction is fetched.
Unlike CALL, there is no option to
update W, Status or BSR.
Words: 1
Cycles: 2
Q Cycle Activity:
Q1 Q2 Q3 Q4
Decode Read
WREG
PUSH PC to
stack
No
operation
No
operation
No
operation
No
operation
No
operation
Example: HERE CALLW
Before Instruction
PC = address (HERE)
PCLATH = 10h
PCLATU = 00h
W = 06h
After Instruction
PC = 001006h
TOS = address (HERE + 2)
PCLATH = 10h
PCLATU = 00h
W = 06h
MOVSF Move Indexed to f
Syntax: MOVSF [zs], fd
Operands: 0 ≤ zs ≤ 127
0 ≤ fd ≤ 4095
Operation: ((FSR2) + zs) → fd
Status Affected: None
Encoding:
1st word (source)
2nd word (destin.)
1110
1111
1011
ffff
0zzz
ffff
zzzzs
ffffd
Description: The contents of the source register are
moved to destination register ‘fd’. The
actual address of the source register is
determined by adding the 7-bit literal
offset ‘zs’ in the first word to the value of
FSR2. The address of the destination
register is specified by the 12-bit literal
‘fd’ in the second word. Both addresses
can be anywhere in the 4096-byte data
space (000h to FFFh).
The MOVSF instruction cannot use the
PCL, TOSU, TOSH or TOSL as the
destination register.
If the resultant source address points to
an indirect addressing register, the
value returned will be 00h.
Words: 2
Cycles: 2
Q Cycle Activity:
Q1 Q2 Q3 Q4
Decode Determine
source addr
Determine
source addr
Read
source reg
Decode No
operation
No dummy
read
No
operation
Write
register ‘f’
(dest)
Example: MOVSF [05h], REG2
Before Instruction
FSR2 = 80h
Contents
of 85h = 33h
REG2 = 11h
After Instruction
FSR2 = 80h
Contents
of 85h = 33h
REG2 = 33h
PIC18F2420/2520/4420/4520
DS39631B-page 312 Preliminary © 2007 Microchip Technology Inc.
MOVSS Move Indexed to Indexed
Syntax: MOVSS [zs], [zd]
Operands: 0 ≤ zs ≤ 127
0 ≤ zd ≤ 127
Operation: ((FSR2) + zs) → ((FSR2) + zd)
Status Affected: None
Encoding:
1st word (source)
2nd word (dest.)
1110
1111
1011
xxxx
1zzz
xzzz
zzzzs
zzzzd
Description The contents of the source register are
moved to the destination register. The
addresses of the source and destination
registers are determined by adding the
7-bit literal offsets ‘zs’ or ‘zd’,
respectively, to the value of FSR2. Both
registers can be located anywhere in
the 4096-byte data memory space
(000h to FFFh).
The MOVSS instruction cannot use the
PCL, TOSU, TOSH or TOSL as the
destination register.
If the resultant source address points to
an indirect addressing register, the
value returned will be 00h. If the
resultant destination address points to
an indirect addressing register, the
instruction will execute as a NOP.
Words: 2
Cycles: 2
Q Cycle Activity:
Q1 Q2 Q3 Q4
Decode Determine
source addr
Determine
source addr
Read
source reg
Decode Determine
dest addr
Determine
dest addr
Write
to dest reg
Example: MOVSS [05h], [06h]
Before Instruction
FSR2 = 80h
Contents
of 85h = 33h
Contents
of 86h = 11h
After Instruction
FSR2 = 80h
Contents
of 85h = 33h
Contents
of 86h = 33h
PUSHL Store Literal at FSR2, Decrement FSR2
Syntax: PUSHL k
Operands: 0 ≤ k ≤ 255
Operation: k → (FSR2),
FSR2 – 1 → FSR2
Status Affected: None
Encoding: 1111 1010 kkkk kkkk
Description: The 8-bit literal ‘k’ is written to the data
memory address specified by FSR2. FSR2
is decremented by 1 after the operation.
This instruction allows users to push values
onto a software stack.
Words: 1
Cycles: 1
Q Cycle Activity:
Q1 Q2 Q3 Q4
Decode Read ‘k’ Process
data
Write to
destination
Example: PUSHL 08h
Before Instruction
FSR2H:FSR2L = 01ECh
Memory (01ECh) = 00h
After Instruction
FSR2H:FSR2L = 01EBh
Memory (01ECh) = 08h
© 2007 Microchip Technology Inc. Preliminary DS39631B-page 313
PIC18F2420/2520/4420/4520
SUBFSR Subtract Literal from FSR
Syntax: SUBFSR f, k
Operands: 0 ≤ k ≤ 63
f ∈ [ 0, 1, 2 ]
Operation: FSR(f) – k → FSRf
Status Affected: None
Encoding: 1110 1001 ffkk kkkk
Description: The 6-bit literal ‘k’ is subtracted from
the contents of the FSR specified by
‘f’.
Words: 1
Cycles: 1
Q Cycle Activity:
Q1 Q2 Q3 Q4
Decode Read
register ‘f’
Process
Data
Write to
destination
Example: SUBFSR 2, 23h
Before Instruction
FSR2 = 03FFh
After Instruction
FSR2 = 03DCh
SUBULNK Subtract Literal from FSR2 and Return
Syntax: SUBULNK k
Operands: 0 ≤ k ≤ 63
Operation: FSR2 – k → FSR2
(TOS) → PC
Status Affected: None
Encoding: 1110 1001 11kk kkkk
Description: The 6-bit literal ‘k’ is subtracted from the
contents of the FSR2. A RETURN is then
executed by loading the PC with the TOS.
The instruction takes two cycles to
execute; a NOP is performed during the
second cycle.
This may be thought of as a special case of
the SUBFSR instruction, where f = 3 (binary
‘11’); it operates only on FSR2.
Words: 1
Cycles: 2
Q Cycle Activity:
Q1 Q2 Q3 Q4
Decode Read
register ‘f’
Process
Data
Write to
destination
No
Operation
No
Operation
No
Operation
No
Operation
Example: SUBULNK 23h
Before Instruction
FSR2 = 03FFh
PC = 0100h
After Instruction
FSR2 = 03DCh
PC = (TOS)
PIC18F2420/2520/4420/4520
DS39631B-page 314 Preliminary © 2007 Microchip Technology Inc.
24.2.3 BYTE-ORIENTED AND
BIT-ORIENTED INSTRUCTIONS IN
INDEXED LITERAL OFFSET MODE
In addition to eight new commands in the extended set,
enabling the extended instruction set also enables
Indexed Literal Offset Addressing mode (Section 5.5.1
“Indexed Addressing with Literal Offset”). This has
a significant impact on the way that many commands of
the standard PIC18 instruction set are interpreted.
When the extended set is disabled, addresses embedded
in opcodes are treated as literal memory locations:
either as a location in the Access Bank (‘a’ = 0), or in a
GPR bank designated by the BSR (‘a’ = 1). When the
extended instruction set is enabled and ‘a’ = 0, however,
a file register argument of 5Fh or less is
interpreted as an offset from the pointer value in FSR2
and not as a literal address. For practical purposes, this
means that all instructions that use the Access RAM bit
as an argument – that is, all byte-oriented and bitoriented
instructions, or almost half of the core PIC18
instructions – may behave differently when the
extended instruction set is enabled.
When the content of FSR2 is 00h, the boundaries of the
Access RAM are essentially remapped to their original
values. This may be useful in creating backward
compatible code. If this technique is used, it may be
necessary to save the value of FSR2 and restore it
when moving back and forth between C and assembly
routines in order to preserve the stack pointer. Users
must also keep in mind the syntax requirements of the
extended instruction set (see Section 24.2.3.1
“Extended Instruction Syntax with Standard PIC18
Commands”).
Although the Indexed Literal Offset Addressing mode
can be very useful for dynamic stack and pointer
manipulation, it can also be very annoying if a simple
arithmetic operation is carried out on the wrong
register. Users who are accustomed to the PIC18 programming
must keep in mind that, when the extended
instruction set is enabled, register addresses of 5Fh or
less are used for Indexed Literal Offset Addressing.
Representative examples of typical byte-oriented and
bit-oriented instructions in the Indexed Literal Offset
Addressing mode are provided on the following page to
show how execution is affected. The operand conditions
shown in the examples are applicable to all
instructions of these types.
24.2.3.1 Extended Instruction Syntax with
Standard PIC18 Commands
When the extended instruction set is enabled, the file
register argument, ‘f’, in the standard byte-oriented and
bit-oriented commands is replaced with the literal offset
value, ‘k’. As already noted, this occurs only when ‘f’ is
less than or equal to 5Fh. When an offset value is used,
it must be indicated by square brackets (“[ ]”). As with
the extended instructions, the use of brackets indicates
to the compiler that the value is to be interpreted as an
index or an offset. Omitting the brackets, or using a
value greater than 5Fh within brackets, will generate an
error in the MPASM Assembler.
If the index argument is properly bracketed for Indexed
Literal Offset Addressing, the Access RAM argument is
never specified; it will automatically be assumed to be
‘0’. This is in contrast to standard operation (extended
instruction set disabled) when ‘a’ is set on the basis of
the target address. Declaring the Access RAM bit in
this mode will also generate an error in the MPASM
Assembler.
The destination argument, ‘d’, functions as before.
In the latest versions of the MPASM assembler,
language support for the extended instruction set must
be explicitly invoked. This is done with either the
command line option, /y, or the PE directive in the
source listing.
24.2.4 CONSIDERATIONS WHEN
ENABLING THE EXTENDED
INSTRUCTION SET
It is important to note that the extensions to the instruction
set may not be beneficial to all users. In particular,
users who are not writing code that uses a software
stack may not benefit from using the extensions to the
instruction set.
Additionally, the Indexed Literal Offset Addressing
mode may create issues with legacy applications
written to the PIC18 assembler. This is because
instructions in the legacy code may attempt to address
registers in the Access Bank below 5Fh. Since these
addresses are interpreted as literal offsets to FSR2
when the instruction set extension is enabled, the
application may read or write to the wrong data
addresses.
When porting an application to the PIC18F2420/2520/
4420/4520, it is very important to consider the type of
code. A large, re-entrant application that is written in ‘C’
and would benefit from efficient compilation will do well
when using the instruction set extensions. Legacy
applications that heavily use the Access Bank will most
likely not benefit from using the extended instruction
set.
Note: Enabling the PIC18 instruction set
extension may cause legacy applications
to behave erratically or fail entirely.
© 2007 Microchip Technology Inc. Preliminary DS39631B-page 315
PIC18F2420/2520/4420/4520
ADDWF
ADD W to Indexed
(Indexed Literal Offset mode)
Syntax: ADDWF [k] {,d}
Operands: 0 ≤ k ≤ 95
d ∈ [0,1]
Operation: (W) + ((FSR2) + k) → dest
Status Affected: N, OV, C, DC, Z
Encoding: 0010 01d0 kkkk kkkk
Description: The contents of W are added to the
contents of the register indicated by
FSR2, offset by the value ‘k’.
If ‘d’ is ‘0’, the result is stored in W. If ‘d’
is ‘1’, the result is stored back in
register ‘f’ (default).
Words: 1
Cycles: 1
Q Cycle Activity:
Q1 Q2 Q3 Q4
Decode Read ‘k’ Process
Data
Write to
destination
Example: ADDWF [OFST] , 0
Before Instruction
W = 17h
OFST = 2Ch
FSR2 = 0A00h
Contents
of 0A2Ch = 20h
After Instruction
W = 37h
Contents
of 0A2Ch = 20h
BSF
Bit Set Indexed
(Indexed Literal Offset mode)
Syntax: BSF [k], b
Operands: 0 ≤ f ≤ 95
0 ≤ b ≤ 7
Operation: 1 → ((FSR2) + k)
Status Affected: None
Encoding: 1000 bbb0 kkkk kkkk
Description: Bit ‘b’ of the register indicated by FSR2,
offset by the value ‘k’, is set.
Words: 1
Cycles: 1
Q Cycle Activity:
Q1 Q2 Q3 Q4
Decode Read
register ‘f’
Process
Data
Write to
destination
Example: BSF [FLAG_OFST], 7
Before Instruction
FLAG_OFST = 0Ah
FSR2 = 0A00h
Contents
of 0A0Ah = 55h
After Instruction
Contents
of 0A0Ah = D5h
SETF
Set Indexed
(Indexed Literal Offset mode)
Syntax: SETF [k]
Operands: 0 ≤ k ≤ 95
Operation: FFh → ((FSR2) + k)
Status Affected: None
Encoding: 0110 1000 kkkk kkkk
Description: The contents of the register indicated by
FSR2, offset by ‘k’, are set to FFh.
Words: 1
Cycles: 1
Q Cycle Activity:
Q1 Q2 Q3 Q4
Decode Read ‘k’ Process
Data
Write
register
Example: SETF [OFST]
Before Instruction
OFST = 2Ch
FSR2 = 0A00h
Contents
of 0A2Ch = 00h
After Instruction
Contents
of 0A2Ch = FFh
PIC18F2420/2520/4420/4520
DS39631B-page 316 Preliminary © 2007 Microchip Technology Inc.
24.2.5 SPECIAL CONSIDERATIONS WITH
MICROCHIP MPLAB® IDE TOOLS
The latest versions of Microchip’s software tools have
been designed to fully support the extended instruction
set of the PIC18F2420/2520/4420/4520 family of
devices. This includes the MPLAB C18 C compiler,
MPASM assembly language and MPLAB Integrated
Development Environment (IDE).
When selecting a target device for software
development, MPLAB IDE will automatically set default
configuration bits for that device. The default setting for
the XINST configuration bit is ‘0’, disabling the
extended instruction set and Indexed Literal Offset
Addressing mode. For proper execution of applications
developed to take advantage of the extended
instruction set, XINST must be set during
programming.
To develop software for the extended instruction set,
the user must enable support for the instructions and
the Indexed Addressing mode in their language tool(s).
Depending on the environment being used, this may be
done in several ways:
• A menu option, or dialog box within the
environment, that allows the user to configure the
language tool and its settings for the project
• A command line option
• A directive in the source code
These options vary between different compilers,
assemblers and development environments. Users are
encouraged to review the documentation accompanying
their development systems for the appropriate
information.
© 2007 Microchip Technology Inc. Preliminary DS39631B-page 317
PIC18F2420/2520/4420/4520
25.0 DEVELOPMENT SUPPORT
The PIC® microcontrollers are supported with a full
range of hardware and software development tools:
• Integrated Development Environment
- MPLAB® IDE Software
• Assemblers/Compilers/Linkers
- MPASMTM Assembler
- MPLAB C17 and MPLAB C18 C Compilers
- MPLINKTM Object Linker/
MPLIBTM Object Librarian
- MPLAB C30 C Compiler
- MPLAB ASM30 Assembler/Linker/Library
• Simulators
- MPLAB SIM Software Simulator
- MPLAB dsPIC30 Software Simulator
• Emulators
- MPLAB ICE 2000 In-Circuit Emulator
- MPLAB ICE 4000 In-Circuit Emulator
• In-Circuit Debugger
- MPLAB ICD 2
• Device Programmers
- PRO MATE® II Universal Device Programmer
- PICSTART® Plus Development Programmer
- MPLAB PM3 Device Programmer
• Low-Cost Demonstration Boards
- PICDEMTM 1 Demonstration Board
- PICDEM.netTM Demonstration Board
- PICDEM 2 Plus Demonstration Board
- PICDEM 3 Demonstration Board
- PICDEM 4 Demonstration Board
- PICDEM 17 Demonstration Board
- PICDEM 18R Demonstration Board
- PICDEM LIN Demonstration Board
- PICDEM USB Demonstration Board
• Evaluation Kits
- KEELOQ® Evaluation and Programming Tools
- PICDEM MSC
- microID® Developer Kits
- CAN
- PowerSmart® Developer Kits
- Analog
25.1 MPLAB Integrated Development
Environment Software
The MPLAB IDE software brings an ease of software
development previously unseen in the 8/16-bit microcontroller
market. The MPLAB IDE is a Windows®
based application that contains:
• An interface to debugging tools
- simulator
- programmer (sold separately)
- emulator (sold separately)
- in-circuit debugger (sold separately)
• A full-featured editor with color coded context
• A multiple project manager
• Customizable data windows with direct edit of
contents
• High-level source code debugging
• Mouse over variable inspection
• Extensive on-line help
The MPLAB IDE allows you to:
• Edit your source files (either assembly or C)
• One touch assemble (or compile) and download
to PIC emulator and simulator tools (automatically
updates all project information)
• Debug using:
- source files (assembly or C)
- mixed assembly and C
- machine code
MPLAB IDE supports multiple debugging tools in a
single development paradigm, from the cost effective
simulators, through low-cost in-circuit debuggers, to
full-featured emulators. This eliminates the learning
curve when upgrading to tools with increasing flexibility
and power.
25.2 MPASM Assembler
The MPASM assembler is a full-featured, universal
macro assembler for all PIC MCUs.
The MPASM assembler generates relocatable object
files for the MPLINK object linker, Intel® standard HEX
files, MAP files to detail memory usage and symbol reference,
absolute LST files that contain source lines and
generated machine code and COFF files for
debugging.
The MPASM assembler features include:
• Integration into MPLAB IDE projects
• User defined macros to streamline assembly code
• Conditional assembly for multi-purpose source
files
• Directives that allow complete control over the
assembly process
PIC18F2420/2520/4420/4520
DS39631B-page 318 Preliminary © 2007 Microchip Technology Inc.
25.3 MPLAB C17 and MPLAB C18
C Compilers
The MPLAB C17 and MPLAB C18 Code Development
Systems are complete ANSI C compilers for
Microchip’s PIC17CXXX and PIC18CXXX family of
microcontrollers. These compilers provide powerful
integration capabilities, superior code optimization and
ease of use not found with other compilers.
For easy source level debugging, the compilers provide
symbol information that is optimized to the MPLAB IDE
debugger.
25.4 MPLINK Object Linker/
MPLIB Object Librarian
The MPLINK object linker combines relocatable
objects created by the MPASM assembler and the
MPLAB C17 and MPLAB C18 C compilers. It can link
relocatable objects from precompiled libraries, using
directives from a linker script.
The MPLIB object librarian manages the creation and
modification of library files of precompiled code. When
a routine from a library is called from a source file, only
the modules that contain that routine will be linked in
with the application. This allows large libraries to be
used efficiently in many different applications.
The object linker/library features include:
• Efficient linking of single libraries instead of many
smaller files
• Enhanced code maintainability by grouping
related modules together
• Flexible creation of libraries with easy module
listing, replacement, deletion and extraction
25.5 MPLAB C30 C Compiler
The MPLAB C30 C compiler is a full-featured, ANSI
compliant, optimizing compiler that translates standard
ANSI C programs into dsPIC30F assembly language
source. The compiler also supports many command
line options and language extensions to take full
advantage of the dsPIC30F device hardware capabilities
and afford fine control of the compiler code
generator.
MPLAB C30 is distributed with a complete ANSI C
standard library. All library functions have been validated
and conform to the ANSI C library standard. The
library includes functions for string manipulation,
dynamic memory allocation, data conversion, timekeeping
and math functions (trigonometric, exponential
and hyperbolic). The compiler provides symbolic
information for high-level source debugging with the
MPLAB IDE.
25.6 MPLAB ASM30 Assembler, Linker
and Librarian
MPLAB ASM30 assembler produces relocatable
machine code from symbolic assembly language for
dsPIC30F devices. MPLAB C30 compiler uses the
assembler to produce it’s object file. The assembler
generates relocatable object files that can then be
archived or linked with other relocatable object files and
archives to create an executable file. Notable features
of the assembler include:
• Support for the entire dsPIC30F instruction set
• Support for fixed-point and floating-point data
• Command line interface
• Rich directive set
• Flexible macro language
• MPLAB IDE compatibility
25.7 MPLAB SIM Software Simulator
The MPLAB SIM software simulator allows code development
in a PC hosted environment by simulating the
PIC series microcontrollers on an instruction level. On
any given instruction, the data areas can be examined
or modified and stimuli can be applied from a file, or
user defined key press, to any pin. The execution can
be performed in Single-Step, Execute Until Break or
Trace mode.
The MPLAB SIM simulator fully supports symbolic
debugging using the MPLAB C17 and MPLAB C18
C Compilers, as well as the MPASM assembler. The
software simulator offers the flexibility to develop and
debug code outside of the laboratory environment,
making it an excellent, economical software
development tool.
25.8 MPLAB SIM30 Software Simulator
The MPLAB SIM30 software simulator allows code
development in a PC hosted environment by simulating
the dsPIC30F series microcontrollers on an instruction
level. On any given instruction, the data areas can be
examined or modified and stimuli can be applied from
a file, or user defined key press, to any of the pins.
The MPLAB SIM30 simulator fully supports symbolic
debugging using the MPLAB C30 C Compiler and
MPLAB ASM30 assembler. The simulator runs in either
a Command Line mode for automated tasks, or from
MPLAB IDE. This high-speed simulator is designed to
debug, analyze and optimize time intensive DSP
routines.
© 2007 Microchip Technology Inc. Preliminary DS39631B-page 319
PIC18F2420/2520/4420/4520
25.9 MPLAB ICE 2000
High-Performance Universal
In-Circuit Emulator
The MPLAB ICE 2000 universal in-circuit emulator is
intended to provide the product development engineer
with a complete microcontroller design tool set for PIC
microcontrollers. Software control of the MPLAB ICE
2000 in-circuit emulator is advanced by the MPLAB
Integrated Development Environment, which allows
editing, building, downloading and source debugging
from a single environment.
The MPLAB ICE 2000 is a full-featured emulator system
with enhanced trace, trigger and data monitoring
features. Interchangeable processor modules allow the
system to be easily reconfigured for emulation of different
processors. The universal architecture of the
MPLAB ICE in-circuit emulator allows expansion to
support new PIC microcontrollers.
The MPLAB ICE 2000 in-circuit emulator system has
been designed as a real-time emulation system with
advanced features that are typically found on more
expensive development tools. The PC platform and
Microsoft® Windows 32-bit operating system were
chosen to best make these features available in a
simple, unified application.
25.10 MPLAB ICE 4000
High-Performance Universal
In-Circuit Emulator
The MPLAB ICE 4000 universal in-circuit emulator is
intended to provide the product development engineer
with a complete microcontroller design tool set for highend
PIC microcontrollers. Software control of the
MPLAB ICE in-circuit emulator is provided by the
MPLAB Integrated Development Environment, which
allows editing, building, downloading and source
debugging from a single environment.
The MPLAB ICD 4000 is a premium emulator system,
providing the features of MPLAB ICE 2000, but with
increased emulation memory and high-speed performance
for dsPIC30F and PIC18XXXX devices. Its
advanced emulator features include complex triggering
and timing, up to 2 Mb of emulation memory and the
ability to view variables in real-time.
The MPLAB ICE 4000 in-circuit emulator system has
been designed as a real-time emulation system with
advanced features that are typically found on more
expensive development tools. The PC platform and
Microsoft Windows 32-bit operating system were
chosen to best make these features available in a
simple, unified application.
25.11 MPLAB ICD 2 In-Circuit Debugger
Microchip’s In-Circuit Debugger, MPLAB ICD 2, is a
powerful, low-cost, run-time development tool,
connecting to the host PC via an RS-232 or high-speed
USB interface. This tool is based on the Flash PIC
MCUs and can be used to develop for these and other
PIC microcontrollers. The MPLAB ICD 2 utilizes the incircuit
debugging capability built into the Flash devices.
This feature, along with Microchip’s In-Circuit Serial
ProgrammingTM (ICSPTM) protocol, offers cost effective
in-circuit Flash debugging from the graphical user interface
of the MPLAB Integrated Development
Environment. This enables a designer to develop and
debug source code by setting breakpoints, singlestepping
and watching variables, CPU status and
peripheral registers. Running at full speed enables testing
hardware and applications in real-time. MPLAB
ICD 2 also serves as a development programmer for
selected PIC devices.
25.12 PRO MATE II Universal Device
Programmer
The PRO MATE II is a universal, CE compliant device
programmer with programmable voltage verification at
VDDMIN and VDDMAX for maximum reliability. It features
an LCD display for instructions and error messages
and a modular detachable socket assembly to support
various package types. In Stand-Alone mode, the
PRO MATE II device programmer can read, verify and
program PIC devices without a PC connection. It can
also set code protection in this mode.
25.13 MPLAB PM3 Device Programmer
The MPLAB PM3 is a universal, CE compliant device
programmer with programmable voltage verification at
VDDMIN and VDDMAX for maximum reliability. It features
a large LCD display (128 x 64) for menus and error
messages and a modular detachable socket assembly
to support various package types. The ICSP™ cable
assembly is included as a standard item. In Stand-
Alone mode, the MPLAB PM3 device programmer can
read, verify and program PIC devices without a PC
connection. It can also set code protection in this mode.
MPLAB PM3 connects to the host PC via an RS-232 or
USB cable. MPLAB PM3 has high-speed communications
and optimized algorithms for quick programming
of large memory devices and incorporates an SD/MMC
card for file storage and secure data applications.
PIC18F2420/2520/4420/4520
DS39631B-page 320 Preliminary © 2007 Microchip Technology Inc.
25.14 PICSTART Plus Development
Programmer
The PICSTART Plus development programmer is an
easy-to-use, low-cost, prototype programmer. It connects
to the PC via a COM (RS-232) port. MPLAB
Integrated Development Environment software makes
using the programmer simple and efficient. The
PICSTART Plus development programmer supports
most PIC devices up to 40 pins. Larger pin count
devices, such as the PIC16C92X and PIC17C76X,
may be supported with an adapter socket. The
PICSTART Plus development programmer is CE
compliant.
25.15 PICDEM 1 PIC
Demonstration Board
The PICDEM 1 demonstration board demonstrates the
capabilities of the PIC16C5X (PIC16C54 to
PIC16C58A), PIC16C61, PIC16C62X, PIC16C71,
PIC16C8X, PIC17C42, PIC17C43 and PIC17C44. All
necessary hardware and software is included to run
basic demo programs. The sample microcontrollers
provided with the PICDEM 1 demonstration board can
be programmed with a PRO MATE II device programmer
or a PICSTART Plus development programmer.
The PICDEM 1 demonstration board can be connected
to the MPLAB ICE in-circuit emulator for testing. A
prototype area extends the circuitry for additional application
components. Features include an RS-232
interface, a potentiometer for simulated analog input,
push button switches and eight LEDs.
25.16 PICDEM.net Internet/Ethernet
Demonstration Board
The PICDEM.net demonstration board is an Internet/
Ethernet demonstration board using the PIC18F452
microcontroller and TCP/IP firmware. The board
supports any 40-pin DIP device that conforms to the
standard pinout used by the PIC16F877 or
PIC18C452. This kit features a user friendly TCP/IP
stack, web server with HTML, a 24L256 Serial
EEPROM for Xmodem download to web pages into
Serial EEPROM, ICSP/MPLAB ICD 2 interface connector,
an Ethernet interface, RS-232 interface and a
16 x 2 LCD display. Also included is the book and
CD-ROM “TCP/IP Lean, Web Servers for Embedded
Systems,” by Jeremy Bentham
25.17 PICDEM 2 Plus
Demonstration Board
The PICDEM 2 Plus demonstration board supports
many 18, 28 and 40-pin microcontrollers, including
PIC16F87X and PIC18FXX2 devices. All the necessary
hardware and software is included to run the demonstration
programs. The sample microcontrollers
provided with the PICDEM 2 demonstration board can
be programmed with a PRO MATE II device programmer,
PICSTART Plus development programmer, or
MPLAB ICD 2 with a Universal Programmer Adapter.
The MPLAB ICD 2 and MPLAB ICE in-circuit emulators
may also be used with the PICDEM 2 demonstration
board to test firmware. A prototype area extends the
circuitry for additional application components. Some
of the features include an RS-232 interface, a 2 x 16
LCD display, a piezo speaker, an on-board temperature
sensor, four LEDs and sample PIC18F452 and
PIC16F877 Flash microcontrollers.
25.18 PICDEM 3 PIC16C92X
Demonstration Board
The PICDEM 3 demonstration board supports the
PIC16C923 and PIC16C924 in the PLCC package. All
the necessary hardware and software is included to run
the demonstration programs.
25.19 PICDEM 4 8/14/18-Pin
Demonstration Board
The PICDEM 4 can be used to demonstrate the capabilities
of the 8, 14 and 18-pin PIC16XXXX and
PIC18XXXX MCUs, including the PIC16F818/819,
PIC16F87/88, PIC16F62XA and the PIC18F1320
family of microcontrollers. PICDEM 4 is intended to
showcase the many features of these low pin count
parts, including LIN and Motor Control using ECCP.
Special provisions are made for low-power operation
with the supercapacitor circuit and jumpers allow onboard
hardware to be disabled to eliminate current
draw in this mode. Included on the demo board are provisions
for Crystal, RC or Canned Oscillator modes, a
five volt regulator for use with a nine volt wall adapter
or battery, DB-9 RS-232 interface, ICD connector for
programming via ICSP and development with MPLAB
ICD 2, 2 x 16 liquid crystal display, PCB footprints for
H-Bridge motor driver, LIN transceiver and EEPROM.
Also included are: header for expansion, eight LEDs,
four potentiometers, three push buttons and a prototyping
area. Included with the kit is a PIC16F627A and
a PIC18F1320. Tutorial firmware is included along
with the User’s Guide.
© 2007 Microchip Technology Inc. Preliminary DS39631B-page 321
PIC18F2420/2520/4420/4520
25.20 PICDEM 17 Demonstration Board
The PICDEM 17 demonstration board is an evaluation
board that demonstrates the capabilities of several
Microchip microcontrollers, including PIC17C752,
PIC17C756A, PIC17C762 and PIC17C766. A programmed
sample is included. The PRO MATE II device
programmer, or the PICSTART Plus development programmer,
can be used to reprogram the device for user
tailored application development. The PICDEM 17
demonstration board supports program download and
execution from external on-board Flash memory. A
generous prototype area is available for user hardware
expansion.
25.21 PICDEM 18R PIC18C601/801
Demonstration Board
The PICDEM 18R demonstration board serves to assist
development of the PIC18C601/801 family of Microchip
microcontrollers. It provides hardware implementation
of both 8-bit Multiplexed/Demultiplexed and 16-bit
Memory modes. The board includes 2 Mb external
Flash memory and 128 Kb SRAM memory, as well as
serial EEPROM, allowing access to the wide range of
memory types supported by the PIC18C601/801.
25.22 PICDEM LIN PIC16C43X
Demonstration Board
The powerful LIN hardware and software kit includes a
series of boards and three PIC microcontrollers. The
small footprint PIC16C432 and PIC16C433 are used
as slaves in the LIN communication and feature onboard
LIN transceivers. A PIC16F874 Flash
microcontroller serves as the master. All three microcontrollers
are programmed with firmware to provide
LIN bus communication.
25.23 PICkitTM 1 Flash Starter Kit
A complete “development system in a box”, the PICkit™
Flash Starter Kit includes a convenient multi-section
board for programming, evaluation and development of
8/14-pin Flash PIC® microcontrollers. Powered via USB,
the board operates under a simple Windows GUI. The
PICkit 1 Starter Kit includes the User’s Guide (on CD
ROM), PICkit 1 tutorial software and code for various
applications. Also included are MPLAB® IDE (Integrated
Development Environment) software, software and
hardware “Tips 'n Tricks for 8-pin Flash PIC®
Microcontrollers” Handbook and a USB interface cable.
Supports all current 8/14-pin Flash PIC microcontrollers,
as well as many future planned devices.
25.24 PICDEM USB PIC16C7X5
Demonstration Board
The PICDEM USB Demonstration Board shows off the
capabilities of the PIC16C745 and PIC16C765 USB
microcontrollers. This board provides the basis for
future USB products.
25.25 Evaluation and
Programming Tools
In addition to the PICDEM series of circuits, Microchip
has a line of evaluation kits and demonstration software
for these products.
• KEELOQ evaluation and programming tools for
Microchip’s HCS Secure Data Products
• CAN developers kit for automotive network
applications
• Analog design boards and filter design software
• PowerSmart battery charging evaluation/
calibration kits
• IrDA® development kit
• microID development and rfLabTM development
software
• SEEVAL® designer kit for memory evaluation and
endurance calculations
• PICDEM MSC demo boards for Switching mode
power supply, high-power IR driver, delta sigma
ADC and flow rate sensor
Check the Microchip web page and the latest Product
Selector Guide for the complete list of demonstration
and evaluation kits.
PIC18F2420/2520/4420/4520
DS39631B-page 322 Preliminary © 2007 Microchip Technology Inc.
NOTES:
© 2007 Microchip Technology Inc. Preliminary DS39631B-page 323
PIC18F2420/2520/4420/4520
26.0 ELECTRICAL CHARACTERISTICS
Absolute Maximum Ratings (†)
Ambient temperature under bias.............................................................................................................-40°C to +125°C
Storage temperature .............................................................................................................................. -65°C to +150°C
Voltage on any pin with respect to VSS (except VDD, MCLR and RA4) .......................................... -0.3V to (VDD + 0.3V)
Voltage on VDD with respect to VSS ......................................................................................................... -0.3V to +7.5V
Voltage on MCLR with respect to VSS (Note 2) ......................................................................................... 0V to +13.25V
Total power dissipation (Note 1) ...............................................................................................................................1.0W
Maximum current out of VSS pin ...........................................................................................................................300 mA
Maximum current into VDD pin ..............................................................................................................................250 mA
Input clamp current, IIK (VI < 0 or VI > VDD)...................................................................................................................... ±20 mA
Output clamp current, IOK (VO < 0 or VO > VDD) .............................................................................................................. ±20 mA
Maximum output current sunk by any I/O pin..........................................................................................................25 mA
Maximum output current sourced by any I/O pin ....................................................................................................25 mA
Maximum current sunk by all ports .......................................................................................................................200 mA
Maximum current sourced by all ports ..................................................................................................................200 mA
Note 1: Power dissipation is calculated as follows:
Pdis = VDD x {IDD – Σ IOH} + Σ {(VDD – VOH) x IOH} + Σ(VOL x IOL)
2: Voltage spikes below VSS at the MCLR/VPP/RE3 pin, inducing currents greater than 80 mA, may cause
latch-up. Thus, a series resistor of 50-100Ω should be used when applying a “low” level to the MCLR/VPP/
RE3 pin, rather than pulling this pin directly to VSS.
† NOTICE: Stresses above those listed under “Absolute Maximum Ratings” may cause permanent damage to the
device. This is a stress rating only and functional operation of the device at those or any other conditions above those
indicated in the operation listings of this specification is not implied. Exposure to maximum rating conditions for
extended periods may affect device reliability.
PIC18F2420/2520/4420/4520
DS39631B-page 324 Preliminary © 2007 Microchip Technology Inc.
FIGURE 26-1: PIC18F2420/2520/4420/4520 VOLTAGE-FREQUENCY GRAPH (INDUSTRIAL)
FIGURE 26-2: PIC18LF2X1X/4X1X VOLTAGE-FREQUENCY GRAPH (INDUSTRIAL)
Frequency
Voltage
6.0V
5.5V
4.5V
4.0V
2.0V
40 MHz
5.0V
3.5V
3.0V
2.5V
PIC18FX42X/X52X
4.2V
Frequency
Voltage
6.0V
5.5V
4.5V
4.0V
2.0V
40 MHz
5.0V
3.5V
3.0V
2.5V
PIC18LFX42X/X52X
FMAX = (16.36 MHz/V) (VDDAPPMIN – 2.0V) + 4 MHz
Note: VDDAPPMIN is the minimum voltage of the PIC® device in the application.
4 MHz
4.2V
© 2007 Microchip Technology Inc. Preliminary DS39631B-page 325
PIC18F2420/2520/4420/4520
26.1 DC Characteristics:Supply Voltage
PIC18F2420/2520/4420/4520 (Industrial)
PIC18LF2X1X/4X1X (Industrial)
PIC18LF2X1X/4X1X
(Industrial)
Standard Operating Conditions (unless otherwise stated)
Operating temperature -40°C ≤ TA ≤ +85°C for industrial
PIC18FX42X/X52X
(Industrial)
Standard Operating Conditions (unless otherwise stated)
Operating temperature -40°C ≤ TA ≤ +85°C for industrial
Param
No.
Symbol Characteristic Min Typ Max Units Conditions
D001 VDD Supply Voltage 2.0 — 5.5 V HS, XT, RC and LP Oscillator modes
D002 VDR RAM Data Retention
Voltage(1)
1.5 — — V
D003 VPOR VDD Start Voltage
to ensure internal
Power-on Reset signal
— — 0.7 V See section on Power-on Reset for details
D004 SVDD VDD Rise Rate
to ensure internal
Power-on Reset signal
0.05 — — V/ms See section on Power-on Reset for details
D005 VBOR Brown-out Reset Voltage
BORV1:BORV0 = 11 1.94 2.05 2.16 V
BORV1:BORV0 = 10 2.65 2.79 2.93 V
BORV1:BORV0 = 01 4.11 4.33 4.55 V
BORV1:BORV0 = 00 4.36 4.59 4.82 V
Legend: Shading of rows is to assist in readability of the table.
Note 1: This is the limit to which VDD can be lowered in Sleep mode, or during a device Reset, without losing RAM
data.
PIC18F2420/2520/4420/4520
DS39631B-page 326 Preliminary © 2007 Microchip Technology Inc.
26.2 DC Characteristics: Power-Down and Supply Current
PIC18F2420/2520/4420/4520 (Industrial)
PIC18LF2X1X/4X1X (Industrial)
PIC18LF2X1X/4X1X
(Industrial)
Standard Operating Conditions (unless otherwise stated)
Operating temperature -40°C ≤ TA ≤ +85°C for industrial
PIC18FX42X/X52X
(Industrial)
Standard Operating Conditions (unless otherwise stated)
Operating temperature -40°C ≤ TA ≤ +85°C for industrial
ParamNo. Device Typ Max Units Conditions
Power-down Current (IPD)(1)
PIC18LF2X1X/4X1X 20 950 nA -40°C
VDD = 2.0V,
(Sleep mode)
0.02 1.0 μA +25°C
0.6 1.1 μA +85°C
PIC18LF2X1X/4X1X 0.03 1.4 μA -40°C
VDD = 3.0V,
(Sleep mode)
0.03 1.5 μA +25°C
0.8 1.6 μA +85°C
All devices 0.04 1.9 μA -40°C
VDD = 5.0V,
(Sleep mode)
0.04 2.0 μA +25°C
1.7 2.1 μA +85°C
Legend: Shading of rows is to assist in readability of the table.
Note 1: The power-down current in Sleep mode does not depend on the oscillator type. Power-down current is measured
with the part in Sleep mode, with all I/O pins in high-impedance state and tied to VDD or VSS and all features that
add delta current disabled (such as WDT, Timer1 Oscillator, BOR, etc.).
2: The supply current is mainly a function of operating voltage, frequency and mode. Other factors, such as I/O pin
loading and switching rate, oscillator type and circuit, internal code execution pattern and temperature, also have
an impact on the current consumption.
The test conditions for all IDD measurements in active operation mode are:
OSC1 = external square wave, from rail-to-rail; all I/O pins tri-stated, pulled to VDD;
MCLR = VDD; WDT enabled/disabled as specified.
3: For RC oscillator configurations, current through REXT is not included. The current through the resistor can be
estimated by the formula Ir = VDD/2REXT (mA) with REXT in kΩ.
4: Standard low-cost 32 kHz crystals have an operating temperature range of -10°C to +70°C. Extended temperature
crystals are available at a much higher cost.
5: BOR and HLVD enable internal band gap reference. With both modules enabled, current consumption will be
less than the sum of both specifications.
© 2007 Microchip Technology Inc. Preliminary DS39631B-page 327
PIC18F2420/2520/4420/4520
Supply Current (IDD)(2,3)
PIC18LF2X1X/4X1X 15 31.5 μA -40°C
FOSC = 31 kHz
(RC_RUN mode,
INTRC source)
15 30 μA +25°C VDD = 2.0V
15 28.5 μA +85°C
PIC18LF2X1X/4X1X 40 63 μA -40°C
35 60 μA +25°C VDD = 3.0V
30 57 μA +85°C
All devices 105 168 μA -40°C
90 160 μA +25°C VDD = 5.0V
80 152 μA +85°C
PIC18LF2X1X/4X1X 0.32 630 μA -40°C
FOSC = 1 MHz
(RC_RUN mode,
INTOSC source)
0.33 600 μA +25°C VDD = 2.0V
0.33 570 μA +85°C
PIC18LF2X1X/4X1X 0.6 1.3 mA -40°C
0.55 1.2 mA +25°C VDD = 3.0V
0.6 1.1 mA +85°C
All devices 1.1 2.3 mA -40°C
1.1 2.2 mA +25°C VDD = 5.0V
1.0 2.1 mA +85°C
26.2 DC Characteristics: Power-Down and Supply Current
PIC18F2420/2520/4420/4520 (Industrial)
PIC18LF2X1X/4X1X (Industrial) (Continued)
PIC18LF2X1X/4X1X
(Industrial)
Standard Operating Conditions (unless otherwise stated)
Operating temperature -40°C ≤ TA ≤ +85°C for industrial
PIC18FX42X/X52X
(Industrial)
Standard Operating Conditions (unless otherwise stated)
Operating temperature -40°C ≤ TA ≤ +85°C for industrial
ParamNo. Device Typ Max Units Conditions
Legend: Shading of rows is to assist in readability of the table.
Note 1: The power-down current in Sleep mode does not depend on the oscillator type. Power-down current is measured
with the part in Sleep mode, with all I/O pins in high-impedance state and tied to VDD or VSS and all features that
add delta current disabled (such as WDT, Timer1 Oscillator, BOR, etc.).
2: The supply current is mainly a function of operating voltage, frequency and mode. Other factors, such as I/O pin
loading and switching rate, oscillator type and circuit, internal code execution pattern and temperature, also have
an impact on the current consumption.
The test conditions for all IDD measurements in active operation mode are:
OSC1 = external square wave, from rail-to-rail; all I/O pins tri-stated, pulled to VDD;
MCLR = VDD; WDT enabled/disabled as specified.
3: For RC oscillator configurations, current through REXT is not included. The current through the resistor can be
estimated by the formula Ir = VDD/2REXT (mA) with REXT in kΩ.
4: Standard low-cost 32 kHz crystals have an operating temperature range of -10°C to +70°C. Extended temperature
crystals are available at a much higher cost.
5: BOR and HLVD enable internal band gap reference. With both modules enabled, current consumption will be
less than the sum of both specifications.
PIC18F2420/2520/4420/4520
DS39631B-page 328 Preliminary © 2007 Microchip Technology Inc.
Supply Current (IDD)(2,3)
PIC18LF2X1X/4X1X 0.8 2.1 μA -40°C
FOSC = 4 MHz
(RC_RUN mode,
INTRC source)
0.8 2.0 μA +25°C VDD = 2.0V
0.8 1.9 μA +85°C
PIC18LF2X1X/4X1X 1.3 2.7 mA -40°C
1.3 2.6 mA +25°C VDD = 3.0V
1.3 2.5 mA +85°C
All devices 2.5 5.3 mA -40°C
2.5 5.0 mA +25°C VDD = 5.0V
2.5 4.8 mA +85°C
PIC18LF2X1X/4X1X 2.9 6.5 μA -40°C
FOSC = 31 kHz
(RC_IDLE mode,
INTRC source)
3.1 6.2 μA +25°C VDD = 2.0V
3.6 5.9 μA +85°C
PIC18LF2X1X/4X1X 4.5 10.1 μA -40°C
4.8 9.6 μA +25°C VDD = 3.0V
5.8 9.1 μA +85°C
All devices 9.2 15.8 μA -40°C
9.8 15 μA +25°C VDD = 5.0V
11.4 14.3 μA +85°C
26.2 DC Characteristics: Power-Down and Supply Current
PIC18F2420/2520/4420/4520 (Industrial)
PIC18LF2X1X/4X1X (Industrial) (Continued)
PIC18LF2X1X/4X1X
(Industrial)
Standard Operating Conditions (unless otherwise stated)
Operating temperature -40°C ≤ TA ≤ +85°C for industrial
PIC18FX42X/X52X
(Industrial)
Standard Operating Conditions (unless otherwise stated)
Operating temperature -40°C ≤ TA ≤ +85°C for industrial
ParamNo. Device Typ Max Units Conditions
Legend: Shading of rows is to assist in readability of the table.
Note 1: The power-down current in Sleep mode does not depend on the oscillator type. Power-down current is measured
with the part in Sleep mode, with all I/O pins in high-impedance state and tied to VDD or VSS and all features that
add delta current disabled (such as WDT, Timer1 Oscillator, BOR, etc.).
2: The supply current is mainly a function of operating voltage, frequency and mode. Other factors, such as I/O pin
loading and switching rate, oscillator type and circuit, internal code execution pattern and temperature, also have
an impact on the current consumption.
The test conditions for all IDD measurements in active operation mode are:
OSC1 = external square wave, from rail-to-rail; all I/O pins tri-stated, pulled to VDD;
MCLR = VDD; WDT enabled/disabled as specified.
3: For RC oscillator configurations, current through REXT is not included. The current through the resistor can be
estimated by the formula Ir = VDD/2REXT (mA) with REXT in kΩ.
4: Standard low-cost 32 kHz crystals have an operating temperature range of -10°C to +70°C. Extended temperature
crystals are available at a much higher cost.
5: BOR and HLVD enable internal band gap reference. With both modules enabled, current consumption will be
less than the sum of both specifications.
© 2007 Microchip Technology Inc. Preliminary DS39631B-page 329
PIC18F2420/2520/4420/4520
Supply Current (IDD)(2,3)
PIC18LF2X1X/4X1X 165 315 μA -40°C
FOSC = 1 MHz
(RC_IDLE mode,
INTOSC source)
175 300 μA +25°C VDD = 2.0V
190 285 μA +85°C
PIC18LF2X1X/4X1X 250 470 μA -40°C
270 450 μA +25°C VDD = 3.0V
290 430 μA +85°C
All devices 500 840 μA -40°C
520 800 μA +25°C VDD = 5.0V
550 760 μA +85°C
PIC18LF2X1X/4X1X 340 525 μA -40°C
FOSC = 4 MHz
(RC_IDLE mode,
INTOSC source)
350 500 μA +25°C VDD = 2.0V
360 475 μA +85°C
PIC18LF2X1X/4X1X 520 735 μA -40°C
540 700 μA +25°C VDD = 3.0V
580 665 μA +85°C
All devices 1.0 1.6 mA -40°C
1.1 1.5 mA +25°C VDD = 5.0V
1.1 1.4 mA +85°C
26.2 DC Characteristics: Power-Down and Supply Current
PIC18F2420/2520/4420/4520 (Industrial)
PIC18LF2X1X/4X1X (Industrial) (Continued)
PIC18LF2X1X/4X1X
(Industrial)
Standard Operating Conditions (unless otherwise stated)
Operating temperature -40°C ≤ TA ≤ +85°C for industrial
PIC18FX42X/X52X
(Industrial)
Standard Operating Conditions (unless otherwise stated)
Operating temperature -40°C ≤ TA ≤ +85°C for industrial
ParamNo. Device Typ Max Units Conditions
Legend: Shading of rows is to assist in readability of the table.
Note 1: The power-down current in Sleep mode does not depend on the oscillator type. Power-down current is measured
with the part in Sleep mode, with all I/O pins in high-impedance state and tied to VDD or VSS and all features that
add delta current disabled (such as WDT, Timer1 Oscillator, BOR, etc.).
2: The supply current is mainly a function of operating voltage, frequency and mode. Other factors, such as I/O pin
loading and switching rate, oscillator type and circuit, internal code execution pattern and temperature, also have
an impact on the current consumption.
The test conditions for all IDD measurements in active operation mode are:
OSC1 = external square wave, from rail-to-rail; all I/O pins tri-stated, pulled to VDD;
MCLR = VDD; WDT enabled/disabled as specified.
3: For RC oscillator configurations, current through REXT is not included. The current through the resistor can be
estimated by the formula Ir = VDD/2REXT (mA) with REXT in kΩ.
4: Standard low-cost 32 kHz crystals have an operating temperature range of -10°C to +70°C. Extended temperature
crystals are available at a much higher cost.
5: BOR and HLVD enable internal band gap reference. With both modules enabled, current consumption will be
less than the sum of both specifications.
PIC18F2420/2520/4420/4520
DS39631B-page 330 Preliminary © 2007 Microchip Technology Inc.
Supply Current (IDD)(2,3)
PIC18LF2X1X/4X1X 250 420 μA -40°C
FOSC = 1 MHZ
(PRI_RUN,
EC oscillator)
260 400 μA +25°C VDD = 2.0V
250 380 μA +85°C
PIC18LF2X1X/4X1X 550 740 μA -40°C
480 700 μA +25°C VDD = 3.0V
460 670 μA +85°C
All devices 1.2 1.6 mA -40°C
1.1 1.5 mA +25°C VDD = 5.0V
1.0 1.4 mA +85°C
PIC18LF2X1X/4X1X 0.72 1.6 mA -40°C
FOSC = 4 MHz
(PRI_RUN,
EC oscillator)
0.74 1.5 mA +25°C VDD = 2.0V
0.74 1.4 mA +85°C
PIC18LF2X1X/4X1X 1.3 2.6 mA -40°C
1.3 2.5 mA +25°C VDD = 3.0V
1.3 2.4 mA +85°C
All devices 2.7 4.7 mA -40°C
2.6 4.5 mA +25°C VDD = 5.0V
2.5 4.3 mA +85°C
All devices 15 26 mA -40°C
FOSC = 40 MHZ
(PRI_RUN,
EC oscillator)
16 25 mA +25°C VDD = 4.2V
16 24 mA +85°C
All devices 21 32 mA -40°C
21 30 mA +25°C VDD = 5.0V
21 28 mA +85°C
26.2 DC Characteristics: Power-Down and Supply Current
PIC18F2420/2520/4420/4520 (Industrial)
PIC18LF2X1X/4X1X (Industrial) (Continued)
PIC18LF2X1X/4X1X
(Industrial)
Standard Operating Conditions (unless otherwise stated)
Operating temperature -40°C ≤ TA ≤ +85°C for industrial
PIC18FX42X/X52X
(Industrial)
Standard Operating Conditions (unless otherwise stated)
Operating temperature -40°C ≤ TA ≤ +85°C for industrial
ParamNo. Device Typ Max Units Conditions
Legend: Shading of rows is to assist in readability of the table.
Note 1: The power-down current in Sleep mode does not depend on the oscillator type. Power-down current is measured
with the part in Sleep mode, with all I/O pins in high-impedance state and tied to VDD or VSS and all features that
add delta current disabled (such as WDT, Timer1 Oscillator, BOR, etc.).
2: The supply current is mainly a function of operating voltage, frequency and mode. Other factors, such as I/O pin
loading and switching rate, oscillator type and circuit, internal code execution pattern and temperature, also have
an impact on the current consumption.
The test conditions for all IDD measurements in active operation mode are:
OSC1 = external square wave, from rail-to-rail; all I/O pins tri-stated, pulled to VDD;
MCLR = VDD; WDT enabled/disabled as specified.
3: For RC oscillator configurations, current through REXT is not included. The current through the resistor can be
estimated by the formula Ir = VDD/2REXT (mA) with REXT in kΩ.
4: Standard low-cost 32 kHz crystals have an operating temperature range of -10°C to +70°C. Extended temperature
crystals are available at a much higher cost.
5: BOR and HLVD enable internal band gap reference. With both modules enabled, current consumption will be
less than the sum of both specifications.
© 2007 Microchip Technology Inc. Preliminary DS39631B-page 331
PIC18F2420/2520/4420/4520
Supply Current (IDD)(2,3)
All devices 7.5 16 mA -40°C
VDD = 4.2V
FOSC = 4 MHZ
(PRI_RUN HS+PLL)
7.4 15 mA +25°C
7.3 14 mA +85°C
All devices 10 21 mA -40°C
VDD = 5.0V
FOSC = 4 MHZ
10 20 mA +25°C (PRI_RUN HS+PLL)
9.7 19 mA +85°C
All devices 17 35 mA -40°C
VDD = 4.2V
FOSC = 10 MHZ
17 34 mA +25°C (PRI_RUN HS+PLL)
17 33 mA +85°C
All devices 23 46 mA -40°C
VDD = 5.0V
FOSC = 10 MHZ
23 45 mA +25°C (PRI_RUN HS+PLL)
23 43 mA +85°C
26.2 DC Characteristics: Power-Down and Supply Current
PIC18F2420/2520/4420/4520 (Industrial)
PIC18LF2X1X/4X1X (Industrial) (Continued)
PIC18LF2X1X/4X1X
(Industrial)
Standard Operating Conditions (unless otherwise stated)
Operating temperature -40°C ≤ TA ≤ +85°C for industrial
PIC18FX42X/X52X
(Industrial)
Standard Operating Conditions (unless otherwise stated)
Operating temperature -40°C ≤ TA ≤ +85°C for industrial
ParamNo. Device Typ Max Units Conditions
Legend: Shading of rows is to assist in readability of the table.
Note 1: The power-down current in Sleep mode does not depend on the oscillator type. Power-down current is measured
with the part in Sleep mode, with all I/O pins in high-impedance state and tied to VDD or VSS and all features that
add delta current disabled (such as WDT, Timer1 Oscillator, BOR, etc.).
2: The supply current is mainly a function of operating voltage, frequency and mode. Other factors, such as I/O pin
loading and switching rate, oscillator type and circuit, internal code execution pattern and temperature, also have
an impact on the current consumption.
The test conditions for all IDD measurements in active operation mode are:
OSC1 = external square wave, from rail-to-rail; all I/O pins tri-stated, pulled to VDD;
MCLR = VDD; WDT enabled/disabled as specified.
3: For RC oscillator configurations, current through REXT is not included. The current through the resistor can be
estimated by the formula Ir = VDD/2REXT (mA) with REXT in kΩ.
4: Standard low-cost 32 kHz crystals have an operating temperature range of -10°C to +70°C. Extended temperature
crystals are available at a much higher cost.
5: BOR and HLVD enable internal band gap reference. With both modules enabled, current consumption will be
less than the sum of both specifications.
PIC18F2420/2520/4420/4520
DS39631B-page 332 Preliminary © 2007 Microchip Technology Inc.
Supply Current (IDD)(2,3)
PIC18LF2X1X/4X1X 65 130 μA -40°C
FOSC = 1 MHz
(PRI_IDLE mode,
EC oscillator)
65 120 μA +25°C VDD = 2.0V
70 115 μA +85°C
PIC18LF2X1X/4X1X 120 270 μA -40°C
120 250 μA +25°C VDD = 3.0V
130 240 μA +85°C
All devices 300 480 μA -40°C
240 450 μA +25°C VDD = 5.0V
300 430 μA +85°C
PIC18LF2X1X/4X1X 260 475 μA -40°C
FOSC = 4 MHz
(PRI_IDLE mode,
EC oscillator)
255 450 μA +25°C VDD = 2.0V
270 430 μA +85°C
PIC18LF2X1X/4X1X 420 900 μA -40°C
430 850 μA +25°C VDD = 3.0V
450 810 μA +85°C
All devices 0.9 1.5 mA -40°C
0.9 1.4 mA +25°C VDD = 5.0V
0.9 1.3 mA +85°C
All devices 6.0 9.5 mA -40°C
FOSC = 40 MHz
(PRI_IDLE mode,
EC oscillator)
6.2 9.0 mA +25°C VDD = 4.2 V
6.6 8.6 mA +85°C
All devices 8.1 12.6 mA -40°C
9.1 12.0 mA +25°C VDD = 5.0V
8.3 11.4 mA +85°C
26.2 DC Characteristics: Power-Down and Supply Current
PIC18F2420/2520/4420/4520 (Industrial)
PIC18LF2X1X/4X1X (Industrial) (Continued)
PIC18LF2X1X/4X1X
(Industrial)
Standard Operating Conditions (unless otherwise stated)
Operating temperature -40°C ≤ TA ≤ +85°C for industrial
PIC18FX42X/X52X
(Industrial)
Standard Operating Conditions (unless otherwise stated)
Operating temperature -40°C ≤ TA ≤ +85°C for industrial
ParamNo. Device Typ Max Units Conditions
Legend: Shading of rows is to assist in readability of the table.
Note 1: The power-down current in Sleep mode does not depend on the oscillator type. Power-down current is measured
with the part in Sleep mode, with all I/O pins in high-impedance state and tied to VDD or VSS and all features that
add delta current disabled (such as WDT, Timer1 Oscillator, BOR, etc.).
2: The supply current is mainly a function of operating voltage, frequency and mode. Other factors, such as I/O pin
loading and switching rate, oscillator type and circuit, internal code execution pattern and temperature, also have
an impact on the current consumption.
The test conditions for all IDD measurements in active operation mode are:
OSC1 = external square wave, from rail-to-rail; all I/O pins tri-stated, pulled to VDD;
MCLR = VDD; WDT enabled/disabled as specified.
3: For RC oscillator configurations, current through REXT is not included. The current through the resistor can be
estimated by the formula Ir = VDD/2REXT (mA) with REXT in kΩ.
4: Standard low-cost 32 kHz crystals have an operating temperature range of -10°C to +70°C. Extended temperature
crystals are available at a much higher cost.
5: BOR and HLVD enable internal band gap reference. With both modules enabled, current consumption will be
less than the sum of both specifications.
© 2007 Microchip Technology Inc. Preliminary DS39631B-page 333
PIC18F2420/2520/4420/4520
Supply Current (IDD)(2,3)
PIC18LF2X1X/4X1X 14 31.5 μA -10°C
FOSC = 32 kHz(4)
(SEC_RUN mode,
Timer1 as clock)
15 30 μA +25°C VDD = 2.0V
16 29 μA +70°C
PIC18LF2X1X/4X1X 40 74 μA -10°C
35 70 μA +25°C VDD = 3.0V
31 67 μA +70°C
All devices 99 126 μA -10°C
81 120 μA +25°C VDD = 5.0V
75 114 μA +70°C
PIC18LF2X1X/4X1X 2.5 7.4 μA -10°C
FOSC = 32 kHz(4)
(SEC_IDLE mode,
Timer1 as clock)
3.7 7.0 μA +25°C VDD = 2.0V
4.5 6.7 μA +70°C
PIC18LF2X1X/4X1X 5.0 10.5 μA -10°C
5.4 10 μA +25°C VDD = 3.0V
6.3 9.5 μA +70°C
All devices 8.5 17 μA -10°C
9.0 16 μA +25°C VDD = 5.0V
10.5 15 μA +70°C
26.2 DC Characteristics: Power-Down and Supply Current
PIC18F2420/2520/4420/4520 (Industrial)
PIC18LF2X1X/4X1X (Industrial) (Continued)
PIC18LF2X1X/4X1X
(Industrial)
Standard Operating Conditions (unless otherwise stated)
Operating temperature -40°C ≤ TA ≤ +85°C for industrial
PIC18FX42X/X52X
(Industrial)
Standard Operating Conditions (unless otherwise stated)
Operating temperature -40°C ≤ TA ≤ +85°C for industrial
ParamNo. Device Typ Max Units Conditions
Legend: Shading of rows is to assist in readability of the table.
Note 1: The power-down current in Sleep mode does not depend on the oscillator type. Power-down current is measured
with the part in Sleep mode, with all I/O pins in high-impedance state and tied to VDD or VSS and all features that
add delta current disabled (such as WDT, Timer1 Oscillator, BOR, etc.).
2: The supply current is mainly a function of operating voltage, frequency and mode. Other factors, such as I/O pin
loading and switching rate, oscillator type and circuit, internal code execution pattern and temperature, also have
an impact on the current consumption.
The test conditions for all IDD measurements in active operation mode are:
OSC1 = external square wave, from rail-to-rail; all I/O pins tri-stated, pulled to VDD;
MCLR = VDD; WDT enabled/disabled as specified.
3: For RC oscillator configurations, current through REXT is not included. The current through the resistor can be
estimated by the formula Ir = VDD/2REXT (mA) with REXT in kΩ.
4: Standard low-cost 32 kHz crystals have an operating temperature range of -10°C to +70°C. Extended temperature
crystals are available at a much higher cost.
5: BOR and HLVD enable internal band gap reference. With both modules enabled, current consumption will be
less than the sum of both specifications.
PIC18F2420/2520/4420/4520
DS39631B-page 334 Preliminary © 2007 Microchip Technology Inc.
Module Differential Currents (ΔIWDT, ΔIBOR, ΔILVD, ΔIOSCB, ΔIAD)
D022
(ΔIWDT)
Watchdog Timer 1.3 7.6 μA -40°C
1.4 8.0 μA +25°C VDD = 2.0V
2.0 8.4 μA +85°C
1.9 11.4 μA -40°C
2.0 12.0 μA +25°C VDD = 3.0V
2.8 12.6 μA +85°C
4.0 14.3 μA -40°C
5.5 15.0 μA +25°C VDD = 5.0V
5.6 15.8 μA +85°C
D022A
(ΔIBOR)
Brown-out Reset(5) 35 52 μA -40°C to +85°C VDD = 3.0V
40 63 μA -40°C to +85°C VDD = 5.0V
40 63 μA -40°C to +85°C VDD = 5.0V Sleep mode,
BOREN1:BOREN0 = 10
D022B
(ΔILVD)
High/Low-Voltage
Detect(5)
22 47 μA -40°C to +85°C VDD = 2.0V
25 58 μA -40°C to +85°C VDD = 3.0V
29 69 μA -40°C to +85°C VDD = 5.0V
D025
(ΔIOSCB)
Timer1 Oscillator 0.01 4.8 μA -10°C
0.01 5.0 μA +25°C VDD = 2.0V 32 kHz on Timer1(4)
0.01 5.3 μA +70°C
0.01 7.6 μA -10°C
0.01 8.0 μA +25°C VDD = 3.0V 32 kHz on Timer1(4)
0.01 8.4 μA +70°C
0.01 9.5 μA -10°C
0.01 10.0 μA +25°C VDD = 5.0V 32 kHz on Timer1(4)
0.01 10.5 μA +70°C
D026
(ΔIAD)
A/D Converter 1.0 2.0 μA VDD = 2.0V
1.0 2.0 μA VDD = 3.0V A/D on, not converting
1.0 2.0 μA VDD = 5.0V
26.2 DC Characteristics: Power-Down and Supply Current
PIC18F2420/2520/4420/4520 (Industrial)
PIC18LF2X1X/4X1X (Industrial) (Continued)
PIC18LF2X1X/4X1X
(Industrial)
Standard Operating Conditions (unless otherwise stated)
Operating temperature -40°C ≤ TA ≤ +85°C for industrial
PIC18FX42X/X52X
(Industrial)
Standard Operating Conditions (unless otherwise stated)
Operating temperature -40°C ≤ TA ≤ +85°C for industrial
ParamNo. Device Typ Max Units Conditions
Legend: Shading of rows is to assist in readability of the table.
Note 1: The power-down current in Sleep mode does not depend on the oscillator type. Power-down current is measured
with the part in Sleep mode, with all I/O pins in high-impedance state and tied to VDD or VSS and all features that
add delta current disabled (such as WDT, Timer1 Oscillator, BOR, etc.).
2: The supply current is mainly a function of operating voltage, frequency and mode. Other factors, such as I/O pin
loading and switching rate, oscillator type and circuit, internal code execution pattern and temperature, also have
an impact on the current consumption.
The test conditions for all IDD measurements in active operation mode are:
OSC1 = external square wave, from rail-to-rail; all I/O pins tri-stated, pulled to VDD;
MCLR = VDD; WDT enabled/disabled as specified.
3: For RC oscillator configurations, current through REXT is not included. The current through the resistor can be
estimated by the formula Ir = VDD/2REXT (mA) with REXT in kΩ.
4: Standard low-cost 32 kHz crystals have an operating temperature range of -10°C to +70°C. Extended temperature
crystals are available at a much higher cost.
5: BOR and HLVD enable internal band gap reference. With both modules enabled, current consumption will be
less than the sum of both specifications.
© 2007 Microchip Technology Inc. Preliminary DS39631B-page 335
PIC18F2420/2520/4420/4520
26.3 DC Characteristics: PIC18F2420/2520/4420/4520 (Industrial)
PIC18LF2X1X/4X1X (Industrial)
DC CHARACTERISTICS
Standard Operating Conditions (unless otherwise stated)
Operating temperature -40°C ≤ TA ≤ +85°C for industrial
Param
No.
Symbol Characteristic Min Max Units Conditions
VIL Input Low Voltage
I/O ports:
D030 with TTL buffer VSS 0.15 VDD V VDD < 4.5V
D030A — 0.8 V 4.5V ≤ VDD ≤ 5.5V
D031 with Schmitt Trigger buffer
RC3 and RC4
VSS
VSS
0.2 VDD
0.3 VDD
V
V
D032 MCLR VSS 0.2 VDD V
D033 OSC1 VSS 0.3 VDD V HS, HSPLL modes
D033A
D033B
D034
OSC1
OSC1
T13CKI
VSS
VSS
VSS
0.2 VDD
0.3 VDD
0.3 VDD
V
V
V
RC, EC modes(1)
XT, LP modes
VIH Input High Voltage
I/O ports:
D040 with TTL buffer 0.25 VDD + 0.8V VDD V VDD < 4.5V
D040A 2.0 VDD V 4.5V ≤ VDD ≤ 5.5V
D041 with Schmitt Trigger buffer
RC3 and RC4
0.8 VDD
0.7 VDD
VDD
VDD
V
V
D042 MCLR 0.8 VDD VDD V
D043 OSC1 0.7 VDD VDD V HS, HSPLL modes
D043A
D043B
D043C
D044
OSC1
OSC1
OSC1
T13CKI
0.8 VDD
0.9 VDD
1.6
1.6
VDD
VDD
VDD
VDD
V
V
V
V
EC mode
RC mode(1)
XT, LP modes
IIL Input Leakage Current(2,3)
D060 I/O ports — ±1 μA VSS ≤ VPIN ≤ VDD,
Pin at high-impedance
D061 MCLR — ±5 μA Vss ≤ VPIN ≤ VDD
D063 OSC1 — ±5 μA Vss ≤ VPIN ≤ VDD
IPU Weak Pull-up Current
D070 IPURB PORTB weak pull-up current 50 400 μA VDD = 5V, VPIN = VSS
Note 1: In RC oscillator configuration, the OSC1/CLKI pin is a Schmitt Trigger input. It is not recommended that the
PIC® device be driven with an external clock while in RC mode.
2: The leakage current on the MCLR pin is strongly dependent on the applied voltage level. The specified
levels represent normal operating conditions. Higher leakage current may be measured at different input
voltages.
3: Negative current is defined as current sourced by the pin.
4: Parameter is characterized but not tested.
PIC18F2420/2520/4420/4520
DS39631B-page 336 Preliminary © 2007 Microchip Technology Inc.
VOL Output Low Voltage
D080 I/O ports — 0.6 V IOL = 8.5 mA, VDD = 4.5V,
-40°C to +85°C
D083 OSC2/CLKO
(RC, RCIO, EC, ECIO modes)
— 0.6 V IOL = 1.6 mA, VDD = 4.5V,
-40°C to +85°C
VOH Output High Voltage(3)
D090 I/O ports VDD – 0.7 — V IOH = -3.0 mA, VDD = 4.5V,
-40°C to +85°C
D092 OSC2/CLKO
(RC, RCIO, EC, ECIO modes)
VDD – 0.7 — V IOH = -1.3 mA, VDD = 4.5V,
-40°C to +85°C
Capacitive Loading Specs
on Output Pins
D100(4) COSC2 OSC2 pin — 15 pF In XT, HS and LP modes
when external clock is
used to drive OSC1
D101 CIO All I/O pins and OSC2
(in RC mode)
— 50 pF To meet the AC Timing
Specifications
D102 CB SCL, SDA — 400 pF I2C™ Specification
26.3 DC Characteristics: PIC18F2420/2520/4420/4520 (Industrial)
PIC18LF2X1X/4X1X (Industrial) (Continued)
DC CHARACTERISTICS
Standard Operating Conditions (unless otherwise stated)
Operating temperature -40°C ≤ TA ≤ +85°C for industrial
Param
No.
Symbol Characteristic Min Max Units Conditions
Note 1: In RC oscillator configuration, the OSC1/CLKI pin is a Schmitt Trigger input. It is not recommended that the
PIC® device be driven with an external clock while in RC mode.
2: The leakage current on the MCLR pin is strongly dependent on the applied voltage level. The specified
levels represent normal operating conditions. Higher leakage current may be measured at different input
voltages.
3: Negative current is defined as current sourced by the pin.
4: Parameter is characterized but not tested.
© 2007 Microchip Technology Inc. Preliminary DS39631B-page 337
PIC18F2420/2520/4420/4520
TABLE 26-1: MEMORY PROGRAMMING REQUIREMENTS
DC CHARACTERISTICS
Standard Operating Conditions (unless otherwise stated)
Operating temperature -40°C ≤ TA ≤ +85°C for industrial
Param
No.
Sym Characteristic Min Typ† Max Units Conditions
Internal Program Memory
Programming Specifications(1)
D110 VPP Voltage on MCLR/VPP/RE3 pin 9.00 — 13.25 V (Note 3)
D113 IDDP Supply Current during
Programming
— — 10 mA
Data EEPROM Memory
D120 ED Byte Endurance 100K 1M — E/W -40°C to +85°C
D121 VDRW VDD for Read/Write VMIN — 5.5 V Using EECON to read/write
VMIN = Minimum operating
voltage
D122 TDEW Erase/Write Cycle Time — 4 — ms
D123 TRETD Characteristic Retention 40 — — Year Provided no other
specifications are violated
D124 TREF Number of Total Erase/Write
Cycles before Refresh(2)
1M 10M — E/W -40°C to +85°C
Program Flash Memory
D130 EP Cell Endurance 10K 100K — E/W -40°C to +85°C
D131 VPR VDD for Read VMIN — 5.5 V VMIN = Minimum operating
voltage
D132 VIE VDD for Block Erase 4.5 — 5.5 V Using ICSP port
D132A VIW VDD for Externally Timed Erase
or Write
4.5 — 5.5 V Using ICSP port
D132B VPEW VDD for Self-timed Write VMIN — 5.5 V VMIN = Minimum operating
voltage
D133 TIE ICSP Block Erase Cycle Time — 4 — ms VDD > 4.5V
D133A TIW ICSP Erase or Write Cycle Time
(externally timed)
1 — — ms VDD > 4.5V
D133A TIW Self-timed Write Cycle Time — 2 — ms
D134 TRETD Characteristic Retention 40 100 — Year Provided no other
specifications are violated
† Data in “Typ” column is at 5.0V, 25°C unless otherwise stated. These parameters are for design guidance
only and are not tested.
Note 1: These specifications are for programming the on-chip program memory through the use of table write
instructions.
2: Refer to Section 7.8 “Using the Data EEPROM” for a more detailed discussion on data EEPROM
endurance.
3: Required only if single-supply programming is disabled.
PIC18F2420/2520/4420/4520
DS39631B-page 338 Preliminary © 2007 Microchip Technology Inc.
TABLE 26-2: COMPARATOR SPECIFICATIONS
TABLE 26-3: VOLTAGE REFERENCE SPECIFICATIONS
Operating Conditions: 3.0V < VDD < 5.5V, -40°C < TA < +85°C (unless otherwise stated).
Param
No.
Sym Characteristics Min Typ Max Units Comments
D300 VIOFF Input Offset Voltage — ±5.0 ±10 mV
D301 VICM Input Common Mode Voltage* 0 — VDD – 1.5 V
D302 CMRR Common Mode Rejection Ratio* 55 — — dB
300 TRESP Response Time(1)* — 150 400 ns PIC18FXXXX
300A — 150 600 ns PIC18LFXXXX,
VDD = 2.0V
301 TMC2OV Comparator Mode Change to
Output Valid*
— — 10 μs
* These parameters are characterized but not tested.
Note 1: Response time measured with one comparator input at (VDD – 1.5)/2, while the other input transitions
from VSS to VDD.
Operating Conditions: 3.0V < VDD < 5.5V, -40°C < TA < +85°C (unless otherwise stated).
Param
No.
Sym Characteristics Min Typ Max Units Comments
D310 VRES Resolution VDD/24 — VDD/32 LSb
D311 VRAA Absolute Accuracy — — 1/2 LSb Low Range (CVRR = 1)
D312 VRUR Unit Resistor Value (R)* — 2k — Ω
310 TSET Settling Time(1)* — — 10 μs
* These parameters are characterized but not tested.
Note 1: Settling time measured while CVRR = 1 and CVR3:CVR0 transitions from ‘0000’ to ‘1111’.
© 2007 Microchip Technology Inc. Preliminary DS39631B-page 339
PIC18F2420/2520/4420/4520
FIGURE 26-3: HIGH/LOW-VOLTAGE DETECT CHARACTERISTICS
TABLE 26-4: HIGH/LOW-VOLTAGE DETECT CHARACTERISTICS
VLVD
HLVDIF
VDD
(HLVDIF set by hardware)
(HLVDIF can be
cleared in software)
Standard Operating Conditions (unless otherwise stated)
Operating temperature -40°C ≤ TA ≤ +85°C for industrial
Param
No.
Symbol Characteristic Min Typ† Max Units Conditions
D420 HLVD Voltage on VDD
Transition High-to-Low
LVV = 0000 2.12 2.17 2.22 V
LVV = 0001 2.18 2.23 2.28 V
LVV = 0010 2.31 2.36 2.42 V
LVV = 0011 2.38 2.44 2.49 V
LVV = 0100 2.54 2.60 2.66 V
LVV = 0101 2.72 2.79 2.85 V
LVV = 0110 2.82 2.89 2.95 V
LVV = 0111 3.05 3.12 3.19 V
LVV = 1000 3.31 3.39 3.47 V
LVV = 1001 3.46 3.55 3.63 V
LVV = 1010 3.63 3.71 3.80 V
LVV = 1011 3.81 3.90 3.99 V
LVV = 1100 4.01 4.11 4.20 V
LVV = 1101 4.23 4.33 4.43 V
LVV = 1110 4.48 4.59 4.69 V
† Production tested at TAMB = 25°C. Specifications over temperature limits ensured by characterization.
PIC18F2420/2520/4420/4520
DS39631B-page 340 Preliminary © 2007 Microchip Technology Inc.
26.4 AC (Timing) Characteristics
26.4.1 TIMING PARAMETER SYMBOLOGY
The timing parameter symbols have been created
using one of the following formats:
1. TppS2ppS 3. TCC:ST (I2C specifications only)
2. TppS 4. Ts (I2C specifications only)
T
F Frequency T Time
Lowercase letters (pp) and their meanings:
pp
cc CCP1 osc OSC1
ck CLKO rd RD
cs CS rw RD or WR
di SDI sc SCK
do SDO ss SS
dt Data in t0 T0CKI
io I/O port t1 T13CKI
mc MCLR wr WR
Uppercase letters and their meanings:
S
F Fall P Period
H High R Rise
I Invalid (High-impedance) V Valid
L Low Z High-impedance
I2C only
AA output access High High
BUF Bus free Low Low
TCC:ST (I2C specifications only)
CC
HD Hold SU Setup
ST
DAT DATA input hold STO Stop condition
STA Start condition
© 2007 Microchip Technology Inc. Preliminary DS39631B-page 341
PIC18F2420/2520/4420/4520
26.4.2 TIMING CONDITIONS
The temperature and voltages specified in Table 26-5
apply to all timing specifications unless otherwise
noted. Figure 26-4 specifies the load conditions for the
timing specifications.
TABLE 26-5: TEMPERATURE AND VOLTAGE SPECIFICATIONS – AC
FIGURE 26-4: LOAD CONDITIONS FOR DEVICE TIMING SPECIFICATIONS
Note: Because of space limitations, the generic
terms “PIC18FXXXX” and “PIC18LFXXXX”
are used throughout this section to refer to
the PIC18F2420/2520/4420/4520 and
PIC18LF2X1X/4X1X families of devices
specifically and only those devices.
AC CHARACTERISTICS
Standard Operating Conditions (unless otherwise stated)
Operating temperature -40°C ≤ TA ≤ +85°C for industrial
Operating voltage VDD range as described in DC spec Section 26.1 and
Section 26.3.
LF parts operate for industrial temperatures only.
VDD/2
CL
RL
Pin
Pin
VSS
VSS
CL
RL = 464Ω
CL = 50 pF for all pins except OSC2/CLKO
and including D and E outputs as ports
Load Condition 1 Load Condition 2
PIC18F2420/2520/4420/4520
DS39631B-page 342 Preliminary © 2007 Microchip Technology Inc.
26.4.3 TIMING DIAGRAMS AND SPECIFICATIONS
FIGURE 26-5: EXTERNAL CLOCK TIMING (ALL MODES EXCEPT PLL)
TABLE 26-6: EXTERNAL CLOCK TIMING REQUIREMENTS
OSC1
CLKO
Q4 Q1 Q2 Q3 Q4 Q1
1
2
3 3 4 4
Param.
No.
Symbol Characteristic Min Max Units Conditions
1A FOSC External CLKI Frequency(1) DC 40 MHz EC, ECIO Oscillator mode
Oscillator Frequency(1) DC 4 MHz RC Oscillator mode
0.1 4 MHz XT Oscillator mode
4 25 MHz HS Oscillator mode
4 10 MHz HS + PLL Oscillator mode
5 33 kHz LP Oscillator mode
1 TOSC External CLKI Period(1) 25 — ns EC, ECIO Oscillator mode
Oscillator Period(1) 250 — ns RC Oscillator mode
250 10,000 ns XT Oscillator mode
40
100
250
250
ns
ns
HS Oscillator mode
HS + PLL Oscillator mode
30 — μs LP Oscillator mode
2 TCY Instruction Cycle Time(1) 100 — ns TCY = 4/FOSC
3 TOSL,
TOSH
External Clock in (OSC1)
High or Low Time
30 — ns XT Oscillator mode
2.5 — μs LP Oscillator mode
10 — ns HS Oscillator mode
4 TOSR,
TOSF
External Clock in (OSC1)
Rise or Fall Time
— 20 ns XT Oscillator mode
— 50 ns LP Oscillator mode
— 7.5 ns HS Oscillator mode
Note 1: Instruction cycle period (TCY) equals four times the input oscillator time base period for all configurations
except PLL. All specified values are based on characterization data for that particular oscillator type under
standard operating conditions with the device executing code. Exceeding these specified limits may result
in an unstable oscillator operation and/or higher than expected current consumption. All devices are tested
to operate at “min.” values with an external clock applied to the OSC1/CLKI pin. When an external clock
input is used, the “max.” cycle time limit is “DC” (no clock) for all devices.
© 2007 Microchip Technology Inc. Preliminary DS39631B-page 343
PIC18F2420/2520/4420/4520
TABLE 26-7: PLL CLOCK TIMING SPECIFICATIONS (VDD = 4.2V TO 5.5V)
TABLE 26-8: AC CHARACTERISTICS: INTERNAL RC ACCURACY
PIC18F2420/2520/4420/4520 (INDUSTRIAL)
PIC18LF2X1X/4X1X (INDUSTRIAL)
Param
No.
Sym Characteristic Min Typ† Max Units Conditions
F10 FOSC Oscillator Frequency Range 4 — 10 MHz HS mode only
F11 FSYS On-Chip VCO System Frequency 16 — 40 MHz HS mode only
F12 trc PLL Start-up Time (Lock Time) — — 2 ms
F13 ΔCLK CLKO Stability (Jitter) -2 — +2 %
† Data in “Typ” column is at 5V, 25°C unless otherwise stated. These parameters are for design guidance
only and are not tested.
PIC18LF2X1X/4X1X
(Industrial)
Standard Operating Conditions (unless otherwise stated)
Operating temperature -40°C ≤ TA ≤ +85°C for industrial
PIC18FX42X/X52X
(Industrial)
Standard Operating Conditions (unless otherwise stated)
Operating temperature -40°C ≤ TA ≤ +85°C for industrial
Param
No.
Device Min Typ Max Units Conditions
INTOSC Accuracy @ Freq = 8 MHz, 4 MHz, 2 MHz, 1 MHz, 500 kHz, 250 kHz, 125 kHz(1)
PIC18LF2X1X/4X1X -2 +/-1 2 % +25°C VDD = 2.7-3.3V
-5 — 5 % -10°C to +85°C VDD = 2.7-3.3V
-10 +/-1 10 % -40°C to +85°C VDD = 2.7-3.3V
PIC18FX42X/X52X -2 +/-1 2 % +25°C VDD = 4.5-5.5V
-5 — 5 % -10°C to +85°C VDD = 4.5-5.5V
-10 +/-1 10 % -40°C to +85°C VDD = 4.5-5.5V
INTRC Accuracy @ Freq = 31 kHz(2)
PIC18LF2X1X/4X1X 26.562 — 35.938 kHz -40°C to +85°C VDD = 2.7-3.3V
PIC18FX42X/X52X 26.562 — 35.938 kHz -40°C to +85°C VDD = 4.5-5.5V
Legend: Shading of rows is to assist in readability of the table.
Note 1: Frequency calibrated at 25°C. OSCTUNE register can be used to compensate for temperature drift.
2: INTRC frequency after calibration.
3: Change of INTRC frequency as VDD changes.
PIC18F2420/2520/4420/4520
DS39631B-page 344 Preliminary © 2007 Microchip Technology Inc.
FIGURE 26-6: CLKO AND I/O TIMING
TABLE 26-9: CLKO AND I/O TIMING REQUIREMENTS
Note: Refer to Figure 26-4 for load conditions.
OSC1
CLKO
I/O pin
(Input)
I/O pin
(Output)
Q4 Q1 Q2 Q3
10
13
14
17
20, 21
19 18
15
11
12
16
Old Value New Value
Param
No.
Symbol Characteristic Min Typ Max Units Conditions
10 TosH2ckL OSC1 ↑ to CLKO ↓ — 75 200 ns (Note 1)
11 TosH2ckH OSC1 ↑ to CLKO ↑ — 75 200 ns (Note 1)
12 TckR CLKO Rise Time — 35 100 ns (Note 1)
13 TckF CLKO Fall Time — 35 100 ns (Note 1)
14 TckL2ioV CLKO ↓ to Port Out Valid — — 0.5 TCY + 20 ns (Note 1)
15 TioV2ckH Port In Valid before CLKO ↑ 0.25 TCY + 25 — — ns (Note 1)
16 TckH2ioI Port In Hold after CLKO ↑ 0 — — ns (Note 1)
17 TosH2ioV OSC1 ↑ (Q1 cycle) to Port Out Valid — 50 150 ns
18 TosH2ioI OSC1 ↑ (Q2 cycle) to
Port Input Invalid
(I/O in hold time)
PIC18FXXXX 100 — — ns
18A PIC18LFXXXX 200 — — ns VDD = 2.0V
19 TioV2osH Port Input Valid to OSC1 ↑ (I/O in setup time) 0 — — ns
20 TioR Port Output Rise Time PIC18FXXXX — 10 25 ns
20A PIC18LFXXXX — — 60 ns VDD = 2.0V
21 TioF Port Output Fall Time PIC18FXXXX — 10 25 ns
21A PIC18LFXXXX — — 60 ns VDD = 2.0V
22† TINP INT pin High or Low Time TCY — — ns
23† TRBP RB7:RB4 Change INT High or Low Time TCY — — ns
24† TRCP RC7:RC4 Change INT High or Low Time 20 ns
† These parameters are asynchronous events not related to any internal clock edges.
Note 1: Measurements are taken in RC mode, where CLKO output is 4 x TOSC.
© 2007 Microchip Technology Inc. Preliminary DS39631B-page 345
PIC18F2420/2520/4420/4520
FIGURE 26-7: RESET, WATCHDOG TIMER, OSCILLATOR START-UP TIMER AND
POWER-UP TIMER TIMING
FIGURE 26-8: BROWN-OUT RESET TIMING
TABLE 26-10: RESET, WATCHDOG TIMER, OSCILLATOR START-UP TIMER, POWER-UP TIMER
AND BROWN-OUT RESET REQUIREMENTS
Param.
No.
Symbol Characteristic Min Typ Max Units Conditions
30 TmcL MCLR Pulse Width (low) 2 — — μs
31 TWDT Watchdog Timer Time-out Period
(no postscaler)
— 4.00 TBD ms
32 TOST Oscillation Start-up Timer Period 1024 TOSC — 1024 TOSC — TOSC = OSC1 period
33 TPWRT Power-up Timer Period — 65.5 TBD ms
34 TIOZ I/O High-Impedance from MCLR
Low or Watchdog Timer Reset
— 2 — μs
35 TBOR Brown-out Reset Pulse Width 200 — — μs VDD ≤ BVDD (see D005)
36 TIVRST Time for Internal Reference
Voltage to become Stable
— 20 50 μs
37 TLVD High/Low-Voltage Detect Pulse Width 200 — — μs VDD ≤ VLVD
38 TCSD CPU Start-up Time 5 — 10 μs
39 TIOBST Time for INTOSC to Stabilize — 1 — ms
Legend: TBD = To Be Determined
VDD
MCLR
Internal
POR
PWRT
Time-out
OSC
Time-out
Internal
Reset
Watchdog
Timer
Reset
33
32
30
31
34
I/O pins
34
Note: Refer to Figure 26-4 for load conditions.
VDD BVDD
35
VBGAP = 1.2V
VIRVST
Enable Internal
Internal Reference
36
Reference Voltage
Voltage Stable
PIC18F2420/2520/4420/4520
DS39631B-page 346 Preliminary © 2007 Microchip Technology Inc.
FIGURE 26-9: TIMER0 AND TIMER1 EXTERNAL CLOCK TIMINGS
TABLE 26-11: TIMER0 AND TIMER1 EXTERNAL CLOCK REQUIREMENTS
Note: Refer to Figure 26-4 for load conditions.
46
47
45
48
41
42
40
T0CKI
T1OSO/T13CKI
TMR0 or
TMR1
Param
No.
Symbol Characteristic Min Max Units Conditions
40 Tt0H T0CKI High Pulse Width No prescaler 0.5 TCY + 20 — ns
With prescaler 10 — ns
41 Tt0L T0CKI Low Pulse Width No prescaler 0.5 TCY + 20 — ns
With prescaler 10 — ns
42 Tt0P T0CKI Period No prescaler TCY + 10 — ns
With prescaler Greater of:
20 ns or
(TCY + 40)/N
— ns N = prescale
value
(1, 2, 4,..., 256)
45 Tt1H T13CKI
High Time
Synchronous, no prescaler 0.5 TCY + 20 — ns
Synchronous,
with prescaler
PIC18FXXXX 10 — ns
PIC18LFXXXX 25 — ns VDD = 2.0V
Asynchronous PIC18FXXXX 30 — ns
PIC18LFXXXX 50 — ns VDD = 2.0V
46 Tt1L T13CKI Low
Time
Synchronous, no prescaler 0.5 TCY + 5 — ns
Synchronous,
with prescaler
PIC18FXXXX 10 — ns
PIC18LFXXXX 25 — ns VDD = 2.0V
Asynchronous PIC18FXXXX 30 — ns
PIC18LFXXXX 50 — ns VDD = 2.0V
47 Tt1P T13CKI
Input Period
Synchronous Greater of:
20 ns or
(TCY + 40)/N
— ns N = prescale
value (1, 2, 4, 8)
Asynchronous 60 — ns
Ft1 T13CKI Oscillator Input Frequency Range DC 50 kHz
48 Tcke2tmrI Delay from External T13CKI Clock Edge to
Timer Increment
2 TOSC 7 TOSC —
© 2007 Microchip Technology Inc. Preliminary DS39631B-page 347
PIC18F2420/2520/4420/4520
FIGURE 26-10: CAPTURE/COMPARE/PWM TIMINGS (ALL CCP MODULES)
TABLE 26-12: CAPTURE/COMPARE/PWM REQUIREMENTS (ALL CCP MODULES)
Note: Refer to Figure 26-4 for load conditions.
CCPx
(Capture Mode)
50 51
52
CCPx
53 54
(Compare or PWM Mode)
Param
No.
Symbol Characteristic Min Max Units Conditions
50 TccL CCPx Input Low
Time
No prescaler 0.5 TCY + 20 — ns
With
prescaler
PIC18FXXXX 10 — ns
PIC18LFXXXX 20 — ns VDD = 2.0V
51 TccH CCPx Input
High Time
No prescaler 0.5 TCY + 20 — ns
With
prescaler
PIC18FXXXX 10 — ns
PIC18LFXXXX 20 — ns VDD = 2.0V
52 TccP CCPx Input Period 3 TCY + 40
N
— ns N = prescale
value (1, 4 or 16)
53 TccR CCPx Output Fall Time PIC18FXXXX — 25 ns
PIC18LFXXXX — 45 ns VDD = 2.0V
54 TccF CCPx Output Fall Time PIC18FXXXX — 25 ns
PIC18LFXXXX — 45 ns VDD = 2.0V
PIC18F2420/2520/4420/4520
DS39631B-page 348 Preliminary © 2007 Microchip Technology Inc.
FIGURE 26-11: PARALLEL SLAVE PORT TIMING (PIC18F4420/4520)
TABLE 26-13: PARALLEL SLAVE PORT REQUIREMENTS (PIC18F4420/4520)
Note: Refer to Figure 26-4 for load conditions.
RE2/CS
RE0/RD
RE1/WR
RD7:RD0
62
63
64
65
Param.
No.
Symbol Characteristic Min Max Units Conditions
62 TdtV2wrH Data In Valid before WR ↑ or CS ↑
(setup time)
20 — ns
63 TwrH2dtI WR ↑ or CS ↑ to Data–In
Invalid (hold time)
PIC18FXXXX 20 — ns
PIC18LFXXXX 35 — ns VDD = 2.0V
64 TrdL2dtV RD ↓ and CS ↓ to Data–Out Valid — 80 ns
65 TrdH2dtI RD ↑ or CS ↓ to Data–Out Invalid 10 30 ns
66 TibfINH Inhibit of the IBF Flag bit being Cleared from
WR ↑ or CS ↑
— 3 TCY
© 2007 Microchip Technology Inc. Preliminary DS39631B-page 349
PIC18F2420/2520/4420/4520
FIGURE 26-12: EXAMPLE SPI MASTER MODE TIMING (CKE = 0)
TABLE 26-14: EXAMPLE SPI MODE REQUIREMENTS (MASTER MODE, CKE = 0)
SS
SCK
(CKP = 0)
SCK
(CKP = 1)
SDO
SDI
70
71 72
73
74
75, 76
79 78 80
78 79
MSb bit 6 - - - - - -1 LSb
MSb In bit 6 - - - -1 LSb In
Note: Refer to Figure 26-4 for load conditions.
Param
No.
Symbol Characteristic Min Max Units Conditions
70 TssL2scH,
TssL2scL
SS ↓ to SCK ↓ or SCK ↑ Input TCY — ns
71 TscH SCK Input High Time
(Slave mode)
Continuous 1.25 TCY + 30 — ns
71A Single Byte 40 — ns (Note 1)
72 TscL SCK Input Low Time
(Slave mode)
Continuous 1.25 TCY + 30 — ns
72A Single Byte 40 — ns (Note 1)
73 TdiV2scH,
TdiV2scL
Setup Time of SDI Data Input to SCK Edge 100 — ns
73A Tb2b Last Clock Edge of Byte 1 to the 1st Clock Edge
of Byte 2
1.5 TCY + 40 — ns (Note 2)
74 TscH2diL,
TscL2diL
Hold Time of SDI Data Input to SCK Edge 100 — ns
75 TdoR SDO Data Output Rise Time PIC18FXXXX — 25 ns
PIC18LFXXXX — 45 ns VDD = 2.0V
76 TdoF SDO Data Output Fall Time — 25 ns
78 TscR SCK Output Rise Time
(Master mode)
PIC18FXXXX — 25 ns
PIC18LFXXXX — 45 ns VDD = 2.0V
79 TscF SCK Output Fall Time (Master mode) — 25 ns
80 TscH2doV,
TscL2doV
SDO Data Output Valid after
SCK Edge
PIC18FXXXX — 50 ns
PIC18LFXXXX — 100 ns VDD = 2.0V
Note 1: Requires the use of Parameter #73A.
2: Only if Parameter #71A and #72A are used.
PIC18F2420/2520/4420/4520
DS39631B-page 350 Preliminary © 2007 Microchip Technology Inc.
FIGURE 26-13: EXAMPLE SPI MASTER MODE TIMING (CKE = 1)
TABLE 26-15: EXAMPLE SPI MODE REQUIREMENTS (MASTER MODE, CKE = 1)
SS
SCK
(CKP = 0)
SCK
(CKP = 1)
SDO
SDI
81
71 72
74
75, 76
78
80
MSb
79
73
MSb In
bit 6 - - - - - -1
bit 6 - - - -1 LSb In
LSb
Note: Refer to Figure 26-4 for load conditions.
Param.
No.
Symbol Characteristic Min Max Units Conditions
71 TscH SCK Input High Time
(Slave mode)
Continuous 1.25 TCY + 30 — ns
71A Single Byte 40 — ns (Note 1)
72 TscL SCK Input Low Time
(Slave mode)
Continuous 1.25 TCY + 30 — ns
72A Single Byte 40 — ns (Note 1)
73 TdiV2scH,
TdiV2scL
Setup Time of SDI Data Input to SCK Edge 100 — ns
73A Tb2b Last Clock Edge of Byte 1 to the 1st Clock Edge
of Byte 2
1.5 TCY + 40 — ns (Note 2)
74 TscH2diL,
TscL2diL
Hold Time of SDI Data Input to SCK Edge 100 — ns
75 TdoR SDO Data Output Rise Time PIC18FXXXX — 25 ns
PIC18LFXXXX 45 ns VDD = 2.0V
76 TdoF SDO Data Output Fall Time — 25 ns
78 TscR SCK Output Rise Time
(Master mode)
PIC18FXXXX — 25 ns
PIC18LFXXXX 45 ns VDD = 2.0V
79 TscF SCK Output Fall Time (Master mode) — 25 ns
80 TscH2doV,
TscL2doV
SDO Data Output Valid after
SCK Edge
PIC18FXXXX — 50 ns
PIC18LFXXXX 100 ns VDD = 2.0V
81 TdoV2scH,
TdoV2scL
SDO Data Output Setup to SCK Edge TCY — ns
Note 1: Requires the use of Parameter #73A.
2: Only if Parameter #71A and #72A are used.
© 2007 Microchip Technology Inc. Preliminary DS39631B-page 351
PIC18F2420/2520/4420/4520
FIGURE 26-14: EXAMPLE SPI SLAVE MODE TIMING (CKE = 0)
TABLE 26-16: EXAMPLE SPI MODE REQUIREMENTS (SLAVE MODE TIMING, CKE = 0)
Param
No.
Symbol Characteristic Min Max Units Conditions
70 TssL2scH,
TssL2scL
SS ↓ to SCK ↓ or SCK ↑ Input TCY — ns
71 TscH SCK Input High Time
(Slave mode)
Continuous 1.25 TCY + 30 — ns
71A Single Byte 40 — ns (Note 1)
72 TscL SCK Input Low Time
(Slave mode)
Continuous 1.25 TCY + 30 — ns
72A Single Byte 40 — ns (Note 1)
73 TdiV2scH,
TdiV2scL
Setup Time of SDI Data Input to SCK Edge 100 — ns
73A Tb2b Last Clock Edge of Byte 1 to the First Clock Edge of Byte 2 1.5 TCY + 40 — ns (Note 2)
74 TscH2diL,
TscL2diL
Hold Time of SDI Data Input to SCK Edge 100 — ns
75 TdoR SDO Data Output Rise Time PIC18FXXXX — 25 ns
PIC18LFXXXX 45 ns VDD = 2.0V
76 TdoF SDO Data Output Fall Time — 25 ns
77 TssH2doZ SS↑ to SDO Output High-Impedance 10 50 ns
78 TscR SCK Output Rise Time (Master mode) PIC18FXXXX — 25 ns
PIC18LFXXXX 45 ns VDD = 2.0V
79 TscF SCK Output Fall Time (Master mode) — 25 ns
80 TscH2doV,
TscL2doV
SDO Data Output Valid after SCK Edge PIC18FXXXX — 50 ns
PIC18LFXXXX 100 ns VDD = 2.0V
83 TscH2ssH,
TscL2ssH
SS ↑ after SCK edge 1.5 TCY + 40 — ns
Note 1: Requires the use of Parameter #73A.
2: Only if Parameter #71A and #72A are used.
SS
SCK
(CKP = 0)
SCK
(CKP = 1)
SDO
70
71 72
73
74
75, 76 77
79 78 80
78 79
SDI
MSb bit 6 - - - - - -1 LSb
MSb In bit 6 - - - -1 LSb In
83
Note: Refer to Figure 26-4 for load conditions.
PIC18F2420/2520/4420/4520
DS39631B-page 352 Preliminary © 2007 Microchip Technology Inc.
FIGURE 26-15: EXAMPLE SPI SLAVE MODE TIMING (CKE = 1)
TABLE 26-17: EXAMPLE SPI SLAVE MODE REQUIREMENTS (CKE = 1)
Param
No.
Symbol Characteristic Min Max Units Conditions
70 TssL2scH,
TssL2scL
SS ↓ to SCK ↓ or SCK ↑ Input TCY — ns
71 TscH SCK Input High Time
(Slave mode)
Continuous 1.25 TCY + 30 — ns
71A Single Byte 40 — ns (Note 1)
72 TscL SCK Input Low Time
(Slave mode)
Continuous 1.25 TCY + 30 — ns
72A Single Byte 40 — ns (Note 1)
73A Tb2b Last Clock Edge of Byte 1 to the First Clock Edge of Byte 2 1.5 TCY + 40 — ns (Note 2)
74 TscH2diL,
TscL2diL
Hold Time of SDI Data Input to SCK Edge 100 — ns
75 TdoR SDO Data Output Rise Time PIC18FXXXX — 25 ns
PIC18LFXXXX 45 ns VDD = 2.0V
76 TdoF SDO Data Output Fall Time — 25 ns
77 TssH2doZ SS↑ to SDO Output High-Impedance 10 50 ns
78 TscR SCK Output Rise Time
(Master mode)
PIC18FXXXX — 25 ns
PIC18LFXXXX — 45 ns VDD = 2.0V
79 TscF SCK Output Fall Time (Master mode) — 25 ns
80 TscH2doV,
TscL2doV
SDO Data Output Valid after SCK
Edge
PIC18FXXXX — 50 ns
PIC18LFXXXX — 100 ns VDD = 2.0V
82 TssL2doV SDO Data Output Valid after SS ↓
Edge
PIC18FXXXX — 50 ns
PIC18LFXXXX — 100 ns VDD = 2.0V
83 TscH2ssH,
TscL2ssH
SS ↑ after SCK Edge 1.5 TCY + 40 — ns
Note 1: Requires the use of Parameter #73A.
2: Only if Parameter #71A and #72A are used.
SS
SCK
(CKP = 0)
SCK
(CKP = 1)
SDO
70
71 72
82
SDI
74
75, 76
MSb bit 6 - - - - - -1 LSb
77
MSb In bit 6 - - - -1 LSb In
80
83
Note: Refer to Figure 26-4 for load conditions.
© 2007 Microchip Technology Inc. Preliminary DS39631B-page 353
PIC18F2420/2520/4420/4520
FIGURE 26-16: I2C BUS START/STOP BITS TIMING
TABLE 26-18: I2C BUS START/STOP BITS REQUIREMENTS (SLAVE MODE)
FIGURE 26-17: I2C BUS DATA TIMING
Note: Refer to Figure 26-4 for load conditions.
91
92
93
SCL
SDA
Start
Condition
Stop
Condition
90
Param.
No.
Symbol Characteristic Min Max Units Conditions
90 TSU:STA Start Condition 100 kHz mode 4700 — ns Only relevant for Repeated
Setup Time 400 kHz mode 600 — Start condition
91 THD:STA Start Condition 100 kHz mode 4000 — ns After this period, the first
Hold Time 400 kHz mode 600 — clock pulse is generated
92 TSU:STO Stop Condition 100 kHz mode 4700 — ns
Setup Time 400 kHz mode 600 —
93 THD:STO Stop Condition 100 kHz mode 4000 — ns
Hold Time 400 kHz mode 600 —
Note: Refer to Figure 26-4 for load conditions.
90
91 92
100
101
103
106 107
109 109
110
102
SCL
SDA
In
SDA
Out
PIC18F2420/2520/4420/4520
DS39631B-page 354 Preliminary © 2007 Microchip Technology Inc.
TABLE 26-19: I2C BUS DATA REQUIREMENTS (SLAVE MODE)
Param.
No.
Symbol Characteristic Min Max Units Conditions
100 THIGH Clock High Time 100 kHz mode 4.0 — μs PIC18FXXXX must operate
at a minimum of 1.5 MHz
400 kHz mode 0.6 — μs PIC18FXXXX must operate
at a minimum of 10 MHz
SSP Module 1.5 TCY —
101 TLOW Clock Low Time 100 kHz mode 4.7 — μs PIC18FXXXX must operate
at a minimum of 1.5 MHz
400 kHz mode 1.3 — μs PIC18FXXXX must operate
at a minimum of 10 MHz
SSP Module 1.5 TCY —
102 TR SDA and SCL Rise
Time
100 kHz mode — 1000 ns
400 kHz mode 20 + 0.1 CB 300 ns CB is specified to be from
10 to 400 pF
103 TF SDA and SCL Fall
Time
100 kHz mode — 300 ns
400 kHz mode 20 + 0.1 CB 300 ns CB is specified to be from
10 to 400 pF
90 TSU:STA Start Condition
Setup Time
100 kHz mode 4.7 — μs Only relevant for Repeated
400 kHz mode 0.6 — μs Start condition
91 THD:STA Start Condition
Hold Time
100 kHz mode 4.0 — μs After this period, the first
400 kHz mode 0.6 — μs clock pulse is generated
106 THD:DAT Data Input Hold
Time
100 kHz mode 0 — ns
400 kHz mode 0 0.9 μs
107 TSU:DAT Data Input Setup
Time
100 kHz mode 250 — ns (Note 2)
400 kHz mode 100 — ns
92 TSU:STO Stop Condition
Setup Time
100 kHz mode 4.7 — μs
400 kHz mode 0.6 — μs
109 TAA Output Valid from
Clock
100 kHz mode — 3500 ns (Note 1)
400 kHz mode — — ns
110 TBUF Bus Free Time 100 kHz mode 4.7 — μs Time the bus must be free
before a new transmission
can start
400 kHz mode 1.3 — μs
D102 CB Bus Capacitive Loading — 400 pF
Note 1: As a transmitter, the device must provide this internal minimum delay time to bridge the undefined region
(min. 300 ns) of the falling edge of SCL to avoid unintended generation of Start or Stop conditions.
2: A fast mode I2C bus device can be used in a standard mode I2C bus system but the requirement,
TSU:DAT ≥ 250 ns, must then be met. This will automatically be the case if the device does not stretch the
LOW period of the SCL signal. If such a device does stretch the LOW period of the SCL signal, it must
output the next data bit to the SDA line, TR max. + TSU:DAT = 1000 + 250 = 1250 ns (according to the
standard mode I2C bus specification), before the SCL line is released.
© 2007 Microchip Technology Inc. Preliminary DS39631B-page 355
PIC18F2420/2520/4420/4520
FIGURE 26-18: MASTER SSP I2C BUS START/STOP BITS TIMING WAVEFORMS
TABLE 26-20: MASTER SSP I2C BUS START/STOP BITS REQUIREMENTS
FIGURE 26-19: MASTER SSP I2C BUS DATA TIMING
Note: Refer to Figure 26-4 for load conditions.
91 93
SCL
SDA
Start
Condition
Stop
Condition
90 92
Param.
No.
Symbol Characteristic Min Max Units Conditions
90 TSU:STA Start Condition 100 kHz mode 2(TOSC)(BRG + 1) — ns Only relevant for
Repeated Start
condition
Setup Time 400 kHz mode 2(TOSC)(BRG + 1) —
1 MHz mode(1) 2(TOSC)(BRG + 1) —
91 THD:STA Start Condition 100 kHz mode 2(TOSC)(BRG + 1) — ns After this period, the
first clock pulse is
generated
Hold Time 400 kHz mode 2(TOSC)(BRG + 1) —
1 MHz mode(1) 2(TOSC)(BRG + 1) —
92 TSU:STO Stop Condition 100 kHz mode 2(TOSC)(BRG + 1) — ns
Setup Time 400 kHz mode 2(TOSC)(BRG + 1) —
1 MHz mode(1) 2(TOSC)(BRG + 1) —
93 THD:STO Stop Condition 100 kHz mode 2(TOSC)(BRG + 1) — ns
Hold Time 400 kHz mode 2(TOSC)(BRG + 1) —
1 MHz mode(1) 2(TOSC)(BRG + 1) —
Note 1: Maximum pin capacitance = 10 pF for all I2C pins.
Note: Refer to Figure 26-4 for load conditions.
90
91 92
100
101
103
106
107
109 109 110
102
SCL
SDA
In
SDA
Out
PIC18F2420/2520/4420/4520
DS39631B-page 356 Preliminary © 2007 Microchip Technology Inc.
TABLE 26-21: MASTER SSP I2C BUS DATA REQUIREMENTS
Param.
No.
Symbol Characteristic Min Max Units Conditions
100 THIGH Clock High Time 100 kHz mode 2(TOSC)(BRG + 1) — ms
400 kHz mode 2(TOSC)(BRG + 1) — ms
1 MHz mode(1) 2(TOSC)(BRG + 1) — ms
101 TLOW Clock Low Time 100 kHz mode 2(TOSC)(BRG + 1) — ms
400 kHz mode 2(TOSC)(BRG + 1) — ms
1 MHz mode(1) 2(TOSC)(BRG + 1) — ms
102 TR SDA and SCL
Rise Time
100 kHz mode — 1000 ns CB is specified to be from
400 kHz mode 20 + 0.1 CB 300 ns 10 to 400 pF
1 MHz mode(1) — 300 ns
103 TF SDA and SCL
Fall Time
100 kHz mode — 300 ns CB is specified to be from
400 kHz mode 20 + 0.1 CB 300 ns 10 to 400 pF
1 MHz mode(1) — 100 ns
90 TSU:STA Start Condition
Setup Time
100 kHz mode 2(TOSC)(BRG + 1) — ms Only relevant for
Repeated Start
condition
400 kHz mode 2(TOSC)(BRG + 1) — ms
1 MHz mode(1) 2(TOSC)(BRG + 1) — ms
91 THD:STA Start Condition
Hold Time
100 kHz mode 2(TOSC)(BRG + 1) — ms After this period, the first
400 kHz mode 2(TOSC)(BRG + 1) — ms clock pulse is generated
1 MHz mode(1) 2(TOSC)(BRG + 1) — ms
106 THD:DAT Data Input
Hold Time
100 kHz mode 0 — ns
400 kHz mode 0 0.9 ms
107 TSU:DAT Data Input
Setup Time
100 kHz mode 250 — ns (Note 2)
400 kHz mode 100 — ns
92 TSU:STO Stop Condition
Setup Time
100 kHz mode 2(TOSC)(BRG + 1) — ms
400 kHz mode 2(TOSC)(BRG + 1) — ms
1 MHz mode(1) 2(TOSC)(BRG + 1) — ms
109 TAA Output Valid
from Clock
100 kHz mode — 3500 ns
400 kHz mode — 1000 ns
1 MHz mode(1) — — ns
110 TBUF Bus Free Time 100 kHz mode 4.7 — ms Time the bus must be free
before a new transmission
can start
400 kHz mode 1.3 — ms
D102 CB Bus Capacitive Loading — 400 pF
Note 1: Maximum pin capacitance = 10 pF for all I2C pins.
2: A fast mode I2C bus device can be used in a standard mode I2C bus system, but parameter 107 ≥ 250 ns
must then be met. This will automatically be the case if the device does not stretch the LOW period of the
SCL signal. If such a device does stretch the LOW period of the SCL signal, it must output the next data bit
to the SDA line, parameter 102 + parameter 107 = 1000 + 250 = 1250 ns (for 100 kHz mode), before the
SCL line is released.
© 2007 Microchip Technology Inc. Preliminary DS39631B-page 357
PIC18F2420/2520/4420/4520
FIGURE 26-20: USART SYNCHRONOUS TRANSMISSION (MASTER/SLAVE) TIMING
TABLE 26-22: USART SYNCHRONOUS TRANSMISSION REQUIREMENTS
FIGURE 26-21: USART SYNCHRONOUS RECEIVE (MASTER/SLAVE) TIMING
TABLE 26-23: USART SYNCHRONOUS RECEIVE REQUIREMENTS
121 121
120
122
RC6/TX/CK
RC7/RX/DT
pin
pin
Note: Refer to Figure 26-4 for load conditions.
Param
No.
Symbol Characteristic Min Max Units Conditions
120 TckH2dtV SYNC XMIT (MASTER & SLAVE)
Clock High to Data Out Valid PIC18FXXXX — 40 ns
PIC18LFXXXX — 100 ns VDD = 2.0V
121 Tckrf Clock Out Rise Time and Fall Time
(Master mode)
PIC18FXXXX — 20 ns
PIC18LFXXXX — 50 ns VDD = 2.0V
122 Tdtrf Data Out Rise Time and Fall Time PIC18FXXXX — 20 ns
PIC18LFXXXX — 50 ns VDD = 2.0V
125
126
RC6/TX/CK
RC7/RX/DT
pin
pin
Note: Refer to Figure 26-4 for load conditions.
Param.
No.
Symbol Characteristic Min Max Units Conditions
125 TdtV2ckl SYNC RCV (MASTER & SLAVE)
Data Hold before CK ↓ (DT hold time) 10 — ns
126 TckL2dtl Data Hold after CK ↓ (DT hold time) 15 — ns
PIC18F2420/2520/4420/4520
DS39631B-page 358 Preliminary © 2007 Microchip Technology Inc.
TABLE 26-24: A/D CONVERTER CHARACTERISTICS: PIC18FX42X/X52X (INDUSTRIAL)
PIC18LF2X1X/4X1X (INDUSTRIAL)
Param
No.
Symbol Characteristic Min Typ Max Units Conditions
A01 NR Resolution — — 10 bit ΔVREF ≥ 3.0V
A03 EIL Integral Linearity Error — — <±1 LSb ΔVREF ≥ 3.0V
A04 EDL Differential Linearity Error — — <±1 LSb ΔVREF ≥ 3.0V
A06 EOFF Offset Error — — <±1 LSb ΔVREF ≥ 3.0V
A07 EGN Gain Error — — <±1 LSb ΔVREF ≥ 3.0V
A10 — Monotonicity Guaranteed(1) — VSS ≤ VAIN ≤ VREF
A20 ΔVREF Reference Voltage Range
(VREFH – VREFL)
1.8
3
—
—
—
—
V
V
VDD < 3.0V
VDD ≥ 3.0V
A21 VREFH Reference Voltage High VSS — VREFH V
A22 VREFL Reference Voltage Low VSS – 0.3V — VDD – 3.0V V
A25 VAIN Analog Input Voltage VREFL — VREFH V
A30 ZAIN Recommended Impedance of
Analog Voltage Source
— — 2.5 kΩ
A50 IREF VREF Input Current(2) —
—
—
—
5
150
μA
μA
During VAIN acquisition.
During A/D conversion
cycle.
Note 1: The A/D conversion result never decreases with an increase in the input voltage and has no missing codes.
2: VREFH current is from RA3/AN3/VREF+ pin or VDD, whichever is selected as the VREFH source.
VREFL current is from RA2/AN2/VREF-/CVREF pin or VSS, whichever is selected as the VREFL source.
© 2007 Microchip Technology Inc. Preliminary DS39631B-page 359
PIC18F2420/2520/4420/4520
FIGURE 26-22: A/D CONVERSION TIMING
TABLE 26-25: A/D CONVERSION REQUIREMENTS
131
130
132
BSF ADCON0, GO
Q4
A/D CLK
A/D DATA
ADRES
ADIF
GO
SAMPLE
OLD_DATA
SAMPLING STOPPED
DONE
NEW_DATA
(Note 2)
9 8 7 2 1 0
Note 1: If the A/D clock source is selected as RC, a time of TCY is added before the A/D clock starts.
This allows the SLEEP instruction to be executed.
2: This is a minimal RC delay (typically 100 ns), which also disconnects the holding capacitor from the analog input.
. . . . . .
TCY
Param
No.
Symbol Characteristic Min Max Units Conditions
130 TAD A/D Clock Period PIC18FXXXX 0.7 25.0(1) μs TOSC based, VREF ≥ 3.0V
PIC18LFXXXX 1.4 25.0(1) μs VDD = 2.0V;
TOSC based, VREF full range
PIC18FXXXX TBD 1 μs A/D RC mode
PIC18LFXXXX TBD 3 μs VDD = 2.0V; A/D RC mode
131 TCNV Conversion Time
(not including acquisition time) (Note 2)
11 12 TAD
132 TACQ Acquisition Time (Note 3) 1.4
TBD
—
—
μs
μs
-40°C to +85°C
0°C ≤ to ≤ +85°C
135 TSWC Switching Time from Convert → Sample — (Note 4)
TBD TDIS Discharge Time 0.2 — μs
Legend: TBD = To Be Determined
Note 1: The time of the A/D clock period is dependent on the device frequency and the TAD clock divider.
2: ADRES register may be read on the following TCY cycle.
3: The time for the holding capacitor to acquire the “New” input voltage when the voltage changes full scale
after the conversion (VDD to VSS or VSS to VDD). The source impedance (RS) on the input channels is 50Ω.
4: On the following cycle of the device clock.
PIC18F2420/2520/4420/4520
DS39631B-page 360 Preliminary © 2007 Microchip Technology Inc.
NOTES:
© 2007 Microchip Technology Inc. Preliminary DS39631B-page 361
PIC18F2420/2520/4420/4520
27.0 DC AND AC
CHARACTERISTICS GRAPHS
AND TABLES
Graphs and tables are not available at this time.
PIC18F2420/2520/4420/4520
DS39631B-page 362 Preliminary © 2007 Microchip Technology Inc.
NOTES:
© 2007 Microchip Technology Inc. Preliminary DS39631B-page 363
PIC18F2420/2520/4420/4520
28.0 PACKAGING INFORMATION
28.1 Package Marking Information
28-Lead PDIP
XXXXXXXXXXXXXXXXX
XXXXXXXXXXXXXXXXX
YYWWNNN
Example
PIC18F2520-I/SP
0710017
28-Lead SOIC
XXXXXXXXXXXXXXXXXXXX
XXXXXXXXXXXXXXXXXXXX
XXXXXXXXXXXXXXXXXXXX
YYWWNNN
Example
PIC18F2520-E/SO
0710017
40-Lead PDIP
XXXXXXXXXXXXXXXXXX
XXXXXXXXXXXXXXXXXX
XXXXXXXXXXXXXXXXXX
YYWWNNN
Example
PIC18F4420-I/P
0710017
Legend: XX...X Customer-specific information
Y Year code (last digit of calendar year)
YY Year code (last 2 digits of calendar year)
WW Week code (week of January 1 is week ‘01’)
NNN Alphanumeric traceability code
Pb-free JEDEC designator for Matte Tin (Sn)
* This package is Pb-free. The Pb-free JEDEC designator ( )
can be found on the outer packaging for this package.
Note: In the event the full Microchip part number cannot be marked on one line, it will
be carried over to the next line, thus limiting the number of available
characters for customer-specific information.
e3
e3
e3
e3
e3
PIC18F2420/2520/4420/4520
DS39631B-page 364 Preliminary © 2007 Microchip Technology Inc.
Package Marking Information (Continued)
44-Lead TQFP
XXXXXXXXXX
XXXXXXXXXX
XXXXXXXXXX
YYWWNNN
Example
PIC18F4420
-I/PT
0710017
XXXXXXXXXX
44-Lead QFN
XXXXXXXXXX
XXXXXXXXXX
YYWWNNN
PIC18F4520
Example
-I/ML
0710017
28-Lead QFN
XXXXXXXX
XXXXXXXX
YYWWNNN
Example
18F2420
-I/ML
0710017
e3
e3
e3
© 2007 Microchip Technology Inc. Preliminary DS39631B-page 365
PIC18F2420/2520/4420/4520
28.2 Package Details
The following sections give the technical details of the packages.
28-Lead Skinny Plastic Dual In-Line (SP) – 300 mil Body [SPDIP]
Notes:
1. Pin 1 visual index feature may vary, but must be located within the hatched area.
2. § Significant Characteristic.
3. Dimensions D and E1 do not include mold flash or protrusions. Mold flash or protrusions shall not exceed .010" per side.
4. Dimensioning and tolerancing per ASME Y14.5M.
BSC: Basic Dimension. Theoretically exact value shown without tolerances.
Note: For the most current package drawings, please see the Microchip Packaging Specification located at
http://www.microchip.com/packaging
Units INCHES
Dimension Limits MIN NOM MAX
Number of Pins N 28
Pitch e .100 BSC
Top to Seating Plane A – – .200
Molded Package Thickness A2 .120 .135 .150
Base to Seating Plane A1 .015 – –
Shoulder to Shoulder Width E .290 .310 .335
Molded Package Width E1 .240 .285 .295
Overall Length D 1.345 1.365 1.400
Tip to Seating Plane L .110 .130 .150
Lead Thickness c .008 .010 .015
Upper Lead Width b1 .040 .050 .070
Lower Lead Width b .014 .018 .022
Overall Row Spacing § eB – – .430
NOTE 1
N
1 2
D
E1
eB
c
E
L
A2
b e
A1 b1
A
3
Microchip Technology Drawing C04-070B
PIC18F2420/2520/4420/4520
DS39631B-page 366 Preliminary © 2007 Microchip Technology Inc.
28-Lead Plastic Small Outline (SO) – Wide, 7.50 mm Body [SOIC]
Notes:
1. Pin 1 visual index feature may vary, but must be located within the hatched area.
2. § Significant Characteristic.
3. Dimensions D and E1 do not include mold flash or protrusions. Mold flash or protrusions shall not exceed 0.15 mm per side.
4. Dimensioning and tolerancing per ASME Y14.5M.
BSC: Basic Dimension. Theoretically exact value shown without tolerances.
REF: Reference Dimension, usually without tolerance, for information purposes only.
Note: For the most current package drawings, please see the Microchip Packaging Specification located at
http://www.microchip.com/packaging
Units MILLMETERS
Dimension Limits MIN NOM MAX
Number of Pins N 28
Pitch e 1.27 BSC
Overall Height A – – 2.65
Molded Package Thickness A2 2.05 – –
Standoff § A1 0.10 – 0.30
Overall Width E 10.30 BSC
Molded Package Width E1 7.50 BSC
Overall Length D 17.90 BSC
Chamfer (optional) h 0.25 – 0.75
Foot Length L 0.40 – 1.27
Footprint L1 1.40 REF
Foot Angle Top φ 0° – 8°
Lead Thickness c 0.18 – 0.33
Lead Width b 0.31 – 0.51
Mold Draft Angle Top α 5° – 15°
Mold Draft Angle Bottom β 5° – 15°
c
h
h
L
L1
A2
A1
A
NOTE 1
1 2 3
b
e
E
E1
D
φ
β
α
N
Microchip Technology Drawing C04-052B
© 2007 Microchip Technology Inc. Preliminary DS39631B-page 367
PIC18F2420/2520/4420/4520
40-Lead Plastic Dual In-Line (P) – 600 mil Body [PDIP]
Notes:
1. Pin 1 visual index feature may vary, but must be located within the hatched area.
2. § Significant Characteristic.
3. Dimensions D and E1 do not include mold flash or protrusions. Mold flash or protrusions shall not exceed .010" per side.
4. Dimensioning and tolerancing per ASME Y14.5M.
BSC: Basic Dimension. Theoretically exact value shown without tolerances.
Note: For the most current package drawings, please see the Microchip Packaging Specification located at
http://www.microchip.com/packaging
Units INCHES
Dimension Limits MIN NOM MAX
Number of Pins N 40
Pitch e .100 BSC
Top to Seating Plane A – – .250
Molded Package Thickness A2 .125 – .195
Base to Seating Plane A1 .015 – –
Shoulder to Shoulder Width E .590 – .625
Molded Package Width E1 .485 – .580
Overall Length D 1.980 – 2.095
Tip to Seating Plane L .115 – .200
Lead Thickness c .008 – .015
Upper Lead Width b1 .030 – .070
Lower Lead Width b .014 – .023
Overall Row Spacing § eB – – .700
N
NOTE 1
E1
D
1 2 3
A
A1
b1
b e
c
eB
E
L
A2
Microchip Technology Drawing C04-016B
PIC18F2420/2520/4420/4520
DS39631B-page 368 Preliminary © 2007 Microchip Technology Inc.
28-Lead Plastic Quad Flat, No Lead Package (ML) – 6x6 mm Body [QFN]
with 0.55 mm Contact Length
Notes:
1. Pin 1 visual index feature may vary, but must be located within the hatched area.
2. Package is saw singulated.
3. Dimensioning and tolerancing per ASME Y14.5M.
BSC: Basic Dimension. Theoretically exact value shown without tolerances.
REF: Reference Dimension, usually without tolerance, for information purposes only.
Note: For the most current package drawings, please see the Microchip Packaging Specification located at
http://www.microchip.com/packaging
Units MILLIMETERS
Dimension Limits MIN NOM MAX
Number of Pins N 28
Pitch e 0.65 BSC
Overall Height A 0.80 0.90 1.00
Standoff A1 0.00 0.02 0.05
Contact Thickness A3 0.20 REF
Overall Width E 6.00 BSC
Exposed Pad Width E2 3.65 3.70 4.20
Overall Length D 6.00 BSC
Exposed Pad Length D2 3.65 3.70 4.20
Contact Width b 0.23 0.30 0.35
Contact Length L 0.50 0.55 0.70
Contact-to-Exposed Pad K 0.20 – –
D
EXPOSED
D2
e
b
K
E2
E
L
N
NOTE 1
1
2 2
1
N
A
A3 A1
TOP VIEW BOTTOM VIEW
PAD
Microchip Technology Drawing C04-105B
© 2007 Microchip Technology Inc. Preliminary DS39631B-page 369
PIC18F2420/2520/4420/4520
44-Lead Plastic Quad Flat, No Lead Package (ML) – 8x8 mm Body [QFN]
Notes:
1. Pin 1 visual index feature may vary, but must be located within the hatched area.
2. Package is saw singulated.
3. Dimensioning and tolerancing per ASME Y14.5M.
BSC: Basic Dimension. Theoretically exact value shown without tolerances.
REF: Reference Dimension, usually without tolerance, for information purposes only.
Note: For the most current package drawings, please see the Microchip Packaging Specification located at
http://www.microchip.com/packaging
Units MILLIMETERS
Dimension Limits MIN NOM MAX
Number of Pins N 44
Pitch e 0.65 BSC
Overall Height A 0.80 0.90 1.00
Standoff A1 0.00 0.02 0.05
Contact Thickness A3 0.20 REF
Overall Width E 8.00 BSC
Exposed Pad Width E2 6.30 6.45 6.80
Overall Length D 8.00 BSC
Exposed Pad Length D2 6.30 6.45 6.80
Contact Width b 0.25 0.30 0.38
Contact Length L 0.30 0.40 0.50
Contact-to-Exposed Pad K 0.20 – –
D
EXPOSED
PAD
D2
e
b
K
L
E2
2
1
N
NOTE 1
2
1
E
N
TOP VIEW BOTTOM VIEW
A3 A1
A
Microchip Technology Drawing C04-103B
PIC18F2420/2520/4420/4520
DS39631B-page 370 Preliminary © 2007 Microchip Technology Inc.
44-Lead Plastic Thin Quad Flatpack (PT) – 10x10x1 mm Body, 2.00 mm Footprint [TQFP]
Notes:
1. Pin 1 visual index feature may vary, but must be located within the hatched area.
2. Chamfers at corners are optional; size may vary.
3. Dimensions D1 and E1 do not include mold flash or protrusions. Mold flash or protrusions shall not exceed 0.25 mm per side.
4. Dimensioning and tolerancing per ASME Y14.5M.
BSC: Basic Dimension. Theoretically exact value shown without tolerances.
REF: Reference Dimension, usually without tolerance, for information purposes only.
Note: For the most current package drawings, please see the Microchip Packaging Specification located at
http://www.microchip.com/packaging
Units MILLIMETERS
Dimension Limits MIN NOM MAX
Number of Leads N 44
Lead Pitch e 0.80 BSC
Overall Height A – – 1.20
Molded Package Thickness A2 0.95 1.00 1.05
Standoff A1 0.05 – 0.15
Foot Length L 0.45 0.60 0.75
Footprint L1 1.00 REF
Foot Angle φ 0° 3.5° 7°
Overall Width E 12.00 BSC
Overall Length D 12.00 BSC
Molded Package Width E1 10.00 BSC
Molded Package Length D1 10.00 BSC
Lead Thickness c 0.09 – 0.20
Lead Width b 0.30 0.37 0.45
Mold Draft Angle Top α 11° 12° 13°
Mold Draft Angle Bottom β 11° 12° 13°
A
E
E1
D
D1
e
b
NOTE 1
NOTE 2
N
1 2 3
c
A1
L
A2
L1
α
φ
β
Microchip Technology Drawing C04-076B
© 2007 Microchip Technology Inc. Preliminary DS39631B-page 371
PIC18F2420/2520/4420/4520
APPENDIX A: REVISION HISTORY
Revision A (June 2004)
Original data sheet for PIC18F2420/2520/4420/4520
devices.
Revision B (January 2007)
This revision includes updates to the packaging
diagrams.
APPENDIX B: DEVICE
DIFFERENCES
The differences between the devices listed in this data
sheet are shown in Table B-1.
TABLE B-1: DEVICE DIFFERENCES
Features PIC18F2420 PIC18F2520 PIC18F4420 PIC18F4520
Program Memory (Bytes) 16384 32768 16384 32768
Program Memory (Instructions) 8192 16384 8192 16384
Interrupt Sources 19 19 20 20
I/O Ports Ports A, B, C, (E) Ports A, B, C, (E) Ports A, B, C, D, E Ports A, B, C, D, E
Capture/Compare/PWM Modules 2 2 1 1
Enhanced
Capture/Compare/PWM Modules
0 0 1 1
Parallel Communications (PSP) No No Yes Yes
10-bit Analog-to-Digital Module 10 input channels 10 input channels 13 input channels 13 input channels
Packages 28-pin PDIP
28-pin SOIC
28-pin QFN
28-pin PDIP
28-pin SOIC
28-pin QFN
40-pin PDIP
44-pin TQFP
44-pin QFN
40-pin PDIP
44-pin TQFP
44-pin QFN
PIC18F2420/2520/4420/4520
DS39631B-page 372 Preliminary © 2007 Microchip Technology Inc.
APPENDIX C: CONVERSION
CONSIDERATIONS
This appendix discusses the considerations for
converting from previous versions of a device to the
ones listed in this data sheet. Typically, these changes
are due to the differences in the process technology
used. An example of this type of conversion is from a
PIC16C74A to a PIC16C74B.
Not Applicable
APPENDIX D: MIGRATION FROM
BASELINE TO
ENHANCED DEVICES
This section discusses how to migrate from a Baseline
device (i.e., PIC16C5X) to an Enhanced MCU device
(i.e., PIC18FXXX).
The following are the list of modifications over the
PIC16C5X microcontroller family:
Not Currently Available
© 2007 Microchip Technology Inc. Preliminary DS39631B-page 373
PIC18F2420/2520/4420/4520
APPENDIX E: MIGRATION FROM
MID-RANGE TO
ENHANCED DEVICES
A detailed discussion of the differences between the
mid-range MCU devices (i.e., PIC16CXXX) and the
enhanced devices (i.e., PIC18FXXX) is provided in
AN716, “Migrating Designs from PIC16C74A/74B to
PIC18C442”. The changes discussed, while device
specific, are generally applicable to all mid-range to
enhanced device migrations.
This Application Note is available as Literature Number
DS00716.
APPENDIX F: MIGRATION FROM
HIGH-END TO
ENHANCED DEVICES
A detailed discussion of the migration pathway and differences
between the high-end MCU devices (i.e.,
PIC17CXXX) and the enhanced devices (i.e.,
PIC18FXXX) is provided in AN726, “PIC17CXXX to
PIC18CXXX Migration”. This Application Note is
available as Literature Number DS00726.
PIC18F2420/2520/4420/4520
DS39631B-page 374 Preliminary © 2007 Microchip Technology Inc.
NOTES:
© 2007 Microchip Technology Inc. Preliminary DS39631B-page 375
PIC18F2420/2520/4420/4520
INDEX
A
A/D ................................................................................... 223
A/D Converter Interrupt, Configuring ....................... 227
Acquisition Requirements ........................................ 228
ADCON0 Register .................................................... 223
ADCON1 Register .................................................... 223
ADCON2 Register .................................................... 223
ADRESH Register ............................................ 223, 226
ADRESL Register .................................................... 223
Analog Port Pins, Configuring .................................. 230
Associated Registers ............................................... 232
Calculating the Minimum Required
Acquisition Time .............................................. 228
Configuring the Module ............................................ 227
Conversion Clock (TAD) ........................................... 229
Conversion Status (GO/DONE Bit) .......................... 226
Conversions ............................................................. 231
Converter Characteristics ........................................ 358
Discharge ................................................................. 231
Operation in Power Managed Modes ...................... 230
Selecting and Configuring Acquisition Time ............ 229
Special Event Trigger (CCP) .................................... 232
Special Event Trigger (ECCP) ................................. 148
Use of the CCP2 Trigger .......................................... 232
Absolute Maximum Ratings ............................................. 323
AC (Timing) Characteristics ............................................. 340
Load Conditions for Device
Timing Specifications ....................................... 341
Parameter Symbology ............................................. 340
Temperature and Voltage Specifications ................. 341
Timing Conditions .................................................... 341
AC Characteristics
Internal RC Accuracy ............................................... 343
Access Bank
Mapping with Indexed Literal Offset Mode ................. 72
ACKSTAT ........................................................................ 191
ACKSTAT Status Flag ..................................................... 191
ADCON0 Register ............................................................ 223
GO/DONE Bit ........................................................... 226
ADCON1 Register ............................................................ 223
ADCON2 Register ............................................................ 223
ADDFSR .......................................................................... 310
ADDLW ............................................................................ 273
ADDULNK ........................................................................ 310
ADDWF ............................................................................ 273
ADDWFC ......................................................................... 274
ADRESH Register ............................................................ 223
ADRESL Register .................................................... 223, 226
Analog-to-Digital Converter. See A/D.
ANDLW ............................................................................ 274
ANDWF ............................................................................ 275
Assembler
MPASM Assembler .................................................. 317
Auto-Wake-up on Sync Break Character ......................... 214
B
Bank Select Register (BSR) ............................................... 59
Baud Rate Generator ....................................................... 187
BC .................................................................................... 275
BCF .................................................................................. 276
BF .................................................................................... 191
BF Status Flag ................................................................. 191
Block Diagrams
A/D ........................................................................... 226
Analog Input Model .................................................. 227
Baud Rate Generator .............................................. 187
Capture Mode Operation ......................................... 141
Comparator Analog Input Model .............................. 237
Comparator I/O Operating Modes ........................... 234
Comparator Output .................................................. 236
Comparator Voltage Reference ............................... 240
Compare Mode Operation ....................................... 142
Device Clock .............................................................. 28
Enhanced PWM ....................................................... 149
EUSART Receive .................................................... 213
EUSART Transmit ................................................... 211
External Power-on Reset Circuit
(Slow VDD Power-up) ........................................ 43
Fail-Safe Clock Monitor (FSCM) .............................. 261
Generic I/O Port ....................................................... 105
High/Low-Voltage Detect with External Input .......... 244
Interrupt Logic ............................................................ 92
MSSP (I2C Master Mode) ........................................ 185
MSSP (I2C Mode) .................................................... 170
MSSP (SPI Mode) ................................................... 161
On-Chip Reset Circuit ................................................ 41
PIC18F2420/2520 ..................................................... 10
PIC18F4420/4520 ..................................................... 11
PLL (HS Mode) .......................................................... 25
PORTD and PORTE (Parallel Slave Port) ............... 120
PWM Operation (Simplified) .................................... 144
Reads from Flash Program Memory ......................... 77
Single Comparator ................................................... 235
Table Read Operation ............................................... 73
Table Write Operation ............................................... 74
Table Writes to Flash Program Memory .................... 79
Timer0 in 16-Bit Mode ............................................. 124
Timer0 in 8-Bit Mode ............................................... 124
Timer1 ..................................................................... 128
Timer1 (16-Bit Read/Write Mode) ............................ 128
Timer2 ..................................................................... 134
Timer3 ..................................................................... 136
Timer3 (16-Bit Read/Write Mode) ............................ 136
Voltage Reference Output Buffer Example ............. 241
Watchdog Timer ...................................................... 258
BN .................................................................................... 276
BNC ................................................................................. 277
BNN ................................................................................. 277
BNOV .............................................................................. 278
BNZ ................................................................................. 278
BOR. See Brown-out Reset.
BOV ................................................................................. 281
BRA ................................................................................. 279
Break Character (12-Bit) Transmit and Receive .............. 216
BRG. See Baud Rate Generator.
Brown-out Reset (BOR) ..................................................... 44
Detecting ................................................................... 44
Disabling in Sleep Mode ............................................ 44
Software Enabled ...................................................... 44
BSF .................................................................................. 279
BTFSC ............................................................................. 280
BTFSS ............................................................................. 280
BTG ................................................................................. 281
BZ .................................................................................... 282
PIC18F2420/2520/4420/4520
DS39631B-page 376 Preliminary © 2007 Microchip Technology Inc.
C
C Compilers
MPLAB C17 ............................................................. 318
MPLAB C18 ............................................................. 318
MPLAB C30 ............................................................. 318
CALL ................................................................................ 282
CALLW ............................................................................. 311
Capture (CCP Module) ..................................................... 141
Associated Registers ...............................................143
CCP Pin Configuration ............................................. 141
CCPRxH:CCPRxL Registers ................................... 141
Prescaler .................................................................. 141
Software Interrupt .................................................... 141
Timer1/Timer3 Mode Selection ................................ 141
Capture (ECCP Module) .................................................. 148
Capture/Compare/PWM (CCP) ........................................ 139
Capture Mode. See Capture.
CCP Mode and Timer Resources ............................140
CCPRxH Register .................................................... 140
CCPRxL Register ..................................................... 140
Compare Mode. See Compare.
Interaction of Two CCP Modules ............................. 140
Module Configuration ...............................................140
Clock Sources .................................................................... 28
Selecting the 31 kHz Source ...................................... 29
Selection Using OSCCON Register ........................... 29
CLRF ................................................................................ 283
CLRWDT .......................................................................... 283
Code Examples
16 x 16 Signed Multiply Routine ................................90
16 x 16 Unsigned Multiply Routine ............................90
8 x 8 Signed Multiply Routine .................................... 89
8 x 8 Unsigned Multiply Routine ................................89
Changing Between Capture Prescalers ................... 141
Computed GOTO Using an Offset Value ................... 56
Data EEPROM Read .................................................85
Data EEPROM Refresh Routine ................................86
Data EEPROM Write .................................................85
Erasing a Flash Program Memory Row ..................... 78
Fast Register Stack .................................................... 56
How to Clear RAM (Bank 1) Using
Indirect Addressing ............................................ 68
Implementing a Real-Time Clock Using
a Timer1 Interrupt Service ............................... 131
Initializing PORTA .................................................... 105
Initializing PORTB .................................................... 108
Initializing PORTC .................................................... 111
Initializing PORTD .................................................... 114
Initializing PORTE .................................................... 117
Loading the SSPBUF (SSPSR) Register ................. 164
Reading a Flash Program Memory Word .................. 77
Saving Status, WREG and
BSR Registers in RAM ..................................... 103
Writing to Flash Program Memory ....................... 80–81
Code Protection ............................................................... 249
COMF ............................................................................... 284
Comparator ......................................................................233
Analog Input Connection Considerations ................. 237
Associated Registers ...............................................237
Configuration ............................................................ 234
Effects of a Reset ..................................................... 236
Interrupts .................................................................. 236
Operation ................................................................. 235
Operation During Sleep ........................................... 236
Outputs ....................................................................235
Reference ................................................................ 235
External Signal ................................................ 235
Internal Signal .................................................. 235
Response Time ........................................................ 235
Comparator Specifications ............................................... 338
Comparator Voltage Reference ....................................... 239
Accuracy and Error .................................................. 240
Associated Registers ............................................... 241
Configuring .............................................................. 239
Connection Considerations ...................................... 240
Effects of a Reset .................................................... 240
Operation During Sleep ........................................... 240
Compare (CCP Module) .................................................. 142
Associated Registers ............................................... 143
CCPRx Register ...................................................... 142
Pin Configuration ..................................................... 142
Software Interrupt .................................................... 142
Special Event Trigger .............................. 137, 142, 232
Timer1/Timer3 Mode Selection ................................ 142
Compare (ECCP Module) ................................................ 148
Special Event Trigger .............................................. 148
Computed GOTO ............................................................... 56
Configuration Bits ............................................................ 249
Configuration Register Protection .................................... 266
Context Saving During Interrupts ..................................... 103
Conversion Considerations .............................................. 372
CPFSEQ .......................................................................... 284
CPFSGT .......................................................................... 285
CPFSLT ........................................................................... 285
Crystal Oscillator/Ceramic Resonator ................................ 23
D
Data Addressing Modes .................................................... 68
Comparing Addressing Modes with the
Extended Instruction Set Enabled ..................... 71
Direct ......................................................................... 68
Indexed Literal Offset ................................................ 70
Instructions Affected .......................................... 70
Indirect ....................................................................... 68
Inherent and Literal .................................................... 68
Data EEPROM
Code Protection ....................................................... 266
Data EEPROM Memory ..................................................... 83
Associated Registers ................................................. 87
EEADR Register ........................................................ 83
EECON1 and EECON2 Registers ............................. 83
Operation During Code-Protect ................................. 86
Protection Against Spurious Write ............................. 86
Reading ..................................................................... 85
Using ......................................................................... 86
Write Verify ................................................................ 85
Writing ....................................................................... 85
Data Memory ..................................................................... 59
Access Bank .............................................................. 62
and the Extended Instruction Set .............................. 70
Bank Select Register (BSR) ...................................... 59
General Purpose Registers ....................................... 62
Map for PIC18F2420/4420 ........................................ 60
Map for PIC18F2520/4520 ........................................ 61
Special Function Registers ........................................ 63
DAW ................................................................................ 286
DC and AC Characteristics
Graphs and Tables .................................................. 361
© 2007 Microchip Technology Inc. Preliminary DS39631B-page 377
PIC18F2420/2520/4420/4520
DC Characteristics ........................................................... 335
Power-Down and Supply Current ............................ 326
Supply Voltage ......................................................... 325
DCFSNZ .......................................................................... 287
DECF ............................................................................... 286
DECFSZ ........................................................................... 287
Demonstration Boards
PICDEM 1 ................................................................ 320
PICDEM 17 .............................................................. 321
PICDEM 18R ........................................................... 321
PICDEM 2 Plus ........................................................ 320
PICDEM 3 ................................................................ 320
PICDEM 4 ................................................................ 320
PICDEM LIN ............................................................ 321
PICDEM USB ........................................................... 321
PICDEM.net Internet/Ethernet ................................. 320
Development Support ...................................................... 317
Device Differences ........................................................... 371
Device Overview .................................................................. 7
Details on Individual Family Members ......................... 8
Features (table) ............................................................ 9
New Core Features ...................................................... 7
Other Special Features ................................................ 8
Device Reset Timers .......................................................... 45
Oscillator Start-up Timer (OST) ................................. 45
PLL Lock Time-out ..................................................... 45
Power-up Timer (PWRT) ........................................... 45
Time-out Sequence .................................................... 45
Direct Addressing ............................................................... 69
E
Effect on Standard PIC Instructions ................................. 314
Effects of Power Managed Modes on
Various Clock Sources ............................................... 31
Electrical Characteristics .................................................. 323
Enhanced Capture/Compare/PWM (ECCP) .................... 147
Associated Registers ............................................... 160
Capture and Compare Modes .................................. 148
Capture Mode. See Capture (ECCP Module).
Outputs and Configuration ....................................... 148
Pin Configurations for ECCP1 ................................. 148
PWM Mode. See PWM (ECCP Module).
Standard PWM Mode ............................................... 148
Timer Resources ...................................................... 148
Enhanced PWM Mode. See PWM (ECCP Module).
Enhanced Universal Synchronous Asynchronous
Receiver Transmitter (EUSART). See EUSART.
Equations
A/D Acquisition Time ................................................ 228
A/D Minimum Charging Time ................................... 228
Errata ................................................................................... 6
EUSART
Asynchronous Mode ................................................ 211
12-Bit Break Transmit and Receive ................. 216
Associated Registers, Receive ........................ 214
Associated Registers, Transmit ....................... 212
Auto-Wake-up on Sync Break ......................... 214
Receiver ........................................................... 213
Setting up 9-Bit Mode with
Address Detect ........................................ 213
Transmitter ....................................................... 211
Baud Rate Generator
Operation in Power Managed Mode ................ 205
Baud Rate Generator (BRG) ................................... 205
Associated Registers ....................................... 206
Auto-Baud Rate Detect .................................... 209
Baud Rate Error, Calculating ........................... 206
Baud Rates, Asynchronous Modes ................. 207
High Baud Rate Select (BRGH Bit) ................. 205
Sampling ......................................................... 205
Synchronous Master Mode ...................................... 217
Associated Registers, Receive ........................ 219
Associated Registers, Transmit ....................... 218
Reception ........................................................ 219
Transmission ................................................... 217
Synchronous Slave Mode ........................................ 220
Associated Registers, Receive ........................ 221
Associated Registers, Transmit ....................... 220
Reception ........................................................ 221
Transmission ................................................... 220
Evaluation and Programming Tools ................................. 321
Extended Instruction Set
ADDFSR .................................................................. 310
ADDULNK ............................................................... 310
and Using MPLAB Tools ......................................... 316
CALLW .................................................................... 311
Considerations for Use ............................................ 314
MOVSF .................................................................... 311
MOVSS .................................................................... 312
PUSHL ..................................................................... 312
SUBFSR .................................................................. 313
SUBULNK ................................................................ 313
Syntax ...................................................................... 309
External Clock Input ........................................................... 24
F
Fail-Safe Clock Monitor ........................................... 249, 261
Exiting Operation ..................................................... 261
Interrupts in Power Managed Modes ....................... 262
POR or Wake from Sleep ........................................ 262
WDT During Oscillator Failure ................................. 261
Fast Register Stack ........................................................... 56
Firmware Instructions ...................................................... 267
Flash Program Memory ..................................................... 73
Associated Registers ................................................. 81
Control Registers ....................................................... 74
EECON1 and EECON2 ..................................... 74
TABLAT (Table Latch) Register ........................ 76
TBLPTR (Table Pointer) Register ...................... 76
Erase Sequence ........................................................ 78
Erasing ...................................................................... 78
Operation During Code-Protect ................................. 81
Reading ..................................................................... 77
Table Pointer
Boundaries Based on Operation ....................... 76
Table Pointer Boundaries .......................................... 76
Table Reads and Table Writes .................................. 73
Write Sequence ......................................................... 79
Writing To .................................................................. 79
Protection Against Spurious Writes ................... 81
Unexpected Termination ................................... 81
Write Verify ........................................................ 81
FSCM. See Fail-Safe Clock Monitor.
G
General Call Address Support ......................................... 184
GOTO .............................................................................. 288
PIC18F2420/2520/4420/4520
DS39631B-page 378 Preliminary © 2007 Microchip Technology Inc.
H
Hardware Multiplier ............................................................ 89
Introduction ................................................................ 89
Operation ................................................................... 89
Performance Comparison .......................................... 89
High/Low-Voltage Detect .................................................243
Applications .............................................................. 246
Associated Registers ...............................................247
Characteristics ......................................................... 339
Current Consumption ...............................................245
Effects of a Reset ..................................................... 247
Operation ................................................................. 244
During Sleep .................................................... 247
Setup ........................................................................245
Start-up Time ........................................................... 245
Typical Application ...................................................246
HLVD. See High/Low-Voltage Detect.
I
I/O Ports ........................................................................... 105
I2C Mode (MSSP)
Acknowledge Sequence Timing ............................... 194
Baud Rate Generator ...............................................187
Bus Collision
During a Repeated Start Condition .................. 198
During a Stop Condition ................................... 199
Clock Arbitration ....................................................... 188
Clock Stretching ....................................................... 180
10-Bit Slave Receive Mode (SEN = 1) ............. 180
10-Bit Slave Transmit Mode ............................. 180
7-Bit Slave Receive Mode (SEN = 1) ............... 180
7-Bit Slave Transmit Mode ............................... 180
Clock Synchronization and the CKP Bit (SEN = 1) .. 181
Effects of a Reset ..................................................... 195
General Call Address Support ................................. 184
I2C Clock Rate w/BRG ............................................. 187
Master Mode ............................................................ 185
Operation ......................................................... 186
Reception ......................................................... 191
Repeated Start Condition Timing ..................... 190
Start Condition Timing ..................................... 189
Transmission .................................................... 191
Multi-Master Communication, Bus Collision
and Arbitration .................................................. 195
Multi-Master Mode ...................................................195
Operation ................................................................. 174
Read/Write Bit Information (R/W Bit) ............... 174, 175
Registers .................................................................. 170
Serial Clock (RC3/SCK/SCL) ................................... 175
Slave Mode .............................................................. 174
Addressing ....................................................... 174
Reception ......................................................... 175
Transmission .................................................... 175
Sleep Operation ....................................................... 195
Stop Condition Timing .............................................. 194
ID Locations ............................................................. 249, 266
INCF ................................................................................. 288
INCFSZ ............................................................................ 289
In-Circuit Debugger .......................................................... 266
In-Circuit Serial Programming (ICSP) ...................... 249, 266
Indexed Literal Offset Addressing
and Standard PIC18 Instructions ............................. 314
Indexed Literal Offset Mode ............................................. 314
Indirect Addressing ............................................................ 69
INFSNZ ............................................................................ 289
Initialization Conditions for all Registers ...................... 49–52
Instruction Cycle ................................................................ 57
Clocking Scheme ....................................................... 57
Instruction Flow/Pipelining ................................................. 57
Instruction Set .................................................................. 267
ADDLW .................................................................... 273
ADDWF .................................................................... 273
ADDWF (Indexed Literal Offset Mode) .................... 315
ADDWFC ................................................................. 274
ANDLW .................................................................... 274
ANDWF .................................................................... 275
BC ............................................................................ 275
BCF ......................................................................... 276
BN ............................................................................ 276
BNC ......................................................................... 277
BNN ......................................................................... 277
BNOV ...................................................................... 278
BNZ ......................................................................... 278
BOV ......................................................................... 281
BRA ......................................................................... 279
BSF .......................................................................... 279
BSF (Indexed Literal Offset Mode) .......................... 315
BTFSC ..................................................................... 280
BTFSS ..................................................................... 280
BTG ......................................................................... 281
BZ ............................................................................ 282
CALL ........................................................................ 282
CLRF ....................................................................... 283
CLRWDT ................................................................. 283
COMF ...................................................................... 284
CPFSEQ .................................................................. 284
CPFSGT .................................................................. 285
CPFSLT ................................................................... 285
DAW ........................................................................ 286
DCFSNZ .................................................................. 287
DECF ....................................................................... 286
DECFSZ .................................................................. 287
Extended Instruction Set ......................................... 309
General Format ........................................................ 269
GOTO ...................................................................... 288
INCF ........................................................................ 288
INCFSZ .................................................................... 289
INFSNZ .................................................................... 289
IORLW ..................................................................... 290
IORWF ..................................................................... 290
LFSR ....................................................................... 291
MOVF ...................................................................... 291
MOVFF .................................................................... 292
MOVLB .................................................................... 292
MOVLW ................................................................... 293
MOVWF ................................................................... 293
MULLW .................................................................... 294
MULWF .................................................................... 294
NEGF ....................................................................... 295
NOP ......................................................................... 295
Opcode Field Descriptions ....................................... 268
POP ......................................................................... 296
PUSH ....................................................................... 296
RCALL ..................................................................... 297
RESET ..................................................................... 297
RETFIE .................................................................... 298
RETLW .................................................................... 298
RETURN .................................................................. 299
RLCF ....................................................................... 299
RLNCF ..................................................................... 300
© 2007 Microchip Technology Inc. Preliminary DS39631B-page 379
PIC18F2420/2520/4420/4520
RRCF ....................................................................... 300
RRNCF .................................................................... 301
SETF ........................................................................ 301
SETF (Indexed Literal Offset Mode) ........................ 315
SLEEP ..................................................................... 302
SUBFWB .................................................................. 302
SUBLW .................................................................... 303
SUBWF .................................................................... 303
SUBWFB .................................................................. 304
SWAPF .................................................................... 304
TBLRD ..................................................................... 305
TBLWT ..................................................................... 306
TSTFSZ ................................................................... 307
XORLW .................................................................... 307
XORWF .................................................................... 308
INTCON Registers ....................................................... 93–95
Inter-Integrated Circuit. See I2C.
Internal Oscillator Block ..................................................... 26
Adjustment ................................................................. 26
INTIO Modes .............................................................. 26
INTOSC Frequency Drift ............................................ 26
INTOSC Output Frequency ........................................ 26
OSCTUNE Register ................................................... 26
PLL in INTOSC Modes .............................................. 26
Internal RC Oscillator
Use with WDT .......................................................... 258
Interrupt Sources ............................................................. 249
A/D Conversion Complete ....................................... 227
Capture Complete (CCP) ......................................... 141
Compare Complete (CCP) ....................................... 142
Interrupt-on-Change (RB7:RB4) .............................. 108
INTn Pin ................................................................... 103
PORTB, Interrupt-on-Change .................................. 103
TMR0 ....................................................................... 103
TMR0 Overflow ........................................................ 125
TMR1 Overflow ........................................................ 127
TMR2 to PR2 Match (PWM) ............................ 144, 149
TMR3 Overflow ................................................ 135, 137
Interrupts ............................................................................ 91
Interrupts, Flag Bits
Interrupt-on-Change (RB7:RB4) Flag
(RBIF Bit) ......................................................... 108
INTOSC, INTRC. See Internal Oscillator Block.
IORLW ............................................................................. 290
IORWF ............................................................................. 290
IPR Registers ................................................................... 100
L
LFSR ................................................................................ 291
Low-Voltage ICSP Programming.
See Single-Supply ICSP Programming
M
Master Clear (MCLR) ......................................................... 43
Master Synchronous Serial Port (MSSP). See MSSP.
Memory Organization ......................................................... 53
Data Memory ............................................................. 59
Program Memory ....................................................... 53
Memory Programming Requirements .............................. 337
Migration from Baseline to Enhanced Devices ................ 372
Migration from High-End to Enhanced Devices ............... 373
Migration from Mid-Range to Enhanced Devices ............ 373
MOVF ............................................................................... 291
MOVFF ............................................................................ 292
MOVLB ............................................................................ 292
MOVLW ........................................................................... 293
MOVSF ............................................................................ 311
MOVSS ............................................................................ 312
MOVWF ........................................................................... 293
MPLAB ASM30 Assembler, Linker, Librarian .................. 318
MPLAB ICD 2 In-Circuit Debugger .................................. 319
MPLAB ICE 2000 High-Performance
Universal In-Circuit Emulator ................................... 319
MPLAB ICE 4000 High-Performance
Universal In-Circuit Emulator ................................... 319
MPLAB Integrated Development
Environment Software ............................................. 317
MPLAB PM3 Device Programmer ................................... 319
MPLINK Object Linker/MPLIB Object Librarian ............... 318
MSSP
ACK Pulse ....................................................... 174, 175
Control Registers (general) ..................................... 161
I2C Mode. See I2C Mode.
Module Overview ..................................................... 161
SPI Master/Slave Connection .................................. 165
SPI Mode. See SPI Mode.
SSPBUF Register .................................................... 166
SSPSR Register ...................................................... 166
MULLW ............................................................................ 294
MULWF ............................................................................ 294
N
NEGF ............................................................................... 295
NOP ................................................................................. 295
O
Oscillator Configuration ..................................................... 23
EC .............................................................................. 23
ECIO .......................................................................... 23
HS .............................................................................. 23
HSPLL ....................................................................... 23
Internal Oscillator Block ............................................. 26
INTIO1 ....................................................................... 23
INTIO2 ....................................................................... 23
LP .............................................................................. 23
RC ............................................................................. 23
RCIO .......................................................................... 23
XT .............................................................................. 23
Oscillator Selection .......................................................... 249
Oscillator Start-up Timer (OST) ................................... 31, 45
Oscillator Switching ........................................................... 28
Oscillator Transitions ......................................................... 29
Oscillator, Timer1 ..................................................... 127, 137
Oscillator, Timer3 ............................................................. 135
P
Packaging Information ..................................................... 363
Marking .................................................................... 363
Parallel Slave Port (PSP) ......................................... 114, 120
Associated Registers ............................................... 121
CS (Chip Select) ...................................................... 120
PORTD .................................................................... 120
RD (Read Input) ...................................................... 120
Select (PSPMODE Bit) .................................... 114, 120
WR (Write Input) ...................................................... 120
PICkit 1 Flash Starter Kit ................................................. 321
PICSTART Plus Development Programmer .................... 320
PIE Registers ..................................................................... 98
PIC18F2420/2520/4420/4520
DS39631B-page 380 Preliminary © 2007 Microchip Technology Inc.
Pin Functions
MCLR/VPP/RE3 .................................................... 12, 16
OSC1/CLKI/RA7 .................................................. 12, 16
OSC2/CLKO/RA6 ................................................ 12, 16
RA0/AN0 .............................................................. 13, 17
RA1/AN1 .............................................................. 13, 17
RA2/AN2/VREF-/CVREF ........................................ 13, 17
RA3/AN3/VREF+ ................................................... 13, 17
RA4/T0CKI/C1OUT .............................................. 13, 17
RA5/AN4/SS/HLVDIN/C2OUT ............................. 13, 17
RB0/INT0/FLT0/AN12 .......................................... 14, 18
RB1/INT1/AN10 ................................................... 14, 18
RB2/INT2/AN8 ..................................................... 14, 18
RB3/AN9/CCP2 ................................................... 14, 18
RB4/KBI0/AN11 ................................................... 14, 18
RB5/KBI1/PGM .................................................... 14, 18
RB6/KBI2/PGC .................................................... 14, 18
RB7/KBI3/PGD .................................................... 14, 18
RC0/T1OSO/T13CKI ........................................... 15, 19
RC1/T1OSI/CCP2 ................................................ 15, 19
RC2/CCP1 ................................................................. 15
RC2/CCP1/P1A ......................................................... 19
RC3/SCK/SCL ..................................................... 15, 19
RC4/SDI/SDA ...................................................... 15, 19
RC5/SDO ............................................................. 15, 19
RC6/TX/CK .......................................................... 15, 19
RC7/RX/DT .......................................................... 15, 19
RD0/PSP0 .................................................................. 20
RD1/PSP1 .................................................................. 20
RD2/PSP2 .................................................................. 20
RD3/PSP3 .................................................................. 20
RD4/PSP4 .................................................................. 20
RD5/PSP5/P1B .......................................................... 20
RD6/PSP6/P1C .......................................................... 20
RD7/PSP7/P1D .......................................................... 20
RE0/RD/AN5 .............................................................. 21
RE1/WR/AN6 ............................................................. 21
RE2/CS/AN7 .............................................................. 21
VDD ....................................................................... 15, 21
VSS ....................................................................... 15, 21
Pinout I/O Descriptions
PIC18F2420/2520 ...................................................... 12
PIC18F4420/4520 ...................................................... 16
PIR Registers ..................................................................... 96
PLL Frequency Multiplier ...................................................25
HSPLL Oscillator Mode .............................................. 25
Use with INTOSC ....................................................... 25
POP .................................................................................. 296
POR. See Power-on Reset.
PORTA
Associated Registers ...............................................107
LATA Register .......................................................... 105
PORTA Register ...................................................... 105
TRISA Register ........................................................ 105
PORTB
Associated Registers ...............................................110
LATB Register .......................................................... 108
PORTB Register ...................................................... 108
RB7:RB4 Interrupt-on-Change Flag
(RBIF Bit) ......................................................... 108
TRISB Register ........................................................ 108
PORTC
Associated Registers ............................................... 113
LATC Register ......................................................... 111
PORTC Register ...................................................... 111
RC3/SCK/SCL Pin ................................................... 175
TRISC Register ........................................................ 111
PORTD
Associated Registers ............................................... 116
LATD Register ......................................................... 114
Parallel Slave Port (PSP) Function .......................... 114
PORTD Register ...................................................... 114
TRISD Register ........................................................ 114
PORTE
Associated Registers ............................................... 119
LATE Register ......................................................... 117
PORTE Register ...................................................... 117
PSP Mode Select (PSPMODE Bit) .......................... 114
TRISE Register ........................................................ 117
Power Managed Modes ..................................................... 33
and A/D Operation ................................................... 230
and EUSART Operation .......................................... 205
and Multiple Sleep Commands .................................. 34
and PWM Operation ................................................ 159
and SPI Operation ................................................... 169
Clock Transitions and Status Indicators .................... 34
Effects on Clock Sources ........................................... 31
Entering ..................................................................... 33
Exiting Idle and Sleep Modes .................................... 39
by Interrupt ........................................................ 39
by Reset ............................................................ 39
by WDT Time-out .............................................. 39
Without a Start-up Delay ................................... 40
Idle Modes ................................................................. 37
PRI_IDLE ........................................................... 38
RC_IDLE ........................................................... 39
SEC_IDLE ......................................................... 38
Run Modes ................................................................ 34
PRI_RUN ........................................................... 34
RC_RUN ............................................................ 35
SEC_RUN ......................................................... 34
Selecting .................................................................... 33
Sleep Mode ............................................................... 37
Summary (table) ........................................................ 33
Power-on Reset (POR) ...................................................... 43
Power-up Timer (PWRT) ........................................... 45
Time-out Sequence ................................................... 45
Power-up Delays ............................................................... 31
Power-up Timer (PWRT) ................................................... 31
Prescaler
Timer2 ..................................................................... 150
Prescaler, Timer0 ............................................................ 125
Prescaler, Timer2 ............................................................ 145
PRI_IDLE Mode ................................................................. 38
PRI_RUN Mode ................................................................. 34
PRO MATE II Universal Device Programmer .................. 319
Program Counter ............................................................... 54
PCL, PCH and PCU Registers .................................. 54
PCLATH and PCLATU Registers .............................. 54
© 2007 Microchip Technology Inc. Preliminary DS39631B-page 381
PIC18F2420/2520/4420/4520
Program Memory
and Extended Instruction Set ..................................... 72
Code Protection ....................................................... 264
Instructions ................................................................. 58
Two-Word .......................................................... 58
Interrupt Vector .......................................................... 53
Look-up Tables .......................................................... 56
Map and Stack (diagram) ........................................... 53
Reset Vector .............................................................. 53
Program Verification and Code Protection ....................... 263
Associated Registers ............................................... 263
Programming, Device Instructions ................................... 267
PSP. See Parallel Slave Port.
Pulse-Width Modulation. See PWM (CCP Module)
and PWM (ECCP Module).
PUSH ............................................................................... 296
PUSH and POP Instructions .............................................. 55
PUSHL ............................................................................. 312
PWM (CCP Module)
Associated Registers ............................................... 146
Auto-Shutdown (CCP1 only) .................................... 145
CCPR1H:CCPR1L Registers ................................... 149
Duty Cycle ........................................................ 144, 150
Example Frequencies/Resolutions .................. 145, 150
Period ............................................................... 144, 149
Setup for PWM Operation ........................................ 145
TMR2 to PR2 Match ........................................ 144, 149
PWM (ECCP Module) ...................................................... 149
Direction Change in Full-Bridge
Output Mode .................................................... 154
Effects of a Reset ..................................................... 159
Enhanced PWM Auto-Shutdown ............................. 156
Full-Bridge Application Example .............................. 154
Full-Bridge Mode ...................................................... 153
Half-Bridge Mode ..................................................... 152
Half-Bridge Output Mode
Applications Example ...................................... 152
Operation in Power Managed Modes ...................... 159
Operation with Fail-Safe Clock Monitor ................... 159
Output Configurations .............................................. 150
Output Relationships (Active-High) .......................... 151
Output Relationships (Active-Low) ........................... 151
Programmable Dead-Band Delay ............................ 156
Setup for PWM Operation ........................................ 159
Start-up Considerations ........................................... 158
Q
Q Clock .................................................................... 145, 150
R
RAM. See Data Memory.
RBIF Bit ............................................................................ 108
RC Oscillator ...................................................................... 25
RCIO Oscillator Mode ................................................ 25
RC_IDLE Mode .................................................................. 39
RC_RUN Mode .................................................................. 35
RCALL ............................................................................. 297
RCON Register
Bit Status During Initialization .................................... 48
Register File ....................................................................... 62
Register File Summary ................................................ 64–66
Registers
ADCON0 (A/D Control 0) ......................................... 223
ADCON1 (A/D Control 1) ......................................... 224
ADCON2 (A/D Control 2) ......................................... 225
BAUDCON (Baud Rate Control) .............................. 204
CCP1CON (Enhanced
Capture/Compare/PWM Control 1) ................. 147
CCPxCON (Standard
Capture/Compare/PWM Control) .................... 139
CMCON (Comparator Control) ................................ 233
CONFIG1H (Configuration 1 High) .......................... 250
CONFIG2H (Configuration 2 High) .......................... 252
CONFIG2L (Configuration 2 Low) ........................... 251
CONFIG3H (Configuration 3 High) .......................... 253
CONFIG4L (Configuration 4 Low) ........................... 253
CONFIG5H (Configuration 5 High) .......................... 254
CONFIG5L (Configuration 5 Low) ........................... 254
CONFIG6H (Configuration 6 High) .......................... 255
CONFIG6L (Configuration 6 Low) ........................... 255
CONFIG7H (Configuration 7 High) .......................... 256
CONFIG7L (Configuration 7 Low) ........................... 256
CVRCON (Comparator Voltage
Reference Control) .......................................... 239
DEVID1 (Device ID 1) .............................................. 257
DEVID2 (Device ID 2) .............................................. 257
ECCP1AS (ECCP Auto-Shutdown Control) ............ 157
EECON1 (Data EEPROM Control 1) ................... 75, 84
HLVDCON (High/Low-Voltage Detect Control) ....... 243
INTCON (Interrupt Control) ....................................... 93
INTCON2 (Interrupt Control 2) .................................. 94
INTCON3 (Interrupt Control 3) .................................. 95
IPR1 (Peripheral Interrupt Priority 1) ....................... 100
IPR2 (Peripheral Interrupt Priority 2) ....................... 101
OSCCON (Oscillator Control) .................................... 30
OSCTUNE (Oscillator Tuning) ................................... 27
PIE1 (Peripheral Interrupt Enable 1) ......................... 98
PIE2 (Peripheral Interrupt Enable 2) ......................... 99
PIR1 (Peripheral Interrupt Request (Flag) 1) ............. 96
PIR2 (Peripheral Interrupt Request (Flag) 2) ............. 97
PWM1CON (PWM Configuration) ........................... 156
RCON (Reset Control) ....................................... 42, 102
RCSTA (Receive Status and Control) ..................... 203
SSPCON1 (MSSP Control 1, I2C Mode) ................. 172
SSPCON1 (MSSP Control 1, SPI Mode) ................ 163
SSPCON2 (MSSP Control 2, I2C Mode) ................. 173
SSPSTAT (MSSP Status, I2C Mode) ...................... 171
SSPSTAT (MSSP Status, SPI Mode) ...................... 162
Status ........................................................................ 67
STKPTR (Stack Pointer) ............................................ 55
T0CON (Timer0 Control) ......................................... 123
T1CON (Timer1 Control) ......................................... 127
T2CON (Timer2 Control) ......................................... 133
T3CON (Timer3 Control) ......................................... 135
TRISE (PORTE/PSP Control) ................................. 118
TXSTA (Transmit Status and Control) ..................... 202
WDTCON (Watchdog Timer Control) ...................... 259
RESET ............................................................................. 297
Reset State of Registers .................................................... 48
Resets ....................................................................... 41, 249
Brown-out Reset (BOR) ........................................... 249
Oscillator Start-up Timer (OST) ............................... 249
Power-on Reset (POR) ............................................ 249
Power-up Timer (PWRT) ......................................... 249
PIC18F2420/2520/4420/4520
DS39631B-page 382 Preliminary © 2007 Microchip Technology Inc.
RETFIE ............................................................................ 298
RETLW ............................................................................. 298
RETURN .......................................................................... 299
Return Address Stack ........................................................ 54
Return Stack Pointer (STKPTR) ........................................ 55
Revision History ............................................................... 371
RLCF ................................................................................ 299
RLNCF ............................................................................. 300
RRCF ............................................................................... 300
RRNCF ............................................................................. 301
S
SCK .................................................................................. 161
SDI ................................................................................... 161
SDO ................................................................................. 161
SEC_IDLE Mode ................................................................ 38
SEC_RUN Mode ................................................................ 34
Serial Clock, SCK ............................................................. 161
Serial Data In (SDI) .......................................................... 161
Serial Data Out (SDO) ..................................................... 161
Serial Peripheral Interface. See SPI Mode.
SETF ................................................................................ 301
Single-Supply ICSP Programming.
Slave Select (SS) ............................................................. 161
Slave Select Synchronization ........................................... 167
SLEEP .............................................................................. 302
Sleep
OSC1 and OSC2 Pin States ...................................... 31
Sleep Mode ........................................................................37
Software Simulator (MPLAB SIM) .................................... 318
Software Simulator (MPLAB SIM30) ................................ 318
Special Event Trigger. See Compare (ECCP Mode).
Special Event Trigger. See Compare (ECCP Module).
Special Features of the CPU ............................................ 249
Special Function Registers ................................................ 63
Map ............................................................................ 63
SPI Mode (MSSP)
Associated Registers ...............................................169
Bus Mode Compatibility ........................................... 169
Effects of a Reset ..................................................... 169
Enabling SPI I/O ...................................................... 165
Master Mode ............................................................ 166
Master/Slave Connection ......................................... 165
Operation ................................................................. 164
Operation in Power Managed Modes ...................... 169
Serial Clock .............................................................. 161
Serial Data In ........................................................... 161
Serial Data Out ........................................................ 161
Slave Mode .............................................................. 167
Slave Select ............................................................. 161
Slave Select Synchronization .................................. 167
SPI Clock ................................................................. 166
Typical Connection .................................................. 165
SS .................................................................................... 161
SSPOV ............................................................................. 191
SSPOV Status Flag .......................................................... 191
SSPSTAT Register
R/W Bit ............................................................. 174, 175
Stack Full/Underflow Resets .............................................. 56
Standard Instructions ....................................................... 267
SUBFSR ........................................................................... 313
SUBFWB .......................................................................... 302
SUBLW ............................................................................ 303
SUBULNK ........................................................................ 313
SUBWF ............................................................................ 303
SUBWFB ......................................................................... 304
SWAPF ............................................................................ 304
T
Table Pointer Operations (table) ........................................ 76
Table Reads/Table Writes ................................................. 56
TBLRD ............................................................................. 305
TBLWT ............................................................................. 306
Time-out in Various Situations (table) ................................ 45
Timer0 .............................................................................. 123
Associated Registers ............................................... 125
Operation ................................................................. 124
Overflow Interrupt .................................................... 125
Prescaler ................................................................. 125
Prescaler Assignment (PSA Bit) .............................. 125
Prescaler Select (T0PS2:T0PS0 Bits) ..................... 125
Prescaler. See Prescaler, Timer0.
Reads and Writes in 16-Bit Mode ............................ 124
Source Edge Select (T0SE Bit) ............................... 124
Source Select (T0CS Bit) ......................................... 124
Switching Prescaler Assignment ............................. 125
Timer1 .............................................................................. 127
16-Bit Read/Write Mode .......................................... 129
Associated Registers ............................................... 131
Interrupt ................................................................... 130
Operation ................................................................. 128
Oscillator .......................................................... 127, 129
Oscillator Layout Considerations ............................. 130
Overflow Interrupt .................................................... 127
Resetting, Using the CCP
Special Event Trigger ...................................... 130
Special Event Trigger (ECCP) ................................. 148
TMR1H Register ...................................................... 127
TMR1L Register ....................................................... 127
Use as a Real-Time Clock ....................................... 130
Timer2 .............................................................................. 133
Associated Registers ............................................... 134
Interrupt ................................................................... 134
Operation ................................................................. 133
Output ...................................................................... 134
PR2 Register ................................................... 144, 149
TMR2 to PR2 Match Interrupt .......................... 144, 149
Timer3 .............................................................................. 135
16-Bit Read/Write Mode .......................................... 137
Associated Registers ............................................... 137
Operation ................................................................. 136
Oscillator .......................................................... 135, 137
Overflow Interrupt ............................................ 135, 137
Special Event Trigger (CCP) ................................... 137
TMR3H Register ...................................................... 135
TMR3L Register ....................................................... 135
Timing Diagrams
A/D Conversion ........................................................ 359
Acknowledge Sequence .......................................... 194
Asynchronous Reception ......................................... 214
Asynchronous Transmission .................................... 212
Asynchronous Transmission (Back to Back) ........... 212
Automatic Baud Rate Calculation ............................ 210
Auto-Wake-up Bit (WUE) During
Normal Operation ............................................ 215
Auto-Wake-up Bit (WUE) During Sleep ................... 215
© 2007 Microchip Technology Inc. Preliminary DS39631B-page 383
PIC18F2420/2520/4420/4520
Baud Rate Generator with Clock Arbitration ............ 188
BRG Overflow Sequence ......................................... 210
BRG Reset Due to SDA Arbitration
During Start Condition ..................................... 197
Brown-out Reset (BOR) ........................................... 345
Bus Collision During a Repeated
Start Condition (Case 1) .................................. 198
Bus Collision During a Repeated
Start Condition (Case 2) .................................. 198
Bus Collision During a Start Condition
(SCL = 0) ......................................................... 197
Bus Collision During a Stop Condition
(Case 1) ........................................................... 199
Bus Collision During a Stop Condition
(Case 2) ........................................................... 199
Bus Collision During Start Condition
(SDA only) ....................................................... 196
Bus Collision for Transmit and Acknowledge ........... 195
Capture/Compare/PWM (CCP) ................................ 347
CLKO and I/O .......................................................... 344
Clock Synchronization ............................................. 181
Clock/Instruction Cycle .............................................. 57
Example SPI Master Mode (CKE = 0) ..................... 349
Example SPI Master Mode (CKE = 1) ..................... 350
Example SPI Slave Mode (CKE = 0) ....................... 351
Example SPI Slave Mode (CKE = 1) ....................... 352
External Clock (All Modes except PLL) .................... 342
Fail-Safe Clock Monitor (FSCM) .............................. 262
First Start Bit Timing ................................................ 189
Full-Bridge PWM Output .......................................... 153
Half-Bridge PWM Output ......................................... 152
High/Low-Voltage Detect Characteristics ................ 339
High/Low-Voltage Detect Operation
(VDIRMAG = 0) ................................................ 245
High/Low-Voltage Detect Operation
(VDIRMAG = 1) ................................................ 246
I2C Bus Data ............................................................ 353
I2C Bus Start/Stop Bits ............................................. 353
I2C Master Mode (7 or 10-Bit Transmission) ........... 192
I2C Master Mode (7-Bit Reception) .......................... 193
I2C Slave Mode (10-Bit Reception, SEN = 0) .......... 178
I2C Slave Mode (10-Bit Reception, SEN = 1) .......... 183
I2C Slave Mode (10-Bit Transmission) ..................... 179
I2C Slave Mode (7-bit Reception, SEN = 0) ............. 176
I2C Slave Mode (7-Bit Reception, SEN = 1) ............ 182
I2C Slave Mode (7-Bit Transmission) ....................... 177
I2C Slave Mode General Call Address
Sequence (7 or 10-Bit Address Mode) ............ 184
I2C Stop Condition Receive or
Transmit Mode ................................................. 194
Master SSP I2C Bus Data ........................................ 355
Master SSP I2C Bus Start/Stop Bits ........................ 355
Parallel Slave Port (PIC18F4420/4520) ................... 348
Parallel Slave Port (PSP) Read ............................... 121
Parallel Slave Port (PSP) Write ............................... 121
PWM Auto-Shutdown (PRSEN = 0,
Auto-Restart Disabled) .................................... 158
PWM Auto-Shutdown (PRSEN = 1,
Auto-Restart Enabled) ..................................... 158
PWM Direction Change ........................................... 155
PWM Direction Change at Near
100% Duty Cycle ............................................. 155
PWM Output ............................................................ 144
Repeat Start Condition ............................................ 190
Reset, Watchdog Timer (WDT),
Oscillator Start-up Timer (OST),
Power-up Timer (PWRT) ................................. 345
Send Break Character Sequence ............................ 216
Slave Synchronization ............................................. 167
Slow Rise Time (MCLR Tied to VDD,
VDD Rise > TPWRT) ............................................ 47
SPI Mode (Master Mode) ........................................ 166
SPI Mode (Slave Mode, CKE = 0) ........................... 168
SPI Mode (Slave Mode, CKE = 1) ........................... 168
Synchronous Reception
(Master Mode, SREN) ..................................... 219
Synchronous Transmission ..................................... 217
Synchronous Transmission (Through TXEN) .......... 218
Time-out Sequence on POR w/PLL Enabled
(MCLR Tied to VDD) .......................................... 47
Time-out Sequence on Power-up
(MCLR Not Tied to VDD, Case 1) ...................... 46
Time-out Sequence on Power-up
(MCLR Not Tied to VDD, Case 2) ...................... 46
Time-out Sequence on Power-up
(MCLR Tied to VDD, VDD Rise < TPWRT) ........... 46
Timer0 and Timer1 External Clock .......................... 346
Transition for Entry to SEC_RUN Mode .................... 35
Transition for Entry to Sleep Mode ............................ 37
Transition for Two-Speed Start-up
(INTOSC to HSPLL) ........................................ 260
Transition for Wake from Sleep (HSPLL) .................. 37
Transition from RC_RUN Mode to
PRI_RUN Mode ................................................. 36
Transition from SEC_RUN Mode to
PRI_RUN Mode (HSPLL) .................................. 35
Transition Timing for Entry to Idle Mode .................... 38
Transition Timing for Wake from
Idle to Run Mode ............................................... 38
Transition to RC_RUN Mode ..................................... 36
USART Synchronous Receive
(Master/Slave) ................................................. 357
USART Synchronous Transmission
(Master/Slave) ................................................. 357
Timing Diagrams and Specifications ............................... 342
A/D Conversion Requirements ................................ 359
Capture/Compare/PWM Requirements ................... 347
CLKO and I/O Requirements ................................... 344
Example SPI Mode Requirements
(Master Mode, CKE = 0) .................................. 349
Example SPI Mode Requirements
(Master Mode, CKE = 1) .................................. 350
Example SPI Mode Requirements
(Slave Mode, CKE = 0) .................................... 351
Example SPI Mode Requirements
(Slave Mode, CKE = 1) .................................... 352
External Clock Requirements .................................. 342
I2C Bus Data Requirements (Slave Mode) .............. 354
I2C Bus Start/Stop Bits Requirements
(Slave Mode) ................................................... 353
Master SSP I2C Bus Data Requirements ................ 356
Master SSP I2C Bus Start/Stop Bits
Requirements .................................................. 355
Parallel Slave Port Requirements
(PIC18F4420/4520) ......................................... 348
PIC18F2420/2520/4420/4520
DS39631B-page 384 Preliminary © 2007 Microchip Technology Inc.
PLL Clock ................................................................. 343
Reset, Watchdog Timer, Oscillator Start-up Timer,
Power-up Timer and Brown-out Reset
Requirements ...................................................345
Timer0 and Timer1 External
Clock Requirements ......................................... 346
USART Synchronous Receive Requirements .........357
USART Synchronous Transmission
Requirements ...................................................357
Top-of-Stack Access .......................................................... 54
TRISE Register
PSPMODE Bit .......................................................... 114
TSTFSZ ............................................................................ 307
Two-Speed Start-up ................................................. 249, 260
Two-Word Instructions
Example Cases .......................................................... 58
TXSTA Register
BRGH Bit ................................................................. 205
V
Voltage Reference Specifications .................................... 338
W
Watchdog Timer (WDT) ........................................... 249, 258
Associated Registers ............................................... 259
Control Register ....................................................... 258
During Oscillator Failure .......................................... 261
Programming Considerations .................................. 258
WCOL ...................................................... 189, 190, 191, 194
WCOL Status Flag ................................... 189, 190, 191, 194
WWW, On-Line Support ...................................................... 6
X
XORLW ............................................................................ 307
XORWF ........................................................................... 308
© 2007 Microchip Technology Inc. Preliminary DS39631B-page 385
PIC18F2420/2520/4420/4520
THE MICROCHIP WEB SITE
Microchip provides online support via our WWW site at
www.microchip.com. This web site is used as a means
to make files and information easily available to
customers. Accessible by using your favorite Internet
browser, the web site contains the following
information:
• Product Support – Data sheets and errata,
application notes and sample programs, design
resources, user’s guides and hardware support
documents, latest software releases and archived
software
• General Technical Support – Frequently Asked
Questions (FAQ), technical support requests,
online discussion groups, Microchip consultant
program member listing
• Business of Microchip – Product selector and
ordering guides, latest Microchip press releases,
listing of seminars and events, listings of
Microchip sales offices, distributors and factory
representatives
CUSTOMER CHANGE NOTIFICATION
SERVICE
Microchip’s customer notification service helps keep
customers current on Microchip products. Subscribers
will receive e-mail notification whenever there are
changes, updates, revisions or errata related to a
specified product family or development tool of interest.
To register, access the Microchip web site at
www.microchip.com, click on Customer Change
Notification and follow the registration instructions.
CUSTOMER SUPPORT
Users of Microchip products can receive assistance
through several channels:
• Distributor or Representative
• Local Sales Office
• Field Application Engineer (FAE)
• Technical Support
• Development Systems Information Line
Customers should contact their distributor,
representative or field application engineer (FAE) for
support. Local sales offices are also available to help
customers. A listing of sales offices and locations is
included in the back of this document.
Technical support is available through the web site
at: http://support.microchip.com
PIC18F2420/2520/4420/4520
DS39631B-page 386 Preliminary © 2007 Microchip Technology Inc.
READER RESPONSE
It is our intention to provide you with the best documentation possible to ensure successful use of your Microchip product.
If you wish to provide your comments on organization, clarity, subject matter, and ways in which our documentation
can better serve you, please FAX your comments to the Technical Publications Manager at (480) 792-4150.
Please list the following information, and use this outline to provide us with your comments about this document.
To: Technical Publications Manager
RE: Reader Response
Total Pages Sent ________
From: Name
Company
Address
City / State / ZIP / Country
Telephone: (_______) _________ - _________
Application (optional):
Would you like a reply? Y N
Device: Literature Number:
Questions:
FAX: (______) _________ - _________
PIC18F2420/2520/4420/4520 DS39631B
1. What are the best features of this document?
2. How does this document meet your hardware and software development needs?
3. Do you find the organization of this document easy to follow? If not, why?
4. What additions to the document do you think would enhance the structure and subject?
5. What deletions from the document could be made without affecting the overall usefulness?
6. Is there any incorrect or misleading information (what and where)?
7. How would you improve this document?
© 2007 Microchip Technology Inc. Preliminary DS39631B-page 387
PIC18F2420/2520/4420/4520
PIC18F2420/2520/4420/4520 PRODUCT IDENTIFICATION SYSTEM
To order or obtain information, e.g., on pricing or delivery, refer to the factory or the listed sales office.
PART NO. X /XX XXX
Temperature Package Pattern
Range
Device
Device PIC18F2420/2520(1), PIC18F4420/4520(1),
PIC18F2420/2520T(2), PIC18F4420/4520T(2);
VDD range 4.2V to 5.5V
PIC18LF2420/2520(1), PIC18LF4420/4520(1),
PIC18LF2420/2520T(2), PIC18LF4420/4520T(2);
VDD range 2.0V to 5.5V
Temperature Range I = -40°C to +85°C (Industrial)
E = -40°C to +125°C (Extended)
Package PT = TQFP (Thin Quad Flatpack)
SO = SOIC
SP = Skinny Plastic DIP
P = PDIP
ML = QFN
Pattern QTP, SQTP, Code or Special Requirements
(blank otherwise)
Examples:
a) PIC18LF4520-I/P 301 = Industrial temp., PDIP
package, Extended VDD limits, QTP pattern
#301.
b) PIC18LF2420-I/SO = Industrial temp., SOIC
package, Extended VDD limits.
c) PIC18F4420-I/P = Industrial temp., PDIP
package, normal VDD limits.
Note 1: F = Standard Voltage Range
LF = Wide Voltage Range
2: T = in tape and reel TQFP
packages only.
DS39631B-page 388 © 2007 Microchip Technology Inc.
AMERICAS
Corporate Office
2355 West Chandler Blvd.
Chandler, AZ 85224-6199
Tel: 480-792-7200
Fax: 480-792-7277
Technical Support:
http://support.microchip.com
Web Address:
www.microchip.com
Atlanta
Duluth, GA
Tel: 678-957-9614
Fax: 678-957-1455
Boston
Westborough, MA
Tel: 774-760-0087
Fax: 774-760-0088
Chicago
Itasca, IL
Tel: 630-285-0071
Fax: 630-285-0075
Dallas
Addison, TX
Tel: 972-818-7423
Fax: 972-818-2924
Detroit
Farmington Hills, MI
Tel: 248-538-2250
Fax: 248-538-2260
Kokomo
Kokomo, IN
Tel: 765-864-8360
Fax: 765-864-8387
Los Angeles
Mission Viejo, CA
Tel: 949-462-9523
Fax: 949-462-9608
Santa Clara
Santa Clara, CA
Tel: 408-961-6444
Fax: 408-961-6445
Toronto
Mississauga, Ontario,
Canada
Tel: 905-673-0699
Fax: 905-673-6509
ASIA/PACIFIC
Asia Pacific Office
Suites 3707-14, 37th Floor
Tower 6, The Gateway
Habour City, Kowloon
Hong Kong
Tel: 852-2401-1200
Fax: 852-2401-3431
Australia - Sydney
Tel: 61-2-9868-6733
Fax: 61-2-9868-6755
China - Beijing
Tel: 86-10-8528-2100
Fax: 86-10-8528-2104
China - Chengdu
Tel: 86-28-8665-5511
Fax: 86-28-8665-7889
China - Fuzhou
Tel: 86-591-8750-3506
Fax: 86-591-8750-3521
China - Hong Kong SAR
Tel: 852-2401-1200
Fax: 852-2401-3431
China - Qingdao
Tel: 86-532-8502-7355
Fax: 86-532-8502-7205
China - Shanghai
Tel: 86-21-5407-5533
Fax: 86-21-5407-5066
China - Shenyang
Tel: 86-24-2334-2829
Fax: 86-24-2334-2393
China - Shenzhen
Tel: 86-755-8203-2660
Fax: 86-755-8203-1760
China - Shunde
Tel: 86-757-2839-5507
Fax: 86-757-2839-5571
China - Wuhan
Tel: 86-27-5980-5300
Fax: 86-27-5980-5118
China - Xian
Tel: 86-29-8833-7250
Fax: 86-29-8833-7256
ASIA/PACIFIC
India - Bangalore
Tel: 91-80-4182-8400
Fax: 91-80-4182-8422
India - New Delhi
Tel: 91-11-4160-8631
Fax: 91-11-4160-8632
India - Pune
Tel: 91-20-2566-1512
Fax: 91-20-2566-1513
Japan - Yokohama
Tel: 81-45-471- 6166
Fax: 81-45-471-6122
Korea - Gumi
Tel: 82-54-473-4301
Fax: 82-54-473-4302
Korea - Seoul
Tel: 82-2-554-7200
Fax: 82-2-558-5932 or
82-2-558-5934
Malaysia - Penang
Tel: 60-4-646-8870
Fax: 60-4-646-5086
Philippines - Manila
Tel: 63-2-634-9065
Fax: 63-2-634-9069
Singapore
Tel: 65-6334-8870
Fax: 65-6334-8850
Taiwan - Hsin Chu
Tel: 886-3-572-9526
Fax: 886-3-572-6459
Taiwan - Kaohsiung
Tel: 886-7-536-4818
Fax: 886-7-536-4803
Taiwan - Taipei
Tel: 886-2-2500-6610
Fax: 886-2-2508-0102
Thailand - Bangkok
Tel: 66-2-694-1351
Fax: 66-2-694-1350
EUROPE
Austria - Wels
Tel: 43-7242-2244-39
Fax: 43-7242-2244-393
Denmark - Copenhagen
Tel: 45-4450-2828
Fax: 45-4485-2829
France - Paris
Tel: 33-1-69-53-63-20
Fax: 33-1-69-30-90-79
Germany - Munich
Tel: 49-89-627-144-0
Fax: 49-89-627-144-44
Italy - Milan
Tel: 39-0331-742611
Fax: 39-0331-466781
Netherlands - Drunen
Tel: 31-416-690399
Fax: 31-416-690340
Spain - Madrid
Tel: 34-91-708-08-90
Fax: 34-91-708-08-91
UK - Wokingham
Tel: 44-118-921-5869
Fax: 44-118-921-5820
WORLDWIDE SALES AND SERVICE
12/08/06
1
LT1961
1961fa
FEATURES DESCRIPTIO U
APPLICATIOU S
TYPICAL APPLICATIO U
1.5A, 1.25MHz Step-Up
Switching Regulator
■ 1.5A Switch in a Small MSOP Package
■ Constant 1.25MHz Switching Frequency
■ Wide Operating Voltage Range: 3V to 25V
■ High Efficiency 0.2Ω Switch
■ 1.2V Feedback Reference Voltage
■ ±2% Overall Output Voltage Tolerance
■ Uses Low Profile Surface Mount External
Components
■ Low Shutdown Current: 6μA
■ Synchronizable from 1.5MHz to 2MHz
■ Current-Mode Loop Control
■ Constant Maximum Switch Current Rating at All Duty
Cycles*
■ Thermally Enhanced Exposed Pad 8-Lead Plastic
MSOP Package
The LT®1961 is a 1.25MHz monolithic boost switching
regulator. A high efficiency 1.5A, 0.2Ω switch is included
on the die together with all the control circuitry required to
complete a high frequency, current-mode switching regulator.
Current-mode control provides fast transient response
and excellent loop stability.
New design techniques achieve high efficiency at high
switching frequencies over a wide operating voltage range.
A low dropout internal regulator maintains consistent
performance over a wide range of inputs from 24V systems
to Li-Ion batteries. An operating supply current of
1mA maintains high efficiency, especially at lower output
currents. Shutdown reduces quiescent current to 6μA.
Maximum switch current remains constant at all duty
cycles. Synchronization allows an external logic level
signal to increase the internal oscillator from 1.5MHz to
2MHz.
The LT1961 is available in an exposed pad, 8-pin MSOP
package. Full cycle-by-cycle switch current limit protection
and thermal shutdown are provided. High frequency
operation allows the reduction of input and output filtering
components and permits the use of chip inductors.
■ DSL Modems
■ Portable Computers
■ Battery-Powered Systems
■ Distributed Power
Efficiency vs Load Current
5V to 12V Boost Converter
LT1961
VIN
VOUT
12V
0.5A*
VIN
5V
1961 TA01
6800pF
100pF
6.8k
10k
1%
90.9k
UPS120
10μF
CERAMIC
2.2μF
CERAMIC
VSW
SHDN FB
OPEN
OR
HIGH
= ON SYNC GND VC
*MAXIMUM OUTPUT CURRENT IS SUBJECT TO THERMAL DERATING.
6.8μH
2
6
8 3,4 7
5
1
LOAD CURRENT (mA)
0
EFFICIENCY (%)
90
85
80
75
70
65
60
100 200 300 400
1961 TA01a
500
VIN = 5V
VOUT = 12V
, LT, LTC and LTM are registered trademarks of Linear Technology Corporation.
All other trademarks are the property of their respective owners. *Patent Pending
2
LT1961
1961fa
ABSOLUTE MAXIMUM RATINGS W W W U
Input Voltage .......................................................... 25V
Switch Voltage ......................................................... 35V
SHDN Pin ............................................................... 25V
FB Pin Current ....................................................... 1mA
SYNC Pin Current .................................................. 1mA
Operating Junction Temperature Range (Note 2)
LT1961E, LT1961I ........................... – 40°C to 125°C
Storage Temperature Range ................ – 65°C to 150°C
Lead Temperature (Soldering, 10 sec)................. 300°C
(Note 1)
TJMAX = 125°C, θJA = 50°C/W
GROUND PAD CONNECTED
TO LARGE COPPER AREA
1234
VIN
SW
GND
GND
8765
SYNC
VC
FB
SHDN
TOP VIEW
MS8E PACKAGE
8-LEAD PLASTIC MSOP
PI CO FIGURATIOU U U
PARAMETER CONDITION MIN TYP MAX UNITS
Recommended Operating Voltage ● 3 25 V
Maximum Switch Current Limit ● 1.5 2 3 A
Oscillator Frequency 3.3V < VIN < 25V ● 1 1.5 MHz
Switch On Voltage Drop ISW = 1.5A ● 310 500 mV
VIN Undervoltage Lockout (Note 3) ● 2.47 2.6 2.73 V
VIN Supply Current ISW = 0A ● 0.9 1.3 mA
VIN Supply Current/ISW ISW = 1.5A 27 mA/A
Shutdown Supply Current VSHDN = 0V, VIN = 25V, VSW = 25V 6 20 μA
● 45 μA
Feedback Voltage 3V < VIN < 25V, 0.4V < VC < 0.9V 1.182 1.2 1.218 V
● 1.176 1.224 V
ELECTRICAL CHARACTERISTICS The ● denotes the specifications which apply over the full operating temperature
range, otherwise specifications are at TA = 25°C. VIN = 15V, VC = 0.8V, SHDN, SYNC and switch open unless otherwise noted.
LEAD FREE FINISH TAPE AND REEL PART MARKING* PACKAGE DESCRIPTION TEMPERATURE RANGE
LT1961EMS8E#PBF LT1961EMS8E#TRPBF LTQY 8-Lead Plastic MSOP –40°C to 125°C
LT1961IMS8E#PBF LT1961IMS8E#TRPBF LTQY 8-Lead Plastic MSOP –40°C to 125°C
LEAD BASED FINISH TAPE AND REEL PART MARKING* PACKAGE DESCRIPTION TEMPERATURE RANGE
LT1961EMS8E LT1961EMS8E#TR LTQY 8-Lead Plastic MSOP –40°C to 125°C
LT1961IMS8E LT1961IMS8E#TR LTQY 8-Lead Plastic MSOP –40°C to 125°C
ORDER IUFORWATIOU
Consult LTC Marketing for parts specified with wider operating temperature ranges. *Temperature grades are identified by a label on the shipping container.
For more information on lead free part marking, go to: http://www.linear.com/leadfree/
For more information on tape and reel specifications, go to: http://www.linear.com/tapeandreel/
3
LT1961
1961fa
ELECTRICAL CHARACTERISTICS
PARAMETER CONDITION MIN TYP MAX UNITS
FB Input Current ● 0 –0.2 –0.4 μA
FB to VC Voltage Gain 0.4V < VC < 0.9V 150 350
FB to VC Transconductance ΔIVC = ±10μA ● 500 850 1300 μMho
VC Pin Source Current VFB = 1V ● – 85 –120 –165 μA
VC Pin Sink Current VFB = 1.4V ● 70 110 165 μA
VC Pin to Switch Current Transconductance 2.4 A/V
VC Pin Minimum Switching Threshold Duty Cycle = 0% 0.3 V
VC Pin 1.5A ISW Threshold 0.9 V
Maximum Switch Duty Cycle VC = 1.2V, ISW = 100mA ● 80 90 %
VC = 1.2V, ISW = 1A, 25°C ≤ TA ≤ 125°C 75 80 %
VC = 1.2V, ISW = 1A, TA ≤ 25°C 70 75 %
SHDN Threshold Voltage ● 1.28 1.35 1.42 V
SHDN Input Current (Shutting Down) SHDN = 60mV Above Threshold ● –7 –10 –13 μA
SHDN Threshold Current Hysteresis SHDN = 100mV Below Threshold 4 7 10 μA
SYNC Threshold Voltage 1.5 2.2 V
SYNC Input Frequency 1.5 2 MHz
SYNC Pin Resistance ISYNC = 1mA 20 kΩ
The ● denotes the specifications which apply over the full operating temperature
range, otherwise specifications are at TA = 25°C. VIN = 15V, VC = 0.8V, SHDN, SYNC and switch open unless otherwise noted.
Note 1: Stresses beyond those listed under Absolute Maximum Ratings
may cause permanent damage to the device. Exposure to any Absolute
Maximum Rating condition for extended periods may affect device
reliability and lifetime.
Note 2: The LT1961E is guaranteed to meet performance specifications
from 0°C to 125°C junction temperature. Specifications over the –40°C to
125°C operating junction temperature range are assured by design,
characterization and correlation with statistical process controls. The
LT1961I is guaranteed over the – 40ºC to 125ºC operating junction
temperature range.
Note 3: Minimum input voltage is defined as the voltage where the
internal regulator enters lockout. Actual minimum input voltage to
maintain a regulated output will depend on output voltage and load
current. See Applications Information.
4
LT1961
1961fa
TYPICAL PERFORMANCE CHARACTERISTICS U W
FB vs Temperature Switch On Voltage Drop Oscillator Frequency
SHDN Threshold vs Temperature SHDN Supply Current vs VIN SHDN IP Current vs Temperature
TEMPERATURE (°C)
–50 –25 0 25 50 75 100 125
FB VOLTAGE (V)
1961 G01
1.22
1.21
1.20
1.19
1.18
SWITCH CURRENT (A)
0 0.5 1 1.5
SWITCH VOLTAGE (mV)
1961 G02
400
350
300
250
200
150
100
50
0
125°C
25°C
–40°C
TEMPERATURE (°C)
–50 –25 0 25 50 75 100 125
FREQUENCY (MHz)
1961 G03
1.5
1.4
1.3
1.2
1.1
TA = 25°C
TEMPERATURE (°C)
–50 –25 0 25 50 75 100 125
SHDN THRESHOLD (V)
1961 G04
1.40
1.38
1.36
1.34
1.32
1.30
VIN (V)
0 5 10 15 20 25 30
VIN CURRENT (μA)
1961 G05
7
6
5
4
3
2
1
0
TA = 25°C
SHDN = 0V
TEMPERATURE (°C)
–50 –25 0 25 50 75 100 125
SHDN INPUT (μA)
1961G06
–12
–10
–8
–6
–4
–2
0
STARTING UP
SHUTTING DOWN
SHDN Supply Current Input Supply Current Current Limit Foldback
SHUTDOWN VOLTAGE (V)
0 0.2 0.4 0.6 0.8 1 1.2 1.4
VIN CURRENT (μA)
1961 G07
300
250
200
150
100
50
0
TA = 25°C
VIN = 15V
INPUT VOLTAGE (V)
0 5 10 15 20 25 30
VIN CURRENT (μA)
1961 G08
1200
1000
800
600
400
200
0
MINIMUM
INPUT
VOLTAGE
TA = 25°C
FEEDBACK VOLTAGE (V)
0 0.2 0.4 0.6 0.8 1 1.2
SWITCH PEAK CURRENT (A)
1961 G09
2.0
1.5
1.0
0.5
0
FB INPUT CURRENT (μA)
40
30
20
10
0
FB CURRENT
SWITCH CURRENT
TA = 25°C
5
LT1961
1961fa
FB: The feedback pin is used to set output voltage using an
external voltage divider that generates 1.2V at the pin with
the desired output voltage. If required, the current limit
can be reduced during start up when the FB pin is below
0.5V (see the Current Limit Foldback graph in the Typical
Performance Characteristics section). An impedance of
less than 5kΩ at the FB pin is needed for this feature to
operate.
VIN: This pin powers the internal circuitry and internal
regulator. Keep the external bypass capacitor close to this
pin.
GND: Short GND pins 3 and 4 and the exposed pad on the
PCB. The GND is the reference for the regulated output, so
load regulation will suffer if the “ground” end of the load
is not at the same voltage as the GND of the IC. This
condition occurs when the load current flows through the
metal path between the GND pins and the load ground
point. Keep the ground path short between the GND pins
and the load and use a ground plane when possible. Keep
the path between the input bypass and the GND pins short.
The exposed pad should be attached to a large copper area
to improve thermal resistance.
VSW: The switch pin is the collector of the on-chip power
NPN switch and has large currents flowing through it.
Keep the traces to the switching components as short as
possible to minimize radiation and voltage spikes.
SYNC: The sync pin is used to synchronize the internal
oscillator to an external signal. It is directly logic compatible
and can be driven with any signal between 20% and
80% duty cycle. The synchronizing range is equal to initial
operating frequency, up to 2MHz. See Synchronization
section in Applications Information for details. When not
in use, this pin should be grounded.
SHDN: The shutdown pin is used to turn off the regulator
and to reduce input drain current to a few microamperes.
The 1.35V threshold can function as an accurate undervoltage
lockout (UVLO), preventing the regulator from
operating until the input voltage has reached a predetermined
level. Float or pull high to put the regulator in the
operating mode.
VC: The VC pin is the output of the error amplifier and the
input of the peak switch current comparator. It is normally
used for frequency compensation, but can do double duty
as a current clamp or control loop override. This pin sits
at about 0.3V for very light loads and 0.9V at maximum
load.
PIN FUNCTIONSU U U
6
LT1961
1961fa
amplifier commands current to be delivered to the output
rather than voltage. A voltage fed system will have low
phase shift up to the resonant frequency of the inductor
and output capacitor, then an abrupt 180° shift will occur.
The current fed system will have 90° phase shift at a much
lower frequency, but will not have the additional 90° shift
until well beyond the LC resonant frequency. This makes
it much easier to frequency compensate the feedback loop
and also gives much quicker transient response.
A comparator connected to the shutdown pin disables the
internal regulator, reducing supply current.
The LT1961 is a constant frequency, current-mode boost
converter. This means that there is an internal clock and
two feedback loops that control the duty cycle of the power
switch. In addition to the normal error amplifier, there is a
current sense amplifier that monitors switch current on a
cycle-by-cycle basis. A switch cycle starts with an oscillator
pulse which sets the RS flip-flop to turn the switch on.
When switch current reaches a level set by the inverting
input of the comparator, the flip-flop is reset and the
switch turns off. Output voltage control is obtained by
using the output of the error amplifier to set the switch
current trip point. This technique means that the error
Figure 1. Block Diagram
BLOCK DIAGRAMW
–
+
–
+
Σ
VIN
2.5V BIAS
REGULATOR
1.25MHz
OSCILLATOR
SW
FB
VC
GND
GND
1767 F01
SLOPE COMP
0.01Ω
INTERNAL
VCC
CURRENT SENSE
AMPLIFIER VOLTAGE
GAIN = 40
SYNC
SHDN
SHUTDOWN
COMPARATOR
CURRENT
COMPARATOR
ERROR
AMPLIFIER
gm = 850μMho
RS
FLIP-FLOP
DRIVER
CIRCUITRY
S
R
0.3V
Q1
POWER
SWITCH
1.2V
–
+
+ –
1.35V
3μA
7μA
1
8
5
7
6
3
4
2
7
LT1961
1961fa
APPLICATIONS INFORMATION W U U U
FB RESISTOR NETWORK
The suggested resistance (R2) from FB to ground is 10k
1%. This reduces the contribution of FB input bias current
to output voltage to less than 0.2%. The formula for the
resistor (R1) from VOUT to FB is:
R
R V
R A
OUT
1
2 12
1 2 2 0 2
=
( − )
− μ
.
. (. )
defines the pole frequency of the output stage, an X7R or
X5R type ceramic, which have good temperature stability,
is recommended.
Tantalum capacitors are usually chosen for their bulk
capacitance properties, useful in high transient load applications.
ESR rather than absolute value defines output
ripple at 1.25MHz. Values in the 22μF to 100μF range are
generally needed to minimize ESR and meet ripple current
ratings. Care should be taken to ensure the ripple ratings
are not exceeded.
Table 1. Surface Mount Solid Tantalum Capacitor ESR and
Ripple Current
E Case Size ESR (Max, Ω) Ripple Current (A)
AVX TPS, Sprague 593D 0.1 to 0.3 0.7 to 1.1
AVX TAJ 0.7 to 0.9 0.4
D Case Size
AVX TPS, Sprague 593D 0.1 to 0.3 0.7 to 1.1
C Case Size
AVX TPS 0.2 (typ) 0.5 (typ)
INPUT CAPACITOR
Unlike the output capacitor, RMS ripple current in the
input capacitor is normally low enough that ripple current
rating is not an issue. The current waveform is triangular,
with an RMS value given by:
I
V V V
L f V RIPPLE RMS
IN OUT IN
OUT ( )= ( )( − )
( )( )( )
0.29
At higher switching frequency, the energy storage requirement
of the input capacitor is reduced so values in the
range of 1μF to 4.7μF are suitable for most applications.
Y5V or similar type ceramics can be used since the
absolute value of capacitance is less important and has no
significant effect on loop stability. If operation is required
close to the minimum input voltage required by either the
output or the LT1961, a larger value may be necessary.
This is to prevent excessive ripple causing dips below the
minimum operating voltage resulting in erratic operation.
Figure 2. Feedback Network
OUTPUT CAPACITOR
Step-up regulators supply current to the output in pulses.
The rise and fall times of these pulses are very fast. The
output capacitor is required to reduce the voltage ripple
this causes. The RMS ripple current can be calculated
from:
IRIPPLE(RMS) =IOUT (VOUT − VIN) / VIN
The LT1961 will operate with both ceramic and tantalum
output capacitors. Ceramic capacitors are generally chosen
for their small size, very low ESR (effective series
resistance), and good high frequency operation, reducing
output ripple voltage. Their low ESR removes a useful zero
in the loop frequency response, common to tantalum
capacitors. To compensate for this, the VC loop compensation
pole frequency must typically be reduced by a factor
of 10. Typical ceramic output capacitors are in the 1μF to
10μF range. Since the absolute value of capacitance
–
+ 1.2V
VSW
VC GND
1961 F02
R1
R2
10k
OUTPUT
ERROR
AMPLIFIER
FB
LT1961
+
8
LT1961
1961fa
APPLICATIONS INFORMATION W U U U
INDUCTOR CHOICE AND MAXIMUM OUTPUT
CURRENT
When choosing an inductor, there are 2 conditions that
limit the minimum inductance; required output current,
and avoidance of subharmonic oscillation. The maximum
output current for the LT1961 in a standard boost converter
configuration with an infinitely large inductor is:
I A
V
V OUT MAX
IN
OUT
( ) .
• = 1 5 η
Where η = converter efficiency (typically 0.87 at high
current).
As the value of inductance is reduced, ripple current
increases and IOUT(MAX) is reduced. The minimum inductance
for a required output current is given by:
L
V V V
V f
V I
V
MIN
IN OUT IN
OUT
OUT OUT
IN
=
⎛
⎝ ⎜
⎞
⎠ ⎟
( – )
( ) . –
( )( )
•
2 15
η
The second condition, avoidance of subharmonic oscillation,
must be met if the operating duty cycle is greater than
50%. The slope compensation circuit within the LT1961
prevents subharmonic oscillation for inductor ripple currents
of up to 0.7AP-P, defining the minimum inductor
value to be:
L
V V V
V f MIN
IN OUT IN
OUT
= ( – )
0.7 ( )
These conditions define the absolute minimum inductance.
However, it is generally recommended that to
prevent excessive output noise, and difficulty in obtaining
stability, the ripple current is no more than 40% of the
average inductor current. Since inductor ripple is:
I
V V V
V L f P P RIPPLE
IN OUT IN
OUT
− = ( – )
( )( )
The recommended minimum inductance is:
L
V V V
V I f
MIN
IN OUT IN
OUT OUT
= ( ) ( – )
. ( ) ( )( )
2
0 4 2
The inductor value may need further adjustment for other
factors such as output voltage ripple and filtering requirements.
Remember also, inductance can drop significantly
with DC current and manufacturing tolerance.
The inductor must have a rating greater than its peak
operating current to prevent saturation resulting in efficiency
loss. Peak inductor current is given by:
I
V I
V
V V V
V L f LPEAK
OUT OUT
IN
IN OUT IN
OUT
= ( )( ) + −
•
( )
η 2 ( )( )
Also, consideration should be given to the DC resistance
of the inductor. Inductor resistance contributes directly to
the efficiency losses in the overall converter.
Suitable inductors are available from Coilcraft, Coiltronics,
Dale, Sumida, Toko, Murata, Panasonic and other manufactures.
Table 2
PART NUMBER VALUE (uH) ISAT(DC) (Amps) DCR (Ω) HEIGHT (mm)
Coiltronics
TP1-2R2 2.2 1.3 0.188 1.8
TP2-2R2 2.2 1.5 0.111 2.2
TP3-4R7 4.7 1.5 0.181 2.2
TP4- 100 10 1.5 0.146 3.0
Murata
LQH1C1R0M04 1.0 0.51 0.28 1.8
LQH3C1R0M24 1.0 1.0 0.06 2.0
LQH3C2R2M24 2.2 0.79 0.1 2.0
LQH4C1R5M04 1.5 1 0.09 2.6
Sumida
CD73- 100 10 1.44 0.080 3.5
CDRH4D18-2R2 2.2 1.32 0.058 1.8
CDRH5D18-6R2 6.2 1.4 0.071 1.8
CDRH5D28-100 10 1.3 0.048 2.8
Coilcraft
1008PS-272M 2.7 1.3 0.14 2.7
LPO1704-222M 2.2 1.6 0.12 1.0
LPO1704-332M 3.3 1.3 0.16 1.0
9
LT1961
1961fa
APPLICATIONS INFORMATION W U U U
shutdown pin can be used. The threshold voltage of the
shutdown pin comparator is 1.35V. A 3μA internal current
source defaults the open pin condition to be operating (see
Typical Performance Graphs). Current hysteresis is added
above the SHDN threshold. This can be used to set voltage
hysteresis of the UVLO using the following:
R
V V
A
R
V
V V
R
A
H L
H
1
7
2
1 35
1 35
1
3
= −
μ
=
( − ) + μ
.
.
VH – Turn-on threshold
VL – Turn-off threshold
Example: switching should not start until the input is
above 4.75V and is to stop if the input falls below 3.75V.
VH = 4.75V
VL = 3.75V
R
V V
A
k
R
V
V V
k
A
k
1
4 75 3 75
7
143
2
1 35
4 75 1 35
143
3
50 4
= −
μ
=
=
( − ) + μ
=
. .
.
. .
.
Keep the connections from the resistors to the SHDN pin
short and make sure that the interplane or surface capacitance
to the switching nodes are minimized. If high resistor
values are used, the SHDN pin should be bypassed with
a 1nF capacitor to prevent coupling problems from the
switch node.
CATCH DIODE
The suggested catch diode (D1) is a UPS120 or 1N5818
Schottky. It is rated at 1A average forward current and
20V/30V reverse voltage. Typical forward voltage is 0.5V
at 1A. The diode conducts current only during switch off
time. Peak reverse voltage is equal to regulator output
voltage. Average forward current in normal operation is
equal to output current.
SHUTDOWN AND UNDERVOLTAGE LOCKOUT
Figure 4 shows how to add undervoltage lockout (UVLO)
to the LT1961. Typically, UVLO is used in situations where
the input supply is current limited, or has a relatively high
source resistance. A switching regulator draws constant
power from the source, so source current increases as
source voltage drops. This looks like a negative resistance
load to the source and can cause the source to current limit
or latch low under low source voltage conditions. UVLO
prevents the regulator from operating at source voltages
where these problems might occur.
Figure 4. Undervoltage Lockout
1.35V
GND
INPUT
R1
1961 F04
SHDN
VCC
IN
LT1961
3μA
C1 R2
7μA
An internal comparator will force the part into shutdown
below the minimum VIN of 2.6V. This feature can be used
to prevent excessive discharge of battery-operated systems.
If an adjustable UVLO threshold is required, the
10
LT1961
1961fa
SYNCHRONIZATION
The SYNC pin, is used to synchronize the internal oscillator
to an external signal. The SYNC input must pass from
a logic level low, through the maximum synchronization
threshold with a duty cycle between 20% and 80%. The
input can be driven directly from a logic level output. The
synchronizing range is equal to initial operating frequency
up to 2MHz. This means that minimum practical sync
frequency is equal to the worst-case high self-oscillating
frequency (1.5MHz), not the typical operating frequency
of 1.25MHz. Caution should be used when synchronizing
above 1.7MHz because at higher sync frequencies the
amplitude of the internal slope compensation used to
prevent subharmonic switching is reduced. Higher inductor
values will tend to eliminate this problem. See Frequency
Compensation section for a discussion of an
entirely different cause of subharmonic switching before
assuming that the cause is insufficient slope compensation.
Application Note 19 has more details on the theory
of slope compensation.
LAYOUT CONSIDERATIONS
As with all high frequency switchers, when considering
layout, care must be taken to achieve optimal electrical,
thermal and noise performance. For maximum efficiency,
switch rise and fall times are typically in the nanosecond
range. To prevent noise both radiated and conducted, the
APPLICATIONS INFORMATION W U U U
high speed switching current path, shown in Figure 5,
must be kept as short as possible. This is implemented in
the suggested layout of Figure 6. Shortening this path will
also reduce the parasitic trace inductance of approximately
25nH/inch. At switch off, this parasitic inductance
produces a flyback spike across the LT1961 switch. When
operating at higher currents and output voltages, with
poor layout, this spike can generate voltages across the
LT1961 that may exceed its absolute maximum rating. A
ground plane should always be used under the switcher
circuitry to prevent interplane coupling and overall noise.
The VC and FB components should be kept as far away as
possible from the switch node. The LT1961 pinout has
been designed to aid in this. The ground for these components
should be separated from the switch current path.
Failure to do so will result in poor stability or subharmonic
like oscillation.
Board layout also has a significant effect on thermal
resistance. The exposed pad is the copper plate that runs
under the LT1961 die. This is the best thermal path for heat
out of the package. Soldering the pad onto the board will
reduce die temperature and increase the power capability
of the LT1961. Provide as much copper area as possible
around this pad. Adding multiple solder filled feedthroughs
under and around the pad to the ground plane will also
help. Similar treatment to the catch diode and inductor
terminations will reduce any additional heating effects.
1961 F05
VOUT
L1
SW
GND
LT1961
D1
C1
C3
VIN
HIGH
FREQUENCY
SWITCHING
PATH
LOAD
Figure 5. High Speed Switching Path
11
LT1961
1961fa
Figure 6. Typical Application and Suggested Layout (Topside Only Shown)
LT1961
VIN
OUTPUT
12V
0.5A*
INPUT
5V
C2
6800pF C4
R3 100pF
6.8k
R2
10k
1%
R1
90.9k
D1
UPS120
C1
10μF
CERAMIC
C3
2.2μF
CERAMIC
VSW
SHDN FB
OPEN
OR
HIGH
= ON SYNC GND VC
*MAXIMUM OUTPUT CURRENT IS SUBJECT TO THERMAL DERATING.
L1
6.8μH
VOUT
INPUT GND
C3
C1
R2 R1
L1
D1
KELVIN SENSE
VOUT
MINIMIZE
LT1961,
C1, D1 LOOP
KEEP FB AND VC
COMPONENTS
AWAY FROM
HIGH FREQUENCY,
HIGH INPUT
COMPONENTS
PLACE FEEDTHROUGHS
AROUND GROUND PIN FOR
GOOD THERMAL CONDUCTIVITY
LT1961EMS8E
GND
C4
U1
SOLDER EXPOSED
GROUND PAD
TO BOARD
R3
C2
APPLICATIONS INFORMATION W U U U
12
LT1961
1961fa
APPLICATIONS INFORMATION W U U U
THERMAL CALCULATIONS
Power dissipation in the LT1961 chip comes from four
sources: switch DC loss, switch AC loss, drive current, and
input quiescent current. The following formulas show how
to calculate each of these losses. These formulas assume
continuous mode operation, so they should not be used
for calculating efficiency at light load currents.
DC duty cycle
V V
V
I
V I
V
OUT IN
OUT
SW
OUT OUT
IN
,
( )
( )( )
= −
=
Switch loss:
PSW = (DC)(ISW)2(RSW)+ 17n(ISW)(VOUT )(f)
VIN loss:
P
V I DC
VIN mA V
IN SW
= + IN ( )( )( )
( )
50
1
RSW = Switch resistance (≈ 0.27Ω hot)
Example: VIN = 5V, VOUT = 12V and IOUT = 0.5A
Total power dissipation = 0.23 + 0.31 + 0.07 + 0.005 =
0.62W
Thermal resistance for LT1961 package is influenced by
the presence of internal or backside planes. With a full
plane under the package, thermal resistance will be about
50°C/W. To calculate die temperature, use the appropriate
thermal resistance number and add in worst-case ambient
temperature:
TJ = TA + θJA (PTOT)
If a true die temperature is required, a measurement of the
SYNC to GND pin resistance can be used. The SYNC pin
resistance across temperature must first be calibrated,
with no device power, in an oven. The same measurement
can then be used in operation to indicate the die temperature.
FREQUENCY COMPENSATION
Loop frequency compensation is performed on the output
of the error amplifier (VC pin) with a series RC network.
The main pole is formed by the series capacitor and the
output impedance (≈500kΩ) of the error amplifier. The
pole falls in the range of 2Hz to 20Hz. The series resistor
creates a “zero” at 1kHz to 5kHz, which improves loop
stability and transient response. A second capacitor, typically
one-tenth the size of the main compensation capacitor,
is sometimes used to reduce the switching frequency
ripple on the VC pin. VC pin ripple is caused by output
voltage ripple attenuated by the output divider and multiplied
by the error amplifier. Without the second capacitor,
VC pin ripple is:
VC Pin Ripple =
VRIPPLE = Output ripple (VP–P)
gm = Error amplifier transconductance
(≈850μmho)
RC = Series resistor on VC pin
VOUT = DC output voltage
1.2(VRIPPLE)(gm)(RC)
(VOUT)
To prevent irregular switching, VC pin ripple should be
kept below 50mVP–P. Worst-case VC pin ripple occurs at
maximum output load current and will also be increased if
poor quality (high ESR) output capacitors are used. The
addition of a 47pF capacitor on the VC pin reduces switching
frequency ripple to only a few millivolts. A low value for
RC will also reduce VC pin ripple, but loop phase margin
may be inadequate.
13
LT1961
1961fa
LT1961
FB VIN
VC
VIN
5V TO 10V
*DALE LPE-4841-100MB
GND
LT1961 • TA02
S/S VSW
P6KE-20A
1N4148
UPS140
UPS140
C1
4.7μF
R2
10k
1%
R1
115k
1%
C2
2.2nF
C3
100pF
R3
10k
–VOUT
–15V
VOUT
15V
C4
47μF
C5
47μF
ON
OFF
2, 3
8, 9
7
T1*
4
10
1
•
•
•
+ +
+
Dual Output Flyback Converter
TYPICAL APPLICATIO SU
LT1961
VIN
GND
VIN**
4V TO 9V
VC
FB
LT1961 • TA03
S/S VSW
C1
4.7μF
20V
C4
2.2nF
C5
100pF
R1
10k
R3
10k
1%
R2
31.6k
1%
VOUT
†
5V
C3
47μF
10V
ON
OFF
L1A*
10μH
•
•
L1B*
10μH
C2
4.7μF
BH ELECTRONICS 511-1012
INPUT VOLTAGE MAY BE GREATER OR
LESS THAN OUTPUT VOLTAGE
D1
UPS120
VIN
4V
5V
6V
7V
9V
IOUT
0.59A
0.65A
0.70A
0.74A
0.80A
†MAX IOUT
*
**
+
+
4V-9VIN to 5VOUT SEPIC Converter**
14
LT1961
1961fa
LT1961
VIN
GND VC
FB
LT1961 • TA04
S/S VSW
L1
4.7μH
C1
10μF
SINGLE
Li-Ion
CELL
C4
47μF
10V
C2
2.2nF
C3
100pF
R3
10k
R2
10k
1%
R1
31.6k
1%
VOUT
5V
D1
UPS120
ON
OFF
+ + +
VIN
2.7V
3.3V
3.6V
IOUT
0.75A
0.93A
1.0A
Single Li-Ion Cell to 5V
TYPICAL APPLICATIO SU
15
LT1961
1961fa
PACKAGE DESCRIPTIONU
Information furnished by Linear Technology Corporation is believed to be accurate and reliable.
However, no responsibility is assumed for its use. Linear Technology Corporation makes no representation
that the interconnection of its circuits as described herein will not infringe on existing patent rights.
MSOP (MS8E) 0307 REV D
0.53 ± 0.152
(.021 ± .006)
SEATING
PLANE
NOTE:
1. DIMENSIONS IN MILLIMETER/(INCH)
2. DRAWING NOT TO SCALE
3. DIMENSION DOES NOT INCLUDE MOLD FLASH, PROTRUSIONS OR GATE BURRS.
MOLD FLASH, PROTRUSIONS OR GATE BURRS SHALL NOT EXCEED 0.152mm (.006") PER SIDE
4. DIMENSION DOES NOT INCLUDE INTERLEAD FLASH OR PROTRUSIONS.
INTERLEAD FLASH OR PROTRUSIONS SHALL NOT EXCEED 0.152mm (.006") PER SIDE
5. LEAD COPLANARITY (BOTTOM OF LEADS AFTER FORMING) SHALL BE 0.102mm (.004") MAX
0.18
(.007)
0.254
(.010)
1.10
(.043)
MAX
0.22 – 0.38
(.009 – .015)
TYP
0.86
(.034)
REF
0.65
(.0256)
BSC
0° – 6° TYP
DETAIL “A”
DETAIL “A”
GAUGE PLANE
1 2 3 4
4.90 ± 0.152
(.193 ± .006)
8
8
1
BOTTOM VIEW OF
EXPOSED PAD OPTION
7 6 5
3.00 ± 0.102
(.118 ± .004)
(NOTE 3)
3.00 ± 0.102
(.118 ± .004)
(NOTE 4)
0.52
(.0205)
REF
1.83 ± 0.102
(.072 ± .004)
2.06 ± 0.102
(.081 ± .004)
5.23
(.206)
MIN
3.20 – 3.45
(.126 – .136)
2.083 ± 0.102
(.082 ± .004)
2.794 ± 0.102
(.110 ± .004)
0.889 ± 0.127
(.035 ± .005)
RECOMMENDED SOLDER PAD LAYOUT
0.42 ± 0.038
(.0165 ± .0015)
TYP
0.65
(.0256)
BSC
0.1016 ± 0.0508
(.004 ± .002)
MS8E Package
8-Lead Plastic MSOP, Exposed Die Pad
(Reference LTC DWG # 05-08-1662 Rev D)
16
LT1961
1961fa
PART NUMBER DESCRIPTION COMMENTS
LT1308A 600kHz, 2A, Step-Up Regulator 30V Switch, VIN = 1V to 6V, Low Battery Comparator,
S8 Package
LT1310 4.5MHz, 1.5A Step-Up with Phase Lock Loop 34V Switch, VIN = 2.75V to 18V, VOUT up to 35V, MS10E Package
LT1370 High Efficiency DC/DC Converter 42V Switch, 6A, 500kHz Switch, DD-Pak, TO-220 Package
LT1371 High Efficiency DC/DC Converter 35V Switch, 3A, 500kHz Switch, DD-Pak, TO-220 Package
LT1372/LT1377 500kHz and 1MHz High Efficiency 1.5A Switching Regulators Boost Topology, VIN(MIN) = 2.7V, S8 Package
LT1946/LT1946A 1.2MHz/2.7MHz, 1.5A, Monolithic Step-Up Regulator VIN = 2.6V to 16V, VOUT up to 34V, Integrated SS, MS8 Package
LTC3400/ 1.2MHz, 600mA, Synchronous Step-Up VIN = 0.85V to 5V, VOUT to 5.5V, Up to 95% Efficiency,
LTC3400B ThinSOT Package
LTC3401 Single Cell, High Current (1A), Micropower, Synchronous 3MHz VIN = 0.85V to 5V, VOUT to 5.5V, Up to 97% Efficiency
Step-Up DC/DC Converter Synchronizable, Oscillator from 100kHz to 3MHz, MS10 Package
LTC3402 Single Cell, High Current (2A), Micropower, Synchronous 3MHz VIN = 0.85V to 5V, VOUT to 5.5V, Up to 95% Efficiency
Step-Up DC/DC Converter Synchronizable, Oscillator from 100kHz to 3MHz, MS10 Package
LTC3405/ 1.5MHz High Efficiency, IOUT = 300mA, Monolithic Synchronous VIN = 2.5V to 5.5V, VOUT to 0.8V, Up to 95% Efficiency, 100%
LTC3405A Step-Down Regulator Duty Cycle, IQ = 20μA, ThinSOT Package
ThinSOT is a trademark of Linear Technology Corporation.
LT 0707 REV A • PRINTED IN USA
© LINEAR TECHNOLOGY CORPORATION 2001
RELATED PARTS
Linear Technology Corporation
1630 McCarthy Blvd., Milpitas, CA 95035-7417
(408) 432-1900 ● FAX: (408) 434-0507 ● www.linear.com
LASER
190Ω
1%
1N4002
0.1μF (ALL)
10k
VIN
10μF
VC
VIN FB
GND
2.2μF
VIN
12V TO 25V
150Ω
MUR405
L2
10μH
LT1961
L1
5 4 1 3
2
11 8 HV DIODES
1800pF
10kV
0.01μF
5kV
1800pF
10kV
47k
5W
2.2μF
0.47μF
L1 =
Q1, Q2 =
0.47μF =
HV DIODES =
LASER =
COILTRONICS CTX02-11128
ZETEX ZTX849
WIMA 3X 0.15μF TYPE MKP-20
SEMTECH-FM-50
HUGHES 3121H-P
10k
LT1961 • TA05
VSW
Q1 Q2
+
+
+
COILTRONICS (407) 241-7876
U TYPICAL APPLICATIO
High Voltage Laser Power Supply
LT3757/LT3757A
1
3757afd
n Wide Input Voltage Range: 2.9V to 40V
n Positive or Negative Output Voltage Programming
with a Single Feedback Pin
n Current Mode Control Provides Excellent Transient
Response
n Programmable Operating Frequency (100kHz to
1MHz) with One External Resistor
n Synchronizable to an External Clock
n Low Shutdown Current < 1μA
n Internal 7.2V Low Dropout Voltage Regulator
n Programmable Input Undervoltage Lockout with
Hysteresis
n Programmable Soft-Start
n Small 10-Lead DFN (3mm × 3mm) and Thermally
Enhanced 10-Pin MSOP Packages
Typical Application
Description
Boost, Flyback, SEPIC and
Inverting Controller
The LT®3757/LT3757A are wide input range, current
mode, DC/DC controllers which are capable of generating
either positive or negative output voltages. They can be
configured as either a boost, flyback, SEPIC or inverting
converter. The LT3757/LT3757A drive a low side external
N-channel power MOSFET from an internal regulated 7.2V
supply. The fixed frequency, current-mode architecture
results in stable operation over a wide range of supply
and output voltages.
The operating frequency of LT3757/LT3757A can be set
with an external resistor over a 100kHz to 1MHz range,
and can be synchronized to an external clock using the
SYNC pin. A low minimum operating supply voltage of
2.9V, and a low shutdown quiescent current of less than
1μA, make the LT3757/LT3757A ideally suited for batteryoperated
systems.
The LT3757/LT3757A feature soft-start and frequency
foldback functions to limit inductor current during start-up
and output short-circuit. The LT3757A has improved load
transient performance compared to the LT3757.
High Efficiency Boost Converter
Features
Applications
n Automotive and Industrial Boost, Flyback, SEPIC and
Inverting Converters
n Telecom Power Supplies
n Portable Electronic Equipment
Efficiency
SENSE
LT3757
VIN
VIN
8V TO 16V 10μF
25V
X5R
VOUT
24V
2A
0.01
41.2k
300kHz
GATE
FBX
GND INTVCC
SHDN/UVLO
SYNC
RT
SS
VC
200k
43.2k
0.1μF
22k
6.8nF
10μH
3757 TA01a
226k
16.2k
4.7μF
10V
X5R
10μF
25V
X5R
47μF
35V
×2
+
OUTPUT CURRENT (A)
0.001
EFFICIENCY (%)
30
50
40
60
70
80
90
100
0.01 0.1 1
3757 TA01b
10
VIN = 8V
VIN = 16V
L, LT, LTC, LTM, Linear Technology, the Linear logo and Burst Mode are registered trademarks
and No RSENSE and ThinSOT are trademarks of Linear Technology Corporation. All other
trademarks are the property of their respective owners.
LT3757/LT3757A
2
3757afd
Pin Configuration
Absolute Maximum Ratings
VIN, SHDN/UVLO (Note 6)..........................................40V
INTVCC.....................................................VIN + 0.3V, 20V
GATE......................................................... INTVCC + 0.3V
SYNC...........................................................................8V
VC, SS..........................................................................3V
RT.............................................................................1.5V
SENSE.....................................................................±0.3V
FBX.................................................................. –6V to 6V
(Note 1)
TOP VIEW
DD PACKAGE
10-LEAD (3mm × 3mm) PLASTIC DFN
10
9
6
7
8
4
5
3 11
2
1 VIN
SHDN/UVLO
INTVCC
GATE
SENSE
VC
FBX
SS
RT
SYNC
TJMAX = 125°C, θJA = 43°C/W
EXPOSED PAD (PIN 11) IS GND, MUST BE SOLDERED TO PCB
12345
VC
FBX
SS
RT
SYNC
10
9876
VIN
SHDN/UVLO
INTVCC
GATE
SENSE
TOP VIEW
MSE PACKAGE
10-LEAD PLASTIC MSOP
11
TJMAX = 150°C, θJA = 40°C/W
EXPOSED PAD (PIN 11) IS GND, MUST BE SOLDERED TO PCB
Order Information
LEAD FREE FINISH TAPE AND REEL PART MARKING* PACKAGE DESCRIPTION TEMPERATURE RANGE
LT3757EDD#PBF LT3757EDD#TRPBF LDYW 10-Lead (3mm × 3mm) Plastic DFN –40°C to 125°C
LT3757IDD#PBF LT3757IDD#TRPBF LDYW 10-Lead (3mm × 3mm) Plastic DFN –40°C to 125°C
LT3757EMSE#PBF LT3757EMSE#TRPBF LTDYX 10-Lead (3mm × 3mm) Plastic MSOP –40°C to 125°C
LT3757IMSE#PBF LT3757IMSE#TRPBF LTDYX 10-Lead (3mm × 3mm) Plastic MSOP –40°C to 125°C
LT3757HMSE#PBF LT3757HMSE#TRPBF LTDYX 10-Lead (3mm × 3mm) Plastic MSOP –40°C to 150°C
LT3757MPMSE#PBF LT3757MPMSE#TRPBF LTDYX 10-Lead (3mm × 3mm) Plastic MSOP –55°C to 150°C
LT3757AEDD#PBF LT3757AEDD#TRPBF LGGR 10-Lead (3mm × 3mm) Plastic DFN –40°C to 125°C
LT3757AIDD#PBF LT3757AIDD#TRPBF LGGR 10-Lead (3mm × 3mm) Plastic DFN –40°C to 125°C
LT3757AEMSE#PBF LT3757AEMSE#TRPBF LTGGM 10-Lead (3mm × 3mm) Plastic MSOP –40°C to 125°C
LT3757AIMSE#PBF LT3757AIMSE#TRPBF LTGGM 10-Lead (3mm × 3mm) Plastic MSOP –40°C to 125°C
LT3757AHMSE#PBF LT3757AHMSE#TRPBF LTGGM 10-Lead (3mm × 3mm) Plastic MSOP –40°C to 150°C
LT3757AMPMSE#PBF LT3757AMPMSE#TRPBF LTGGM 10-Lead (3mm × 3mm) Plastic MSOP –55°C to 150°C
Consult LTC Marketing for parts specified with wider operating temperature ranges. *The temperature grade is identified by a label on the shipping container.
For more information on lead free part marking, go to: http://www.linear.com/leadfree/
For more information on tape and reel specifications, go to: http://www.linear.com/tapeandreel/
Operating Temperature Range (Notes 2, 8)
LT3757E/LT3757AE............................ –40°C to 125°C
LT3757I/LT3757AI.............................. –40°C to 125°C
LT3757H/LT3757AH............................ –40°C to 150°C
LT3757MP/LT3757AMP...................... –55°C to 150°C
Storage Temperature Range
DFN..................................................... –65°C to 125°C
MSOP................................................. –65°C to 150°C
Lead Temperature (Soldering, 10 sec)
MSOP................................................................300°C
LT3757/LT3757A
3
3757afd
E lectrical Characteristics The l denotes the specifications which apply over the full operating temperature
range, otherwise specifications are at TA = 25°C. VIN = 24V, SHDN/UVLO = 24V, SENSE = 0V, unless otherwise noted.
PARAMETER CONDITIONS MIN TYP MAX UNITS
VIN Operating Range 2.9 40 V
VIN Shutdown IQ SHDN/UVLO = 0V
SHDN/UVLO = 1.15V
0.1 1
6
μA
μA
VIN Operating IQ VC = 0.3V, RT = 41.2k 1.6 2.2 mA
VIN Operating IQ with Internal LDO Disabled VC = 0.3V, RT = 41.2k, INTVCC = 7.5V 280 400 μA
SENSE Current Limit Threshold l 100 110 120 mV
SENSE Input Bias Current Current Out of Pin –65 μA
Error Amplifier
FBX Regulation Voltage (VFBX(REG)) VFBX > 0V (Note 3)
VFBX < 0V (Note 3)
l
l
1.569
–0.816
1.6
–0.80
1.631
–0.784
V
V
FBX Overvoltage Lockout VFBX > 0V (Note 4)
VFBX < 0V (Note 4)
6
7
8
11
10
14
%
%
FBX Pin Input Current VFBX = 1.6V (Note 3)
VFBX = –0.8V (Note 3)
–10
70 100
10
nA
nA
Transconductance gm (ΔIVC/ΔVFBX) (Note 3) 230 μS
VC Output Impedance (Note 3) 5 MΩ
VFBX Line Regulation [ΔVFBX /(ΔVIN • VFBX(REG))] VFBX > 0V, 2.9V < VIN < 40V (Notes 3, 7)
VFBX < 0V, 2.9V < VIN < 40V (Notes 3, 7)
0.002
0.0025
0.056
0.05
%/V
%/V
VC Current Mode Gain (ΔVVC /ΔVSENSE) 5.5 V/V
VC Source Current VFBX = 0V, VC = 1.5V –15 μA
VC Sink Current VFBX = 1.7V
VFBX = –0.85V
12
11
μA
μA
Oscillator
Switching Frequency RT = 41.2k to GND, VFBX = 1.6V
RT = 140k to GND, VFBX = 1.6V
RT = 10.5k to GND, VFBX = 1.6V
270 300
100
1000
330 kHz
kHz
kHz
RT Voltage VFBX = 1.6V 1.2 V
Minimum Off-Time 220 ns
Minimum On-Time 220 ns
SYNC Input Low 0.4 V
SYNC Input High 1.5 V
SS Pull-Up Current SS = 0V, Current Out of Pin –10 μA
Low Dropout Regulator
INTVCC Regulation Voltage l 7 7.2 7.4 V
INTVCC Undervoltage Lockout Threshold Falling INTVCC
UVLO Hysteresis
2.6 2.7
0.1
2.8 V
V
INTVCC Overvoltage Lockout Threshold 16 17.5 V
INTVCC Current Limit VIN = 40V
VIN = 15V
30 40
95
55 mA
mA
INTVCC Load Regulation (ΔVINTVCC/ VINTVCC) 0 < IINTVCC < 20mA, VIN = 8V –0.9 –0.5 %
INTVCC Line Regulation ΔVINTVCC/(VINTVCC • ΔVIN) 8V < VIN < 40V 0.008 0.03 %/V
Dropout Voltage (VIN – VINTVCC) VIN = 6V, IINTVCC = 20mA 400 mV
INTVCC Current in Shutdown SHDN/UVLO = 0V, INTVCC = 8V 16 μA
LT3757/LT3757A
4
3757afd
TEMPERATURE (°C)
–75 –50
1580
1585
REGULATED FEEDBACK VOLTAGE (mV)
1590
1605
1600
0 50 75
1595
–25 25 100 125 150
3757 G01
VIN = 40V
VIN = 24V
VIN = 8V
VIN = INTVCC = 2.9V
SHDN/UVLO = 1.33V
TEMPERATURE (°C)
REGULATED FEEDBACK VOLTAGE (mV)
–802
–800
–798
–788
–790
–792
–794
–804
–796
3757 G02
–75 –50 –25 0 25 50 75 100 125 150
VIN = 40V
VIN = 24V
VIN = 8V
VIN = INTVCC = 2.9V
SHDN/UVLO = 1.33V
Typical Performance Characteristics
Positive Feedback Voltage
vs Temperature, VIN
Negative Feedback Voltage
vs Temperature, VIN
Quiescent Current
vs Temperature, VIN
TA = 25°C, unless otherwise noted.
E lectrical Characteristics The l denotes the specifications which apply over the full operating temperature
range, otherwise specifications are at TA = 25°C. VIN = 24V, SHDN/UVLO = 24V, SENSE = 0V, unless otherwise noted.
PARAMETER CONDITIONS MIN TYP MAX UNITS
INTVCC Voltage to Bypass Internal LDO 7.5 V
Logic Inputs
SHDN/UVLO Threshold Voltage Falling VIN = INTVCC = 8V l 1.17 1.22 1.27 V
SHDN/UVLO Input Low Voltage I(VIN) Drops Below 1μA 0.4 V
SHDN/UVLO Pin Bias Current Low SHDN/UVLO = 1.15V 1.7 2 2.5 μA
SHDN/UVLO Pin Bias Current High SHDN/UVLO = 1.30V 10 100 nA
Gate Driver
tr Gate Driver Output Rise Time CL = 3300pF (Note 5), INTVCC = 7.5V 22 ns
tf Gate Driver Output Fall Time CL = 3300pF (Note 5), INTVCC = 7.5V 20 ns
Gate VOL 0.05 V
Gate VOH INTVCC –0.05 V
Note 1: Stresses beyond those listed under Absolute Maximum Ratings
may cause permanent damage to the device. Exposure to any Absolute
Maximum Rating condition for extended periods may affect device
reliability and lifetime.
Note 2: The LT3757E/LT3757AE are guaranteed to meet performance
specifications from the 0°C to 125°C junction temperature. Specifications
over the –40°C to 125°C operating junction temperature range are
assured by design, characterization and correlation with statistical process
controls. The LT3757I/LT3757AI are guaranteed over the full –40°C to
125°C operating junction temperature range. The LT3757H/LT3757AH are
guaranteed over the full –40°C to 150°C operating junction temperature
range. High junction temperatures degrade operating lifetimes. Operating
lifetime is derated at junction temperatures greater than 125°C. The
LT3757MP/LT3757AMP are 100% tested and guaranteed over the full
–55°C to 150°C operating junction temperature range.
Note 3: The LT3757/LT3757A are tested in a feedback loop which servos
VFBX to the reference voltages (1.6V and –0.8V) with the VC pin forced
to 1.3V.
Note 4: FBX overvoltage lockout is measured at VFBX(OVERVOLTAGE) relative
to regulated VFBX(REG).
Note 5: Rise and fall times are measured at 10% and 90% levels.
Note 6: For VIN below 6V, the SHDN/UVLO pin must not exceed VIN.
Note 7: SHDN/UVLO = 1.33V when VIN = 2.9V.
Note 8: The LT3757/LT3757A include overtemperature protection that
is intended to protect the device during momentary overload conditions.
Junction temperature will exceed the maximum operating junction
temperature when overtemperature protection is active. Continuous
operation above the specified maximum operating junction temperature
may impair device reliability.
–75 –50 –25 0 25 50 75 100 125 150
TEMPERATURE (°C)
1.4
QUIESCENT CURRENT (mA)
1.6
1.8
1.5
1.7
3757 G03
VIN = 40V
VIN = 24V
VIN = INTVCC = 2.9V
LT3757/LT3757A
5
3757afd
Typical Performance Characteristics
Switching Frequency
vs Temperature
SENSE Current Limit Threshold
vs Temperature
SENSE Current Limit Threshold
vs Duty Cycle
SHDN/UVLO Threshold
vs Temperature SHDN/UVLO Current vs Voltage
SHDN/UVLO Hysteresis Current
vs Temperature
Dynamic Quiescent Current
vs Switching Frequency RT vs Switching Frequency
Normalized Switching Frequency
vs FBX
TA = 25°C, unless otherwise noted.
FBX VOLTAGE (V)
–0.8
0
NORMALIZED FREQUENCY (%)
20
40
60
80
120
–0.4 0 0.4 0.8
3757 G06
1.2 1.6
100
–75 –50 –25 0 25 50 75 100 125 150
TEMPERATURE (°C)
100
SENSE THRESHOLD (mV)
105
110
115
120
3757 G08
DUTY CYCLE (%)
0
95
SENSE THRESHOLD (mV)
105
20 40 60 80
115
100
110
100
3757 G09
SHDN/UVLO VOLTAGE (V)
0
0
SHDN/UVLO CURRENT (μA)
20
10 20 30
40
10
30
40
3757 G11
–75 –50 –25 0 25 50 75 100 125 150
TEMPERATURE (°C)
1.6
ISHDN/UVLO (μA)
1.8
2.0
2.2
2.4
3757 G12
SWITCHING FREQUENCY (KHz)
0
0
IQ(mA)
15
20
35
300 500 600 700
10
5
25
30
100 200 400 800 900 1000
3757 G04
CL = 3300pF
SWITCHING FREQUENCY (KHz)
0
10
RT (k)
100
1000
100 200 300 400 500 600 700 800 900 1000
3757 G05
–75 –50 –25 0 25 50 75 100 125 150
TEMPERATURE (°C)
270
SWITCHING FREQUENCY (kHz)
280
290
300
310
330
3757 G07
320
RT = 41.2K
–75 –50 –25 0 25 50 75 100 125 150
TEMPERATURE (°C)
1.18
SHDN/UVLO VOLTAGE (V)
1.22
1.24
1.26
1.28
1.20
3757 G10
SHDN/UVLO FALLING
SHDN/UVLO RISING
LT3757/LT3757A
6
3757afd
Typical Performance Characteristics
INTVCC Line Regulation
INTVCC Dropout Voltage
vs Current, Temperature
Gate Drive Rise
and Fall Time vs INTVCC Typical Start-Up Waveforms
INTVCC vs Temperature
INTVCC Minimum Output Current
vs VIN INTVCC Load Regulation
TA = 25°C, unless otherwise noted.
Gate Drive Rise
and Fall Time vs CL
FBX Frequency Foldback
Waveforms During Overcurrent
–75 –50 –25 0 25 50 75 100 125 150
TEMPERATURE (°C)
7.0
INTVCC (V)
7.1
7.2
7.3
7.4
3757 G13
VIN (V)
0
INTVCC VOLTAGE (V)
35
7.25
7.20
5 10 15 20 25 30 40
7.15
7.10
7.30
3757 G16
CL (nF)
0
TIME (ns)
60
70
80
50
40
5 10 15 20 25 30
10
0
30
90
20
3757 G18
RISE TIME
INTVCC = 7.2V
FALL TIME
INTVCC (V)
3
TIME (ns)
20
25
15
10
6 9 12 15
5
0
30
3757 G19
CL = 3300pF
RISE TIME
FALL TIME
2ms/DIV
VOUT
5V/DIV
IL1A + IL1B
5A/DIV
3757 G20
VIN = 12V
PAGE 31 CIRCUIT
50μs/DIV
PAGE 31 CIRCUIT
VOUT
10V/DIV
VSW
20V/DIV
IL1A + IL1B
5A/DIV
3757 G21
VIN = 12V
INTVCC LOAD (mA)
0
6.8
7
7.1
7.2
7.3
20 40 50 60
6.9
10 30 70
3757 G15
INTVCC VOLTAGE (V)
VIN = 8V
INTVCC LOAD (mA)
0
DROPOUT VOLTAGE (mV)
500
600
300
400
200
5 10 15 20
100
0
700
3757 G17
150°C
125°C
25°C
0°C
–55°C
75°C
VIN = 6V
VIN (V)
0
INTVCC CURRENT (mA)
50
60
70
40
3757 G14
40
30
0
10
5 10 15 20 25 30 35
20
90
80
TJ = 150°C
INTVCC = 6V
INTVCC = 4.5V
LT3757/LT3757A
7
3757afd
Pin Functions
VC (Pin 1): Error Amplifier Compensation Pin. Used to
stabilize the voltage loop with an external RC network.
FBX (Pin 2): Positive and Negative Feedback Pin. Receives
the feedback voltage from the external resistor divider
across the output. Also modulates the frequency during
start-up and fault conditions when FBX is close to GND.
SS (Pin 3): Soft-Start Pin. This pin modulates compensation
pin voltage (VC) clamp. The soft-start interval is set with
an external capacitor. The pin has a 10μA (typical) pull-up
current source to an internal 2.5V rail. The soft-start pin
is reset to GND by an undervoltage condition at SHDN/
UVLO, an INTVCC undervoltage or overvoltage condition
or an internal thermal lockout.
RT (Pin 4): Switching Frequency Adjustment Pin. Set the
frequency using a resistor to GND. Do not leave this pin
open.
SYNC (Pin 5): Frequency Synchronization Pin. Used to
synchronize the switching frequency to an outside clock.
If this feature is used, an RT resistor should be chosen to
program a switching frequency 20% slower than the SYNC
pulse frequency. Tie the SYNC pin to GND if this feature
is not used. SYNC is ignored when FBX is close to GND.
SENSE (Pin 6): The Current Sense Input for the Control
Loop. Kelvin connect this pin to the positive terminal of
the switch current sense resistor in the source of the
N-channel MOSFET. The negative terminal of the current
sense resistor should be connected to GND plane close
to the IC.
GATE (Pin 7): N-Channel MOSFET Gate Driver Output.
Switches between INTVCC and GND. Driven to GND when
IC is shut down, during thermal lockout or when INTVCC
is above or below the OV or UV thresholds, respectively.
INTVCC (Pin 8): Regulated Supply for Internal Loads and
Gate Driver. Supplied from VIN and regulated to 7.2V (typical).
INTVCC must be bypassed with a minimum of 4.7μF
capacitor placed close to pin. INTVCC can be connected
directly to VIN, if VIN is less than 17.5V. INTVCC can also
be connected to a power supply whose voltage is higher
than 7.5V, and lower than VIN, provided that supply does
not exceed 17.5V.
SHDN/UVLO (Pin 9): Shutdown and Undervoltage Detect
Pin. An accurate 1.22V (nominal) falling threshold with
externally programmable hysteresis detects when power
is okay to enable switching. Rising hysteresis is generated
by the external resistor divider and an accurate internal
2μA pull-down current. An undervoltage condition resets
sort-start. Tie to 0.4V, or less, to disable the device and
reduce VIN quiescent current below 1μA.
VIN (Pin 10): Input Supply Pin. Must be locally bypassed
with a 0.22μF, or larger, capacitor placed close to the pin.
Exposed Pad (Pin 11): Ground. This pin also serves as the
negative terminal of the current sense resistor. The Exposed
Pad must be soldered directly to the local ground plane.
LT3757/LT3757A
8
3757afd
Block Diagram
Figure 1. LT3757 Block Diagram Working as a SEPIC Converter
L1
R1
R4 R3
M1
L2 R2
FBX
1.22V
2.5V
CDC D1
CIN
VOUT
COUT2
COUT1
CVCC
INTVCC
VIN
RSENSE
VISENSE
• +
+
VIN
IS1
2μA
10
8
7
1
9
SHDN/UVLO
INTERNAL
REGULATOR
AND UVLO
TSD
165°C
A10
Q3
VC
VC
17.5V
2.7V UP
2.6V DOWN
A8
UVLO
IS2
10μA
IS3
CC1
CC2 RC
DRIVER
SLOPE
SENSE
GND
GATE
108mV
SR1
+
–
+
–
CURRENT
LIMIT
RAMP
GENERATOR
7.2V LDO
•
+
–
+
–
R O
S
2.5V
G1
RT
RT
SS
CSS
SYNC
1.25V
1.25V
FBX
FBX
1.6V
–0.8V
+
–
+
–
+
–
2
3 5 4
+
–
+
–
6
11
RAMP
PWM
COMPARATOR
FREQUENCY
FOLDBACK
100kHz-1MHz
OSCILLATOR
FREQ
FOLDBACK
FREQ
PROG
3757 F01
–
++
Q1
A1
A2
1.72V
–0.88V
+
–
+
–
A11
A12
A3
A4
A5
A6
G5 G2
G6
A7
A9
Q2
D2
R5
8k D3
G4 G3
LT3757/LT3757A
9
3757afd
Applications Information
Main Control Loop
The LT3757 uses a fixed frequency, current mode control
scheme to provide excellent line and load regulation. Operation
can be best understood by referring to the Block
Diagram in Figure 1.
The start of each oscillator cycle sets the SR latch (SR1) and
turns on the external power MOSFET switch M1 through
driver G2. The switch current flows through the external
current sensing resistor RSENSE and generates a voltage
proportional to the switch current. This current sense
voltage VISENSE (amplified by A5) is added to a stabilizing
slope compensation ramp and the resulting sum (SLOPE)
is fed into the positive terminal of the PWM comparator A7.
When SLOPE exceeds the level at the negative input of A7
(VC pin), SR1 is reset, turning off the power switch. The
level at the negative input of A7 is set by the error amplifier
A1 (or A2) and is an amplified version of the difference
between the feedback voltage (FBX pin) and the reference
voltage (1.6V or –0.8V, depending on the configuration).
In this manner, the error amplifier sets the correct peak
switch current level to keep the output in regulation.
The LT3757 has a switch current limit function. The current
sense voltage is input to the current limit comparator A6.
If the SENSE pin voltage is higher than the sense current
limit threshold VSENSE(MAX) (110mV, typical), A6 will reset
SR1 and turn off M1 immediately.
The LT3757 is capable of generating either positive or
negative output voltage with a single FBX pin. It can be
configured as a boost, flyback or SEPIC converter to generate
positive output voltage, or as an inverting converter
to generate negative output voltage. When configured as
a SEPIC converter, as shown in Figure 1, the FBX pin is
pulled up to the internal bias voltage of 1.6V by a voltage
divider (R1 and R2) connected from VOUT to GND.
Comparator A2 becomes inactive and comparator A1
performs the inverting amplification from FBX to VC. When
the LT3757 is in an inverting configuration, the FBX pin
is pulled down to –0.8V by a voltage divider connected
from VOUT to GND. Comparator A1 becomes inactive and
comparator A2 performs the noninverting amplification
from FBX to VC.
The LT3757 has overvoltage protection functions to
protect the converter from excessive output voltage
overshoot during start-up or recovery from a short-circuit
condition. An overvoltage comparator A11 (with 20mV
hysteresis) senses when the FBX pin voltage exceeds the
positive regulated voltage (1.6V) by 8% and provides a
reset pulse. Similarly, an overvoltage comparator A12
(with 10mV hysteresis) senses when the FBX pin voltage
exceeds the negative regulated voltage (–0.8V) by 11%
and provides a reset pulse. Both reset pulses are sent to
the main RS latch (SR1) through G6 and G5. The power
MOSFET switch M1 is actively held off for the duration of
an output overvoltage condition.
Programming Turn-On and Turn-Off Thresholds with
the SHDN/UVLO Pin
The SHDN/UVLO pin controls whether the LT3757 is
enabled or is in shutdown state. A micropower 1.22V
reference, a comparator A10 and a controllable current
source IS1 allow the user to accurately program the supply
voltage at which the IC turns on and off. The falling value
can be accurately set by the resistor dividers R3 and R4.
When SHDN/UVLO is above 0.7V, and below the 1.22V
threshold, the small pull-down current source IS1 (typical
2μA) is active.
The purpose of this current is to allow the user to program
the rising hysteresis. The Block Diagram of the comparator
and the external resistors is shown in Figure 1. The typical
falling threshold voltage and rising threshold voltage can
be calculated by the following equations:
VVIN,FALLING = 1.22 •
(R3 +R4)
R4
VVIN,RISING = 2μA •R3+ VIN,FALLING
For applications where the SHDN/UVLO pin is only used
as a logic input, the SHDN/UVLO pin can be connected
directly to the input voltage VIN for always-on operation.
LT3757/LT3757A
10
3757afd
Applications Information
INTVCC Regulator Bypassing and Operation
An internal, low dropout (LDO) voltage regulator produces
the 7.2V INTVCC supply which powers the gate driver,
as shown in Figure 1. If a low input voltage operation is
expected (e.g., supplying power from a lithium-ion battery
or a 3.3V logic supply), low threshold MOSFETs should
be used. The LT3757 contains an undervoltage lockout
comparator A8 and an overvoltage lockout comparator
A9 for the INTVCC supply. The INTVCC undervoltage (UV)
threshold is 2.7V (typical), with 100mV hysteresis, to
ensure that the MOSFETs have sufficient gate drive voltage
before turning on. The logic circuitry within the LT3757 is
also powered from the internal INTVCC supply.
The INTVCC overvoltage (OV) threshold is set to be 17.5V
(typical) to protect the gate of the power MOSFET. When
INTVCC is below the UV threshold, or above the OV threshold,
the GATE pin will be forced to GND and the soft-start
operation will be triggered.
The INTVCC regulator must be bypassed to ground immediately
adjacent to the IC pins with a minimum of 4.7μF ceramic
capacitor. Good bypassing is necessary to supply the
high transient currents required by the MOSFET gate driver.
In an actual application, most of the IC supply current is
used to drive the gate capacitance of the power MOSFET.
The on-chip power dissipation can be a significant concern
when a large power MOSFET is being driven at a high frequency
and the VIN voltage is high. It is important to limit
the power dissipation through selection of MOSFET and/
or operating frequency so the LT3757 does not exceed its
maximum junction temperature rating. The junction temperature
TJ can be estimated using the following equations:
TJ = TA + PIC • θJA
TA = ambient temperature
θJA = junction-to-ambient thermal resistance
PIC = IC power consumption
= VIN • (IQ + IDRIVE)
IQ = VIN operation IQ = 1.6mA
IDRIVE = average gate drive current = f • QG
f = switching frequency
QG = power MOSFET total gate charge
The LT3757 uses packages with an Exposed Pad for enhanced
thermal conduction. With proper soldering to the
Exposed Pad on the underside of the package and a full
copper plane underneath the device, thermal resistance
(θJA) will be about 43°C/W for the DD package and 40°C/W
for the MSE package. For an ambient board temperature of
TA = 70°C and maximum junction temperature of 125°C,
the maximum IDRIVE (IDRIVE(MAX)) of the DD package can
be calculated as:
IDRIVE(MAX) =
(TJ − TA)
(θJA • VIN)
−IQ =
1.28W
VIN
− 1.6mA
The LT3757 has an internal INTVCC IDRIVE current limit
function to protect the IC from excessive on-chip power
dissipation. The IDRIVE current limit decreases as the VIN
increases (see the INTVCC Minimum Output Current vs VIN
graph in the Typical Performance Characteristics section).
If IDRIVE reaches the current limit, INTVCC voltage will fall
and may trigger the soft-start.
Based on the preceding equation and the INTVCC Minimum
Output Current vs VIN graph, the user can calculate the
maximum MOSFET gate charge the LT3757 can drive at
a given VIN and switch frequency. A plot of the maximum
QG vs VIN at different frequencies to guarantee a minimum
4.5V INTVCC is shown in Figure 2.
As illustrated in Figure 2, a trade-off between the operating
frequency and the size of the power MOSFET may be needed
in order to maintain a reliable IC junction temperature.
Figure 2. Recommended Maximum QG vs VIN at Different
Frequencies to Ensure INTVCC Higher Than 4.5V
VIN (V)
0
QG (nC)
200
250
150
100
5 10 15 20 25 30 35 40
50
0
300
3757 F02
300kHz
1MHz
LT3757/LT3757A
11
3757afd
Applications Information
Prior to lowering the operating frequency, however, be
sure to check with power MOSFET manufacturers for their
most recent low QG, low RDS(ON) devices. Power MOSFET
manufacturing technologies are continually improving, with
newer and better performance devices being introduced
almost yearly.
An effective approach to reduce the power consumption
of the internal LDO for gate drive is to tie the INTVCC pin
to an external voltage source high enough to turn off the
internal LDO regulator.
If the input voltage VIN does not exceed the absolute
maximum rating of both the power MOSFET gate-source
voltage (VGS) and the INTVCC overvoltage lockout threshold
voltage (17.5V), the INTVCC pin can be shorted directly
to the VIN pin. In this condition, the internal LDO will be
turned off and the gate driver will be powered directly
from the input voltage, VIN. With the INTVCC pin shorted to
VIN, however, a small current (around 16μA) will load the
INTVCC in shutdown mode. For applications that require
the lowest shutdown mode input supply current, do not
connect the INTVCC pin to VIN.
In SEPIC or flyback applications, the INTVCC pin can be
connected to the output voltage VOUT through a blocking
diode, as shown in Figure 3, if VOUT meets the following
conditions:
1. VOUT < VIN (pin voltage)
2. VOUT < 17.5V
3. VOUT < maximum VGS rating of power MOSFET
A resistor RVCC can be connected, as shown in Figure 3, to
limit the inrush current from VOUT. Regardless of whether
or not the INTVCC pin is connected to an external voltage
source, it is always necessary to have the driver circuitry
bypassed with a 4.7μF low ESR ceramic capacitor to
ground immediately adjacent to the INTVCC and GND pins.
Figure 3. Connecting INTVCC to VOUT
CVCC
4.7μF
VOUT
3757 F03
INTVCC
GND
LT3757 RVCC
DVCC
Operating Frequency and Synchronization
The choice of operating frequency may be determined
by on-chip power dissipation, otherwise it is a trade-off
between efficiency and component size. Low frequency
operation improves efficiency by reducing gate drive current
and MOSFET and diode switching losses. However,
lower frequency operation requires a physically larger
inductor. Switching frequency also has implications for
loop compensation. The LT3757 uses a constant-frequency
architecture that can be programmed over a 100kHz to
1000kHz range with a single external resistor from the
RT pin to ground, as shown in Figure 1. The RT pin must
have an external resistor to GND for proper operation of
the LT3757. A table for selecting the value of RT for a given
operating frequency is shown in Table 1.
Table 1. Timing Resistor (RT) Value
OSCILLATOR FREQUENCY (kHz) RT (kΩ)
100 140
200 63.4
300 41.2
400 30.9
500 24.3
600 19.6
700 16.5
800 14
900 12.1
1000 10.5
The operating frequency of the LT3757 can be synchronized
to an external clock source. By providing a digital clock
signal into the SYNC pin, the LT3757 will operate at the
SYNC clock frequency. If this feature is used, an RT resistor
should be chosen to program a switching frequency 20%
slower than SYNC pulse frequency. The SYNC pulse should
have a minimum pulse width of 200ns. Tie the SYNC pin
to GND if this feature is not used.
LT3757/LT3757A
12
3757afd
Applications Information
Duty Cycle Consideration
Switching duty cycle is a key variable defining converter
operation. As such, its limits must be considered. Minimum
on-time is the smallest time duration that the LT3757 is
capable of turning on the power MOSFET. This time is
generally about 220ns (typical) (see Minimum On-Time
in the Electrical Characteristics table). In each switching
cycle, the LT3757 keeps the power switch off for at least
220ns (typical) (see Minimum Off-Time in the Electrical
Characteristics table).
The minimum on-time and minimum off-time and the
switching frequency define the minimum and maximum
switching duty cycles a converter is able to generate:
Minimum duty cycle = minimum on-time • frequency
Maximum duty cycle = 1 – (minimum off-time • frequency)
Programming the Output Voltage
The output voltage (VOUT) is set by a resistor divider, as
shown in Figure 1. The positive and negative VOUT are set
by the following equations:
VOUT,POSITIVE = 1.6V • 1+
R2
R1
VOUT,NEGATIVE = –0.8V • 1+
R2
R1
The resistors R1 and R2 are typically chosen so that
the error caused by the current flowing into the FBX pin
during normal operation is less than 1% (this translates
to a maximum value of R1 at about 158k).
In the applications where VOUT is pulled up by an external
positive power supply, the FBX pin is also pulled up through
the R2 and R1 network. Make sure the FBX does not exceed
its absolute maximum rating (6V). The R5, D2, and D3 in
Figure 1 provide a resistive clamp in the positive direction.
To ensure FBX is lower than 6V, choose sufficiently large
R1 and R2 to meet the following condition:
6V • 1+
R2
R1
+
3.5V •
R2
8kΩ
> VOUT(MAX)
where VOUT(MAX) is the maximum VOUT that is pulled up
by an external power supply.
Soft-Start
The LT3757 contains several features to limit peak switch
currents and output voltage (VOUT) overshoot during
start-up or recovery from a fault condition. The primary
purpose of these features is to prevent damage to external
components or the load.
High peak switch currents during start-up may occur in
switching regulators. Since VOUT is far from its final value,
the feedback loop is saturated and the regulator tries to
charge the output capacitor as quickly as possible, resulting
in large peak currents. A large surge current may cause
inductor saturation or power switch failure.
The LT3757 addresses this mechanism with the SS pin. As
shown in Figure 1, the SS pin reduces the power MOSFET
current by pulling down the VC pin through Q2. In this
way the SS allows the output capacitor to charge gradually
toward its final value while limiting the start-up peak
currents. The typical start-up waveforms are shown in the
Typical Performance Characteristics section. The inductor
current IL slewing rate is limited by the soft-start function.
Besides start-up, soft-start can also be triggered by the
following faults:
1. INTVCC > 17.5V
2. INTVCC < 2.6V
3. Thermal lockout
Any of these three faults will cause the LT3757 to stop
switching immediately. The SS pin will be discharged by
Q3. When all faults are cleared and the SS pin has been
discharged below 0.2V, a 10μA current source IS2 starts
charging the SS pin, initiating a soft-start operation.
The soft-start interval is set by the soft-start capacitor
selection according to the equation:
TSS =CSS •
1.25V
10μA
LT3757/LT3757A
13
3757afd
Applications Information
FBX Frequency Foldback
When VOUT is very low during start-up or a short-circuit
fault on the output, the switching regulator must operate
at low duty cycles to maintain the power switch current
within the current limit range, since the inductor current
decay rate is very low during switch off time. The minimum
on-time limitation may prevent the switcher from attaining
a sufficiently low duty cycle at the programmed switching
frequency. So, the switch current will keep increasing
through each switch cycle, exceeding the programmed
current limit. To prevent the switch peak currents from
exceeding the programmed value, the LT3757 contains
a frequency foldback function to reduce the switching
frequency when the FBX voltage is low (see the Normalized
Switching Frequency vs FBX graph in the Typical
Performance Characteristics section).
The typical frequency foldback waveforms are shown
in the Typical Performance Characteristics section. The
frequency foldback function prevents IL from exceeding
the programmed limits because of the minimum on-time.
During frequency foldback, external clock synchronization
is disabled to prevent interference with frequency
reducing operation.
Thermal Lockout
If LT3757 die temperature reaches 165°C (typical), the
part will go into thermal lockout. The power switch will
be turned off. A soft-start operation will be triggered. The
part will be enabled again when the die temperature has
dropped by 5°C (nominal).
Loop Compensation
Loop compensation determines the stability and transient
performance. The LT3757/LT3757A use current mode
control to regulate the output which simplifies loop compensation.
The LT3757A improves the no-load to heavy
load transient response, when compared to the LT3757.
New internal circuits ensure that the transient from not
switching to switching at high current can be made in a
few cycles.
The optimum values depend on the converter topology, the
component values and the operating conditions (including
the input voltage, load current, etc.). To compensate the
feedback loop of the LT3757/LT3757A, a series resistorcapacitor
network is usually connected from the VC pin to
GND. Figure 1 shows the typical VC compensation network.
For most applications, the capacitor should be in the range
of 470pF to 22nF, and the resistor should be in the range
of 5k to 50k. A small capacitor is often connected in parallel
with the RC compensation network to attenuate the
VC voltage ripple induced from the output voltage ripple
through the internal error amplifier. The parallel capacitor
usually ranges in value from 10pF to 100pF. A practical
approach to design the compensation network is to start
with one of the circuits in this data sheet that is similar
to your application, and tune the compensation network
to optimize the performance. Stability should then be
checked across all operating conditions, including load
current, input voltage and temperature.
SENSE Pin Programming
For control and protection, the LT3757 measures the
power MOSFET current by using a sense resistor (RSENSE)
between GND and the MOSFET source. Figure 4 shows a
typical waveform of the sense voltage (VSENSE) across the
sense resistor. It is important to use Kelvin traces between
the SENSE pin and RSENSE, and to place the IC GND as
close as possible to the GND terminal of the RSENSE for
proper operation.
Figure 4. The Sense Voltage During a Switching Cycle
3757 F04
VSENSE(PEAK)
ΔVSENSE = χ • VSENSE(MAX)
VSENSE
DT t S
VSENSE(MAX)
TS
LT3757/LT3757A
14
3757afd
Applications Information
Due to the current limit function of the SENSE pin, RSENSE
should be selected to guarantee that the peak current sense
voltage VSENSE(PEAK) during steady state normal operation
is lower than the SENSE current limit threshold (see the
Electrical Characteristics table). Given a 20% margin,
VSENSE(PEAK) is set to be 80mV. Then, the maximum
switch ripple current percentage can be calculated using
the following equation:
c =
ΔVSENSE
80mV − 0.5 • ΔVSENSE
c is used in subsequent design examples to calculate inductor
value. ΔVSENSE is the ripple voltage across RSENSE.
The LT3757 switching controller incorporates 100ns timing
interval to blank the ringing on the current sense signal
immediately after M1 is turned on. This ringing is caused
by the parasitic inductance and capacitance of the PCB
trace, the sense resistor, the diode, and the MOSFET. The
100ns timing interval is adequate for most of the LT3757
applications. In the applications that have very large and
long ringing on the current sense signal, a small RC filter
can be added to filter out the excess ringing. Figure 5
shows the RC filter on SENSE pin. It is usually sufficient
to choose 22Ω for RFLT and 2.2nF to 10nF for CFLT.
Keep RFLT’s resistance low. Remember that there is 65μA
(typical) flowing out of the SENSE pin. Adding RFLT will
affect the SENSE current limit threshold:
VSENSE_ILIM = 108mV – 65μA • RFLT
Application Circuits
The LT3757 can be configured as different topologies. The
first topology to be analyzed will be the boost converter,
followed by the flyback, SEPIC and inverting converters.
Boost Converter: Switch Duty Cycle and Frequency
The LT3757 can be configured as a boost converter for
the applications where the converter output voltage is
higher than the input voltage. Remember that boost converters
are not short-circuit protected. Under a shorted
output condition, the inductor current is limited only by
the input supply capability. For applications requiring a
step-up converter that is short-circuit protected, please
refer to the Applications Information section covering
SEPIC converters.
The conversion ratio as a function of duty cycle is
VOUT
VIN
=
1
1−D
in continuous conduction mode (CCM).
For a boost converter operating in CCM, the duty cycle
of the main switch can be calculated based on the output
voltage (VOUT) and the input voltage (VIN). The maximum
duty cycle (DMAX) occurs when the converter has the
minimum input voltage:
DMAX =
VOUT − VIN(MIN)
VOUT
Discontinuous conduction mode (DCM) provides higher
conversion ratios at a given frequency at the cost of reduced
efficiencies and higher switching currents.
Figure 5. The RC Filter on SENSE Pin
CFLT
3757 F05
LT3757
RFLT
RSENSE
M1
SENSE
GATE
GND
LT3757/LT3757A
15
3757afd
Applications Information
Boost Converter: Inductor and Sense Resistor Selection
For the boost topology, the maximum average inductor
current is:
IL(MAX) =IO(MAX) •
1
1−DMAX
Then, the ripple current can be calculated by:
ΔIL = c •IL(MAX) = c •IO(MAX) •
1
1−DMAX
The constant c in the preceding equation represents the
percentage peak-to-peak ripple current in the inductor,
relative to IL(MAX).
The inductor ripple current has a direct effect on the choice
of the inductor value. Choosing smaller values of ΔIL
requires large inductances and reduces the current loop
gain (the converter will approach voltage mode). Accepting
larger values of ΔIL provides fast transient response and
allows the use of low inductances, but results in higher input
current ripple and greater core losses. It is recommended
that c fall within the range of 0.2 to 0.6.
Given an operating input voltage range, and having chosen
the operating frequency and ripple current in the inductor,
the inductor value of the boost converter can be determined
using the following equation:
L =
VIN(MIN)
ΔIL • f
•DMAX
The peak and RMS inductor current are:
IL(PEAK) =IL(MAX) • 1+ c
2
IL(RMS) =IL(MAX) • 1+ c2
12
Based on these equations, the user should choose the
inductors having sufficient saturation and RMS current
ratings.
Set the sense voltage at IL(PEAK) to be the minimum of the
SENSE current limit threshold with a 20% margin. The
sense resistor value can then be calculated to be:
RSENSE =
80mV
IL(PEAK)
Boost Converter: Power MOSFET Selection
Important parameters for the power MOSFET include the
drain-source voltage rating (VDS), the threshold voltage
(VGS(TH)), the on-resistance (RDS(ON)), the gate to source
and gate to drain charges (QGS and QGD), the maximum
drain current (ID(MAX)) and the MOSFET’s thermal
resistances (RθJC and RθJA).
The power MOSFET will see full output voltage, plus a
diode forward voltage, and any additional ringing across
its drain-to-source during its off-time. It is recommended
to choose a MOSFET whose BVDSS is higher than VOUT by
a safety margin (a 10V safety margin is usually sufficient).
The power dissipated by the MOSFET in a boost converter
is:
PFET = I2
L(MAX) • RDS(ON) • DMAX + 2 • V2
OUT • IL(MAX)
• CRSS • f /1A
The first term in the preceding equation represents the
conduction losses in the device, and the second term, the
switching loss. CRSS is the reverse transfer capacitance,
which is usually specified in the MOSFET characteristics.
For maximum efficiency, RDS(ON) and CRSS should be
minimized. From a known power dissipated in the power
MOSFET, its junction temperature can be obtained using
the following equation:
TJ = TA + PFET • θJA = TA + PFET • (θJC + θCA)
TJ must not exceed the MOSFET maximum junction
temperature rating. It is recommended to measure the
MOSFET temperature in steady state to ensure that absolute
maximum ratings are not exceeded.
LT3757/LT3757A
16
3757afd
Applications Information
Figure 6. The Output Ripple Waveform of a Boost Converter
VOUT
(AC)
tON
ΔVESR
RINGING DUE TO
TOTAL INDUCTANCE
(BOARD + CAP)
ΔVCOUT
3757 F05
tOFF
Boost Converter: Output Diode Selection
To maximize efficiency, a fast switching diode with low
forward drop and low reverse leakage is desirable. The
peak reverse voltage that the diode must withstand is
equal to the regulator output voltage plus any additional
ringing across its anode-to-cathode during the on-time.
The average forward current in normal operation is equal
to the output current, and the peak current is equal to:
ID(PEAK) =IL(PEAK) = 1+ c
2
•IL(MAX)
It is recommended that the peak repetitive reverse voltage
rating VRRM is higher than VOUT by a safety margin (a 10V
safety margin is usually sufficient).
The power dissipated by the diode is:
PD = IO(MAX) • VD
and the diode junction temperature is:
TJ = TA + PD • RθJA
The RθJA to be used in this equation normally includes the
RθJC for the device plus the thermal resistance from the
board to the ambient temperature in the enclosure. TJ must
not exceed the diode maximum junction temperature rating.
Boost Converter: Output Capacitor Selection
Contributions of ESR (equivalent series resistance), ESL
(equivalent series inductance) and the bulk capacitance
must be considered when choosing the correct output
capacitors for a given output ripple voltage. The effect of
The choice of component(s) begins with the maximum
acceptable ripple voltage (expressed as a percentage of
the output voltage), and how this ripple should be divided
between the ESR step ΔVESR and the charging/discharging
ΔVCOUT. For the purpose of simplicity, we will choose
2% for the maximum output ripple, to be divided equally
between ΔVESR and ΔVCOUT. This percentage ripple will
change, depending on the requirements of the application,
and the following equations can easily be modified. For a
1% contribution to the total ripple voltage, the ESR of the
output capacitor can be determined using the following
equation:
ESRCOUT ≤
0.01• VOUT
ID(PEAK)
these three parameters (ESR, ESL and bulk C) on the output
voltage ripple waveform for a typical boost converter is
illustrated in Figure 6.
LT3757/LT3757A
17
3757afd
Applications Information
For the bulk C component, which also contributes 1% to
the total ripple:
COUT ≥
IO(MAX)
0.01• VOUT • f
The output capacitor in a boost regulator experiences high
RMS ripple currents, as shown in Figure 6. The RMS ripple
current rating of the output capacitor can be determined
using the following equation:
IRMS(COUT) ≥IO(MAX) •
DMAX
1−DMAX
Multiple capacitors are often paralleled to meet ESR
requirements. Typically, once the ESR requirement is
satisfied, the capacitance is adequate for filtering and has
the required RMS current rating. Additional ceramic capacitors
in parallel are commonly used to reduce the effect of
parasitic inductance in the output capacitor, which reduces
high frequency switching noise on the converter output.
Boost Converter: Input Capacitor Selection
The input capacitor of a boost converter is less critical
than the output capacitor, due to the fact that the inductor
is in series with the input, and the input current waveform
is continuous. The input voltage source impedance
determines the size of the input capacitor, which is typically
in the range of 10μF to 100μF. A low ESR capacitor
is recommended, although it is not as critical as for the
output capacitor.
The RMS input capacitor ripple current for a boost converter
is:
IRMS(CIN) = 0.3 • ΔIL
Flyback Converter Applications
The LT3757 can be configured as a flyback converter
for the applications where the converters have multiple
outputs, high output voltages or isolated outputs. Figure
7 shows a simplified flyback converter.
The flyback converter has a very low parts count for multiple
outputs, and with prudent selection of turns ratio, can
have high output/input voltage conversion ratios with a
desirable duty cycle. However, it has low efficiency due to
the high peak currents, high peak voltages and consequent
power loss. The flyback converter is commonly used for
an output power of less than 50W.
The flyback converter can be designed to operate either
in continuous or discontinuous mode. Compared to continuous
mode, discontinuous mode has the advantage of
smaller transformer inductances and easy loop compensation,
and the disadvantage of higher peak-to-average
current and lower efficiency. In the high output voltage
applications, the flyback converters can be designed
to operate in discontinuous mode to avoid using large
transformers.
Figure 7. A Simplified Flyback Converter
RSENSE
NP:NS
VIN
CIN CSN VSN
LP
D
SUGGESTED
RCD SNUBBER
ID
ISW
VDS
3757 F06
GATE
GND
LT3757
SENSE
LS
M
+
–
+
–
RSN
DSN
–
+
+
COUT
+
LT3757/LT3757A
18
3757afd
Applications Information
Flyback Converter: Switch Duty Cycle and Turns Ratio
The flyback converter conversion ratio in the continuous
mode operation is:
VOUT
VIN
=
NS
NP
•
D
1−D
where NS/NP is the second to primary turns ratio.
Figure 8 shows the waveforms of the flyback converter
in discontinuous mode operation. During each switching
period TS, three subintervals occur: DTS, D2TS, D3TS.
During DTS, M is on, and D is reverse-biased. During
D2TS, M is off, and LS is conducting current. Both LP and
LS currents are zero during D3TS.
The flyback converter conversion ratio in the discontinuous
mode operation is:
VOUT
VIN
=
NS
NP
•
D
D2
According to the preceding equations, the user has relative
freedom in selecting the switch duty cycle or turns ratio to
suit a given application. The selections of the duty cycle
and the turns ratio are somewhat iterative processes, due
to the number of variables involved. The user can choose
either a duty cycle or a turns ratio as the start point. The
following trade-offs should be considered when selecting
the switch duty cycle or turns ratio, to optimize the
converter performance. A higher duty cycle affects the
flyback converter in the following aspects:
• Lower MOSFET RMS current ISW(RMS), but higher
MOSFET VDS peak voltage
• Lower diode peak reverse voltage, but higher diode
RMS current ID(RMS)
• Higher transformer turns ratio (NP/NS)
The choice,
D
D+D2
=
1
3
(for discontinuous mode operation with a given D3) gives
the power MOSFET the lowest power stress (the product
of RMS current and peak voltage). However, in the high
output voltage applications, a higher duty cycle may be
adopted to limit the large peak reverse voltage of the
diode. The choice,
D
D+D2
=
2
3
(for discontinuous mode operation with a given D3) gives
the diode the lowest power stress (the product of RMS
current and peak voltage). An extreme high or low duty
cycle results in high power stress on the MOSFET or diode,
and reduces efficiency. It is recommended to choose a
duty cycle, D, between 20% and 80%.
Figure 8. Waveforms of the Flyback Converter
in Discontinuous Mode Operation
3757 F07
ISW
VDS
ID
DTS D2TS D3TS t
ISW(MAX)
ID(MAX)
TS
LT3757/LT3757A
19
3757afd
Applications Information
Flyback Converter: Transformer Design for
Discontinuous Mode Operation
The transformer design for discontinuous mode of operation
is chosen as presented here. According to Figure 8,
the minimum D3 (D3MIN) occurs when the converter
has the minimum VIN and the maximum output power
(POUT). Choose D3MIN to be equal to or higher than 10%
to guarantee the converter is always in discontinuous
mode operation (choosing higher D3 allows the use of low
inductances, but results in a higher switch peak current).
The user can choose a DMAX as the start point. Then, the
maximum average primary currents can be calculated by
the following equation:
ILP(MAX) =ISW(MAX) =
POUT(MAX)
DMAX • VIN(MIN) • h
where h is the converter efficiency.
If the flyback converter has multiple outputs, POUT(MAX)
is the sum of all the output power.
The maximum average secondary current is:
ILS(MAX) =ID(MAX) =
IOUT(MAX)
D2
where:
D2 = 1 – DMAX – D3
the primary and secondary RMS currents are:
ILP(RMS) = 2 •ILP(MAX) •
DMAX
3
ILS(RMS) = 2 •ILS(MAX) •
D2
3
According to Figure 8, the primary and secondary peak
currents are:
ILP(PEAK) = ISW(PEAK) = 2 • ILP(MAX)
ILS(PEAK) = ID(PEAK) = 2 • ILS(MAX)
The primary and second inductor values of the flyback
converter transformer can be determined using the following
equations:
LP =
D2
MAX • V2
IN(MIN) • h
2 • POUT(MAX) • f
LS =
D22 • (VOUT + VD)
2 • IOUT(MAX) • f
The primary to second turns ratio is:
NP
NS
=
LP
LS
Flyback Converter: Snubber Design
Transformer leakage inductance (on either the primary or
secondary) causes a voltage spike to occur after the MOSFET
turn-off. This is increasingly prominent at higher load
currents, where more stored energy must be dissipated.
In some cases a snubber circuit will be required to avoid
overvoltage breakdown at the MOSFET’s drain node. There
are different snubber circuits, and Application Note 19 is
a good reference on snubber design. An RCD snubber is
shown in Figure 7.
The snubber resistor value (RSN) can be calculated by the
following equation:
RSN = 2 •
V2
SN − VSN • VOUT •
NP
NS
I2
SW(PEAK) •LLK • f
LT3757/LT3757A
20
3757afd
Applications Information
where VSN is the snubber capacitor voltage. A smaller
VSN results in a larger snubber loss. A reasonable VSN is
2 to 2.5 times of:
VOUT •NP
NS
LLK is the leakage inductance of the primary winding, which
is usually specified in the transformer characteristics. LLK
can be obtained by measuring the primary inductance with
the secondary windings shorted. The snubber capacitor
value (CCN) can be determined using the following equation:
CCN =
VSN
ΔVSN •RCN • f
where ΔVSN is the voltage ripple across CCN. A reasonable
ΔVSN is 5% to 10% of VSN. The reverse voltage rating of
DSN should be higher than the sum of VSN and VIN(MAX).
Flyback Converter: Sense Resistor Selection
In a flyback converter, when the power switch is turned
on, the current flowing through the sense resistor
(ISENSE) is:
ISENSE = ILP
Set the sense voltage at ILP(PEAK) to be the minimum of
the SENSE current limit threshold with a 20% margin. The
sense resistor value can then be calculated to be:
RSENSE =
80mV
ILP(PEAK)
Flyback Converter: Power MOSFET Selection
For the flyback configuration, the MOSFET is selected with
a VDC rating high enough to handle the maximum VIN, the
reflected secondary voltage and the voltage spike due to
the leakage inductance. Approximate the required MOSFET
VDC rating using:
BVDSS > VDS(PEAK)
where:
VDS(PEAK) = VIN(MAX) + VSN
The power dissipated by the MOSFET in a flyback converter
is:
PFET = I2
M(RMS) • RDS(ON) + 2 • V2
DS(PEAK) • IL(MAX) •
CRSS • f /1A
The first term in this equation represents the conduction
losses in the device, and the second term, the switching
loss. CRSS is the reverse transfer capacitance, which is
usually specified in the MOSFET characteristics.
From a known power dissipated in the power MOSFET, its
junction temperature can be obtained using the following
equation:
TJ = TA + PFET • θJA = TA + PFET • (θJC + θCA)
TJ must not exceed the MOSFET maximum junction
temperature rating. It is recommended to measure the
MOSFET temperature in steady state to ensure that absolute
maximum ratings are not exceeded.
LT3757/LT3757A
21
3757afd
Applications Information
Flyback Converter: Output Diode Selection
The output diode in a flyback converter is subject to large
RMS current and peak reverse voltage stresses. A fast
switching diode with a low forward drop and a low reverse
leakage is desired. Schottky diodes are recommended if
the output voltage is below 100V.
Approximate the required peak repetitive reverse voltage
rating VRRM using:
VRRM >
NS
NP
• VIN(MAX) + VOUT
The power dissipated by the diode is:
PD = IO(MAX) • VD
and the diode junction temperature is:
TJ = TA + PD • RθJA
The RθJA to be used in this equation normally includes the
RθJC for the device, plus the thermal resistance from the
board to the ambient temperature in the enclosure. TJ must
not exceed the diode maximum junction temperature rating.
Flyback Converter: Output Capacitor Selection
The output capacitor of the flyback converter has a similar
operation condition as that of the boost converter. Refer
to the Boost Converter: Output Capacitor Selection section
for the calculation of COUT and ESRCOUT.
The RMS ripple current rating of the output capacitors
in discontinuous operation can be determined using the
following equation:
IRMS(COUT),DISCONTINUOUS ≥ IO(MAX) •
4 − (3 •D2)
3 •D2
Flyback Converter: Input Capacitor Selection
The input capacitor in a flyback converter is subject to
a large RMS current due to the discontinuous primary
current. To prevent large voltage transients, use a low
ESR input capacitor sized for the maximum RMS current.
The RMS ripple current rating of the input capacitors in
discontinuous operation can be determined using the
following equation:
IRMS(CIN),DISCONTINUOUS ≥
POUT(MAX)
VIN(MIN) • h
•
4 − (3 •DMAX )
3 •DMAX
SEPIC Converter Applications
The LT3757 can be configured as a SEPIC (single-ended
primary inductance converter), as shown in Figure 1. This
topology allows for the input to be higher, equal, or lower
than the desired output voltage. The conversion ratio as
a function of duty cycle is:
VOUT + VD
VIN
=
D
1−D
in continuous conduction mode (CCM).
In a SEPIC converter, no DC path exists between the input
and output. This is an advantage over the boost converter
for applications requiring the output to be disconnected
from the input source when the circuit is in shutdown.
Compared to the flyback converter, the SEPIC converter
has the advantage that both the power MOSFET and the
output diode voltages are clamped by the capacitors (CIN,
CDC and COUT), therefore, there is less voltage ringing
across the power MOSFET and the output diodes. The
SEPIC converter requires much smaller input capacitors
than those of the flyback converter. This is due to the fact
that, in the SEPIC converter, the inductor L1 is in series
with the input, and the ripple current flowing through the
input capacitor is continuous.
LT3757/LT3757A
22
3757afd
Applications Information
Figure 9. The Switch Current Waveform of the SEPIC Converter
3757 F08
ΔISW = χ • ISW(MAX)
ISW
DT t S
ISW(MAX)
TS
SEPIC Converter: Switch Duty Cycle and Frequency
For a SEPIC converter operating in CCM, the duty cycle
of the main switch can be calculated based on the output
voltage (VOUT), the input voltage (VIN) and the diode
forward voltage (VD).
The maximum duty cycle (DMAX) occurs when the converter
has the minimum input voltage:
DMAX =
VOUT + VD
VIN(MIN) + VOUT + VD
SEPIC Converter: Inductor and Sense Resistor Selection
As shown in Figure 1, the SEPIC converter contains two
inductors: L1 and L2. L1 and L2 can be independent, but can
also be wound on the same core, since identical voltages
are applied to L1 and L2 throughout the switching cycle.
For the SEPIC topology, the current through L1 is the
converter input current. Based on the fact that, ideally, the
output power is equal to the input power, the maximum
average inductor currents of L1 and L2 are:
IL1(MAX) = IIN(MAX) = IO(MAX) •
DMAX
1− DMAX
IL2(MAX) = IO(MAX)
In a SEPIC converter, the switch current is equal to IL1 +
IL2 when the power switch is on, therefore, the maximum
average switch current is defined as:
ISW(MAX) =IL1(MAX) +IL2(MAX) =IO(MAX) •
1
1−DMAX
and the peak switch current is:
ISW(PEAK) = 1+ c
2
•IO(MAX) •
1
1−DMAX
The constant c in the preceding equations represents the
percentage peak-to-peak ripple current in the switch, relative
to ISW(MAX), as shown in Figure 9. Then, the switch
ripple current ΔISW can be calculated by:
ΔISW = c • ISW(MAX)
The inductor ripple currents ΔIL1 and ΔIL2 are identical:
ΔIL1 = ΔIL2 = 0.5 • ΔISW
The inductor ripple current has a direct effect on the
choice of the inductor value. Choosing smaller values of
ΔIL requires large inductances and reduces the current
loop gain (the converter will approach voltage mode).
Accepting larger values of ΔIL allows the use of low inductances,
but results in higher input current ripple and
greater core losses. It is recommended that c falls in the
range of 0.2 to 0.4.
LT3757/LT3757A
23
3757afd
Given an operating input voltage range, and having chosen
the operating frequency and ripple current in the inductor,
the inductor value (L1 and L2 are independent) of the SEPIC
converter can be determined using the following equation:
L1=L2 =
VIN(MIN)
0.5 • ΔISW • f
•DMAX
For most SEPIC applications, the equal inductor values
will fall in the range of 1μH to 100μH.
By making L1 = L2, and winding them on the same core, the
value of inductance in the preceding equation is replaced
by 2L, due to mutual inductance:
L =
VIN(MIN)
ΔISW • f
•DMAX
This maintains the same ripple current and energy storage
in the inductors. The peak inductor currents are:
IL1(PEAK) = IL1(MAX) + 0.5 • ΔIL1
IL2(PEAK) = IL2(MAX) + 0.5 • ΔIL2
The RMS inductor currents are:
IL1(RMS) =IL1(MAX) • 1+
c2
L1
12
where:
cL1 =
ΔIL1
IL1(MAX)
IL2(RMS) =IL2(MAX) • 1+
c2
L2
12
where:
cL2 =
ΔIL2
IL2 (MAX)
Based on the preceding equations, the user should choose
the inductors having sufficient saturation and RMS current
ratings.
In a SEPIC converter, when the power switch is turned on,
the current flowing through the sense resistor (ISENSE) is
the switch current.
Set the sense voltage at ISENSE(PEAK) to be the minimum
of the SENSE current limit threshold with a 20% margin.
The sense resistor value can then be calculated to be:
RSENSE =
80mV
ISW(PEAK)
SEPIC Converter: Power MOSFET Selection
For the SEPIC configuration, choose a MOSFET with a
VDC rating higher than the sum of the output voltage and
input voltage by a safety margin (a 10V safety margin is
usually sufficient).
The power dissipated by the MOSFET in a SEPIC converter
is:
PFET = I2
SW(MAX) • RDS(ON) • DMAX
+ 2 • (VIN(MIN) + VOUT)2 • IL(MAX) • CRSS • f /1A
The first term in this equation represents the conduction
losses in the device, and the second term, the switching
loss. CRSS is the reverse transfer capacitance, which is
usually specified in the MOSFET characteristics.
For maximum efficiency, RDS(ON) and CRSS should be
minimized. From a known power dissipated in the power
MOSFET, its junction temperature can be obtained using
the following equation:
TJ = TA + PFET • θJA = TA + PFET • (θJC + θCA)
TJ must not exceed the MOSFET maximum junction
temperature rating. It is recommended to measure the
MOSFET temperature in steady state to ensure that absolute
maximum ratings are not exceeded.
Applications Information
LT3757/LT3757A
24
3757afd
Applications Information
Figure 10. A Simplified Inverting Converter
RSENSE
CDC
VIN
CIN
L1
D1 COUT
VOUT
3757 F09
GATE +
GND
LT3757
SENSE
L2
M1
+
–
+ –
+
SEPIC Converter: Output Diode Selection
To maximize efficiency, a fast switching diode with a low
forward drop and low reverse leakage is desirable. The
average forward current in normal operation is equal to
the output current, and the peak current is equal to:
ID(PEAK) = 1+ c
2
•IO(MAX) •
1
1−DMAX
It is recommended that the peak repetitive reverse voltage
rating VRRM is higher than VOUT + VIN(MAX) by a safety
margin (a 10V safety margin is usually sufficient).
The power dissipated by the diode is:
PD = IO(MAX) • VD
and the diode junction temperature is:
TJ = TA + PD • RθJA
The RθJA used in this equation normally includes the RθJC
for the device, plus the thermal resistance from the board,
to the ambient temperature in the enclosure. TJ must not
exceed the diode maximum junction temperature rating.
SEPIC Converter: Output and Input Capacitor Selection
The selections of the output and input capacitors of the
SEPIC converter are similar to those of the boost converter.
Please refer to the Boost Converter, Output Capacitor
Selection and Boost Converter, Input Capacitor Selection
sections.
SEPIC Converter: Selecting the DC Coupling Capacitor
The DC voltage rating of the DC coupling capacitor (CDC,
as shown in Figure 1) should be larger than the maximum
input voltage:
VCDC > VIN(MAX)
CDC has nearly a rectangular current waveform. During
the switch off-time, the current through CDC is IIN, while
approximately –IO flows during the on-time. The RMS
rating of the coupling capacitor is determined by the following
equation:
IRMS(CDC) > IO(MAX) •
VOUT + VD
VIN(MIN)
A low ESR and ESL, X5R or X7R ceramic capacitor works
well for CDC.
Inverting Converter Applications
The LT3757 can be configured as a dual-inductor inverting
topology, as shown in Figure 10. The VOUT to VIN ratio is:
VOUT − VD
VIN
= −
D
1−D
in continuous conduction mode (CCM).
LT3757/LT3757A
25
3757afd
Inverting Converter: Switch Duty Cycle and Frequency
For an inverting converter operating in CCM, the duty
cycle of the main switch can be calculated based on the
negative output voltage (VOUT) and the input voltage (VIN).
The maximum duty cycle (DMAX) occurs when the converter
has the minimum input voltage:
DMAX =
VOUT − VD
VOUT − VD − VIN(MIN)
Inverting Converter: Inductor, Sense Resistor, Power
MOSFET, Output Diode and Input Capacitor Selections
The selections of the inductor, sense resistor, power
MOSFET, output diode and input capacitor of an inverting
converter are similar to those of the SEPIC converter.
Please refer to the corresponding SEPIC converter sections.
Inverting Converter: Output Capacitor Selection
The inverting converter requires much smaller output
capacitors than those of the boost, flyback and SEPIC
converters for similar output ripples. This is due to the fact
that, in the inverting converter, the inductor L2 is in series
with the output, and the ripple current flowing through the
output capacitors are continuous. The output ripple voltage
is produced by the ripple current of L2 flowing through
the ESR and bulk capacitance of the output capacitor:
ΔVOUT(P–P) = ΔIL2 • ESRCOUT +
1
8 • f •COUT
After specifying the maximum output ripple, the user can
select the output capacitors according to the preceding
equation.
The ESR can be minimized by using high quality X5R or
X7R dielectric ceramic capacitors. In many applications,
ceramic capacitors are sufficient to limit the output voltage
ripple.
The RMS ripple current rating of the output capacitor
needs to be greater than:
IRMS(COUT) > 0.3 • ΔIL2
Inverting Converter: Selecting the DC Coupling Capacitor
The DC voltage rating of the DC coupling capacitor (CDC,
as shown in Figure 10) should be larger than the maximum
input voltage minus the output voltage (negative voltage):
VCDC > VIN(MAX) – VOUT
CDC has nearly a rectangular current waveform. During
the switch off-time, the current through CDC is IIN, while
approximately –IO flows during the on-time. The RMS
rating of the coupling capacitor is determined by the following
equation:
IRMS(CDC) >IO(MAX) •
DMAX
1−DMAX
A low ESR and ESL, X5R or X7R ceramic capacitor works
well for CDC.
Applications Information
LT3757/LT3757A
26
3757afd
Applications Information
Figure 11. 8V to 16V Input, 24V/2A Output Boost Converter Suggested Layout
VIN
3757 F10
VOUT
L1
VIAS TO GROUND
PLANE
C D1 COUT2 OUT1
1
2
8
7
3
4
6
5
M1
CIN
R4
RC
R1
R2
RSS
RT
R3
CVCC
CC1
CC2
LT3757
1
2
3
4
5
9
10
6
7
8
RS
Board Layout
The high speed operation of the LT3757 demands careful
attention to board layout and component placement. The
Exposed Pad of the package is the only GND terminal of
the IC, and is important for thermal management of the
IC. Therefore, it is crucial to achieve a good electrical and
thermal contact between the Exposed Pad and the ground
plane of the board. For the LT3757 to deliver its full output
power, it is imperative that a good thermal path be provided
to dissipate the heat generated within the package.
It is recommended that multiple vias in the printed circuit
board be used to conduct heat away from the IC and into
a copper plane with as much area as possible.
To prevent radiation and high frequency resonance problems,
proper layout of the components connected to the
IC is essential, especially the power paths with higher di/
dt. The following high di/dt loops of different topologies
should be kept as tight as possible to reduce inductive
ringing:
• In boost configuration, the high di/dt loop contains
the output capacitor, the sensing resistor, the power
MOSFET and the Schottky diode.
• In flyback configuration, the high di/dt primary loop
contains the input capacitor, the primary winding, the
power MOSFET and the sensing resistor. The high di/
dt secondary loop contains the output capacitor, the
secondary winding and the output diode.
• In SEPIC configuration, the high di/dt loop contains
the power MOSFET, sense resistor, output capacitor,
Schottky diode and the coupling capacitor.
• In inverting configuration, the high di/dt loop contains
power MOSFET, sense resistor, Schottky diode and the
coupling capacitor.
LT3757/LT3757A
27
3757afd
Table 2. Recommended Component Manufacturers
VENDOR COMPONENTS WEB ADDRESS
AVX Capacitors avx.com
BH Electronics Inductors,
Transformers
bhelectronics.com
Coilcraft Inductors coilcraft.com
Cooper Bussmann Inductors bussmann.com
Diodes, Inc Diodes diodes.com
Fairchild MOSFETs fairchildsemi.com
General
Semiconductor
Diodes generalsemiconductor.com
International Rectifier MOSFETs, Diodes irf.com
IRC Sense Resistors irctt.com
Kemet Capacitors kemet.com
Magnetics Inc Toroid Cores mag-inc.com
Microsemi Diodes microsemi.com
Murata-Erie Inductors,
Capacitors
murata.co.jp
Nichicon Capacitors nichicon.com
On Semiconductor Diodes onsemi.com
Panasonic Capacitors panasonic.com
Sanyo Capacitors sanyo.co.jp
Sumida Inductors sumida.com
Taiyo Yuden Capacitors t-yuden.com
TDK Capacitors,
Inductors
component.tdk.com
Thermalloy Heat Sinks aavidthermalloy.com
Tokin Capacitors nec-tokinamerica.com
Toko Inductors tokoam.com
United Chemi-Con Capacitors chemi-con.com
Vishay/Dale Resistors vishay.com
Vishay/Siliconix MOSFETs vishay.com
Vishay/Sprague Capacitors vishay.com
Würth Elektronik Inductors we-online.com
Zetex Small-Signal
Discretes
zetex.com
Applications Information
Check the stress on the power MOSFET by measuring its
drain-to-source voltage directly across the device terminals
(reference the ground of a single scope probe directly to
the source pad on the PC board). Beware of inductive
ringing, which can exceed the maximum specified voltage
rating of the MOSFET. If this ringing cannot be avoided,
and exceeds the maximum rating of the device, either
choose a higher voltage device or specify an avalancherated
power MOSFET.
The small-signal components should be placed away from
high frequency switching nodes. For optimum load regulation
and true remote sensing, the top of the output voltage
sensing resistor divider should connect independently to
the top of the output capacitor (Kelvin connection), staying
away from any high dV/dt traces. Place the divider resistors
near the LT3757 in order to keep the high impedance
FBX node short.
Figure 11 shows the suggested layout of the 8V to 16V
Input, 24V/2A Output Boost Converter.
Recommended Component Manufacturers
Some of the recommended component manufacturers
are listed in Table 2.
LT3757/LT3757A
28
3757afd
Typical Applications
3.3V Input, 5V/10A Output Boost Converter
Boost Preregulator for Automotive Stop-Start/Idle
Efficiency vs Output Current
Transient VIN and VOUT Waveforms
SENSE
LT3757
VIN
VIN
3.3V CIN
22μF
6.3V
×2
VOUT
5V
10A
0.004
1W
M1
41.2k
300kHz
GATE
FBX
GND
INTVCC
SHDN/UVLO
SYNC
RT
SS VC
49.9k
34k
0.1μF
6.8k
22nF 2.2nF
22
L1
0.5μH
D1
3757 TA02a
34k
1%
15.8k
1%
COUT1
150μF
6.3V
×4
COUT2
22μF
6.3V
X5R
×4
+
CVCC
4.7μF
10V
X5R
CIN: TAIYO YUDEN JMK325BJ226MM
COUT1: PANASONIC EEFUEOJ151R
COUT2: TAIYO YUDEN JMK325BJ226MM
D1: MBRB2515L
L1: VISHAY SILICONIX IHLP-5050FD-01
M1: VISHAY SILICONIX SI4448DY
OUTPUT CURRENT (A)
EFFICIENCY (%)
3757 TA02b
0.001
20
30
40
50
60
70
80
90
100
0.01 0.1 1 10
SENSE
LT3757A
VIN
VIN
3V TO 36V 10μF
50V
X5R
×2
VOUT
9VMIN
2A
41.2k
300kHz
GATE
FBX
GND
INTVCC
SHDN/UVLO
SYNC
RT
SS
VC
1M
698k
0.1μF
10k
10nF 4.7μF
L1
3.3μH D1
3757 TA03a
M1
75k
8m
16.2k
C1
10μF
50V
×4
+
10μF
50V
X5R
L1: COILTRONIX DR127-3R3
M1: VISHAY SILICONIX Si7848BDP
D1: VISHAY SILICONIX 50SQ04FN
C1: KEMET T495X106K050A
10ms/DIV
VOUT
5V/DIV
VIN
5V/DIV
0V
3757 TA03b
OUTPUT POWER = 10W
LT3757/LT3757A
29
3757afd
Typical Applications
8V to 16V Input, 24V/2A Output Boost Converter
Efficiency vs Output Current Load Step Response at VIN = 12V
SENSE
LT3757
VIN
VIN
8V TO 16V CIN
10μF
25V
X5R
CVCC
4.7μF
10V
X5R
VOUT
24V
2A
RS
0.01
1W
M1
RT
41.2k
300kHz
GATE
FBX
GND INTVCC
SHDN/UVLO
SYNC
RT
SS
VC
R3
200k
R4
43.2k
CSS
0.1μF
CC2
100pF
RC
22k
CC1
6.8nF
L1
10μH
D1
3757 TA04a
R2
226k
1%
R1
16.2k
1%
COUT1
47μF
35V
×4
COUT2
10μF
25V
X5R
+
CIN, COUT2: MURATA GRM31CR61E106KA12
COUT1: KEMET T495X476K035AS
D1: ON SEMI MBRS340T3G
L1: VISHAY SILICONIX IHLP-5050FD-01 10μH
M1: VISHAY SILICONIX Si4840BDP
OUTPUT CURRENT (A)
0.001
EFFICIENCY (%)
30
50
40
60
70
80
90
100
0.01 0.1 1
3757 TA04b
10
VIN = 8V
VIN = 16V
500μs/DIV
VOUT
500mV/DIV
(AC)
1.6A
0.4A
IOUT
1A/DIV
3757 TA04c
LT3757/LT3757A
30
3757afd
2ms/DIV
VOUT
100V/DIV
3757 TA05b
5μs/DIV
VOUT
5V/DIV
(AC)
VSW
20V/DIV
3757 TA05c
Typical Applications
High Voltage Flyback Power Supply
Start-Up Waveforms Switching Waveforms
SENSE
LT3757
VIN
VSW
VIN
5V TO 12V CIN
47μF
16V
×4
INTVCC
COUT
68nF
×2
VOUT
350V
10mA
0.02
22
M1
140k
100kHz
GATE
GND FBX
SHDN/UVLO
DANGER! HIGH VOLTAGE OPERATION BY HIGH VOLTAGE TRAINED PERSONNEL ONLY
SYNC
RT
SS
VC
•
105k •
46.4k
0.1μF
220pF
100pF
6.8k
22nF
T1
1:10
D1
CIN: MURATA GRM32ER61C476K
COUT: TDK C3225X7R2J683K
D1: VISHAY SILICONIX GSD2004S DUAL DIODE CONNECTED IN SERIES
M1: VISHAY SILICONIX Si7850DP
T1: TDK DCT15EFD-U44S003
3757 TA05a
1M
1%
1M
1%
1.50M
1%
16.2k
1%
10nF
CVCC
47μF
25V
X5R
22
LT3757/LT3757A
31
3757afd
Typical Applications
5.5V to 36V Input, 12V/2A Output SEPIC Converter
Efficiency vs Output Current Load Step Waveforms
Start-Up Waveforms Frequency Foldback Waveforms When Output Short-Circuits
SENSE
LT3757A
VIN
VIN
5.5V TO 36V CIN1
4.7μF
50V
×2
CDC
4.7μF
50V, X5R, ×2
4.7μF
10V
X5R
VOUT
12V
2A
0.01
1W
M1
41.2k
300kHz
GATE
FBX
GND INTVCC
SHDN/UVLO
SYNC
RT
SS
CIN2
4.7μF
50V
×2
•
•
105k
46.4k
0.1μF 6.8nF
10k
L1A
IL1B L1B
D1
CIN1, CDC: TAIYO YUDEN UMK316BJ475KL
CIN2: KEMET T495X475K050AS
COUT1: KEMET T495X476K020AS
COUT2: TAIYO YUDEN TMK432BJ106MM
D1: ON SEMI MBRS360T3G
L1A, L1B: COILTRONICS DRQ127-4R7 (*COUPLED INDUCTORS)
M1: VISHAY SILICONIX Si7460DP
3757 TA06a
105k
1%
15.8k
1%
COUT1
47μF
20V
×4
COUT2
10μF
25V
X5R
+
VSW
IL1A
VC
+
2ms/DIV
VOUT
5V/DIV
IL1A + IL1B
5A/DIV
3757 TA06d
VIN = 12V
50μs/DIV
VOUT
10V/DIV
VSW
20V/DIV
IL1A + IL1B
5A/DIV
3757 TA06e
VIN = 12V
OUTPUT CURRENT (A)
0.001
20
EFFICIENCY (%)
30
40
50
60
70
80
90
100
0.01 0.1 1
3757 TA06b
10
VIN = 16V
VIN = 8V
500μs/DIV
VOUT
2V/DIV
AC-COUPLED
IOUT
2A/DIV 0A
2A
3757 TA06c
VIN = 12V
LT3757/LT3757A
32
3757afd
Typical Applications
5V to 12V Input, ±12V/0.4A Output SEPIC Converter
Nonisolated Inverting SLIC Supply
SENSE
LT3757
VIN
VIN
5V TO 12V
CIN1
1μF
16V, X5R
CIN2
47μF
16V
CDC1
4.7μF
16V, X5R
CDC2
4.7μF
16V
X5R
COUT2
4.7μF
16V, X5R
×3
VOUT1
12V
0.4A
VOUT2
–12V
0.4A
COUT2
4.7μF
16V, X5R
×3
CVCC
4.7μF
10V
X5R
0.02
M1
30.9k
400kHz
D1, D2: MBRS140T3
T1: COILTRONICS VP1-0076 (*PRIMARY = 4 WINDINGS IN PARALLEL)
M1: SILICONIX/VISHAY Si4840BDY
GATE
FBX
GND INTVCC
SHDN/UVLO
SYNC
RT
SS
+ 105k •
46.4k
0.1μF 100pF
22k
6.8nF
T1
1,2,3,4
D1
GND
1.05k
1%
158
1%
D2
5
6
•
•
3757 TA07
VC
SENSE
LT3757
VIN
VIN
5V TO 16V CIN
22μF
25V, X5R
×2
C2
10μF
50V
X5R
D1
DFLS160
CVCC
4.7μF
10V, X5R
C3
22μF
25V
X5R
C4
22μF
25V
X5R
COUT
3.3μF
100V
GND
C5
22μF
25V
X5R
VOUT1
–24V
200mA
VOUT1
–72V
200mA
0.012
0.5W
M1
Si7850DP
63.4k
200kHz
GATE
FBX
GND INTVCC
SHDN/UVLO
SYNC
RT
SS
•
•
•
R2 •
105k
R1
46.4k
0.1μF
100pF
15.8k
464k
9.1k
10nF
T1
1,2,3
4
D2
DFLS160
5
D3
DFLS160
6
VP5-0155 (PRIMARY = 3 WINDINGS IN PARALLEL)
3757 TA08
VC
LT3757/LT3757A
33
3757afd
Package Description
3.00 ±0.10
(4 SIDES)
NOTE:
1. DRAWING TO BE MADE A JEDEC PACKAGE OUTLINE M0-229 VARIATION OF (WEED-2).
CHECK THE LTC WEBSITE DATA SHEET FOR CURRENT STATUS OF VARIATION ASSIGNMENT
2. DRAWING NOT TO SCALE
3. ALL DIMENSIONS ARE IN MILLIMETERS
4. DIMENSIONS OF EXPOSED PAD ON BOTTOM OF PACKAGE DO NOT INCLUDE
MOLD FLASH. MOLD FLASH, IF PRESENT, SHALL NOT EXCEED 0.15mm ON ANY SIDE
5. EXPOSED PAD SHALL BE SOLDER PLATED
6. SHADED AREA IS ONLY A REFERENCE FOR PIN 1 LOCATION ON THE
TOP AND BOTTOM OF PACKAGE
0.40 ± 0.10
BOTTOM VIEW—EXPOSED PAD
1.65 ± 0.10
(2 SIDES)
0.75 ±0.05
R = 0.125
TYP
2.38 ±0.10
(2 SIDES)
5 1
6 10
PIN 1
TOP MARK
(SEE NOTE 6)
0.200 REF
0.00 – 0.05
(DD) DFN REV C 0310
0.25 ± 0.05
2.38 ±0.05
(2 SIDES)
RECOMMENDED SOLDER PAD PITCH AND DIMENSIONS
1.65 ±0.05
2.15 ±0.05 (2 SIDES)
0.50
BSC
0.70 ±0.05
3.55 ±0.05
PACKAGE
OUTLINE
0.25 ± 0.05
0.50 BSC
DD Package
10-Lead Plastic DFN (3mm × 3mm)
(Reference LTC DWG # 05-08-1699 Rev C)
PIN 1 NOTCH
R = 0.20 OR
0.35 × 45°
CHAMFER
LT3757/LT3757A
34
3757afd
Package Description
MSOP (MSE) 0911 REV H
0.53 ±0.152
(.021 ±.006)
SEATING
PLANE
0.18
(.007)
1.10
(.043)
MAX
0.17 –0.27
(.007 – .011)
TYP
0.86
(.034)
REF
0.50
(.0197)
BSC
1 2 3 4 5
4.90 ±0.152
(.193 ±.006)
0.497 ±0.076
(.0196 ±.003)
REF
10 9 8
10
1
7 6
3.00 ±0.102
(.118 ±.004)
(NOTE 3)
3.00 ±0.102
(.118 ±.004)
(NOTE 4)
NOTE:
1. DIMENSIONS IN MILLIMETER/(INCH)
2. DRAWING NOT TO SCALE
3. DIMENSION DOES NOT INCLUDE MOLD FLASH, PROTRUSIONS OR GATE BURRS.
MOLD FLASH, PROTRUSIONS OR GATE BURRS SHALL NOT EXCEED 0.152mm (.006") PER SIDE
4. DIMENSION DOES NOT INCLUDE INTERLEAD FLASH OR PROTRUSIONS.
INTERLEAD FLASH OR PROTRUSIONS SHALL NOT EXCEED 0.152mm (.006") PER SIDE
5. LEAD COPLANARITY (BOTTOM OF LEADS AFTER FORMING) SHALL BE 0.102mm (.004") MAX
6. EXPOSED PAD DIMENSION DOES INCLUDE MOLD FLASH. MOLD FLASH ON E-PAD
SHALL NOT EXCEED 0.254mm (.010") PER SIDE.
0.254
(.010) 0° – 6° TYP
DETAIL “A”
DETAIL “A”
GAUGE PLANE
5.23
(.206)
MIN
3.20 – 3.45
(.126 – .136)
0.889 ±0.127
(.035 ±.005)
RECOMMENDED SOLDER PAD LAYOUT
1.68 ±0.102
(.066 ±.004)
1.88 ±0.102
(.074 ±.004)
0.50
(.0197)
BSC
0.305 ± 0.038
(.0120 ±.0015)
TYP
BOTTOM VIEW OF
EXPOSED PAD OPTION
1.68
(.066)
1.88
(.074)
0.1016 ±0.0508
(.004 ±.002)
DETAIL “B”
DETAIL “B”
CORNER TAIL IS PART OF
THE LEADFRAME FEATURE.
FOR REFERENCE ONLY
NO MEASUREMENT PURPOSE
0.05 REF
0.29
REF
MSE Package
10-Lead Plastic MSOP, Exposed Die Pad
(Reference LTC DWG # 05-08-1664 Rev H)
LT3757/LT3757A
35
3757afd
Information furnished by Linear Technology Corporation is believed to be accurate and reliable.
However, no responsibility is assumed for its use. Linear Technology Corporation makes no representation
that the interconnection of its circuits as described herein will not infringe on existing patent rights.
Revision History
REV DATE DESCRIPTION PAGE NUMBER
B 3/10 Deleted Bullet from Features and Last Line of Description
Updated Entire Page to Add H-Grade and Military Grade
Updated Electrical Characteristics Notes and Typical Performance Characteristics for H-Grade and Military Grade
Revised TA04a and Replaced TA04c in Typical Applications
Updated Related Parts
1
2
4 to 6
30
36
C 5/11 Revised MP-grade temperature range in Absolute Maximum Ratings and Order Information sections
Revised Note 2
Revised formula in Applications Information
Updated Typical Application drawing TA04a values
Revised Typical Application title TA06
2
4
19
30
32
D 07/12 Added LT3757A version Throughout
Updated Block Diagram 8
Updated Programming the Output Voltage section 12
Updated Loop Compensation section 13
Added an application circuit in the Typical Applications section 28
Updated the schematic and Load Step Waveforms in the Typical Applications section 31
(Revision history begins at Rev B)
LT3757/LT3757A
36
3757afd
Linear Technology Corporation
1630 McCarthy Blvd., Milpitas, CA 95035-7417
(408) 432-1900 ● FAX: (408) 434-0507 ● www.linear.com LINEAR TECHNOLOGY CORPORATION 2008
LT 0712 REV D • PRINTED IN USA
Related Parts
Typical Application
PART NUMBER DESCRIPTION COMMENTS
LT3758A Boost, Flyback, SEPIC and Inverting Controller 5.5V ≤ VIN ≤ 100V, Current Mode Control, 100kHz to 1MHz Programmable
Operation Frequency, 3mm × 3mm DFN-10 and MSOP-10E Packages
LT3759 Boost, SEPIC and Inverting Controller 1.6V ≤ VIN ≤ 42V, Current Mode Control, 100kHz to 1MHz Programmable
Operation Frequency, MSOP-12E Packages
LT3957A Boost, Flyback, SEPIC and Inverting Controller
with 5A, 40V Switch
3V ≤ VIN ≤ 40V, Current Mode Control, 100kHz to 1MHz Programmable Operation
Frequency, 5mm × 6mm QFN Package
LT3958 Boost, Flyback, SEPIC and Inverting Controller
with 3.3A, 84V Switch
5V ≤ VIN ≤ 80V, Current Mode Control, 100kHz to 1MHz Programmable Operation
Frequency, 5mm × 6mm QFN Package
LT3573/LT3574/
LT3575
40V Isolated Flyback Converters Monolithic No-Opto Flybacks with Integrated 1.25A/0.65A/2.5A Switch
LT3511/LT3512 100V Isolated Flyback Converters Monolithic No-Opto Flybacks with Integrated 240mA/420mA Switch
LT3798 Offline Isolated No Opto-Coupler Flyback
Controller with Active PFC
VIN and VOUT Limited Only by External Components, MSOP-16 Package
LT3799/LT3799-1 Offline Isolated Flyback LED Controllers with
Active PFC
VIN and VOUT Limited Only by External Components, MSOP-16 Package
High Efficiency Inverting Power Supply
Efficiency vs Output Current
OUTPUT CURRENT (A)
0.001
10
EFFICIENCY (%)
20
30
40
50
60
70
80
90
100
0.01 0.1 1
3757 TA09b
10
VIN = 16V
VIN = 5V
SENSE
LT3757
VIN
VIN
5V TO
15V
CIN
47μF
16V
X5R
CDC
47μF
25V, X5R
VOUT
–5V
3A to 5A
0.006
1W
M1
Si7848BDP
41.2k
300kHz
GATE
FBX
GND INTVCC
SHDN/UVLO
SYNC
RT
SS
•
R2 •
105k
R1
46.4k
0.1μF
9.1k
10nF
L1
L2
D1
MBRD835L
L1, L2: COILTRONICS DRQ127-3R3 (*COUPLED INDUCTORS) 3757 TA09a
84.5k
CVCC 16k
4.7μF
10V
X5R
COUT
100μF
6.3V, X5R
×2
VC
Photoelectric proximity
switches HGA
Photoelectric proximity
switches ener.
Photoelectric proximity
switches V
Photoelectric
reflex switches
T
W 9-2: A Versatile, Complete
and Compact Series
D A T A S H E E T
The W 9-2 series is as versatile
as the tasks in automation.
The standardized, compact housing
model makes it possible to
use high-performance sensors
that operate reliably even in
cramped mounting conditions.
All W 9-2 models have red light
transmitters as a standard feature.
The sensor can be aligned
on the object quickly and precisely
using the visible light spot.
In the models with Teach-In function,
the sensor optimizes its sensitivity
automatically to the given
operating conditions at the push
of a button.
Depending on the job, the most
suitable sensor can be selected
from the W 9-2 series.
Overview of the sensors:
WT 9-2, with adjustable background
suppression,
max. scanning distance 250 mm,
WT 9-2, energetic,
max. scanning distance 450 mm,
WT 9-2, V model,
max. scanning distance 20 mm,
WL 9-2, basic model,
max. scanning range 4 m,
WL 9-2, Teach-In model,
max. scanning range 4 m,
WL 9-2, focus,
max. scanning range 0.4 m.
There are multifaceted applications
in the targeted main
branches thanks to this great
variety of products:
Storage and handling
engineering
Packaging industry
Electronics industry
Elevator construction.
2 SENSICK
WT 9-2 Photoelectric Proximity Switch with Background Suppression
Setting options
Dimension illustration
LED light source, visible red light
Background suppression
Scanning distance adjustable
Switching frequency 1500/s
Outputs short-circuit protected
Scanning distance
30 ... 250 mm
12
22
40
20 3
3
1.5
1
3
2
3
18.5 10.5
11
4
5
7
Axis of the sender optics
Axis of the receiver optics
Mounting hole Ø 3.2 mm
LED signal strength indicator
Plug M 12 or M 8, 4 pin,
2 m connection cable or
120 mm cable with plug M 12, 4 pin
Scanning distance adjuster
Standard direction of the material to be scanned
1
2
3
4
5
Photoelectric proximity switch
6
7
WT 9-2P130
WT 9-2P430
WT 9-2N130
WT 9-2N430
4
6
Cable receptacles
Adapter plate
Mounting bracket
Accessories
Connection type
L+
Q
Q
M
brn
wht
blu
blk
4 pin, M 12
WT 9-2P330
WT 9-2P630
WT 9-2P430
WT 9-2N430
1
L+
Q
Q
4
2
3
M
brn
wht
blu
blk
4 pin, M 8
1
L+
Q
Q
4
2
3
M
brn
wht
blu
blk
4 x 0,14 mm2
1
L+
Q
Q
4
2
3
M
brn
wht
blu
blk
4 pin, M 12 with 120 mm cable
WT 9-2P330 WT 9-2P130
WT 9-2N130
WT 9-2P630
Scanning distance adjustable 1) 30 ... 250 mm
Scanning range 5 ... 250 mm
Supply voltage VS
2) DC 10 ... 30 V
Ripple 3) ≤ 5 VPP
Current consumption 4) ≤ 40 mA
Light source LED, visible red light 5)
Light spot diameter 15 x 15 mm at a distance of 200 mm
Switching outputs Q and Q– PNP
NPN
Signal voltage HIGH VS – 2.9 V
VS
Signal voltage LOW 6) Approx. 0 V
≤ 1.5 V
Output current IA max. ≤ 100 mA
Response time 7) ≤ 333 μs
Switching frequency max. 8) 1500/s
Connection technology Connection cable, 2 m
Cable, 120 mm, with plug M 12, 4 pin
Plug M 12, 4 pin
Plug M 8, 4 pin
VDE protection class M 12 9)
VDE protection class M 8 9) III
Protection type IP 67
Protection circuits 10) A, B, C
Ambient temperature 11) Operation –40 ... +60 °C
Storage –40 ... +75 °C
Weight
with connection cable 2 m/120 mm Approx. 80 g
with equipment plug M 12/M 8, 4 pin Approx. 20 g
SENSICK 3
WT 9-2
Scanning distance Ordering information
Technical data WT 9-2 P130 P430 N130 N430 P330 P630
1) Object with 90% reflectance
(referred to standard white DIN 5033)
2) Limit values
3) Must be within VS tolerances
4) Without load
5) Average service life at
room temperature 100,000 h
6) At TU = +25 °C and 100 mA
output current
7) With resistive load
8) With light/dark ratio 1:1
9) Withstand voltage 50 V
10) A = supply connections reverse
polarity protected
B = outputs short-circuit protected
C = interference suppression
11) Do not distort cable below 0 °C
Type
WT 9-2P130
WT 9-2P430
WT 9-2N130
WT 9-2N430
WT 9-2P330
WT 9-2P630
Order no.
1 018 293
1 018 295
1 018 294
1 018 296
1 019 026
1 019 272
(mm) 50 100 150 200 250
30
15
20
25
10
0
5
% of scanning distance
1
3
2
WT 9-2 HGA
90%/90%
18%/90%
6%/90%
Scanning range on gray, white background,
Black = 6% reflectance
1 Scanning range on black ), white background,
2
White = 90% reflectance
Scanning range on white, white background,
Gray = 18% reflectance
3
0(mm) 50 100 150 200 250
3
1
2
Operating distance
30 150
30 220
30 250
4 SENSICK
WT 9-2 Photoelectric Proximity Switch, Energetic, Teach-In
Setting options
Dimension illustration
Red-light emitter LED as
alignment aid
Scanning distance adjustable
Switching frequency 800/s
Outputs short-circuit protected
Teach-In
Scanning distance
18 ... 450 mm
12
22
40
20 3
3
1.5
1
3
2 3
25.55 6.5
11
4
5
Axis of the receiver optics
Axis of the sender optics
Mounting hole Ø 3.2 mm
LED signal strength indicator
Plug M 12 or M 8, 4 pin,
2 m connection cable or
120 mm cable with plug M 12, 4 pin
Scanning distance adjuster, teachable
1
2
3
4
5
Photoelectric proximity switch
6
WT 9-2P151
WT 9-2P451
WT 9-2N151
WT 9-2N451
4
6
Cable receptacles
Adapter plate
Mounting bracket
Accessories
Connection type
L+
Q
Q
M
brn
wht
blu
blk
4 pin, M 12
WT 9-2P351
WT 9-2P651
WT 9-2P451
WT 9-2N451
1
L+
Q
Q
4
2
3
M
brn
wht
blu
blk
4 pin, M 8
1
L+
Q
Q
4
2
3
M
brn
wht
blu
blk
4 x 0,14 mm2
1
L+
Q
Q
4
2
3
M
brn
wht
blu
blk
4 pin, M 12 with 120 mm cable
WT 9-2P351 WT 9-2P151
WT 9-2N151
WT 9-2P651
SENSICK 5
Scanning distance adjustable 1) 10 ... 450 mm
Supply voltage VS
2) DC 10 ... 30 V
Ripple 3) ≤ 5 VPP
Current consumption 4) ≤ 30 mA
Light source LED, visible red light 5)
Light spot diameter 80 x 80 mm at a distance of 500 mm
Switching outputs Q and Q– PNP
NPN
Signal voltage HIGH VS – 2.9 V
VS
Signal voltage LOW6) Approx. 0 V
≤ 2.9 V
Output current IA max. ≤ 100 mA
Response time 7) ≤ 625 μs
Switching frequency max. 8) 800/s
Connection technology Connection cable, 2 m
Cable, 120 mm, with plug M 12, 4 pin
Plug M 12, 4 pin
Plug M 8, 4 pin
VDE protection class M 12 9)
VDE protection class M 8 9) III
Protection type IP 67
Protection circuits 10) A, B, C
Ambient temperature 11) Operation –40 ... +60 °C
Storage –40 ... +75 °C
Weight
with connection cable 2 m/120 mm Approx. 80 g
with equipment plug M 12/M 8, 4 pin Approx. 20 g
WT 9-2
Scanning distance Ordering information
Technical data WT 9-2 P151 P451 N151 N451 P351 P651
1) Object with 90% reflectance
(referred to standard white DIN 5033)
2) Limit values
3) Must be within VS tolerances
4) Without load
5) Average service life at
room temperature 50,000 h
6) At TU = +25 °C and 100 mA
output current
7) With resistive load
8) With light/dark ratio 1:1
9) Withstand voltage 50 V
10) A = supply connections reverse
polarity protected
B = outputs short-circuit protected
C = interference suppression
11) Do not distort cable below 0 °C
Type
WT 9-2P151
WT 9-2P451
WT 9-2N151
WT 9-2N451
WT 9-2P351
WT 9-2P651
Order no.
1 018 297
1 018 299
1 018 298
1 018 300
1 019 027
1 019 273
(mm) 100 200
1000
10
100
1
300 400 500
Function reserve
Operating
distance
Limiting
scanning distance
WT 9-2 energetic
3
90%
2
18%
1
6%
Programming via Teach-In button.
Simple programming:
Position object in the beam and push the button:
finished;
LED confirms the Teach-In procedure.
Teach-In values can be stored.
Teach-In function
Two operating modes:
Default setting: short Teach-In time (< 8 s);
for standard applications;
approx. double reserve via switching threshold;
LED lights continuously.
Precise setting: long Teach-In time (> 8 s);
for precise applications;
small switching hysteresis;
LED blinks.
Scanning range on white, 90 % reflectance
Scanning range on gray, 18% reflectance
1 Scanning range on black, 6% reflectance
2
3
0(mm) 100 200 300 400 500
1
2
3
Operating distance Limiting scanning distance
10 180 220
10/100 130
10 350 450
6 SENSICK
WT 9-2 Photoelectric Proximity Switch, V-type, Teach-In
Setting options
Dimension illustration
Red-light emitter LED as
alignment aid
Scanning distance adjustable
Switching frequency 800/s
Outputs short-circuit protected
Teach-In
Scanning distance
10 ... 20 mm
12
22
40
20 3
3
1.5
1
3
2 3
26.45 4.7
11
4
5
Axis of the receiver optics
Axis of the receiver optics
Mounting hole Ø 3.2 mm
LED signal strength indicator
Plug M 12 or M 8, 4 pin,
2 m connection cable or
120 mm cable with plug M 12, 4 pin
Scanning distance adjuster, teachable
1
2
3
4
5
Photoelectric proximity switch
6
WT 9-2P141
WT 9-2P441
WT 9-2N141
WT 9-2N441
4
6
Cable receptacles
Adapter plate
Mounting bracket
Accessories
Connection type
L+
Q
Q
M
brn
wht
blu
blk
4 pin, M 12
WT 9-2P341
WT 9-2P641
WT 9-2P441
WT 9-2N441
1
L+
Q
Q
4
2
3
M
brn
wht
blu
blk
4 pin, M 8
1
L+
Q
Q
4
2
3
M
brn
wht
blu
blk
4 x 0,14 mm2
1
L+
Q
Q
4
2
3
M
brn
wht
blu
blk
4 pin, M 12 with 120 mm cable
WT 9-2P341 WT 9-2P141
WT 9-2N141
WT 9-2P641
SENSICK 7
Scanning distance adjustable 1) 10 ... 20 mm
Supply voltage VS
2) DC 10 ... 30 V
Ripple 3) ≤ 5 VPP
Current consumption 4) ≤ 30 mA
Light source LED, visible red light 5)
Light spot diameter 3 mm at a distance of 20 mm
Switching outputs Q and Q– PNP
NPN
Signal voltage HIGH VS – 2.9 V
VS
Signal voltage LOW6) Approx. 0 V
≤ 2.9 V
Output current IA max. ≤ 100 mA
Response time 7) ≤ 625 μs
Switching frequency max. 8) 800/s
Connection technology Connection cable, 2 m
Cable, 120 mm, with plug M 12, 4 pin
Plug M 12, 4 pin
Plug M 8, 4 pin
VDE protection class M 12 9)
VDE protection class M 8 9) III
Protection type IP 67
Protection circuits 10) A, B, C
Ambient temperature 11) Operation –40 ... +60 °C
Storage –40 ... +75 °C
Weight
with connection cable 2 m/120 mm Approx. 80 g
with equipment plug M 12/M 8, 4 pin Approx. 20 g
WT 9-2
Scanning distance Ordering information
Technical data WT 9-2 P141 P441 N141 N441 P341 P641
1) Object with 90% reflectance
(referred to standard white DIN 5033)
2) Limit values
3) Must be within VS tolerances
4) Without load
5) Average service life at
room temperature 100,000 h
6) At TU = +25 °C and 100 mA
output current
7) With resistive load
8) With light/dark ratio 1:1
9) Withstand voltage 50 V
10) A = supply connections reverse
polarity protected
B = outputs short-circuit protected
C = interference suppression
11) Do not distort cable below 0 °C
Type
WT 9-2P141
WT 9-2P441
WT 9-2N141
WT 9-2N441
WT 9-2P341
WT 9-2P641
Order no.
1 018 301
1 018 303
1 018 302
1 018 304
1 019 274
1 019 275
Programming via Teach-In button.
Simple programming:
Position object in the beam and push the button:
finished;
LED confirms the Teach-In procedure.
Teach-In values can be stored.
Teach-In function
Two operating modes:
Default setting: short Teach-In time (< 8 s);
for standard applications;
approx. double reserve via switching threshold;
LED lights continuously.
Precise setting: long Teach-In time (> 8 s);
for precise applications;
small switching hysteresis;
LED blinks.
(mm) 4
1
10
100
8 12 16 20 24 28
Function reserve
1
3
2
6%
18%
90%
Operating
distance
WT 9-2
0(mm) 10 20 30
1
2
3
Scanning distance
10 22
10 20
10 24
Scanning range on white, 90 % reflectance
Scanning range on gray, 18% reflectance
1 Scanning range on black, 6% reflectance
2
3
8 SENSICK
WL 9-2 Photoelectric Reflex Switch, Standard
Without setting options
Dimension illustration
Red-light emitter LED as
alignment aid
Switching frequency 800/s
Outputs short-circuit protected
Scanning range
0 ... 4 m
12
22
40
20 3
3
1.5
1
2
2
29.5
11
3
4
Middle of optic axis
Mounting hole Ø 3.2 mm
LED signal strength indicator
Plug M 12 or M 8, 4 pin,
2 m connection cable or
120 mm cable with plug M 12, 4 pin
1
2
3
4
Photoelectric reflex switch
WL 9-2P130
WL 9-2P430
WL 9-2N130
WL 9-2N430
3
Cable receptacles
Adapter plate
Mounting bracket
Reflectors
Accessories
Connection type
L+
Q
Q
M
brn
wht
blu
blk
4 pin, M 12
WT 9-2P330
WT 9-2P630
WT 9-2P430
WT 9-2N430
1
L+
Q
Q
4
2
3
M
brn
wht
blu
blk
4 pin, M 8
1
L+
Q
Q
4
2
3
M
brn
wht
blu
blk
4 x 0,14 mm2
1
L+
Q
Q
4
2
3
M
brn
wht
blu
blk
4 pin, M 12 with 120 mm cable
WT 9-2P330 WT 9-2P130
WT 9-2N130
WT 9-2P630
SENSICK 9
Scanning range typ. max./on reflector 4 m/PL 80 A
Supply voltage VS
1) DC 10 ... 30 V
Ripple 2) ≤ 5 VPP
Current consumption 3) ≤ 30 mA
Light source LED, visible red light 4)
Angle of dispersion 2.5°
Light spot diameter 120 x 120 mm at a distance of 3 m
Switching outputs Q and Q– PNP
NPN
Signal voltage HIGH VS – 2.9 V
VS
Signal voltage LOW5) Approx. 0 V
≤ 2.9 V
Output current IA max. ≤ 100 mA
Response time 6) ≤ 625 μs
Max. switching frequency 7) 800/s
Connection technology Connection cable, 2 m
Cable, 120 mm, with plug M 12, 4 pin
Plug M 12, 4 pin
Plug M 8, 4 pin
VDE protection class M 12 8)
VDE protection class M 8 8) III
Protection type IP 67
Protection circuits 9) A, B, C
Ambient temperature 10) Operation –40 ... +60 °C
Storage –40 ... +75 °C
Weight
with connection cable 2 m/120 mm Approx. 80 g
with equipment plug M 12/M 8, 4 pin Approx. 20 g
WL 9-2
Scanning range Ordering information
Technical data WL 9-2 P130 P430 N130 N430 P330 P630
1) Limit values
2) Must be within VS tolerances
3) Without load
4) Average service life at
room temperature 100,000 h
5) At TU = +25 °C and 100 mA
output current
6) With resistive load
7) With light/dark ratio 1:1
8) Withstand voltage 50 V
19) A = supply connections reverse
polarity protected
B = outputs short-circuit protected
C = interference suppression
10) Do not distort cable below 0 °C
Type
WL 9-2P130
WL 9-2P430
WL 9-2N130
WL 9-2N430
WL 9-2P330
WL 9-2P630
Order no.
1 018 281
1 018 283
1 018 282
1 018 284
1 019 024
1 019 268
(m) 1 2 3 4 5
100
10
1
Function reserve
1
3
2 Operating
range
WL 9-2
Limiting
scanning range
0(m) 1 2 3 4 5
1
2
3
Operating range Scanning range typ. max.
0 3.0 4.0
0 2.0 3.0
0 0.6/1.0
Reflective tape 0 ... 0.6 m
Diamond Grade*
3
2 PL 40 A 0 ... 2 m
1 PL 80 A 0 ... 3 m
Reflector type Operating range
* 100 x 100 mm2
10 SENSICK
WL 9-2 Photoelectric Reflex Switch, Standard, Teach-In
Setting options
Dimension illustration
Red-light emitter LED as
alignment aid
Switching frequency 800/s
Outputs short-circuit protected
Teach-In
Scanning range
0 ... 4 m
12
22
40
20 3
3
1.5
1
2
2
29.5
11
3
4
Middle of optic axis
Mounting hole Ø 3.2 mm
LED signal strength indicator
Plug M 12 or M 8, 4 pin,
2 m connection cable or
120 mm cable with plug M 12, 4 pin
Sensitivity control, teachable
1
2
3
4
5
Photoelectric reflex switch
WL 9-2P131
WL 9-2P431
WL 9-2N131
WL 9-2N431
3
5
Cable receptacles
Adapter plate
Mounting bracket
Reflectors
Accessories
Connection type
L+
Q
Q
M
brn
wht
blu
blk
4 pin, M 12
WT 9-2P331
WT 9-2P631
WT 9-2P431
WT 9-2N431
1
L+
Q
Q
4
2
3
M
brn
wht
blu
blk
4 pin, M 8
1
L+
Q
Q
4
2
3
M
brn
wht
blu
blk
4 x 0,14 mm2
1
L+
Q
Q
4
2
3
M
brn
wht
blu
blk
4 pin, M 12 with 120 mm cable
WT 9-2P331 WT 9-2P131
WT 9-2N131
WT 9-2P631
SENSICK 11
Scanning range typ. max./on reflector 4 m/PL 80 A
Supply voltage VS
1) DC 10 ... 30 V
Ripple 2) ≤ 5 VPP
Current consumption 3) ≤ 30 mA
Light source LED, visible red light 4)
Angle of dispersion 2.5°
Light spot diameter 120 x 120 mm at a distance of 3 m
Switching outputs Q and Q– PNP
NPN
Signal voltage HIGH VS – 2.9 V
VS
Signal voltage LOW5) Approx. 0 V
≤ 2.9 V
Output current IA max. ≤ 100 mA
Response time 6) ≤ 625 μs
Max. switching frequency 7) 800/s
Connection technology Connection cable, 2 m
Cable, 120 mm, with plug M 12, 4 pin
Plug M 12, 4 pin
Plug M 8, 4 pin
VDE protection class M 12 8)
VDE protection class M 8 8) III
Protection type IP 67
Protection circuits 9) A, B, C
Ambient temperature 10) Operation –40 ... +60 °C
Storage –40 ... +75 °C
Weight
with connection cable 2 m/120 mm Approx. 80 g
with equipment plug M 12/M 8, 4 pin Approx. 20 g
WL 9-2
Scanning range Ordering information
Technical data WL 9-2 P131 P431 N131 N431 P331 P631
1) Limit values
2) Must be within VS tolerances
3) Without load
4) Average service life at
room temperature 100,000 h
5) At TU = +25 °C and 100 mA
output current
6) With resistive load
7) With light/dark ratio 1:1
8) Withstand voltage 50 V
19) A = supply connections reverse
polarity protected
B = outputs short-circuit protected
C = interference suppreasion
10) Do not distort cable below 0 °C
Type
WL 9-2P131
WL 9-2P431
WL 9-2N131
WL 9-2N431
WL 9-2P331
WL 9-2P631
Order no.
1 018 285
1 018 287
1 018 286
1 018 288
1 019 025
1 019 269
Programming via Teach-In button.
Simple programming:
Position reflector in the beam and push the button:
finished;
LED confirms the Teach-In procedure.
Teach-In values can be stored.
Teach-In function
Two operating modes:
Default setting: short Teach-In time (< 8 s);
for standard applications;
approx. double reserve via switching threshold;
LED lights continuously.
Precise setting: long Teach-In time (> 8 s);
for precise applications;
small switching hysteresis;
LED blinks.
(m) 1 2 3 4 5
100
10
1
Function reserve
1
3
2 Operating
range
WL 9-2
Limiting
scanning range
0(m) 1 2 3 4 5
1
2
3
Operating range Scanning range typ. max.
0 3.0 4.0
0 2.0 3.0
0 0.6/1.0
Reflective tape 0 ... 0.6 m
Diamond Grade*
3
2 PL 40 A 0 ... 2 m
1 PL 80 A 0 ... 3 m
Reflector type Operating range
* 100 x 100 mm2
12 SENSICK
WL 9-2 Photoelectric Reflex Switch, Focus 35 mm, Teach-In
Setting options
Dimension illustration
LED light source, visible red light
Sensitivity adjustment using the
Teach-In method
Switching frequency 800/s
Outputs short-circuit protected
Scanning range
0 ... 0.4 m
12
22
40
20 3
3
1.5
1
2
2
29.5
11
3
4
Middle of optic axis
Mounting hole Ø 3.2 mm
LED signal strength indicator
Plug M 12 or M 8, 4 pin,
2 m connection cable or
120 mm cable with plug M 12, 4 pin
Sensitivity control, teachable
1
2
3
4
5
Photoelectric reflex switch
WL 9-2P121
WL 9-2P421
WL 9-2N121
WL 9-2N421
3
5
Cable receptacles
Adapter plate
Mounting bracket
Reflectors
Accessories
Connection type
L+
Q
Q
M
brn
wht
blu
blk
4 pin, M 12
WT 9-2P321
WT 9-2P621
WT 9-2P421
WT 9-2N421
1
L+
Q
Q
4
2
3
M
brn
wht
blu
blk
4 pin, M 8
1
L+
Q
Q
4
2
3
M
brn
wht
blu
blk
4 x 0,14 mm2
1
L+
Q
Q
4
2
3
M
brn
wht
blu
blk
4 pin, M 12 with 120 mm cable
WT 9-2P321 WT 9-2P121
WT 9-2N121
WT 9-2P621
SENSICK 13
Scanning range typ. max./on reflector 0.4 m/PL 80 A
Supply voltage VS
1) DC 10 ... 30 V
Ripple 2) ≤ 5 VPP
Current consumption 3) ≤ 30 mA
Light source LED, visible red light 4)
Light spot diameter 1.5 x 1.5 mm at a distance of 35 mm
Switching outputs Q and Q– PNP
NPN
Signal voltage HIGH VS – 2.9 V
VS
Signal voltage LOW5) Approx. 0 V
≤ 2.9 V
Output current IA max. ≤ 100 mA
Response time 6) ≤ 625 μs
Max. switching frequency 7) 800/s
Connection technology Connection cable, 2 m
Cable, 120 mm, with plug M 12, 4 pin
Plug M 12, 4 pin
Plug M 8, 4 pin
VDE protection class M 12 8)
VDE protection class M 8 8) III
Protection type IP 67
Protection circuits 9) A, B, C
Ambient temperature 10) Operation –40 ... +60 °C
Storage –40 ... +75 °C
Weight
with connection cable 2 m/120 mm Approx. 80 g
with equipment plug M 12/M 8, 4 pin Approx. 20 g
WL 9-2
Scanning range Ordering information
Technical data WL 9-2 P121 P421 N121 N421 P321 P621
1) Limit values
2) Must be within VS tolerances
3) Without load
4) Average service life at
room temperature 100,000 h
5) At TU = +25 °C and 100 mA
output current
6) With resistive load
7) With light/dark ratio 1:1
8) Withstand voltage 50 V
19) A = supply connections reverse
polarity protected
B = outputs short-circuit protected
C = interference suppression
10) Do not distort cable below 0 °C
Type
WL 9-2P121
WL 9-2P421
WL 9-2N121
WL 9-2N421
WL 9-2P321
WL 9-2P621
Order no.
1 018 289
1 018 291
1 018 290
1 018 292
1 019 270
1 019 271
Programming via Teach-In button.
Simple programming:
Position reflector in the beam and push the button:
finished;
LED confirms the Teach-In procedure.
Teach-In values can be stored.
Teach-In function
Two operating modes:
Default setting: short Teach-In time (< 8 s);
for standard applications;
approx. double reserve via switching threshold;
LED lights continuously.
Precise setting: long Teach-In time (> 8 s);
for precise applications;
small switching hysteresis;
LED blinks.
(m) 0.1 0.2 0.3 0.4 0.5 0.6
100
10
1
Function reserve
Limiting
scanning range
Operating
range
WL 9-2
2
1
3
0(m) 0.1 0.2 0.3 0.4 0.5
1
2
3
Operating range Limiting scanning range
0 0.3 0.4
0 0.2 0.3
0 0,.1 0.2
Reflective tape 0 ... 0.25 m
Diamond Grade*
3
2 PL 40 A 0 ... 0.3 m
1 PL 80 A 0 ... 0.5 m
Reflector type Operating range
* 100 x 100 mm2
14
Accessoires
SENSICK
Dimension illustrations of reflectors
Reflector 20 x 40 mm
Order no.
1 012 719
Type
PL 20 A
Reflector 30 x 50 mm
Order no.
1 002 314
Type
PL 30 A
15
18
38
ø8
ø4.6
50
60
4.2
7.3
3.4
ø4.5
ø8
71
82
29.8 7.2
Reflector 40 x 60 mm
Order no.
1 012 720
Type
PL 40 A
Reflector hexagonal, SW 48 mm
Order no.
1 000 132
Type
PL 50 A
34
38 7.8
40.2
52
56.6
59.8
ø8.5
ø4.5
8
78
68
59
Reflector 80 x 80 mm
Order no.
1 003 865
Type
PL 80 A
Reflector ø 83 mm, center hole mounting
Order no.
5 304 549
Type
C 110
84
68
71
84
4.5
8
8.5
2.5
ø4.8
83 9
Also available as heatable model:
Continuous heating: PL 50HK,
Order no. 1 001 545
Regulated heating: PL 50HS,
Order no. 1 009 871
Reflective tape
fabricated
sheet 749 x 914 mm
Order no.
4 019 634
5 304 334
Type
REF-DG-K
REF-DG
Dimension illustrations and ordering information
15
Accessoires
SENSICK
Contact assignments according to
EN 50044
DC coding
Dimension illustrations of cable receptacles
Cable receptacles M 12, 4 pin, straight
Order no.
6 007 302
Cable lengths
–
5
ø18
M12x1
54
5
ø10.5
ø8.8
1.5
12
M12x1
14.5
27
25.5
42
Rmin 571)
Rmin 571)
38.3
12
45°
M12x1
26.5
14.5
12
1.5
ø8.8
ø10.5
Pin assignments
Pin 1 = brown
Pin 2 = white
Pin 3 = blue
Pin 4 = black
3
2
4
1
Pins
4
Type
DOS-1204-G
Cable receptacles M 12, 4 pin, angled
Order no.
6 007 303
Cable lengths
–
36
25
5
14.8
M12x1
ø18
36 5
20.5
Pins
4
Type
DOS-1204-W
Cable receptacles M 12, 4 pin, straight
Pins
4
4
4
Type
DOS-1204-G02M
DOS-1204-G05M
DOS-1204-G10M
Order no.
6 009 382
6 009 866
6 010 543
Cable lengths
2 m
5 m
10 m
Cable receptacles M 12, 4 pin, angled
Pins
4
4
4
Type
DOS-1204-W02M
DOS-1204-W05M
DOS-1204-W10M
Order no.
6 009 383
6 009 867
6 010 541
Cable lengths
2 m
5 m
10 m
Can be self-made for cables Ø 4.5 to 6.5 mm
1) Minimum bending radius with dynamic use
Can be self-made for cables Ø 4.5 to 6.5 mm
1) Minimum bending radius with dynamic use
Dimension illustrations and ordering information
16
Accessoires
SENSICK
Dimension illustrations and ordering information
ø 11.6
M 8x1
38.4 Cable diameter
max. 5.0 mm
28.0
ø 11.6
M 8x1
12.5
Cable diameter
max. 5.0 mm
SENSICK circular screwing system, M 8 plug, 4 pin, enclosure rating IP 67
M 8 cable receptacle, 4 pin, straight
Type
DOS-0804-G
Order no.
6 009 974
M 8 cable receptacles, 4 pin, angled
Type
DOS-0804-W
Order no.
6 009 975
2/wht 1/brn
4/blk 3/blu
ø 10
30.5
Rmin1)
M 8x1
3/blu
6
26
M 8x1
16.5
ø 10
Rmin1)
1/brn
4/blk
2/wht
M 8 cable receptacle, 4 pin, straight M 8 cable receptacles, 4 pin, angled
Cable diameter 5 mm, 4 x 0.25 mm2, PVC coating Cable diameter 5 mm, 4 x 0.25 mm2, PVC coating
Cable length
2 m
5 m
10 m
Type
DOL-0804-G02M
DOL-0804-G05M
DOL-0804-G10M
Order no.
6 009 870
6 009 872
6 010 754
Cable length
2 m
5 m
10 m
Type
DOL-0804-W02M
DOL-0804-W05M
DOL-0804-W10M
Order no.
6 009 871
6 009 873
6 010 755
1) Minimum bending radius with dynamic use
Rmin= 20x cable diameter
SENSICK 17
Dimension illustration adapter plate
Adapter plate
Order no.
4 033 145
Type
BEF-AP-W9
22
63.25
1
8.25
5
3.25
20 3
M 3
ø 3.2
Dimension illustration mounting bracket
Mounting bracket
Order no.
4 033 146
Type
BEF-WN-W9-2
44
1 4
4
3.5 5
6
14.8
12
6.4
8
14.8
16
17
17
Accessoires
Dimension illustrations and ordering information
8 008 988.0700 HJS • SM • Printed in Germany • We reserve the right to make changes
Contact:
Au s t r a l i a
Phone +61 3 94 97 41 00
0 08 33 48 02 – toll free
Fax +61 3 94 97 11 87
Au s t r i a
Phone +43 2 23 66 22 88-0
Fax +43 2 23 66 22 88-5
Bel g i u m / Luxembourg
Phone +32 24 66 55 66
Fax +32 24 63 35 07
Br a z i l
Phone +55 11 55 61 26 83
Fax +55 11 5 35 41 53
C h i n a / Ho n g Kong
Phone +8 52 27 63 69 66
Fax +8 52 27 63 63 11
Czech Re p u b l i c
Phone +42 02 57 81 05 61
Fax +42 02 57 81 05 59
Denmark
Phone +45 45 82 64 00
Fax +45 45 82 64 01
F i n l a n d
Phone +3 58 9-7 28 85 00
Fax +3 58 9-72 88 50 55
Fr a n c e
Phone +33 1-64 62 35 00
Fax +33 1-64 62 35 77
Germany
Phone +49 76 81 2 02-0
Fax +49 76 81 2 02-36 09
G re a t B r i t a i n
Phone +44 17 27-83 11 21
Fax +44 17 27-85 67 67
I t a l y
Phone +390 2-92 14 20 62
Fax +390 2-92 14 20 67
Japan
Phone +813 33 58-13 41
Fax +813 33 58-05 86
Ne t h e r l a n d s
Phone +31 3 02 29 25 44
Fax +31 3 02 29 39 94
Nor w a y
Phone +47 67 56 75 00
Fax +47 67 56 66 10
P o l a n d
Phone +48 2 26 44-83 45
Fax +48 2 26 44-83 42
Si n g a p o re
Phone +65 7 44 37 32
Fax +65 8 41 77 47
Sp a i n
Phone +34 9 34 80 31 00
Fax +34 9 34 73 44 69
Sweden
Phone +46 8-6 80 64 50
Fax +46 8-7 10 18 75
S w i t z e r l a n d
Phone +41 4 16 19 29 39
Fax +41 4 16 19 29 21
Ta i w a n
Phone +88 62 23 65 62 92
Fax +88 62 23 68 73 97
USA
Phone +1 9 52 9 41-67 80
Fax +1 9 52 9 41-92 87
Representatives and agencies
in all major industrial nations.
SICK AG • Industrial Sensors • Sebastian-Kneipp-Straße 1 • D-79183 Waldkirch
Phone +49/76 81/2 02-0 • Fax +49/76 81/2 02-36 09 • www.sick.de
1. Product profile
1.1 General description
The BGA7124 MMIC is a one-stage amplifier, available in a low-cost leadless
surface-mount package. It delivers 25 dBm output power at 1 dB gain compression and
superior performance up to 2700 MHz. Its power saving features include easy quiescent
current adjustment enabling class-AB operation and logic-level shutdown control to
reduce the supply current to 4 μA.
1.2 Features and benefits
400 MHz to 2700 MHz frequency operating range
16 dB small signal gain at 2 GHz
25 dBm output power at 1 dB gain compression
Integrated active biasing
External matching allows broad application optimization of the electrical performance
3.3 V or 5 V single supply operation
All pins ESD protected
1.3 Applications
1.4 Quick reference data
[1] The supply current is adjustable; see Section 8.1 “Supply current adjustment”.
[2] Operation outside this range is possible but not guaranteed.
[3] PL = 11 dBm per tone; spacing = 1 MHz.
BGA7124
400 MHz to 2700 MHz 0.25 W high linearity silicon amplifier
Rev. 3 — 9 September 2010 Product data sheet
Wireless infrastructure (base station,
repeater, backhaul systems)
E-metering
Broadband CPE/MoCA Satellite Master Antenna TV (SMATV)
Industrial applications WLAN/ISM/RFID
Table 1. Quick reference data
Input and output impedances matched to 50 Ω, SHDN = HIGH (shutdown disabled). Typical values
at VCC = 5 V; ICC = 130 mA; Tcase = 25 °C; unless otherwise specified.
Symbol Parameter Conditions Min Typ Max Unit
ICC supply current VCC = 5.0 V [1] 50 - 170 mA
f frequency [2] 400 - 2700 MHz
Gp power gain f = 2140 MHz 14.5 16 17.5 dB
PL(1dB) output power at 1 dB gain compression f = 2140 MHz 23.5 24.5 - dBm
IP3O output third-order intercept point f = 2140 MHz [3] 34.5 37.5 - dBm
BGA7124 All information provided in this document is subject to legal disclaimers. © NXP B.V. 2010. All rights reserved.
Product data sheet Rev. 3 — 9 September 2010 2 of 33
NXP Semiconductors BGA7124
400 MHz to 2700 MHz 0.25 W high linearity silicon amplifier
2. Pinning information
2.1 Pinning
2.2 Pin description
[1] This pin is DC-coupled and requires an external DC-blocking capacitor.
[2] RF decoupled.
[3] The center metal base of the SOT908-1 also functions as heatsink for the power amplifier.
3. Ordering information
Fig 1. HVSON8 package pin configuration
014aab046
VCC(BIAS)
VCC(RF) SHDN
VCC(RF) RF_IN
ICQ_ADJ
GND PAD
n.c.
Transparent top view
4 5
3 6
2 7
1 8
terminal 1
index area
BGA7124
n.c.
Table 2. Pin description
Symbol Pin Description
n.c. 1, 4 not connected
VCC(RF) 2, 3 RF output for the power amplifier and DC supply input for the
RF transistor collector [1]
VCC(BIAS) 5 bias supply voltage [2]
SHDN 6 shutdown control function enabled/disabled
RF_IN 7 RF input for the power amplifier [1]
ICQ_ADJ 8 quiescent collector current adjustment controlled by an external resistor
GND GND pad RF and DC ground[3]
Table 3. Ordering information
Type number Package
Name Description Version
BGA7124 HVSON8 plastic thermal enhanced very thin small outline
package; no leads; 8 terminals; body 3 × 3 × 0.85 mm
SOT908-1
BGA7124 All information provided in this document is subject to legal disclaimers. © NXP B.V. 2010. All rights reserved.
Product data sheet Rev. 3 — 9 September 2010 3 of 33
NXP Semiconductors BGA7124
400 MHz to 2700 MHz 0.25 W high linearity silicon amplifier
4. Functional diagram
5. Shutdown control
Fig 2. Functional diagram
BANDGAP
INPUT MATCH OUTPUT MATCH
BIAS
ENABLE
V/I
CONVERTER
RF_OUT
GND
R1
R2
RF_IN
SHDN
VCC
ICQ_ADJ
6
7
5 8
2, 3
014aab047
VCC(BIAS)
VCC(RF)
Table 4. Shutdown control settings
Mode Mode description Function description Pin
SHDN
Vctrl(sd) (V) Ictrl(sd) (μA)
Min Max Min Max
Idle medium power MMIC fully off;
minimal supply current
shutdown control enabled 0 0 0.7 - 2
TX medium power MMIC transmit mode shutdown control disabled 1 2.5 VCC(BIAS)- 9
BGA7124 All information provided in this document is subject to legal disclaimers. © NXP B.V. 2010. All rights reserved.
Product data sheet Rev. 3 — 9 September 2010 4 of 33
NXP Semiconductors BGA7124
400 MHz to 2700 MHz 0.25 W high linearity silicon amplifier
6. Limiting values
[1] See Figure 3 for safe operating area.
[2] The supply current is adjustable; see Section 8.1 “Supply current adjustment”.
[3] If Vctrl(sd) exceeds VCC(BIAS), the internal ESD circuit can be damaged. To prevent this, it is recommended that the Ictrl(sd) is limited to
20 mA. If the SHDN function is not used, the SHDN pin should be connected to the VCC(BIAS) pin.
Table 5. Limiting values
In accordance with the Absolute Maximum Rating System (IEC 60134).
Symbol Parameter Conditions Min Max Unit
VCC(RF) RF supply voltage [1]- 6.0 V
VCC(BIAS) bias supply voltage [1]- 6.0 V
ICC supply current [1][2] 50 200 mA
Vctrl(sd) shutdown control voltage [3] 0.0 VCC(BIAS) V
Pi(RF) RF input power - 20 dBm
Tcase case temperature −40 +85 °C
Tj junction temperature - 150 °C
VESD electrostatic discharge voltage Human Body Model (HBM);
According JEDEC standard 22-A114E
- 2000 V
Charged Device Model (CDM);
According JEDEC standard 22-C101B
- 500 V
Exceeding the safe operating area limits may cause serious damage to the product.
The impact on ICC due to the spread of the external ICQ resistor (R2) should be taken into account.
The product-spread on ICC should be taken into account (see Section 8 “Static characteristics”).
Fig 3. BGA7124 DC safe operating area
VCC(RF) (V)
2 3 4 5 6 7
014aab048
150
100
200
250
ICC
(mA)
50
BGA7124 All information provided in this document is subject to legal disclaimers. © NXP B.V. 2010. All rights reserved.
Product data sheet Rev. 3 — 9 September 2010 5 of 33
NXP Semiconductors BGA7124
400 MHz to 2700 MHz 0.25 W high linearity silicon amplifier
7. Thermal characteristics
[1] defined as thermal resistance from junction to GND paddle.
8. Static characteristics
[1] The supply current is adjustable; see Section 8.1 “Supply current adjustment”.
[2] See Section 12 “Application information”.
8.1 Supply current adjustment
The supply current can be adjusted by changing the value of external ICQ resistor (R2);
(see Figure 4).
Table 6. Thermal characteristics
Symbol Parameter Conditions Typ Max Unit
Rth(j-mb) thermal resistance from junction to
mounting base
Tcase = 85 °C; VCC = 5 V;
ICC = 130 mA
[1] 32 - K/W
Table 7. Characteristics
Input and output impedances matched to 50 Ω, pin SHDN = HIGH (shutdown disabled). Typical
values at VCC = 3.3 V or VCC = 5 V; Tcase = 25°C; unless otherwise specified.
Symbol Parameter Conditions Min Typ Max Unit
ICC supply current VCC = 3.3 V [1] 50 - 200 mA
R1 = 0 Ω; R2 = 1330 Ω [2] 115 130 145 mA
R1 = 2.2 Ω; R2 = 1070 Ω [2] 135 160 185 mA
VCC = 5.0 V [1] 50 - 170 mA
R1 = 0 Ω; R2 = 1960 Ω [2] 110 130 150 mA
R1 = 2.2 Ω; R2 = 1650 Ω [2] 125 150 175 mA
during shutdown; pin
SHDN = LOW (shutdown enabled)
- 4 6 μA
BGA7124 All information provided in this document is subject to legal disclaimers. © NXP B.V. 2010. All rights reserved.
Product data sheet Rev. 3 — 9 September 2010 6 of 33
NXP Semiconductors BGA7124
400 MHz to 2700 MHz 0.25 W high linearity silicon amplifier
9. Dynamic characteristics
a. 5 V supply voltage. b. 3.3 V supply voltage
Fig 4. Supply current as a function of the value of R2
VCC = 5 V; R1 = 0
R2 (kΩ)
1.6 2.0 2.4 2.8 3.2 3.6 4.0 4.4
014aab049
90
130
170
ICC
(mA)
50
VCC = 3.3 V; R1 = 0
R2 (kΩ)
0.9 1.4 1.9 2.4 2.9 3.4
014aab050
110
140
80
170
200
ICC
(mA)
50
Table 8. Characteristics at VCC = 5 V
Input and output impedances matched to 50 Ω, pin SHDN = HIGH (shutdown disabled). Typical values at VCC = 5 V;
ICC = 130 mA; Tcase = 25°C; see Section 12 “Application information”; unless otherwise specified.
Symbol Parameter Conditions Min Typ Max Unit
f frequency [1] 400 - 2700 MHz
Gp power gain for small signals
f = 940 MHz - 22.7 - dB
f = 1960 MHz - 16.4 - dB
f = 2140 MHz 14.5 16.0 17.5 dB
f = 2445 MHz [2] - 14.2 - dB
PL(1dB) output power at 1 dB gain compression f = 940 MHz - 25.0 - dBm
f = 1960 MHz - 24.5 - dBm
f = 2140 MHz 23.5 24.5 - dBm
f = 2445 MHz [2] - 23.5 - dBm
IP3O output third-order intercept point f = 940 MHz [3] - 38.5 - dBm
f = 1960 MHz [3] - 38.0 - dBm
f = 2140 MHz [3] 34.5 37.5 - dBm
f = 2445 MHz [2][3] - 36.0 - dBm
NF noise figure f = 940 MHz [4]- 5.2 - dB
f = 1960 MHz [4]- 4.6 - dB
f = 2140 MHz [4]- 4.8 6.5 dB
f = 2445 MHz [2][4]- 5.4 - dB
BGA7124 All information provided in this document is subject to legal disclaimers. © NXP B.V. 2010. All rights reserved.
Product data sheet Rev. 3 — 9 September 2010 7 of 33
NXP Semiconductors BGA7124
400 MHz to 2700 MHz 0.25 W high linearity silicon amplifier
[1] Operation outside this range is possible but not guaranteed.
[2] ICC = 150 mA; see Section 12 “Application information”.
[3] PL = 11 dBm per tone; spacing = 1 MHz.
[4] Defined at Pi = −40 dBm; small signal conditions.
RLin input return loss f = 940 MHz - −15 - dB
f = 1960 MHz - −11 - dB
f = 2140 MHz - −17 - dB
f = 2445 MHz [2] - −13 - dB
RLout output return loss f = 940 MHz - −8 - dB
f = 1960 MHz - −12 - dB
f = 2140 MHz - −15 - dB
f = 2445 MHz [2] - −25 - dB
Table 8. Characteristics at VCC = 5 V …continued
Input and output impedances matched to 50 Ω, pin SHDN = HIGH (shutdown disabled). Typical values at VCC = 5 V;
ICC = 130 mA; Tcase = 25°C; see Section 12 “Application information”; unless otherwise specified.
Symbol Parameter Conditions Min Typ Max Unit
BGA7124 All information provided in this document is subject to legal disclaimers. © NXP B.V. 2010. All rights reserved.
Product data sheet Rev. 3 — 9 September 2010 8 of 33
NXP Semiconductors BGA7124
400 MHz to 2700 MHz 0.25 W high linearity silicon amplifier
[1] Operation outside this range is possible but not guaranteed.
[2] ICC = 160 mA; see Section 12 “Application information”.
[3] PL= 11 dBm per tone; spacing = 1 MHz.
[4] Defined at Pi = −40 dBm; small signal conditions.
Table 9. Characteristics at VCC = 3.3 V
Input and output impedances matched to 50 Ω, pin SHDN = HIGH (shutdown disabled). Typical values at VCC = 3.3 V;
ICC = 130 mA; Tcase = 25°C, see Section 12 “Application information”; unless otherwise specified.
Symbol Parameter Conditions Min Typ Max Unit
f frequency [1] 400 - 2700 MHz
Gp power gain for small signals
f = 940 MHz - 22.5 - dB
f = 2445 MHz [2]- 13.8 - dB
PL(1dB) output power at 1 dB gain compression f = 940 MHz - 23.5 - dBm
f = 2445 MHz [2]- 22.0 - dBm
IP3O output third-order intercept point f = 940 MHz [3]- 36.4 - dBm
f = 2445 MHz [2][3]- 35.2 - dBm
NF noise figure f = 940 MHz [4]- 5.5 - dB
f = 2445 MHz [2][4]- 5.5 - dB
RLin input return loss f = 940 MHz - −15 - dB
f = 2445 MHz [2] - −10 - dB
RLout output return loss f = 940 MHz - −9 - dB
f = 2445 MHz [2] - −25 - dB
BGA7124 All information provided in this document is subject to legal disclaimers. © NXP B.V. 2010. All rights reserved.
Product data sheet Rev. 3 — 9 September 2010 9 of 33
NXP Semiconductors BGA7124
400 MHz to 2700 MHz 0.25 W high linearity silicon amplifier
9.1 Scattering parameters
Table 10. Scattering parameters at 5 V, MMIC only
VCC = 5 V; ICC = 130mA; Tcase = 25°C.
f (MHz) s11 s21 s12 s22
Magnitude
(ratio)
Angle
(degree)
Magnitude
(ratio)
Angle
(degree)
Magnitude
(ratio)
Angle
(degree)
Magnitude
(ratio)
Angle
(degree)
400 0.85 161.56 22.94 82.35 0.01 17.02 0.46 −156.50
500 0.90 159.44 11.82 82.58 0.01 27.08 0.63 176.13
600 0.90 152.15 9.98 73.86 0.01 24.10 0.64 169.61
700 0.89 145.75 8.59 66.00 0.01 21.41 0.64 164.34
800 0.88 139.33 7.55 58.86 0.02 18.47 0.65 159.29
900 0.87 133.19 6.74 51.66 0.02 14.00 0.65 154.44
1000 0.87 127.07 6.14 45.11 0.02 11.25 0.65 149.58
1100 0.87 120.67 5.61 38.20 0.02 7.99 0.65 144.25
1200 0.87 114.18 5.19 31.60 0.02 4.20 0.64 139.60
1300 0.86 107.68 4.82 25.08 0.02 0.31 0.64 134.85
1400 0.86 100.86 4.51 18.49 0.02 −4.01 0.63 130.13
1500 0.86 94.14 4.23 11.74 0.02 −8.65 0.63 125.02
1600 0.86 87.48 3.99 5.25 0.03 −13.15 0.63 120.13
1700 0.86 80.83 3.77 −1.50 0.03 −18.16 0.62 114.98
1800 0.86 74.14 3.56 −8.13 0.03 −23.28 0.62 109.78
1900 0.86 67.39 3.37 −14.94 0.03 −28.54 0.62 104.46
2000 0.86 60.70 3.19 −21.68 0.03 −33.68 0.63 99.01
2100 0.86 53.97 3.02 −28.68 0.03 −39.37 0.63 93.58
2200 0.86 47.78 2.85 −35.14 0.03 −44.84 0.63 88.17
2300 0.86 41.57 2.69 −41.70 0.03 −50.27 0.64 83.06
2400 0.86 35.43 2.54 −48.11 0.03 −55.62 0.64 78.10
2500 0.86 29.74 2.39 −54.19 0.04 −60.71 0.65 73.31
2600 0.86 24.79 2.27 −60.06 0.04 −65.48 0.65 68.64
2700 0.85 19.58 2.15 −66.14 0.04 −70.66 0.66 64.16
BGA7124 All information provided in this document is subject to legal disclaimers. © NXP B.V. 2010. All rights reserved.
Product data sheet Rev. 3 — 9 September 2010 10 of 33
NXP Semiconductors BGA7124
400 MHz to 2700 MHz 0.25 W high linearity silicon amplifier
10. Reliability information
11. Moisture sensitivity
Table 11. Scattering parameters at 3.3 V, MMIC only
VCC = 3.3 V; ICC = 130mA; Tcase = 25°C.
f (MHz) s11 s21 s12 s22
Magnitude
(ratio)
Angle
(degree)
Magnitude
(ratio)
Angle
(degree)
Magnitude
(ratio)
Angle
(degree)
Magnitude
(ratio)
Angle
(degree)
400 0.84 161.94 21.25 73.81 0.01 17.66 0.57 −154.41
500 0.91 159.25 11.56 79.01 0.01 28.15 0.65 178.05
600 0.90 151.98 9.67 70.71 0.01 24.80 0.66 171.32
700 0.90 145.57 8.29 63.37 0.01 21.89 0.66 165.59
800 0.89 139.18 7.26 56.54 0.02 19.04 0.66 160.37
900 0.88 132.87 6.48 49.74 0.02 15.35 0.66 155.28
1000 0.88 126.78 5.90 43.30 0.02 11.89 0.66 150.23
1100 0.87 120.46 5.39 36.53 0.02 8.33 0.66 144.88
1200 0.87 113.94 4.97 30.05 0.02 4.50 0.65 140.03
1300 0.87 107.48 4.62 23.62 0.02 0.35 0.65 135.35
1400 0.87 100.69 4.32 17.15 0.02 −3.92 0.64 130.48
1500 0.86 93.93 4.05 10.48 0.02 −8.62 0.64 125.46
1600 0.86 87.28 3.81 4.05 0.03 −13.28 0.64 120.31
1700 0.86 80.71 3.61 −2.66 0.03 −18.26 0.64 115.13
1800 0.86 74.00 3.40 −9.21 0.03 −23.51 0.64 109.99
1900 0.86 67.27 3.22 −15.97 0.03 −28.87 0.63 104.66
2000 0.86 60.64 3.05 −22.71 0.03 −34.22 0.64 99.36
2100 0.86 53.84 2.89 −29.68 0.03 −39.95 0.64 93.93
2200 0.86 47.60 2.72 −36.12 0.03 −45.44 0.64 88.55
2300 0.86 41.43 2.57 −42.66 0.03 −51.06 0.65 83.38
2400 0.86 35.35 2.42 −49.01 0.04 −56.53 0.65 78.44
2500 0.85 29.64 2.28 −55.12 0.04 −61.72 0.66 73.56
2600 0.85 24.72 2.16 −60.91 0.04 −66.76 0.66 68.80
2700 0.85 19.59 2.04 −66.91 0.04 −71.84 0.67 64.30
Table 12. Reliability
Life test Conditions Intrinsic failure rate
HTOL According JESD85; confidence level 60 %; Tj = 55 °C;
activation energy = 0.7 eV; acceleration factor determined
according Arrhenius
4
Table 13. Moisture sensitivity level
Test methodology Class
JESD-22-A113 1
BGA7124 All information provided in this document is subject to legal disclaimers. © NXP B.V. 2010. All rights reserved.
Product data sheet Rev. 3 — 9 September 2010 11 of 33
NXP Semiconductors BGA7124
400 MHz to 2700 MHz 0.25 W high linearity silicon amplifier
12. Application information
12.1 5 V applications
12.1.1 920 MHz to 960 MHz
See Table 14 for a list of components.
PCB board specification: Rogers RO4003C; Height = 0.508 mm; εr = 3.38; Copper thickness = 35 μm.
Fig 5. 5 V/130 mA application schematic; 920 MHz to 960 MHz
C3
C10
C4
C6
C8
C9 C7
R1
R2
ICQ_ADJ SHDN
enable
L1
L2
C2
MSL1 C1 MSL2 MSL3 MSL5 MSL6 MSL7 MSL8 RF_IN
J1
J3
J2
RF_OUT
BGA7124
50 Ω 50 Ω
VCC
C5
014aab051
V MSL4 CC(RF)
VCC(BIAS)
(1) Tcase = −40 °C.
(2) Tcase = 25 °C.
(3) Tcase = 85 °C.
(1) Tcase = −40 °C.
(2) Tcase = 25 °C.
(3) Tcase = 85 °C.
Fig 6. Output power at 1 dB gain compression as a
function of frequency
Fig 7. Power gain as a function of frequency
f (GHz)
0.92 0.93 0.94 0.95 0.96
014aab052
24
26
22
28
30
PL(1dB)
(dBm)
20
(1)
(2)
(3)
f (GHz)
0.92 0.93 0.94 0.95 0.96
014aab053
22
24
20
26
28
Gp
(dB)
18
(1)
(2)
(3)
BGA7124 All information provided in this document is subject to legal disclaimers. © NXP B.V. 2010. All rights reserved.
Product data sheet Rev. 3 — 9 September 2010 12 of 33
NXP Semiconductors BGA7124
400 MHz to 2700 MHz 0.25 W high linearity silicon amplifier
Tcase = 25 °C. (1) Tcase = −40 °C.
(2) Tcase = 25 °C.
(3) Tcase = 85 °C.
Fig 8. Input return loss, output return loss and
isolation as a function of frequency
Fig 9. Output third-order intercept point as a function
of frequency
RLout
RLin
ISL
f (GHz)
0.92 0.93 0.94 0.95 0.96
014aab054
−20
−10
0
RLin, RLout, ISL
(dB)
−30
f (GHz)
0.92 0.93 0.94 0.95 0.96
014aab055
38
40
42
IP3O
(dBm)
36
(1)
(3)
(2)
See Table 14 for a list of components.
Fig 10. 5 V/130 mA application reference board; 920 MHz to 960 MHz
J3
GND
VCC
GND
n.c.
enable
GND
C9
C10
C8
C6
C4 C5
L2
C1
C3
R2
C2
L1
C7
R1
MSL6 MSL7
MSL4 MSL5
MSL1 MSL3 MSL8
MSL2
J1
J I HG F E D C B A 1 2 3 4 5 6 7 8 910
11
12
13
RF in
J2
RF out
014aab056
BGA7124 All information provided in this document is subject to legal disclaimers. © NXP B.V. 2010. All rights reserved.
Product data sheet Rev. 3 — 9 September 2010 13 of 33
NXP Semiconductors BGA7124
400 MHz to 2700 MHz 0.25 W high linearity silicon amplifier
[1] MSL1 to MSL8 dimensions specified as Width (W), Spacing (S) and Length (L).
Table 14. 5 V/130 mA application list of components; 920 MHz to 960 MHz
See Figure 5 and Figure 10 for component layout. Printed-Circuit Board (PCB): Rogers RO4003C stack; height = 0.508 mm;
copper plating thickness = 35 μm.
Component Description Value Function Remarks
C1, C6 capacitor 68 pF DC blocking Murata GRM1885C1H680JA01D
C2, C3 capacitor 3.3 pF input match Murata GRM1885C1H3R3CZ01D
C4 capacitor 3.9 pF output match Murata GRM1885C1H3R9CZ01D
C5 capacitor 1.0 pF output match Murata GRM1885C1H1R0CZ01D
C7 capacitor 68 pF RF decoupling Murata GRM1885C1H680JA01D
C8 capacitor 100 nF DC decoupling AVX 0603YC104KAT2A
C9 capacitor 10 μF DC decoupling AVX 1206ZG106ZAT2A
C10 capacitor 12 pF noise decoupling Murata GRM1555C1H120JZ01D
J1, J2 RF connector SMA Emerson Network Power
142-0701-841
J3 DC connector 6-pins MOLEX
L1 inductor 2.2 nH output match Tyco electronics 36501J2N2JTDG
L2 inductor 22 nH DC feed Tyco electronics 36501J022JTDG
MSL1[1] micro stripline 1.14 mm × 0.8 mm × 10.95 mm input match
MSL2[1] micro stripline 1.14 mm × 0.8 mm × 2.95 mm input match
MSL3[1] micro stripline 1.14 mm × 0.8 mm × 7.75 mm input match
MSL4[1] micro stripline 1.14 mm × 0.8 mm × 23.4 mm output match
MSL5[1] micro stripline 1.14 mm × 0.8 mm × 2.2 mm output match
MSL6[1] micro stripline 1.14 mm × 0.8 mm × 3.15 mm output match
MSL7[1] micro stripline 1.14 mm × 0.8 mm × 2.3 mm output match
MSL8[1] micro stripline 1.14 mm × 0.8 mm × 10.95 mm output match
R1 resistor 0 Ω Multicomp MC 0.063W 0603 0R
R2 resistor
(trimmer)
2 kΩ bias adjustment Bourns 3214W-1-202E
BGA7124 All information provided in this document is subject to legal disclaimers. © NXP B.V. 2010. All rights reserved.
Product data sheet Rev. 3 — 9 September 2010 14 of 33
NXP Semiconductors BGA7124
400 MHz to 2700 MHz 0.25 W high linearity silicon amplifier
12.1.2 1930 MHz to 1990 MHz
See Table 15 for a list of components.
PCB board specification: Rogers RO4003C; Height = 0.508 mm; εr = 3.38; Copper thickness = 35 μm.
Fig 11. 5 V/130 mA application schematic; 1930 MHz to 1990 MHz
C3
C4
C6
C7 C5
R1
R2
ICQ_ADJ SHDN
enable
L1
C2
MSL1 C1 MSL2 MSL4 MSL5 MSL6 RF_IN
RF_OUT
BGA7124
50 Ω 50 Ω
VCC
014aab057
MSL3
VCC(BIAS)
VCC(RF)
J1
J3
J2
(1) Tcase = −40 °C.
(2) Tcase = 25 °C.
(3) Tcase = 85 °C.
(1) Tcase = −40 °C.
(2) Tcase = 25 °C.
(3) Tcase = 85 °C.
Fig 12. Output power at 1 dB gain compression as a
function of frequency
Fig 13. Power gain as a function of frequency
014aab058
f (GHz)
1.93 1.95 1.97 1.99
24
26
22
28
30
PL(1dB)
(dBm)
20
(1)
(2)
(3)
014aab059
f (GHz)
1.93 1.95 1.97 1.99
14
16
12
18
20
Gp
(dB)
10
(1)
(2)
(3)
BGA7124 All information provided in this document is subject to legal disclaimers. © NXP B.V. 2010. All rights reserved.
Product data sheet Rev. 3 — 9 September 2010 15 of 33
NXP Semiconductors BGA7124
400 MHz to 2700 MHz 0.25 W high linearity silicon amplifier
Tcase = 25 °C. (1) Tcase = −40 °C.
(2) Tcase = 25 °C.
(3) Tcase = 85 °C.
Fig 14. Input return loss, output return loss and
isolation as a function of frequency
Fig 15. Output third-order intercept point as a function
of frequency
RLout
RLin
ISL
f (GHz)
1.93 1.95 1.97 1.99
014aab060
−20
−10
0
RLin, RLout, ISL
(dB)
−30
f (GHz)
1.93 1.95 1.97 1.99
014aab061
36
38
40
34
IP3O
(dBm) (2)
(1)
(3)
See Table 15 for a list of components.
Fig 16. 5 V/130 mA application reference board; 1930 MHz to 1990 MHz
J3
GND
VCC
GND
n.c.
enable
GND
C7
C6
C4
C2 C3
C1
R2
L1
C5
R1
MSL6
MSL4 MSL5
MSL1 MSL2 MSL3
J1
J I HG F E D C B A 1 2 3 4 5 6 7 8 910
11
12
13
RF in
J2
RF out
014aab062
BGA7124 All information provided in this document is subject to legal disclaimers. © NXP B.V. 2010. All rights reserved.
Product data sheet Rev. 3 — 9 September 2010 16 of 33
NXP Semiconductors BGA7124
400 MHz to 2700 MHz 0.25 W high linearity silicon amplifier
[1] MSL1 to MSL6 dimensions specified as Width (W), Spacing (S) and Length (L).
12.1.3 2110 MHz to 2170 MHz
Table 15. 5 V/130 mA application list of components; 1930 MHz to 1990 MHz
See Figure 11 and Figure 16 for component layout. Printed-Circuit Board (PCB): Rogers RO4003C stack; height = 0.508 mm;
copper plating thickness = 35 μm.
Component Description Value Function Remarks
C1, C4 capacitor 15 pF DC blocking Murata GRM1885C1H150JA01D
C2 capacitor 2.2 pF input match Murata GRM1885C1H2R2CZ01D
C3 capacitor 1.2 pF output match Murata GRM1885C1H1R2CZ01D
C5 capacitor 15 pF RF decoupling Murata GRM1885C1H150JA01D
C6 capacitor 100 nF DC decoupling AVX 0603YC104KAT2A
C7 capacitor 10 μF DC decoupling AVX 1206ZG106ZAT2A
J1, J2 RF connector SMA Emerson Network Power
142-0701-841
J3 DC connector 6-pins MOLEX
L1 inductor 22 nH DC feed Tyco electronics 36501J022JTDG
MSL1[1] micro stripline 1.14 mm × 0.8 mm × 10.95 mm input match
MSL2[1] micro stripline 1.14 mm × 0.8 mm × 10.8 mm input match
MSL3[1] micro stripline 1.14 mm × 0.8 mm × 5.8 mm output match
MSL4[1] micro stripline 1.14 mm × 0.8 mm × 2.2 mm output match
MSL5[1] micro stripline 1.14 mm × 0.8 mm × 3.7 mm output match
MSL6[1] micro stripline 1.14 mm × 0.8 mm × 10.95 mm output match
R1 resistor 0 Ω Multicomp MC 0.063W 0603 0R
R2 resistor (trimmer) 2 kΩ bias adjustment Bourns 3214W-1-202E
See Table 16 for a list of components.
PCB board specification: Rogers RO4003C; Height = 0.508 mm; εr = 3.38; Copper thickness = 35 μm.
Fig 17. 5 V/130 mA application schematic; 2110 MHz to 2170 MHz
RF_OUT
C3
C4
C6
C7 C5
R1
R2
ICQ_ADJ SHDN
enable
L1
C2
MSL1 C1 MSL2 MSL4 MSL5 MSL6 RF_IN
BGA7124
50 Ω 50 Ω
VCC
014aab063
MSL3
VCC(BIAS)
VCC(RF)
J1
J3
J2
BGA7124 All information provided in this document is subject to legal disclaimers. © NXP B.V. 2010. All rights reserved.
Product data sheet Rev. 3 — 9 September 2010 17 of 33
NXP Semiconductors BGA7124
400 MHz to 2700 MHz 0.25 W high linearity silicon amplifier
(1) Tcase = −40 °C.
(2) Tcase = 25 °C.
(3) Tcase = 85 °C.
(1) Tcase = −40 °C.
(2) Tcase = 25 °C.
(3) Tcase = 85 °C.
Fig 18. Output power at 1 dB gain compression as a
function of frequency
Fig 19. Power gain as a function of frequency
014aab064
f (GHz)
2.11 2.13 2.15 2.17
24
26
22
28
30
PL(1dB)
(dBm)
20
(1)
(2)
(3)
014aab065
f (GHz)
2.11 2.13 2.15 2.17
14
16
12
18
20
Gp
(dB)
10
(1)
(2)
(3)
Tcase = 25 °C. (1) Tcase = −40 °C.
(2) Tcase = 25 °C.
(3) Tcase = 85 °C.
Fig 20. Input return loss, output return loss and
isolation as a function of frequency
Fig 21. Output third-order intercept point as a function
of frequency
RLout
RLin
ISL
f (GHz)
2.11 2.13 2.15 2.17
014aab066
−20
−10
0
RLin, RLout, ISL
(dB)
−30
(3)
(2)
(1)
f (GHz)
2.11 2.13 2.15 2.17
014aab067
36
38
40
IP3O
(dBm)
34
BGA7124 All information provided in this document is subject to legal disclaimers. © NXP B.V. 2010. All rights reserved.
Product data sheet Rev. 3 — 9 September 2010 18 of 33
NXP Semiconductors BGA7124
400 MHz to 2700 MHz 0.25 W high linearity silicon amplifier
See Table 16 for a list of components.
Fig 22. 5 V/130 mA application reference board; 2110 MHz to 2170 MHz
J3
GND
VCC
GND
n.c.
enable
GND
C7
C6
C4
C2 C3
C1
R2
L1
C5
R1
MSL6
MSL4 MSL5
MSL1 MSL2 MSL3
J1
J I HG F E D C B A 1 2 3 4 5 6 7 8 910
11
12
13
RF in
J2
RF out
014aab068
Table 16. 5 V/130 mA application list of components; 2110 MHz to 2170 MHz
See Figure 17 and Figure 22 for component layout. Printed-Circuit Board (PCB): Rogers RO4003C stack; height = 0.508 mm;
copper plating thickness = 35 μm.
Component Description Value Function Remarks
C1, C4 capacitor 15 pF DC blocking Murata GRM1885C1H150JA01D
C2 capacitor 2.7 pF input match Murata GRM1885C1H2R7CZ01D
C3 capacitor 1.5 pF output match Murata GRM1885C1H1R5CZ01D
C5 capacitor 15 pF RF decoupling Murata GRM1885C1H150JA01D
C6 capacitor 100 nF DC decoupling AVX 0603YC104KAT2A
C7 capacitor 10 μF DC decoupling AVX 1206ZG106ZAT2A
J1, J2 RF connector SMA Emerson Network Power 142-0701-841
J3 DC connector 6-pins MOLEX
L1 inductor 22 nH DC feed Tyco electronics 36501J022JTDG
MSL1[1] micro stripline 1.14 mm × 0.8 mm × 10.95 mm input match
MSL2[1] micro stripline 1.14 mm × 0.8 mm × 10.8 mm input match
MSL3[1] micro stripline 1.14 mm × 0.8 mm × 5.8 mm output match
MSL4[1] micro stripline 1.14 mm × 0.8 mm × 2.5 mm output match
MSL5[1] micro stripline 1.14 mm × 0.8 mm × 3.5 mm output match
BGA7124 All information provided in this document is subject to legal disclaimers. © NXP B.V. 2010. All rights reserved.
Product data sheet Rev. 3 — 9 September 2010 19 of 33
NXP Semiconductors BGA7124
400 MHz to 2700 MHz 0.25 W high linearity silicon amplifier
[1] MSL1 to MSL6 dimensions specified as Width (W), Spacing (S) and Length (L).
12.1.4 2405 MHz to 2485 MHz
MSL6[1] micro stripline 1.14 mm × 0.8 mm × 10.95 mm output match
R1 resistor 0 Ω Multicomp MC 0.063W 0603 0R
R2 resistor
(trimmer)
2 kΩ bias
adjustment
Bourns 3214W-1-202E
Table 16. 5 V/130 mA application list of components; 2110 MHz to 2170 MHz …continued
See Figure 17 and Figure 22 for component layout. Printed-Circuit Board (PCB): Rogers RO4003C stack; height = 0.508 mm;
copper plating thickness = 35 μm.
Component Description Value Function Remarks
See Table 17 for a list of components.
PCB board specification: Rogers RO4003C; Height = 0.508 mm; εr = 3.38; Copper thickness = 35 μm.
Fig 23. 5 V/130 mA application schematic; 2405 MHz to 2485 MHz
C3 C4
C5
C7
C8 C6
R1
R2
ICQ_ADJ SHDN
enable
L1
C2
MSL1 C1 MSL2 MSL3 MSL4 MSL5 RF_IN
RF_OUT
BGA7124
50 Ω 50 Ω
VCC
014aab069
VCC(BIAS)
VCC(RF)
J1
J3
J2
BGA7124 All information provided in this document is subject to legal disclaimers. © NXP B.V. 2010. All rights reserved.
Product data sheet Rev. 3 — 9 September 2010 20 of 33
NXP Semiconductors BGA7124
400 MHz to 2700 MHz 0.25 W high linearity silicon amplifier
(1) Tcase = −40 °C.
(2) Tcase = 25 °C.
(3) Tcase = 85 °C.
(1) Tcase = −40 °C.
(2) Tcase = 25 °C.
(3) Tcase = 85 °C.
Fig 24. Output power at 1 dB gain compression as a
function of frequency
Fig 25. Power gain as a function of frequency
(3)
(2)
(1)
f (GHz)
2.405 2.425 2.445 2.465 2.485
014aab070
20
22
18
24
26
PL(1dB)
(dBm)
16
(3)
(2)
(1)
f (GHz)
2.405 2.425 2.445 2.465 2.485
014aab071
14
16
12
18
20
Gp
(dB)
10
Tcase = 25 °C. (1) Tcase = −40 °C.
(2) Tcase = 25 °C.
(3) Tcase = 85 °C.
Fig 26. Input return loss, output return loss and
isolation as a function of frequency
Fig 27. Output third-order intercept point as a function
of frequency
RLout
RLin
ISL
f (GHz)
2.405 2.425 2.445 2.465 2.485
014aab072
−20
−10
0
RLin, RLout, ISL
(dB)
−30
f (GHz)
2.405 2.425 2.445 2.465 2.485
014aab073
34
36
38
32
IP3O
(dBm)
(1)
(2)
(3)
BGA7124 All information provided in this document is subject to legal disclaimers. © NXP B.V. 2010. All rights reserved.
Product data sheet Rev. 3 — 9 September 2010 21 of 33
NXP Semiconductors BGA7124
400 MHz to 2700 MHz 0.25 W high linearity silicon amplifier
See Table 17 for a list of components.
Fig 28. 5 V/130 mA application reference board; 2405 MHz to 2485 MHz
J3
GND
VCC
GND
n.c.
enable
GND
C8
C7
C5
C2 C3 C4
C1
R2
L1
C6
R1
MSL1 MSL2 MSL3 MSL4 MSL5
J1
J I HG F E D C B A 1 2 3 4 5 6 7 8 910
11
12
13
RF in
J2
RF out
014aab074
Table 17. 5 V/130 mA application list of components; 2405 MHz to 2485 MHz
See Figure 23 and Figure 28 for component layout. Printed-Circuit Board (PCB): Rogers RO4003C stack; height = 0.508 mm;
copper plating thickness = 35 μm.
Component Description Value Function Remarks
C1, C5 capacitor 12 pF DC blocking Murata GRM1885C1H120JA01D
C2 capacitor 2.2 pF input match Murata GRM1885C1H2R2CZ01D
C3 capacitor 0.82 pF output match Murata GRM1885C1HR82CZ01D
C4 capacitor 0.68 pF output match Murata GRM1885C1HR68CZ01D
C6 capacitor 12 pF RF decoupling Murata GRM1885C1H120JA01D
C7 capacitor 100 nF DC decoupling AVX 0603YC104KAT2A
C8 capacitor 10 μF DC decoupling AVX 1206ZG106ZAT2A
J1, J2 RF connector SMA Emerson Network Power
142-0701-841
J3 DC connector 6-pins MOLEX
L1 inductor 22 nH DC feed Tyco electronics 36501J022JTDG
MSL1[1] micro stripline 1.14 mm × 0.8 mm × 10.95 mm input match
MSL2[1] micro stripline 1.14 mm × 0.8 mm × 10.8 mm input match
MSL3[1] micro stripline 1.14 mm × 0.8 mm × 7.3 mm output match
MSL4[1] micro stripline 1.14 mm × 0.8 mm × 4.3 mm output match
BGA7124 All information provided in this document is subject to legal disclaimers. © NXP B.V. 2010. All rights reserved.
Product data sheet Rev. 3 — 9 September 2010 22 of 33
NXP Semiconductors BGA7124
400 MHz to 2700 MHz 0.25 W high linearity silicon amplifier
[1] MSL1 to MSL5 dimensions specified as Width (W), Spacing (S) and Length (L).
12.2 3.3 V applications
12.2.1 920 MHz to 960 MHz
MSL5[1] micro stripline 1.14 mm × 0.8 mm × 10.95 mm output match
R1 resistor 2.2 Ω Multicomp MC 0.063W 0603 2R2
R2 resistor (trimmer) 2 kΩ bias adjustment Bourns 3214W-1-202E
Table 17. 5 V/130 mA application list of components; 2405 MHz to 2485 MHz …continued
See Figure 23 and Figure 28 for component layout. Printed-Circuit Board (PCB): Rogers RO4003C stack; height = 0.508 mm;
copper plating thickness = 35 μm.
Component Description Value Function Remarks
See Table 18 for a list of components.
PCB board specification: Rogers RO4003C; Height = 0.508 mm; εr = 3.38; Copper thickness = 35 μm.
Fig 29. 3.3 V/130 mA application schematic; 920 MHz to 960 MHz
C3 C4
C6
C8
C9 C7
R1
R2
ICQ_ADJ SHDN
enable
L1
L2
C2
MSL1 C1 MSL2 MSL3 RF_IN MSL4 MSL5 MSL6 MSL7 MSL8
RF_OUT
BGA7124
50 Ω 50 Ω
VCC
C5
014aab075
VCC(BIAS)
VCC(RF)
J1
J3
J2
BGA7124 All information provided in this document is subject to legal disclaimers. © NXP B.V. 2010. All rights reserved.
Product data sheet Rev. 3 — 9 September 2010 23 of 33
NXP Semiconductors BGA7124
400 MHz to 2700 MHz 0.25 W high linearity silicon amplifier
(1) Tcase = −40 °C.
(2) Tcase = 25 °C.
(3) Tcase = 85 °C.
(1) Tcase = −40 °C.
(2) Tcase = 25 °C.
(3) Tcase = 85 °C.
Fig 30. Output power at 1 dB gain compression as a
function of frequency
Fig 31. Power gain as a function of frequency
f (GHz)
0.92 0.93 0.94 0.95 0.96
014aab076
24
26
22
28
30
PL(1dB)
(dBm)
20
(1)
(2)
(3)
f (GHz)
0.92 0.93 0.94 0.95 0.96
014aab077
22
24
20
26
28
Gp
(dB)
18
(1)
(2)
(3)
Tcase = 25 °C. (1) Tcase = −40 °C.
(2) Tcase = 25 °C.
(3) Tcase = 85 °C.
Fig 32. Input return loss, output return loss and
isolation as a function of frequency
Fig 33. Output third-order intercept point as a function
of frequency
RLout
RLin
ISL
f (GHz)
0.92 0.93 0.94 0.95 0.96
014aab078
−20
−10
0
RLin, RLout, ISL
(dB)
−30
f (GHz)
0.92 0.93 0.94 0.95 0.96
014aab079
36
38
40
IP3O
(dBm)
34
(1)
(3)
(2)
BGA7124 All information provided in this document is subject to legal disclaimers. © NXP B.V. 2010. All rights reserved.
Product data sheet Rev. 3 — 9 September 2010 24 of 33
NXP Semiconductors BGA7124
400 MHz to 2700 MHz 0.25 W high linearity silicon amplifier
See Table 18 for a list of components.
Fig 34. 3.3 V/130 mA application reference board; 920 MHz to 960 MHz
J3
GND
VCC
GND
n.c.
enable
GND
C9
C8
C6
C2 C3 C4 C5
C1
R2
L2
L1
C7
R1
MSL1 MSL3 MSL8
MSL2
MSL4 MSL6 MSL7
MSL5
J1
J I HG F E D C B A 1 2 3 4 5 6 7 8 910
11
12
13
RF in
J2
RF out
014aab080
Table 18. 3.3 V/130 mA application list of components; 920 MHz to 960 MHz
See Figure 29 and Figure 34 for component layout. Printed-Circuit Board (PCB): Rogers RO4003C stack; height = 0.508 mm;
copper plating thickness = 35 μm.
Component Description Value Function Remarks
C1, C6 capacitor 68 pF DC blocking Murata GRM1885C1H680JA01D
C2, C3 capacitor 3.3 pF input match Murata GRM1885C1H3R3CZ01D
C4 capacitor 3.9 pF output match Murata GRM1885C1H3R9CZ01D
C5 capacitor 1.0 pF output match Murata GRM1885C1H1R0CZ01D
C7 capacitor 68 pF RF decoupling Murata GRM1885C1H680JA01D
C8 capacitor 100 nF DC decoupling AVX 0603YC104KAT2A
C9 capacitor 10 μF DC decoupling AVX 1206ZG106ZAT2A
J1, J2 RF connector SMA Emerson Network Power
142-0701-841
J3 DC connector 6-pins MOLEX
L1 inductor 2.2 nH output match Tyco electronics 36501J2N2JTDG
L2 inductor 22 nH DC feed Tyco electronics 36501J022JTDG
MSL1[1] micro stripline 1.14 mm × 0.8 mm × 10.95 mm input match
MSL2[1] micro stripline 1.14 mm × 0.8 mm × 2.95 mm input match
MSL3[1] micro stripline 1.14 mm × 0.8 mm × 7.75 mm input match
MSL4[1] micro stripline 1.14 mm × 0.8 mm × 23.4 mm output match
MSL5[1] micro stripline 1.14 mm × 0.8 mm × 2.2 mm output match
BGA7124 All information provided in this document is subject to legal disclaimers. © NXP B.V. 2010. All rights reserved.
Product data sheet Rev. 3 — 9 September 2010 25 of 33
NXP Semiconductors BGA7124
400 MHz to 2700 MHz 0.25 W high linearity silicon amplifier
[1] MSL1 to MSL8 dimensions specified as Width (W), Spacing (S) and Length (L).
12.2.2 2405 MHz to 2485 MHz
MSL6[1] micro stripline 1.14 mm × 0.8 mm × 2.4 mm output match
MSL7[1] micro stripline 1.14 mm × 0.8 mm × 2.3 mm output match
MSL8[1] micro stripline 1.14 mm × 0.8 mm × 10.95 mm output match
R1 resistor 0 Ω Multicomp MC 0.063W 0603 0R
R2 resistor (trimmer) 2 kΩ bias adjustment Bourns 3214W-1-202E
Table 18. 3.3 V/130 mA application list of components; 920 MHz to 960 MHz …continued
See Figure 29 and Figure 34 for component layout. Printed-Circuit Board (PCB): Rogers RO4003C stack; height = 0.508 mm;
copper plating thickness = 35 μm.
Component Description Value Function Remarks
See Table 19 for a list of components.
PCB board specification: Rogers RO4003C; Height = 0.508 mm; εr = 3.38; Copper thickness = 35 μm
Fig 35. 3.3 V/130 mA application schematic; 2405 MHz to 2485 MHz
RF_OUT
C3
C5
C7
C8 C6
R1
R2
ICQ_ADJ SHDN
enable
L1
C2
MSL1 C1 MSL2 MSL3 MSL4 MSL5
RF_IN
BGA7124
50 Ω 50 Ω
VCC
C4
014aab081
VCC(BIAS)
VCC(RF)
J1
J3
J2
BGA7124 All information provided in this document is subject to legal disclaimers. © NXP B.V. 2010. All rights reserved.
Product data sheet Rev. 3 — 9 September 2010 26 of 33
NXP Semiconductors BGA7124
400 MHz to 2700 MHz 0.25 W high linearity silicon amplifier
(1) Tcase = −40 °C.
(2) Tcase = 25 °C.
(3) Tcase = 85 °C.
(1) Tcase = −40 °C.
(2) Tcase = 25 °C.
(3) Tcase = 85 °C.
Fig 36. Output power at 1 dB gain compression as a
function of frequency
Fig 37. Power gain as a function of frequency
f (GHz)
2.405 2.425 2.445 2.465 2.485
014aab082
20
22
18
24
26
PL(1dB)
(dBm)
16
(3)
(1)
(2)
f (GHz)
2.405 2.425 2.445 2.465 2.485
014aab083
14
16
12
18
20
Gp
(dB)
10
(1)
(2)
(3)
Tcase = 25 °C. (1) Tcase = −40 °C.
(2) Tcase = 25 °C.
(3) Tcase = 85 °C.
Fig 38. Input return loss, output return loss and
isolation as a function of frequency
Fig 39. Output third-order intercept point as a function
of frequency
RLout
RLin
ISL
f (GHz)
2.405 2.425 2.445 2.465 2.485
014aab084
−20
−10
0
RLin, RLout, ISL
(dB)
−30
(2)
(1)
(3)
f (GHz)
2.405 2.425 2.445 2.465 2.485
014aab085
34
36
38
IP3O
(dBm)
32
BGA7124 All information provided in this document is subject to legal disclaimers. © NXP B.V. 2010. All rights reserved.
Product data sheet Rev. 3 — 9 September 2010 27 of 33
NXP Semiconductors BGA7124
400 MHz to 2700 MHz 0.25 W high linearity silicon amplifier
See Table 19 for a list of components.
Fig 40. 3.3 V/130 mA application reference board; 2405 MHz to 2485 MHz
J3
GND
VCC
GND
n.c.
enable
GND
C8
C7
C5
C2 C3 C4
C1
R2
L1
C6
R1
MSL1 MSL2 MSL3 MSL4 MSL5
J1
J I HG F E D C B A 1 2 3 4 5 6 7 8 910
11
12
13
RF in
J2
RF out
014aab086
Table 19. 3.3 V/130 mA application list of components; 2405 MHz to 2485 MHz
See Figure 35 and Figure 40 for component layout. Printed-Circuit Board (PCB): Rogers RO4003C stack; height = 0.508 mm;
copper plating thickness = 35 μm.
Component Description Value Function Remarks
C1, C5 capacitor 12 pF DC blocking Murata GRM1885C1H120JA01D
C2 capacitor 2.2 pF input match Murata GRM1885C1H2R2CZ01D
C3 capacitor 0.82 pF output match Murata GRM1885C1HR82CZ01D
C4 capacitor 0.68 pF output match Murata GRM1885C1HR68CZ01D
C6 capacitor 12 pF RF decoupling Murata GRM1885C1H120JA01D
C7 capacitor 100 nF DC decoupling AVX 0603YC104KAT2A
C8 capacitor 10 μF DC decoupling AVX 1206ZG106ZAT2A
J1, J2 RF connector SMA Emerson Network Power
142-0701-841
J3 DC connector 6-pins MOLEX
L1 inductor 22 nH DC feed Tyco electronics 36501J022JTDG
MSL1[1] micro stripline 1.14 mm × 0.8 mm × 10.95 mm input match
MSL2[1] micro stripline 1.14 mm × 0.8 mm × 10.8 mm input match
MSL3[1] micro stripline 1.14 mm × 0.8 mm × 7.3 mm output match
MSL4[1] micro stripline 1.14 mm × 0.8 mm × 4.3 mm output match
BGA7124 All information provided in this document is subject to legal disclaimers. © NXP B.V. 2010. All rights reserved.
Product data sheet Rev. 3 — 9 September 2010 28 of 33
NXP Semiconductors BGA7124
400 MHz to 2700 MHz 0.25 W high linearity silicon amplifier
[1] MSL1 to MSL5 dimensions specified as Width (W), Spacing (S) and Length (L).
12.3 PCB stack
MSL5[1] micro stripline 1.14 mm × 0.8 mm × 10.95 mm output match
R1 resistor 2.2 Ω Multicomp MC 0.063W 0603 2R2
R2 resistor (trimmer) 2 kΩ bias adjustment Bourns 3214W-1-202E
Table 19. 3.3 V/130 mA application list of components; 2405 MHz to 2485 MHz …continued
See Figure 35 and Figure 40 for component layout. Printed-Circuit Board (PCB): Rogers RO4003C stack; height = 0.508 mm;
copper plating thickness = 35 μm.
Component Description Value Function Remarks
(1) Pre-pregnated
RO4003Cdielectric constant εr = 3.38
Fig 41. PCB stack
through via
RF and analog ground
RF and analog routing
analog routing
RF and analog ground
35 μm (1 oz.) copper + 0.3 μm
gold plating
RO4003C, 0.51 mm (20 mil)
35 μm (1 oz.) copper
(1) 0.2 mm (8 mil)
FR4, 0.15 mm (6 mil)
35 μm (1 oz.) copper
35 μm (1 oz.) copper
014aab087
BGA7124 All information provided in this document is subject to legal disclaimers. © NXP B.V. 2010. All rights reserved.
Product data sheet Rev. 3 — 9 September 2010 29 of 33
NXP Semiconductors BGA7124
400 MHz to 2700 MHz 0.25 W high linearity silicon amplifier
13. Package outline
Fig 42. Package outline SOT908-1 (HVSON8)
1 0.2 0.5
0.05
0.00
UNIT A1 b D(1) Eh e y
1.5
e1
OUTLINE REFERENCES
VERSION
EUROPEAN
PROJECTION ISSUE DATE
IEC JEDEC JEITA
mm 3.1
2.9
c Dh
1.65
1.35
y1
3.1
2.9
2.25
1.95
0.3
0.2
0.05 0.1
DIMENSIONS (mm are the original dimensions)
SOT908-1 MO-229
E(1)
0.5
0.3
L
0.1
v
0.05
w
SOT908-1
HVSON8: plastic thermal enhanced very thin small outline package; no leads;
8 terminals; body 3 x 3 x 0.85 mm
A(1)
max.
05-09-26
05-10-05
Note
1. Plastic or metal protrusions of 0.075 mm maximum per side are not included.
X
terminal 1
index area
D B A
E
detail X
A
A1
c
C
y1 C y
exposed tie bar (4×)
exposed tie bar (4×)
b
terminal 1
index area
e1
e
v M C A B
w M C
Eh
Dh
L
1 4
8 5
0 1 2 mm
scale
BGA7124 All information provided in this document is subject to legal disclaimers. © NXP B.V. 2010. All rights reserved.
Product data sheet Rev. 3 — 9 September 2010 30 of 33
NXP Semiconductors BGA7124
400 MHz to 2700 MHz 0.25 W high linearity silicon amplifier
14. Abbreviations
15. Revision history
Table 20. Abbreviations
Acronym Description
CPE Customer-Premises Equipment
DC Direct Current
ESD ElectroStatic Discharge
HTOL High Temperature Operating Life
ISM Industrial, Scientific and Medical
MMIC Monolithic Microwave Integrated Circuit
MoCA Multimedia over Coax Alliance
RFID Radio Frequency IDentification
SMA SubMiniature version A
TX Transmit
WLAN Wireless Local Area Network
Table 21. Revision history
Document ID Release date Data sheet status Change notice Supersedes
BGA7124 v.3 20100909 Product data sheet - BGA7124 v.2
Modifications: • Figure 5 on page 11: MSL symbols have been corrected.
• Figure 11 on page 14: MSL symbols have been corrected.
• Figure 17 on page 16: MSL symbols have been corrected.
• Figure 23 on page 19: MSL symbols have been corrected.
• Figure 29 on page 22: MSL symbols have been corrected.
• Figure 35 on page 25: MSL symbols have been corrected.
BGA7124 v.2 20100623 Product data sheet - BGA7124 v.1
BGA7124 v.1 20100421 Product data sheet - -
BGA7124 All information provided in this document is subject to legal disclaimers. © NXP B.V. 2010. All rights reserved.
Product data sheet Rev. 3 — 9 September 2010 31 of 33
NXP Semiconductors BGA7124
400 MHz to 2700 MHz 0.25 W high linearity silicon amplifier
16. Legal information
16.1 Data sheet status
[1] Please consult the most recently issued document before initiating or completing a design.
[2] The term ‘short data sheet’ is explained in section “Definitions”.
[3] The product status of device(s) described in this document may have changed since this document was published and may differ in case of multiple devices. The latest product status
information is available on the Internet at URL http://www.nxp.com.
16.2 Definitions
Draft — The document is a draft version only. The content is still under
internal review and subject to formal approval, which may result in
modifications or additions. NXP Semiconductors does not give any
representations or warranties as to the accuracy or completeness of
information included herein and shall have no liability for the consequences of
use of such information.
Short data sheet — A short data sheet is an extract from a full data sheet
with the same product type number(s) and title. A short data sheet is intended
for quick reference only and should not be relied upon to contain detailed and
full information. For detailed and full information see the relevant full data
sheet, which is available on request via the local NXP Semiconductors sales
office. In case of any inconsistency or conflict with the short data sheet, the
full data sheet shall prevail.
Product specification — The information and data provided in a Product
data sheet shall define the specification of the product as agreed between
NXP Semiconductors and its customer, unless NXP Semiconductors and
customer have explicitly agreed otherwise in writing. In no event however,
shall an agreement be valid in which the NXP Semiconductors product is
deemed to offer functions and qualities beyond those described in the
Product data sheet.
16.3 Disclaimers
Limited warranty and liability — Information in this document is believed to
be accurate and reliable. However, NXP Semiconductors does not give any
representations or warranties, expressed or implied, as to the accuracy or
completeness of such information and shall have no liability for the
consequences of use of such information.
In no event shall NXP Semiconductors be liable for any indirect, incidental,
punitive, special or consequential damages (including - without limitation - lost
profits, lost savings, business interruption, costs related to the removal or
replacement of any products or rework charges) whether or not such
damages are based on tort (including negligence), warranty, breach of
contract or any other legal theory.
Notwithstanding any damages that customer might incur for any reason
whatsoever, NXP Semiconductors’ aggregate and cumulative liability towards
customer for the products described herein shall be limited in accordance
with the Terms and conditions of commercial sale of NXP Semiconductors.
Right to make changes — NXP Semiconductors reserves the right to make
changes to information published in this document, including without
limitation specifications and product descriptions, at any time and without
notice. This document supersedes and replaces all information supplied prior
to the publication hereof.
Suitability for use — NXP Semiconductors products are not designed,
authorized or warranted to be suitable for use in life support, life-critical or
safety-critical systems or equipment, nor in applications where failure or
malfunction of an NXP Semiconductors product can reasonably be expected
to result in personal injury, death or severe property or environmental
damage. NXP Semiconductors accepts no liability for inclusion and/or use of
NXP Semiconductors products in such equipment or applications and
therefore such inclusion and/or use is at the customer’s own risk.
Applications — Applications that are described herein for any of these
products are for illustrative purposes only. NXP Semiconductors makes no
representation or warranty that such applications will be suitable for the
specified use without further testing or modification.
Customers are responsible for the design and operation of their applications
and products using NXP Semiconductors products, and NXP Semiconductors
accepts no liability for any assistance with applications or customer product
design. It is customer’s sole responsibility to determine whether the NXP
Semiconductors product is suitable and fit for the customer’s applications and
products planned, as well as for the planned application and use of
customer’s third party customer(s). Customers should provide appropriate
design and operating safeguards to minimize the risks associated with their
applications and products.
NXP Semiconductors does not accept any liability related to any default,
damage, costs or problem which is based on any weakness or default in the
customer’s applications or products, or the application or use by customer’s
third party customer(s). Customer is responsible for doing all necessary
testing for the customer’s applications and products using NXP
Semiconductors products in order to avoid a default of the applications and
the products or of the application or use by customer’s third party
customer(s). NXP does not accept any liability in this respect.
Limiting values — Stress above one or more limiting values (as defined in
the Absolute Maximum Ratings System of IEC 60134) will cause permanent
damage to the device. Limiting values are stress ratings only and (proper)
operation of the device at these or any other conditions above those given in
the Recommended operating conditions section (if present) or the
Characteristics sections of this document is not warranted. Constant or
repeated exposure to limiting values will permanently and irreversibly affect
the quality and reliability of the device.
Terms and conditions of commercial sale — NXP Semiconductors
products are sold subject to the general terms and conditions of commercial
sale, as published at http://www.nxp.com/profile/terms, unless otherwise
agreed in a valid written individual agreement. In case an individual
agreement is concluded only the terms and conditions of the respective
agreement shall apply. NXP Semiconductors hereby expressly objects to
applying the customer’s general terms and conditions with regard to the
purchase of NXP Semiconductors products by customer.
No offer to sell or license — Nothing in this document may be interpreted or
construed as an offer to sell products that is open for acceptance or the grant,
conveyance or implication of any license under any copyrights, patents or
other industrial or intellectual property rights.
Export control — This document as well as the item(s) described herein
may be subject to export control regulations. Export might require a prior
authorization from national authorities.
Document status[1][2] Product status[3] Definition
Objective [short] data sheet Development This document contains data from the objective specification for product development.
Preliminary [short] data sheet Qualification This document contains data from the preliminary specification.
Product [short] data sheet Production This document contains the product specification.
BGA7124 All information provided in this document is subject to legal disclaimers. © NXP B.V. 2010. All rights reserved.
Product data sheet Rev. 3 — 9 September 2010 32 of 33
NXP Semiconductors BGA7124
400 MHz to 2700 MHz 0.25 W high linearity silicon amplifier
Non-automotive qualified products — Unless this data sheet expressly
states that this specific NXP Semiconductors product is automotive qualified,
the product is not suitable for automotive use. It is neither qualified nor tested
in accordance with automotive testing or application requirements. NXP
Semiconductors accepts no liability for inclusion and/or use of
non-automotive qualified products in automotive equipment or applications.
In the event that customer uses the product for design-in and use in
automotive applications to automotive specifications and standards, customer
(a) shall use the product without NXP Semiconductors’ warranty of the
product for such automotive applications, use and specifications, and (b)
whenever customer uses the product for automotive applications beyond
NXP Semiconductors’ specifications such use shall be solely at customer’s
own risk, and (c) customer fully indemnifies NXP Semiconductors for any
liability, damages or failed product claims resulting from customer design and
use of the product for automotive applications beyond NXP Semiconductors’
standard warranty and NXP Semiconductors’ product specifications.
Export control — This document as well as the item(s) described herein
may be subject to export control regulations. Export might require a prior
authorization from national authorities.
16.4 Trademarks
Notice: All referenced brands, product names, service names and trademarks
are the property of their respective owners.
17. Contact information
For more information, please visit: http://www.nxp.com
For sales office addresses, please send an email to: salesaddresses@nxp.com
NXP Semiconductors BGA7124
400 MHz to 2700 MHz 0.25 W high linearity silicon amplifier
© NXP B.V. 2010. All rights reserved.
For more information, please visit: http://www.nxp.com
For sales office addresses, please send an email to: salesaddresses@nxp.com
Date of release: 9 September 2010
Document identifier: BGA7124
Please be aware that important notices concerning this document and the product(s)
described herein, have been included in section ‘Legal information’.
18. Contents
1 Product profile . . . . . . . . . . . . . . . . . . . . . . . . . . 1
1.1 General description . . . . . . . . . . . . . . . . . . . . . 1
1.2 Features and benefits. . . . . . . . . . . . . . . . . . . . 1
1.3 Applications . . . . . . . . . . . . . . . . . . . . . . . . . . . 1
1.4 Quick reference data . . . . . . . . . . . . . . . . . . . . 1
2 Pinning information. . . . . . . . . . . . . . . . . . . . . . 2
2.1 Pinning . . . . . . . . . . . . . . . . . . . . . . . . . . . . . . . 2
2.2 Pin description . . . . . . . . . . . . . . . . . . . . . . . . . 2
3 Ordering information. . . . . . . . . . . . . . . . . . . . . 2
4 Functional diagram . . . . . . . . . . . . . . . . . . . . . . 3
5 Shutdown control . . . . . . . . . . . . . . . . . . . . . . . 3
6 Limiting values. . . . . . . . . . . . . . . . . . . . . . . . . . 4
7 Thermal characteristics . . . . . . . . . . . . . . . . . . 5
8 Static characteristics. . . . . . . . . . . . . . . . . . . . . 5
8.1 Supply current adjustment . . . . . . . . . . . . . . . . 5
9 Dynamic characteristics . . . . . . . . . . . . . . . . . . 6
9.1 Scattering parameters . . . . . . . . . . . . . . . . . . . 9
10 Reliability information . . . . . . . . . . . . . . . . . . . 10
11 Moisture sensitivity . . . . . . . . . . . . . . . . . . . . . 10
12 Application information. . . . . . . . . . . . . . . . . . 11
12.1 5 V applications . . . . . . . . . . . . . . . . . . . . . . . 11
12.1.1 920 MHz to 960 MHz . . . . . . . . . . . . . . . . . . . 11
12.1.2 1930 MHz to 1990 MHz . . . . . . . . . . . . . . . . . 14
12.1.3 2110 MHz to 2170 MHz . . . . . . . . . . . . . . . . . 16
12.1.4 2405 MHz to 2485 MHz . . . . . . . . . . . . . . . . . 19
12.2 3.3 V applications . . . . . . . . . . . . . . . . . . . . . . 22
12.2.1 920 MHz to 960 MHz . . . . . . . . . . . . . . . . . . . 22
12.2.2 2405 MHz to 2485 MHz . . . . . . . . . . . . . . . . . 25
12.3 PCB stack. . . . . . . . . . . . . . . . . . . . . . . . . . . . 28
13 Package outline . . . . . . . . . . . . . . . . . . . . . . . . 29
14 Abbreviations. . . . . . . . . . . . . . . . . . . . . . . . . . 30
15 Revision history. . . . . . . . . . . . . . . . . . . . . . . . 30
16 Legal information. . . . . . . . . . . . . . . . . . . . . . . 31
16.1 Data sheet status . . . . . . . . . . . . . . . . . . . . . . 31
16.2 Definitions. . . . . . . . . . . . . . . . . . . . . . . . . . . . 31
16.3 Disclaimers . . . . . . . . . . . . . . . . . . . . . . . . . . . 31
16.4 Trademarks. . . . . . . . . . . . . . . . . . . . . . . . . . . 32
17 Contact information. . . . . . . . . . . . . . . . . . . . . 32
18 Contents . . . . . . . . . . . . . . . . . . . . . . . . . . . . . . 33
REV. C
Information furnished by Analog Devices is believed to be accurate and
reliable. However, no responsibility is assumed by Analog Devices for its
use, nor for any infringements of patents or other rights of third parties
which may result from its use. No license is granted by implication or
otherwise under any patent or patent rights of Analog Devices.
a 12-Bit Serial Daisy-Chain
CMOS D/A Converter
DAC8143
FUNCTIONAL BLOCK DIAGRAM
INPUT 12-BIT
SHIFT REGISTER
DAC REGISTER
12-BIT
D/A CONVERTER
DAC8143
LOAD
IN OUT
CLK
VDD
RFB
IOUT1
IOUT2
AGND
SRO
DGND
SRI
STB2
STB3
STB4
STB1
LD2
LD1
VREF
CLR
ADDRESS BUS
ADDRESS
DECODER
STROBE
LOAD
SRI
SRO
DAC8143
STROBE
LOAD
SRI
SRO
DAC8143
STROBE
LOAD
SRI
SRO
DAC8143
STROBE
LOAD
SRI
SRO
DAC8143
WR
DBX
mP
Figure 1. Multiple DAC8143s with Three-Wire Interface
FEATURES
Fast, Flexible, Microprocessor Interfacing in Serially
Controlled Systems
Buffered Digital Output Pin for Daisy-Chaining
Multiple DACs
Minimizes Address-Decoding in Multiple DAC
Systems—Three-Wire Interface for Any Number of DACs
One Data Line
One CLK Line
One Load Line
Improved Resistance to ESD
–408C to +858C for the Extended Industrial Temperature
Range
APPLICATIONS
Multiple-Channel Data Acquisition Systems
Process Control and Industrial Automation
Test Equipment
Remote Microprocessor-Controlled Systems
GENERAL INFORMATION
The DAC8143 is a 12-bit serial-input daisy-chain CMOS D/A
converter that features serial data input and buffered serial data
output. It was designed for multiple serial DAC systems, where
serially daisy-chaining one DAC after another is greatly simplified.
The DAC8143 also minimizes address decoding lines enabling
simpler logic interfacing. It allows three-wire interface for any
number of DACs: one data line, one CLK line and one load line.
Serial data in the input register (MSB first) is sequentially
clocked out to the SRO pin as the new data word (MSB first) is
simultaneously clocked in from the SRI pin. The strobe inputs
are used to clock in/out data on the rising or falling (user
selected) strobe edges (STB1, STB2, STB3, STB4).
When the shift register’s data has been updated, the new data
word is transferred to the DAC register with use of LD1 and
LD2 inputs.
Separate LOAD control inputs allow simultaneous output updating
of multiple DACs. An asynchronous CLEAR input
resets the DAC register without altering data in the input
register.
Improved linearity and gain error performance permits reduced
circuit parts count through the elimination of trimming components.
Fast interface timing reduces timing design considerations
while minimizing microprocessor wait states.
The DAC8143 is available in plastic packages that are compatible
with autoinsertion equipment.
Plastic packaged devices come in the extended industrial temperature
range of –40°C to +85°C.
One Technology Way, P.O. Box 9106, Norwood, MA 02062-9106, U.S.A.
Tel: 781/329-4700 World Wide Web Site: http://www.analog.com
Fax: 781/326-8703 © Analog Devices, Inc., 1999
ELECTRICAL CHARACTERISTICS
Parameter Symbol Conditions Min Typ Max Units
STATIC ACCURACY
Resolution N 12 Bits
Nonlinearity INL ±1 LSB
Differential Nonlinearity1 DNL ±1 LSB
Gain Error2 GFSE ±2 LSB
Gain Tempco (DGain/DTemp)3 TCGFS ±5 ppm/°C
Power Supply Rejection Ratio
(DGain/DVDD) PSRR DVDD = ±5% ±0.0006 ±0.002 %/%
Output Leakage Current4 ILKG TA = +25°C ±5 nA
TA = Full Temperature Range ±25 nA
Zero Scale Error5, 6 IZSE TA = +25°C ±0.002 ±0.03 LSB
TA = Full Temperature Range ±0.01 ±0.15 LSB
Input Resistance7 RIN VREF Pin 7 11 15 kW
AC PERFORMANCE
Output Current Settling Time3, 8 tS 0.380 1 ms
AC Feedthrough Error
(VREF to IOUT1)3, 9 FT VREF = 20 V p-p @ f = 10 kHz, TA = +25°C 2.0 mV p-p
Digital-to-Analog Glitch Energy3, 10 Q VREF = 0 V, IOUT Load = 100 W, CEXT = 13 pF 20 nVs
Total Harmonic Distortion3 THD VREF = 6 V rms @ 1 kHz
DAC Register Loaded with All 1s –92 dB
Output Noise Voltage Density3, 11 en 10 Hz to 100 kHz Between RFB and IOUT 13 nV/ÖHz
DIGITAL INPUTS/OUTPUT
Digital Input HIGH VIH 2.4 V
Digital Input LOW VIL 0.8 V
Input Leakage Current12 IIN VIN = 0 V to +5 V ±1 mA
Input Capacitance CIN VIN = 0 V 8 pF
Digital Output High VOH IOH = –200 mA 4 V
Digital Output Low VOL IOL = 1.6 mA 0.4 V
ANALOG OUTPUTS
Output Capacitance3 COUT1 Digital Inputs = All 1s 90 pF
COUT2 Digital Inputs = All 0s 90 pF
Output Capacitance3 COUT1 Digital Inputs = All 0s 60 pF
COUT2 Digital Inputs = All 1s 60 pF
TIMING CHARACTERISTICS3
Serial Input to Strobe Setup Times tDS1 STB1 Used as the Strobe 50 ns
(tSTB = 80 ns) tDS2 STB2 Used as the Strobe 20 ns
tDS3 STB3 Used as the Strobe TA = +25°C 10 ns
TA = Full Temperature Range 20 ns
tDS4 STB4 Used as the Strobe 20 ns
tDH1 STB1 Used as the Strobe TA = +25°C 40 ns
TA = Full Temperature Range 50 ns
tDH2 STB2 Used as the Strobe TA = +25°C 50 ns
TA = Full Temperature Range 60 ns
Serial Input to Strobe Hold Times
(tSTB = 80 ns) tDH3 STB3 Used as the Strobe 80 ns
tDH4 STB4 Used as the Strobe 80 ns
–2– REV. C
(@ VDD = +5 V; VREF = +10 V; VOUT1 = VOUT2 = VAGND = VDGND = 0 V; TA = Full Temperature
Range specified under Absolute Maximum Ratings, unless otherwise noted.)
DAC8143–SPECIFICATIONS
ELECTRICAL CHARACTERISTICS
DAC8143
Parameter Symbol Conditions Min Typ Max Units
STB to SRO Propagation Delay13 tPD TA = +25°C 220 ns
TA = Full Temperature Range 300 ns
SRI Data Pulsewidth tSRI 100 ns
STB1 Pulsewidth (STB1 = 80 ns)14 tSTB1 80 ns
STB2 Pulsewidth (STB2 = 100 ns)14 tSTB2 80 ns
STB3 Pulsewidth (STB3 = 80 ns)14 tSTB3 80 ns
STB4 Pulsewidth (STB4 = 80 ns)14 tSTB4 80 ns
Load Pulsewidth tLD1, tLD2 TA = +25°C 140 ns
TA = Full Temperature Range 180 ns
LSB Strobe into Input Register
to Load DAC Register Time tASB 0 ns
CLR Pulsewidth tCLR 80 ns
POWER SUPPLY
Supply Voltage VDD 4.75 5 5.25 V
Supply Current IDD All Digital Inputs = VIH or VIL 2 mA
All Digital Inputs = 0 V or VDD 0.1 mA
Power Dissipation PD Digital Inputs = 0 V or VDD 0.5 mW
5 V ´ 0.1 mA
Digital Inputs = VIH or VIL 10 mW
5 V ´ 2 mA
NOTES
11All grades are monotonic to 12 bits over temperature.
12Using internal feedback resistor.
13Guaranteed by design and not tested.
14Applies to IOUT1; all digital inputs = VIL, VREF = +10 V; specification also applies for IOUT2 when all digital inputs = VIH.
15VREF = +10 V, all digital inputs = 0 V.
16Calculated from worst case RREF: IZSE (in LSBs) = (RREF ´ ILKG ´ 4096) /VREF.
17Absolute temperature coefficient is less than +300 ppm/°C.
18IOUT, Load = 100 W. CEXT = 13 pF, digital input = 0 V to VDD or VDD to 0 V. Extrapolated to 1/2 LSB: tS = propagation delay (tPD) +9 t, where t equals measured
time constant of the final RC decay.
19All digital inputs = 0 V.
10VREF = 0 V, all digital inputs = 0 V to VDD or VDD to 0 V.
11Calculations from en = Ö4K TRB where:
K = Boltzmann constant, J/KR = resistance W
T = resistor temperature, K B = bandwidth, Hz
12Digital inputs are CMOS gates; IIN typically 1 nA at +25°C.
13Measured from active strobe edge (STB) to new data output at SRO; CL = 50 pF.
14Minimum low time pulsewidth for STB1, STB2, and STB4, and minimum high time pulsewidth for STB3.
Specifications subject to change without notice.
(@ VDD = +5 V; VREF = +10 V; VOUT1 = V0UT2 = VAGND = VDGND = 0 V; TA = Full
Temperature Range specified under Absolute Maximum Ratings, unless otherwise noted.)
DAC8143
REV. C –3–
DAC8143
–4– REV. C
PIN CONNECTIONS
16-Lead Epoxy Plastic DIP
16-Lead SOIC
TOP VIEW
(Not to Scale)
16
15
14
13
12
11
10
9
1
2
3
4
5
6
7
8
IOUT1 RFB
DAC8143
IOUT2 VREF
AGND VDD
STB1 CLR
LD1 DGND
SRO STB4
SRI STB3
STB2 LD2
ABSOLUTE MAXIMUM RATINGS
(TA = +25°C, unless otherwise noted.)
VDD to DGND . . . . . . . . . . . . . . . . . . . . . . . . . . . . . . . . +17 V
VREF to DGND . . . . . . . . . . . . . . . . . . . . . . . . . . . . . . . ±25 V
VRFB to DGND . . . . . . . . . . . . . . . . . . . . . . . . . . . . . . . ±25 V
AGND to DGND . . . . . . . . . . . . . . . . . . . . . . . . VDD + 0.3 V
DGND to AGND . . . . . . . . . . . . . . . . . . . . . . . . VDD + 0.3 V
Digital Input Voltage Range . . . . . . . . . . . . . . . –0.3 V to VDD
Output Voltage (Pin 1, Pin 2) . . . . . . . . . . . . . . –0.3 V to VDD
Operating Temperature Range
FP/FS Versions . . . . . . . . . . . . . . . . . . . . . –40°C to +85°C
Junction Temperature . . . . . . . . . . . . . . . . . . . . . . . . .+150°C
Storage Temperature . . . . . . . . . . . . . . . . . . –65°C to +150°C
Lead Temperature (Soldering, 60 sec) . . . . . . . . . . . .+300°C
Package Type uJA* uJC Units
16-Lead Plastic DIP 76 33 °C/W
16-Lead SOIC 92 27 °C/W
*qJA is specified for worst case mounting conditions, i.e., qJA is specified for
device in socket for P-DIP package; qJA is specified for device soldered to
printed circuit board for SOIC package.
CAUTION
1. Do not apply voltage higher than VDD or less than DGND potential
on any terminal except VREF (Pin 15) and RFB (Pin 16).
2. The digital control inputs are Zener-protected; however,
permanent damage may occur on unprotected units from
high energy electrostatic fields. Keep units in conductive
foam at all times until ready to use.
3. Use proper antistatic handling procedures.
4. Absolute Maximum Ratings apply to packaged devices.
Stresses above those listed under Absolute Maximum Ratings
may cause permanent damage to the device.
ORDERING GUIDE
Gain Temperature Package Package
Model Nonlinearity Error Range Descriptions Options
DAC8143FP ±1 LSB ±2 LSB –40°C to +85°C 16-Lead Plastic DIP N-16
DAC8143FS ±1 LSB ±2 LSB –40°C to +85°C 16-Lead SOIC R-16W
Die Size: 99 ´ 107 mil, 10,543 sq. mils.
CAUTION
ESD (electrostatic discharge) sensitive device. Electrostatic charges as high as 4000 V readily
accumulate on the human body and test equipment and can discharge without detection.
Although the DAC8143 features proprietary ESD protection circuitry, permanent damage may
occur on devices subjected to high energy electrostatic discharges. Therefore, proper ESD
precautions are recommended to avoid performance degradation or loss of functionality.
WARNING!
ESD SENSITIVE DEVICE
DAC8143
REV. C –5–
10
FREQUENCY – Hz
THD – dB
–90
0.032
THD – %
0.010
–85
–80
–75
–70
–95
0.018
0.0056
0.0032
0.0018
100 1k 10k 100k
VIN = 5V rms
OUTPUT OP AMP: OP-42
Figure 3. Multiplying Mode Total Harmonic
Distortion vs. Frequency
Typical Performance Characteristics–
ALL BITS ON
100
FREQUENCY – Hz
B10
0
ATTENUATION – dB
(MSB) B11
B9
B8
B7
B6
B5
B4
B3
B2
B1
(LSB) B0
DATA BITS "ON"
(ALL OTHER
DATA BITS "OFF")
1k 10k 100k 1M 10M
12
24
36
48
60
72
84
96
108
Figure 2. Multiplying Mode Frequency
Response vs. Digital Code
3
0
VIN – Volts
IDD – mA
2
1
0
1 2 3 4 5
Figure 4. Supply Current vs. Logic
Input Voltage
4
1
VDD – Volts
THRESHOLD VOLTAGE – Volts
3
2
0
1
2.4
–0.8
3 5 7 9 11 13 15 17
Figure 7. Logic Threshold Voltage
vs. Supply Voltage
0.5
0
DIGITAL INPUT CODE – Decimal
LINEARITY ERROR – LSB
0.4
0.3
0.2
0.1
0.0
–0.1
–0.2
–0.3
–0.4
–0.5
512 1024 1536 2048 2560 3072 3584 4095
Figure 5. Linearity Error vs. Digital
Code
0.5
2
VREF – Volts
DNL – LSB
4 6 8 10
0.25
0
–0.25
–0.5
Figure 8. DNL Error vs. Reference
Voltage
0.5
2
VREF – Volts
INL – LSB
4 6 8 10
0.25
0
–0.25
–0.5
Figure 6. Linearity Error vs. Reference
Voltage
40
0
SRO – VOLTAGE OUT – Volts
SINK
30
20
10
0
–10
–20
–30
–40
1 2 3 4 5
SOURCE
OUTPUT CURRENT – mA
TA = +258C
LOGIC 1
LOGIC 0
Figure 9. Digital Output Voltage vs.
Output Current
DAC8143
–6– REV. C
DEFINITION OF SPECIFICATIONS
RESOLUTION
The resolution of a DAC is the number of states (2n) into which
the full-scale range (FSR) is divided (or resolved), where “n” is
equal to the number of bits.
SETTLING TIME
Time required for the analog output of the DAC to settle to
within 1/2 LSB of its final value for a given digital input stimulus;
i.e., zero to full-scale.
GAIN
Ratio of the DAC’s external operational amplifier output voltage
to the VREF input voltage when all digital inputs are HIGH.
FEEDTHROUGH ERROR
Error caused by capacitive coupling from VREF to output.
Feedthrough error limits are specified with all switches off.
OUTPUT CAPACITANCE
Capacitance from IOUT1 to ground.
OUTPUT LEAKAGE CURRENT
Current appearing at IOUT1 when all digital inputs are LOW, or
at IOUT2 terminal when all inputs are HIGH.
GENERAL CIRCUIT INFORMATION
The DAC8143 is a 12-bit serial-input, buffered serial-output,
multiplying CMOS D/A converter. It has an R-2R resistor ladder
network, a 12-bit input shift register, 12-bit DAC register,
control logic circuitry, and a buffered digital output stage.
The control logic forms an interface in which serial data is
loaded, under microprocessor control, into the input shift register
and then transferred, in parallel, to the DAC register. In
addition, buffered serial output data is present at the SRO pin
when input data is loaded into the input register. This buffered
data follows the digital input data (SRI) by 12 clock cycles and
is available for daisy-chaining additional DACs.
An asynchronous CLEAR function allows resetting the DAC
register to a zero code (0000 0000 0000) without altering data
stored in the registers.
A simplified circuit of the DAC8143 is shown in Figure 10. An
inversed R-2R ladder network consisting of silicon-chrome,
thin-film resistors, and twelve pairs of NMOS current-steering
switches. These switches steer binarily weighted currents into
either IOUT1 or IOUT2. Switching current to IOUT1 or IOUT2 yields
a constant current in each ladder leg, regardless of digital input
code. This constant current results in a constant input resistance
at VREF equal to R (typically 11 kW). The VREF input may
be driven by any reference voltage or current, ac or dc, that is
within the limits stated in the Absolute Maximum Ratings chart.
The twelve output current-steering switches are in series with
the R-2R resistor ladder, and therefore, can introduce bit errors.
It was essential to design these switches such that the switch
“ON” resistance be binarily scaled so that the voltage drop
across each switch remains constant. If, for example, Switch 1
of Figure 10 was designed with an “ON” resistance of 10 W,
Switch 2 for 20 W, etc., a constant 5 mV drop would then be
maintained across each switch.
To further ensure accuracy across the full temperature range,
permanently “ON” MOS switches were included in series with
the feedback resistor and the R-2R ladder’s terminating resistor.
The Simplified DAC Circuit, Figure 10, shows the location of
these switches. These series switches are equivalently scaled to
two times Switch 1 (MSB) and top Switch 12 (LSB) to maintain
constant relative voltage drops with varying temperature.
During any testing of the resistor ladder or RFEEDBACK (such as
incoming inspection), VDD must be present to turn “ON” these
series switches.
VREF
RFEEDBACK
IOUT2
IOUT1
10kV 10kV 10kV
20kV 20kV 20kV 20kV 20kV
S1 S2 S3 S12
10kV
BIT 1 (MSB) BIT 2 BIT 3 BIT 12 (LSB)
DIGITAL INPUTS
(SWITCHES SHOWN FOR DIGITAL INPUTS "HIGH")
*
*
*THESE SWITCHES
PERMANENTLY "ON"
Figure 10. Simplified DAC Circuit
DAC8143
REV. C –7–
ESD PROTECTION
The DAC8143 digital inputs have been designed with ESD
resistance incorporated through careful layout and the inclusion
of input protection circuitry.
Figure 11 shows the input protection diodes. High voltage static
charges applied to the digital inputs are shunted to the supply
and ground rails through forward biased diodes.
These protection diodes were designed to clamp the inputs well
below dangerous levels during static discharge conditions.
VDD
DTL/TTL/CMOS
INPUTS
Figure 11. Digital Input Protection
EQUIVALENT CIRCUIT ANALYSIS
Figures 12 and 13 show equivalent circuits for the DAC8143’s
internal DAC with all bits LOW and HIGH, respectively. The
reference current is switched to IOUT2 when all data bits are LOW,
and to IOUT1 when all bits are HIGH. The ILEAKAGE current
source is the combination of surface and junction leakages to the
substrate. The 1/4096 current source represents the constant
1-bit current drain through the ladder’s terminating resistor.
Output capacitance is dependent upon the digital input code.
This is because the capacitance of a MOS transistor changes
with applied gate voltage. This output capacitance varies between
the low and high values.
RFEEDBACK
IOUT1
IOUT2
R = 10kV
ILEAKAGE 60pF
1/4096 ILEAKAGE 90pF
R = 10kV
IREF
VREF
Figure 12. Equivalent Circuit (All Inputs LOW)
IOUT2
ILEAKAGE 60pF
RFEEDBACK
IOUT1
R = 10kV
1/4096 ILEAKAGE 90pF
R = 10kV
IREF
VREF
Figure 13. Equivalent Circuit (All Inputs HIGH)
DYNAMIC PERFORMANCE
ANALOG OUTPUT IMPEDANCE
The output resistance, as in the case of the output capacitance,
varies with the digital input code. This resistance, looking back
into the IOUT1 terminal, varies between 11 kW (the feedback
resistor alone when all digital input are LOW) and 7.5 kW (the
feedback resistor in parallel with approximately 30 kW of the
R-2R ladder network resistance when any single bit logic is
HIGH). Static accuracy and dynamic performance will be affected
by these variations.
The gain and phase stability of the output amplifier, board
layout, and power supply decoupling will all affect the dynamic
performance of the DAC8143. The use of a small compensation
capacitor may be required when high speed operational amplifiers
are used. It may be connected across the amplifier’s feedback
resistor to provide the necessary phase compensation to
critically damp the output.
The considerations when using high speed amplifiers are:
1. Phase compensation (see Figures 16 and 17).
2. Power supply decoupling at the device socket and use of
proper grounding techniques.
OUTPUT AMPLIFIER CONSIDERATIONS
When using high speed op amps, a small feedback capacitor
(typically 5 pF–30 pF) should be used across the amplifiers to
minimize overshoot and ringing. For low speed or static
applications, ac specifications of the amplifier are not very critical.
In high speed applications, slew rate, settling time, openloop
gain and gain/phase margin specifications of the amplifier
should be selected for the desired performance. It has already
been noted that an offset can be caused by including the usual
bias current compensation resistor in the amplifier’s noninverting
input terminal. This resistor should not be used. Instead, the
amplifier should have a bias current that is low over the temperature
range of interest.
Static accuracy is affected by the variation in the DAC’s output
resistance. This variation is best illustrated by using the circuit
of Figure 14 and the equation:
VERROR = VOS
1+RFB
RO
æ
è ç
ö
ø ÷
VOS
VREF
R R R
ETC
RFB
R2 R2 R2
OP-77
Figure 14. Simplified Circuit
DAC8143
–8– REV. C
Where RO is a function of the digital code, and:
RO = 10 kW for more than four bits of Logic 1,
RO = 30 kW for any single bit of Logic 1.
Therefore, the offset gain varies as follows:
at code 0011 1111 1111,
VERROR1 = VOS
1+10 kW
10 kW
æ
è ç
ö
ø ÷
= 2 VOS
at code 0100 0000 0000,
VERROR2 = VOS
1+10 kW
30 kW
æ
è ç
ö
ø ÷
= 4/3 VOS
The error difference is 2/3 VOS.
Since one LSB has a weight (for VREF = +10 V) of 2.4 mV for
the DAC8143, it is clearly important that VOS be minimized,
using either the amplifier’s pulling pins, an external pulling
network, or by selection of an amplifier with inherently low VOS.
Amplifiers with sufficiently low VOS include OP77, OP97, OP07,
OP27, and OP42.
INTERFACE LOGIC OPERATION
The microprocessor interface of the DAC8143 has been designed
with multiple STROBE and LOAD inputs to maximize interfacing
options. Control signals decoding may be done on chip or
with the use of external decoding circuitry (see Figure 21).
Serial data is clocked into the input register and buffered output
stage with STB1, STB2, or STB4. The strobe inputs are active
on the rising edge. STB3 may be used with a falling edge clock
data.
WORD N –1 WORD N
WORD N –2 WORD N –1 WORD N
BIT 2 BIT 11 BIT 12
LSB
BIT 1
MSB
BIT 12
LSB
BIT 1 BIT 2
SRI MSB
BIT 1 BIT 2
MSB
BIT 1
MSB
BIT 2 BIT 12
LSB
BIT 1
LSB
tDS1, tDS2, tDS3, tDS4
SRO
tDH1, tDH2, tDH3, tDH4
tPD
tSTB1
tSTB2
tSTB3
tSTB4
tSTB1
tSTB2
tSTB3
tSTB4
* STROBE
(STB1, STB2, STB4)
1 2 12 1 2
tLD1
tLD2
tSR1
11 12
tASB
LD1 AND LD2
LOAD NEW 12-BIT WORD INTO
INPUT REGISTER AND SHIFT
OUT PREVIOUS WORD
LOAD INPUT REGISTER'S
DATA INTO DAC REGISTER
NOTES:
* STROBE WAVEFORM IS INVERTED IF
STB3 IS USED TO STROBE SERIAL DATA
BITS INTO INPUT REGISTER.
** DATA IS STROBED INTO AND OUT OF
THE INPUT SHIFT REGISTER MSB FIRST.
Figure 15. Timing Diagram
Serial data output (SRO) follows the serial data input (SRI) by
12 clocked bits.
Holding any STROBE input at its selected state (i.e., STB1,
STB2 or STB4 at logic HIGH or STB3 at logic LOW) will act to
prevent any further data input.
When a new data word has been entered into the input register,
it is transferred to the DAC register by asserting both LOAD
inputs.
The CLR input allows asynchronous resetting of the DAC register
to 0000 0000 0000. This reset does not affect data held in
the input registers. While in unipolar mode, a CLEAR will
result in the analog output going to 0 V. In bipolar mode, the
output will go to –VREF.
INTERFACE INPUT DESCRIPTION
STB1 (Pin 4), STB2 (Pin 8), STB4 (Pin 11)—Input Register
and Buffered Output Strobe. Inputs Active on Rising
Edge. Selected to load serial data into input register and buffered
output stage. See Table I for details.
STB3 (Pin 10)—Input Register and Buffered Output
Strobe Input. Active on Falling Edge. Selected to load serial
data into input register and buffered output stage. See Table I
for details.
LD1 (Pin 5), LD2 (Pin 9)—Load DAC Register Inputs.
Active Low. Selected together to load contents of input register
into DAC register.
CLR (Pin 13)—Clear Input. Active Low. Asynchronous.
When LOW, 12-bit DAC register is forced to a zero code (0000
0000 0000) regardless of other interface inputs.
DAC8143
REV. C –9–
Table I. Truth Table
DAC8143 Logic Inputs
Input Register/
Digital Output Control Inputs DAC Register Control Inputs
STB4 STB3 STB2 STB1 CLR LD2 LD1 DAC8143 Operation Notes
0 1 0 g X X X
0 1 g 0 X X X Serial Data Bit Loaded from SRI
0 f 0 0 X X X into Input Register and Digital Output 2, 3
g 1 0 0 X X X (SRO Pin) after 12 Clocked Bits.
1 X X X
X 0 X X No Operation (Input Register and SRO) 3
X X 1 X
X X X 1
Reset DAC Register to Zero Code
0 X X (Code: 0000 0000 0000) 1, 3
(Asynchronous Operation)
1 1 X No Operation (DAC Register and SRO) 3
1 X 1
1 0 0 Load DAC Register with the Contents 3
of Input Register
NOTES
1CLR = 0 asynchronously resets DAC Register to 0000 0000 0000, but has no effect on Input Register.
2Serial data is loaded into Input Register MSB first, on edges shown. g is positive edge, f is negative edge.
30 = Logic LOW, 1 = Logic HIGH, X = Don’t Care.
APPLICATIONS INFORMATION
UNIPOLAR OPERATION (2-QUADRANT)
The circuit shown in Figures 16 and 17 may be used with an ac
or dc reference voltage. The circuit’s output will range between
0 V and +10(4095/4096) V depending upon the digital input
code. The relationship between the digital input and the analog
output is shown in Table II. The VREF voltage range is the maximum
input voltage range of the op amp or ±25 V, whichever is
lowest.
Table II. Unipolar Code Table
Digital Input Nominal Analog Output
(VOUT as Shown
MSB LSB in Figures 16 and 17)
1 1 1 1 1 1 1 1 1 1 1 1 –VREF
4095
4096
æ
è ç
ö
ø ÷
1 0 0 0 0 0 0 0 0 0 0 1 –VREF
2049
4096
æèöø
1 0 0 0 0 0 0 0 0 0 0 0 –VREF
2048
4096
æè
öø
= –
VREF
2
0 1 1 1 1 1 1 1 1 1 1 1 –VREF
2047
4096
æè
öø
0 0 0 0 0 0 0 0 0 0 0 1 –VREF
1
4096
æè
öø
0 0 0 0 0 0 0 0 0 0 0 0 –VREF
0
4096
æè
öø
= 0
NOTES
1Nominal full scale for the circuits of Figures 16 and 17 is given by
FS = –VREF
4095
4096
æ
è ç
ö
ø ÷
.
2Nominal LSB magnitude for the circuits of Figures 16 and 17 is given by
LSB = VREF
1
4096
æ
è ç
ö
ø ÷
or VREF(2–n).
OP-77
+5V
VREF VDD
RFEEDBACK
IOUT1
IOUT2
AGND
DGND
SRO
(BUFFERED
DIGITAL
DATA OUT)
15pF
+15V
–15V
VOUT
7
6
4
3
2
15 14
13
4, 5
8–11
7
1
2
3
6
12
CONTROL DAC8143
INPUTS
SRI
(SERIAL
DATA IN)
VREF
–10V
CLR
Figure 16. Unipolar Operation with High Accuracy Op
Amp (2-Quadrant)
OP-42
+5V
VREF VDD RFEEDBACK
IOUT1
IOUT2
AGND
DGND
SRO
(BUFFERED
DIGITAL
DATA OUT)
15pF
+15V
–15V
VOUT
7
6
4
3
2
15 14
13
4, 5
8–11
7
1
2
3
6
12
CONTROL DAC8143
INPUTS
SRI
(SERIAL
DATA IN)
VREF
–10V
R2
50V
R1
100V
CLR
Figure 17. Unipolar Operation with Fast Op Amp and
Gain Error Trimming (2-Quadrant)
DAC8143
–10– REV. C
In many applications, the DAC8143’s zero scale error and low
gain error, permit the elimination of external trimming components
without adverse effects on circuit performance.
For applications requiring a tighter gain error than 0.024% at
25°C for the top grade part, or 0.048% for the lower grade part,
the circuit in Figure 17 may be used. Gain error may be trimmed
by adjusting R1.
The DAC register must first be loaded with all 1s. R1 is then
adjusted until VOUT = –VREF (4095/4096). In the case of an
adjustable VREF, R1 and RFEEDBACK may be omitted, with VREF
adjusted to yield the desired full-scale output.
BIPOLAR OPERATION (4-QUADRANT)
Figure 18 details a suggested circuit for bipolar, or offset binary,
operation. Table III shows the digital input-to-analog output
relationship. The circuit uses offset binary coding. Twos complement
code can be converted to offset binary by software inversion
of the MSB or by the addition of an external inverter to the
MSB input.
Resistor R3, R4 and R5 must be selected to match within 0.01%
and must all be of the same (preferably metal foil) type to assure
temperature coefficient match. Mismatching between R3 and
R4 causes offset and full-scale error.
Calibration is performed by loading the DAC register with
1000 0000 0000 and adjusting R1 until VOUT = 0 V. R1 and
R2 may be omitted by adjusting the ratio of R3 to R4 to yield
VOUT = 0 V. Full scale can be adjusted by loading the DAC
register with 1111 1111 1111 and adjusting either the amplitude
of VREF or the value of R5 until the desired VOUT is achieved.
Table III. Bipolar (Offset Binary) Code Table
Digital Input Nominal Analog Output
MSB LSB (VOUT as Shown in Figure 18)
1 1 1 1 1 1 1 1 1 1 1 1 +VREF
2047
2048
æè
öø
1 0 0 0 0 0 0 0 0 0 0 1 +VREF
1
2048
æè
öø
1 0 0 0 0 0 0 0 0 0 0 0 0
0 1 1 1 1 1 1 1 1 1 1 1 –VREF
1
2048
æè
öø
0 0 0 0 0 0 0 0 0 0 0 1 –VREF
2047
2048
æè
öø
0 0 0 0 0 0 0 0 0 0 0 0 –VREF
2048
2048
æè
öø
NOTES
1Nominal full scale for the circuits of Figure 18 is given by
FS = VREF
2047
2048
æè
öø
.
2Nominal LSB magnitude for the circuits of Figure 18 is given by
LSB = VREF
1
2048
æè
öø
.
DAISY-CHAINING DAC8143s
Many applications use multiple serial input DACs that use
numerous interconnecting lines for address decoding and data
lines. In addition, they use some type of buffering to reduce
loading on the bus. The DAC8143 is ideal for just such an
application. It not only reduces the number of interconnecting
lines, but also reduces bus loading. The DAC8143 can be daisychained
with only three lines: one data line, one CLK line and
one load line, see Figure 19.
VOUT
1/2 OP200
+5V
R2
50V
12
15
7
R1
100V
SERIAL
DATA INPUT
VIN
14 15
1
2
3
8-11 4, 5 13 6
DGND
VREF
SRI
CONTROL
BITS SRO
CONTROL
INPUTS
FROM
SYSTEM
RESET
BUFFERED SERIAL
DATA OUT
VDD RFB
AGND
IOUT2
IOUT1
DAC8143
C1
10-33pF
COMMON GROUND
R3
A1 10kV
R4
20kV R5
20kV
1/2 OP200
A2
CLR
Figure 18. Bipolar Operation (4-Quadrant, Offset Binary)
DAC8143
REV. C –11–
ANALOG/DIGITAL DIVISION
The transfer function for the DAC8143 connect in the multiplying
mode as shown in Figures 16 and 17 is:
VO = –VIN
A1
21 + A2
22 + A3
23 + ...
A12
212
æ
è ç
ö
ø ÷
where AX assumes a value of 1 for an “ON” bit and 0 for an
“OFF” bit.
The transfer function is modified when the DAC is connected in
the feedback of an operational amplifier as shown in Figure 20
and is:
VO =
–VIN
A1
21 + A2
22 + A3
23 + ...
A12
212
æ
è
ççç
ö
ø
÷÷÷
The above transfer function is the division of an analog voltage
(VREF) by a digital word. The amplifier goes to the rails with all
bits “OFF” since division by zero is infinity. With all bits “ON”
the gain is 1 (±1 LSB). The gain becomes 4096 with the LSB,
Bit 12, “ON”.
BUFFERED DIGITAL
DATA OUT
+5V
SRO
VREF
RFB VDD
IOUT1
DAC8143
AGND 3 2 12 DGND
15
6
16 14
1
3
2
6
VIN
VOUT
4 13
DIGITAL
INPUTS
OP-42
+
–
Figure 20. Analog/Digital Divider
APPLICATION TIPS
In most applications, linearity depends on the potential of IOUT1,
IOUT2, and AGND (Pins 1, 2 and 3) being exactly equal to each
other. In most applications, the DAC is connected to an external
op amp with its noninverting input tied to ground (see Figures
16 and 17). The amplifier selected should have a low input
bias current and low drift over temperature. The amplifier’s
input offset voltage should be nulled to less than ±200 mV (less
than 10% of 1 LSB).
The operational amplifier’s noninverting input should have a
minimum resistance connection to ground; the usual bias current
compensation resistor should not be used. This resistor can
cause a variable offset voltage appearing as a varying output
error. All grounded pins should tie to a single common ground
point, avoiding ground loops. The VDD power supply should
have a low noise level with no transients greater than +17 V.
It is recommended that the digital inputs be taken to ground or
VDD via a high value (1 MW) resistor; this will prevent the accumulation
of static charge if the PC card is disconnected from the
system.
Peak supply current flows as the digital input pass through the
transition region (see Figure 4). The supply current decreases as
the input voltage approaches the supply rails (VDD or DGND),
i.e., rapidly slewing logic signals that settle very near the supply
rails will minimize supply current.
INTERFACING TO THE MC6800
As shown in Figure 21, the DAC8143 may be interfaced to the
6800 by successively executing memory WRITE instruction
while manipulating the data between WRITEs, so that each
WRITE presents the next bit.
In this example, the most significant bits are found in memory
locations 0000 and 0001. The four MSBs are found in the lower
half of 0000, the eight LSBs in 0001. The data is taken from the
DB7 line.
The serial data loading is triggered by STB4 which is asserted by
a decoded memory WRITE to a memory location, R/W, and
F2. A WRITE to another address location transfers data from
input register to DAC register.
STB1
DAC8143*
SRI
SRO
LD2
LD1
STB3
STB2
STB4 CLR
74LS138
ADDRESS
DECODER
E1 A0 A2
E3
E2
A0
A15
R/W
DB0
DB7
MC6800
16-BIT ADDRESS BUS
8-BIT DATA BUS
+5V
FROM SYSTEM RESET
*ANALOG CIRCUITRY OMITTED FOR SIMPLICITY
f2
Figure 21. DAC8143—MC6800 Interface
ADDRESS
DECODER
STROBE
LOAD
DAC8143
SRI
SRO
ADDRESS BUS
STROBE
LOAD
DAC8143
SRI
SRO
STROBE
LOAD
DAC8143
SRI
SRO
STROBE
LOAD
DAC8143
SRI
SRO
DBX
mP
WR
Figure 19. Multiple DAC8143s with Three-Wire Interface
DAC8143
–12– REV. C
DAC8143 INTERFACE TO THE 8085
The DAC8143’s interface to the 8085 microprocessor is shown
in Figure 22. Note that the microprocessor’s SOD line is used
to present data serially to the DAC.
Data is strobed into the DAC8143 by executing memory write
instructions. The strobe 2 input is generated by decoding an
address location and WR. Data is loaded into the DAC register
with a memory write instruction to another address location.
Serial data supplied to the DAC8143 must be present in the
right-justified format in registers H and L of the microprocessor.
STB1 DAC8143*
SRI
SRO
LD2
LD1
STB3
STB2
STB4
CLR
74LS138
ADDRESS
DECODER
E1 A0 A2
E3
E2 WR
ALE
SOD
8085
ADDRESS BUS (16)
DATA
+5V
FROM SYSTEM RESET
*ANALOG CIRCUITRY OMITTED FOR SIMPLICITY
+5V
A0–A15
8212
(8)
(8)AD0–7
Figure 22. DAC8143—8085 Interface
DAC8143 INTERFACE TO THE 68000
Figure 23 shows the DAC8143 configured to the 68000 microprocessor.
Serial data input is similar to that of the 6800 in
Figure 21.
STB1
DAC8143
SRI
LD2
LD1
STB3
STB4
CLR
ADDRESS
DECODER
A1
A23
AS
DB15
DB0
68000mP
ADDRESS BUS
DATA BUS
FROM SYSTEM RESET
CS
VMA
VPA
UDS
+5V
1/4 74HC125
+
STB2
Figure 23. DAC8143 to 68000 mP Interface
OUTLINE DIMENSIONS
Dimensions are shown in inches and (mm).
16-Lead Plastic DIP
(N-16)
16
1 8
9
PIN 1
0.840 (21.34)
0.745 (18.92)
0.280 (7.11)
0.240 (6.10)
SEATING
PLANE
0.060 (1.52)
0.015 (0.38)
0.210 (5.33)
MAX
0.022 (0.558)
0.014 (0.356)
0.160 (4.06)
0.115 (2.93)
0.100
(2.54)
BSC
0.070 (1.77)
0.045 (1.15)
0.130
(3.30)
MIN
0.195 (4.95)
0.115 (2.93)
0.015 (0.381)
0.008 (0.204)
0.325 (8.25)
0.300 (7.62)
16-Lead SOIC
(R-16W)
SEATING
PLANE
0.0118 (0.30)
0.0040 (0.10)
0.0192 (0.49)
0.0138 (0.35)
0.1043 (2.65)
0.0926 (2.35)
0.050 (1.27)
BSC
16 9
1 8
0.4193 (10.65)
0.3937 (10.00)
0.2992 (7.60)
0.2914 (7.40)
PIN 1
0.4133 (10.50)
0.3977 (10.00)
0.0125 (0.32)
0.0091 (0.23)
88
08
0.0291 (0.74)
0.0098 (0.25)3 458
0.0500 (1.27)
0.0157 (0.40)
PRINTED IN U.S.A. C3114c–2–3/99
Low Voltage, Low Power,
Factory-Calibrated 16-/24-Bit Dual - ADC
FEATURES
HIGH RESOLUTION - ADCs
2 Independent ADCs (16- and 24-Bit Resolution)
Factory-Calibrated (Field Calibration Not Required)
Output Settles in 1 Conversion Cycle (Single
Conversion Mode)
Programmable Gain Front End
Simultaneous Sampling and Conversion of 2
Signal Sources
Separate Reference Inputs for Each Channel
Simultaneous 50 Hz and 60 Hz Rejection at 20 Hz
Update Rate
ISOURCE Select™
24-Bit No Missing Codes—Main ADC
13-Bit p-p Resolution @ 20 Hz, 20 mV Range
18-Bit p-p Resolution @ 20 Hz, 2.56 V Range
INTERFACE
3-Wire Serial
SPI®, QSPI™, MICROWIRE™, and DSP Compatible
Schmitt Trigger on SCLK
POWER
Specified for Single 3 V and 5 V Operation
Normal: 1.5 mA Typ @ 3 V
Power-Down: 10 A (32 kHz Crystal Running)
ON-CHIP FUNCTIONS
Rail-Rail Input Buffer and PGA
4-Bit Digital I/O Port
On-Chip Temperature Sensor
Dual Switchable Excitation Current Sources
Low-Side Power Switches
Reference Detect Circuit
APPLICATIONS
Sensor Measurement
Temperature Measurement
Pressure Measurement
Weigh Scales
Portable Instrumentation
4 to 20 mA Transmitters
GENERAL DESCRIPTION
The AD7719 is a complete analog front end for low frequency
measurement applications. It contains two high resolution Σ-Δ
ADCs, switchable matched excitation current sources, low-side
power switches, digital I/O port, and temperature sensor. The
24-bit main channel with PGA accepts fully differential, unipolar,
and bipolar input signal ranges from 1.024 × REFIN1/128 to
1.024 × REFIN1. Signals can be converted directly from a transducer
without the need for signal conditioning. The 16-bit auxiliary
channel has an input signal range of REFIN2 or REFIN2/2.
The device operates from a 32 kHz crystal with an on-chip
PLL generating the required internal operating frequency. The
output data rate from the part is software programmable. The
peak-to-peak resolution from the part varies with the programmed
gain and output data rate.
The part operates from a single 3 V or 5 V supply. When operating
from 3 V supplies, the power dissipation for the part is
4.5 mW with both ADCs enabled and 2.85 mW with only the
main ADC enabled in unbuffered mode. The AD7719 is housed
in 28-lead SOIC and TSSOP packages.
FUNCTIONAL BLOCK DIAGRAM
MUX
AVDD
IEXC1
200A
IEXC2
200A
AVDD
AGND
MUX1 BUF PGA
TEMP
SENSOR
I/O PORT
AVDD
MAIN CHANNEL
24-BIT - ADC
AUXILIARY CHANNEL
16-BIT - ADC
REFERENCE
DETECT
OSC. AND
PLL
SERIAL
INTERFACE
AND
CONTROL
LOGIC
DVDD DGND
IOUT1
IOUT2
AIN1
AIN2
AIN3
AIN4
AIN5
AIN6
AVDD AGND REFIN2 PWRGND P1/SW1 P2/SW2 P3 P4
DOUT
DIN
SCLK
CS
RDY
RESET
REFIN1(+) REFIN1(–) XTAL1 XTAL2
AD7719
MUX2
REV. A
AD7719
–2–
FEATURES . . . . . . . . . . . . . . . . . . . . . . . . . . . . . . . . . . . . . 1
GENERAL DESCRIPTION . . . . . . . . . . . . . . . . . . . . . . . . . 1
SPECIFICATIONS . . . . . . . . . . . . . . . . . . . . . . . . . . . . . . . 3
ABSOLUTE MAXIMUM RATINGS . . . . . . . . . . . . . . . . . 6
ORDERING GUIDE . . . . . . . . . . . . . . . . . . . . . . . . . . . . . . 6
TIMING CHARACTERISTICS . . . . . . . . . . . . . . . . . . . . . 7
DIGITAL INTERFACE . . . . . . . . . . . . . . . . . . . . . . . . . . . . 8
PIN CONFIGURATION . . . . . . . . . . . . . . . . . . . . . . . . . . . 9
PIN FUNCTION DESCRIPTIONS . . . . . . . . . . . . . . . . . . 9
TYPICAL PERFORMANCE CHARACTERISTICS . . . . 11
DUAL-CHANNEL ADC CIRCUIT INFORMATION . . . 12
Overview . . . . . . . . . . . . . . . . . . . . . . . . . . . . . . . . . . . . . 12
Main Channel . . . . . . . . . . . . . . . . . . . . . . . . . . . . . . . . . 12
Auxiliary Channel . . . . . . . . . . . . . . . . . . . . . . . . . . . . . . 12
Both Channels . . . . . . . . . . . . . . . . . . . . . . . . . . . . . . . . . 13
MAIN AND AUXILIARY ADC NOISE
PERFORMANCE . . . . . . . . . . . . . . . . . . . . . . . . . . . . . . 14
ON-CHIP REGISTERS . . . . . . . . . . . . . . . . . . . . . . . . . . . 16
Communications Register
(A3, A2, A1, A0 = 0, 0, 0, 0) . . . . . . . . . . . . . . . . . . . . 19
Status Register (A3, A2, A1, A0 = 0, 0, 0, 0;
Power-On Reset = 0x00) . . . . . . . . . . . . . . . . . . . . . . . 20
Mode Register (A3, A2, A1, A0 = 0, 0, 0, 1;
Power-On Reset = 0x00) . . . . . . . . . . . . . . . . . . . . . . . 21
Operating Characteristics when Addressing the
Mode and Control Registers . . . . . . . . . . . . . . . . . . . . . 22
Main ADC Control Register (AD0CON):
(A3, A2, A1, A0 = 0, 0, 1, 0;
Power-On Reset = 0x07) . . . . . . . . . . . . . . . . . . . . . . . 22
Aux ADC Control Registers (AD1CON):
(A3, A2, A1, A0 = 0, 0, 1, 1;
Power-On Reset = 0x01) . . . . . . . . . . . . . . . . . . . . . . . 23
Filter Register (A3, A2, A1, A0 = 0, 1, 0, 0;
Power-On Reset = 0x45) . . . . . . . . . . . . . . . . . . . . . . . 24
I/O and Current Source Control Register (IOCON):
(A3, A2, A1, A0 = 0, 1, 1, 1;
Power-On Reset = 0x0000) . . . . . . . . . . . . . . . . . . . . . 24
Main ADC Data Result Registers (DATA0):
(A3, A2, A1, A0 = 0, 1, 0, 1;
Power-On Reset = 0x00 0000) . . . . . . . . . . . . . . . . . . . 26
Aux ADC Data Result Registers (DATA1):
(A3, A2, A1, A0 = 0, 1, 1, 0;
Power-On Reset = 0x0000) . . . . . . . . . . . . . . . . . . . . . 26
Main ADC Offset Calibration Coefficient Registers (OF0):
(A3, A2, A1, A0 = 1, 0, 0, 0;
Power-On Reset = 0x80 0000) . . . . . . . . . . . . . . . . . . . 26
Aux ADC Offset Calibration Coefficient Registers (OF1):
(A3, A2, A1, A0 = 1, 0, 0, 1;
Power-On Reset = 0x8000) . . . . . . . . . . . . . . . . . . . . . 26
Main ADC Gain Calibration Coefficient Registers (GNO):
(A3, A2, A1, A0 = 1, 0, 1, 0;
Power-On Reset = 0x5X XXX5) . . . . . . . . . . . . . . . . . 26
Aux ADC Gain Calibration Coefficient Registers (GN1):
(A3, A2, A1, A0 = 1, 0, 1, 1;
Power-On Reset = 0x59XX) . . . . . . . . . . . . . . . . . . . . . 26
ID Register (ID): (A3, A2, A1, A0 = 1, 1, 1, 1;
Power-On Reset = 0x0X) . . . . . . . . . . . . . . . . . . . . . . . 26
User Nonprogrammable Test Registers . . . . . . . . . . . . . . 26
CONFIGURING THE AD7719 . . . . . . . . . . . . . . . . . . . . . 27
MICROCOMPUTER/MICROPROCESSOR
INTERFACING . . . . . . . . . . . . . . . . . . . . . . . . . . . . . . . 27
AD7719-to-68HC11 Interface . . . . . . . . . . . . . . . . . . . . . 29
AD7719-to-8051 Interface . . . . . . . . . . . . . . . . . . . . . . . . 29
AD7719-to-ADSP-2103/ADSP-2105 Interface . . . . . . . . 30
CIRCUIT DESCRIPTION . . . . . . . . . . . . . . . . . . . . . . . . 30
Analog Input Channels . . . . . . . . . . . . . . . . . . . . . . . . . . 31
Programmable Gain Amplifier . . . . . . . . . . . . . . . . . . . . . 32
Bipolar/Unipolar Configuration . . . . . . . . . . . . . . . . . . . . 32
Data Output Coding . . . . . . . . . . . . . . . . . . . . . . . . . . . . 33
Burnout Currents . . . . . . . . . . . . . . . . . . . . . . . . . . . . . . . 33
Excitation Currents . . . . . . . . . . . . . . . . . . . . . . . . . . . . . 33
Crystal Oscillator . . . . . . . . . . . . . . . . . . . . . . . . . . . . . . . 33
Reference Input . . . . . . . . . . . . . . . . . . . . . . . . . . . . . . . . 33
Reference Detect . . . . . . . . . . . . . . . . . . . . . . . . . . . . . . . 34
Reset Input . . . . . . . . . . . . . . . . . . . . . . . . . . . . . . . . . . . 34
Power-Down Mode . . . . . . . . . . . . . . . . . . . . . . . . . . . . . 34
Idle Mode . . . . . . . . . . . . . . . . . . . . . . . . . . . . . . . . . . . . 34
ADC Disable Mode . . . . . . . . . . . . . . . . . . . . . . . . . . . . . 34
Calibration . . . . . . . . . . . . . . . . . . . . . . . . . . . . . . . . . . . . 34
Grounding and Layout . . . . . . . . . . . . . . . . . . . . . . . . . . . 35
APPLICATIONS . . . . . . . . . . . . . . . . . . . . . . . . . . . . . . . . 35
Pressure Measurement . . . . . . . . . . . . . . . . . . . . . . . . . . . 36
Temperature Measurement . . . . . . . . . . . . . . . . . . . . . . . 36
3-Wire RTD Configurations . . . . . . . . . . . . . . . . . . . . . . 37
Smart Transmitters . . . . . . . . . . . . . . . . . . . . . . . . . . . . . 38
OUTLINE DIMENSIONS . . . . . . . . . . . . . . . . . . . . . . . . . 39
REVISION HISTORY . . . . . . . . . . . . . . . . . . . . . . . . . . . . 40
TABLE OF CONTENTS
REV. A –3–
AD7719 (AVDD = 2.7 V to 3.6 V or 4.75 V to 5.25 V, DVDD = 2.7 V to 3.6 V or 4.75 V to 5.25 V,
REFIN(+) = 2.5 V; REFIN(–) = AGND; AGND = DGND = 0 V; XTAL1/XTAL2 = 32.768 kHz Crystal; all specifications TMIN to TMAX, unless otherwise noted.)
Parameter AD7719B Unit Test Conditions
ADC CHANNEL SPECIFICATION
Output Update Rate 5.4 Hz min Both Channels Synchronized
105 Hz max 0.732 ms Increments
MAIN CHANNEL
No Missing Codes2 24 Bits min 20 Hz Update Rate
Resolution 13 Bits p-p ±20 mV Range, 20 Hz Update Rate
18 Bits p-p ±2.56 V Range, 20 Hz Update Rate
Output Noise and Update Rates See Tables II to V
Integral Nonlinearity ±10 ppm of FSR max Typically 2 ppm.
Offset Error3 ±3 μV typ
Offset Error Drift vs. Temperature4 ±10 nV/°C typ
Full-Scale Error5, 6, 7 ±10 μV typ At the Calibrated Conditions
Gain Drift vs. Temperature4 ±0.5 ppm/°C typ
Power Supply Rejection (PSR) 80 dB min Input Range = ±2.56 V, 100 dB typ.
110 dB typ on ±20 mV Range
ANALOG INPUTS
Differential Input Voltage Ranges ±1.024 × REFIN1/GAIN V nom REFIN1 = REFIN1(+) – REFIN1(–)
GAIN = 1 to 128.
ADC Range Matching ±2 μV typ Input Voltage = 19 mV on All Ranges
Absolute AIN Voltage Limits AGND + 100 mV V min BUF = 0; Buffered Mode of Operation
AVDD – 100 mV V max
Analog Input Current2 BUF = 0
DC Input Current ±1 nA max
DC Input Current Drift ±5 pA /°C typ
Absolute AIN Voltage Limits AGND – 30 mV V min BUF = 1; Unbuffered mode of operation.
AVDD + 30 mV V max
Analog Input Current BUF = 1. Unbuffered Mode of Operation.
DC Input Current ±125 nA/V typ Input Current Varies with Input Voltage
DC Input Current Drift ±2 pA/V/°C typ
Normal-Mode Rejection2, 8
@ 50 Hz 100 dB min 50 Hz ± 1 Hz, 16.65 Hz Update Rate, SF = 82
@ 60 Hz 100 dB min 60 Hz ± 1 Hz, 20 Hz Update Rate, SF = 68
Common-Mode Rejection
@ DC 90 dB min Input Range = ±2.56 V, AIN = 1 V.
100 dB typ. 110 dB typ on ±20 mV Range
@ 50 Hz2 100 dB min 50 Hz ± 1 Hz, Range = ±2.56 V, AIN = 1 V
@ 60 Hz2 100 dB min 60 Hz ± 1 Hz, Range = ±2.56 V, AIN = 1 V
REFERENCE INPUT (REFIN1)
REFIN1 Voltage 2.5 V nom REFIN1 = REFIN1(+) – REFIN1(–)
REFIN1 Voltage Range2 1 V min
AVDD V max
REFIN1 Common-Mode Range AGND – 30 mV V min
AVDD + 30 mV V max
Reference DC Input Current 0.5 μA/V typ
Reference DC Input Current Drift ±0.01 nA/V/°C typ
Normal-Mode Rejection2, 8
@ 50 Hz 100 dB min 50 Hz ± 1 Hz, SF = 82
@ 60 Hz 100 dB min 60 Hz ± 1 Hz, SF = 68
Common-Mode Rejection
@ DC 110 dB typ Input Range = ±2.56 V, AIN = 1 V
@ 50 Hz 110 dB typ 50 Hz ± 1 Hz, Range = 2.56 V, AIN = 1 V
@ 60 Hz 110 dB typ 60 Hz ± 1 Hz, Range = 2.56 V, AIN = 1 V
Reference Detect Levels 0.3 V min NOXREF Bit Active if VREF < 0.3 V
0.65 V max NOXREF Bit Inactive if VREF > 0.65 V
AUXILIARY CHANNEL
No Missing Codes2 16 Bits min
Resolution 16 Bits p-p ±2.5 V Range, 20 Hz Update Rate
Output Noise and Update Rates See Tables VI and VIII
Integral Nonlinearity ±15 ppm of FSR max
FSR
REFIN
Gain
= 2 × 1.024 1
–SPECIFICATIONS1
REV. A
AD7719
–4–
Parameter AD7719B Unit Test Conditions
AUXILIARY CHANNEL (continued)
Offset Error3 ±3 μV typ Selected Channel = AIN5/AIN6
Offset Error Drift vs. Temperature4 ±10 nV/°C typ
Full-Scale Error6, 7 ±0.75 LSB typ
Gain Drift vs. Temperature4 0.5 ppm/°C typ
Negative Full-Scale Error ±1 LSB typ
Power Supply Rejection (PSR) 70 dB min AIN = 1 V Input Range = ±2.5 V, Typically 80 dB
ANALOG INPUTS
Differential Input Voltage Ranges ±REFIN2 V nom ARN = 1
±REFIN2/2 V nom ARN = 0
Absolute AIN Voltage Limits AGND – 30 mV V min Unbuffered Input
AVDD + 30 mV V max
Analog Input Current
DC Input Current ±125 nA/V typ Input Current Varies with Input Voltage
DC Input Current Drift ±2 pA/V/°C typ
Normal-Mode Rejection2, 8
@ 50 Hz 100 dB min 50 Hz ±1 Hz, SF = 82
@ 60 Hz 100 dB min 60 Hz ±1 Hz, SF = 68
Common-Mode Rejection
@ DC 85 dB min Input Range = ±2.5 V, AIN = 1 V
@ 50 Hz2 90 dB min 50 Hz ±1 Hz, Range = 2.5 V, AIN = 1 V
@ 60 Hz2 90 dB min 60 Hz ±1 Hz, Range = 2.5 V, AIN = 1 V
REFERENCE INPUT (REFIN2) With Respect to AGND
REFIN2 Voltage 2.5 V nom
REFIN2 Range2 1 V min
AVDD V max
Reference DC Input Current2 0.2 μA/V typ
Reference DC Input Current Drift 0.003 nA/V/°C typ
EXCITATION CURRENT SOURCES
(IEXC1 and IEXC2)
Output Current 200 μA nom
Initial Tolerance at 25°C ±10 % typ
Drift 200 ppm/°C typ
Initial Current Matching at 25°C ±1 % typ Matching between IEXC1 and IEXC2
No Load
Drift Matching 20 ppm/°C typ
Line Regulation (AVDD) 2.1 μA/V max AVDD = 5 V ± 5%. Typically 1.25 μA/V
Load Regulation 300 nA/V typ
Output Compliance AVDD – 0.6 V max
AGND – 30 mV V min
LOW-SIDE POWER SWITCHES
(SW1, SW2)
RON 5 Ω max AVDD = 5 V. Typically 3 Ω
7 Ω max AVDD = 3 V. Typically 4.5 Ω
Allowable Current2 20 mA max Continuous Current per Switch
TEMPERATURE SENSOR
Accuracy See TPC 5 °C typ
TRANSDUCER BURNOUT
AIN(+) Current –100 nA typ
AIN(–) Current 100 nA typ
Initial Tolerance @ 25°C ±15 % typ
Drift 0.03 %/°C typ
SYSTEM CALIBRATION2, 9
Full-Scale Calibration Limit 1.05 × FS10 V max
Zero-Scale Calibration Limit –1.05 × FS V min
Input Span 0.8 × FS V min
2.1 × FS V max
REV. A –5–
AD7719
Parameter AD7719B Unit Test Conditions
LOGIC INPUTS
All Inputs Except SCLK and XTAL12
VINL, Input Low Voltage 0.8 V max DVDD = 5 V
0.4 V max DVDD = 3 V
VINH, Input High Voltage 2.0 V min DVDD = 3 V or 5 V
SCLK Only (Schmitt-Triggered Input)2
VT(+) 1.4/2 V min/V max DVDD = 5 V
VT(–) 0.8/1.4 V min/V max DVDD = 5 V
VT(+) – VT(–) 0.3/0.85 V min/V max DVDD = 5 V
VT(+) 0.95/2 V min/V max DVDD = 3 V
VT(–) 0.4/1.1 V min/V max DVDD = 3 V
VT(+) – VT(–) 0.3/0.85 V min/V max DVDD = 3 V
XTAL1 Only2
VINL, Input Low Voltage 0.8 V max DVDD = 5 V
VINH, Input High Voltage 3.5 V min DVDD = 5 V
VINL, Input Low Voltage 0.4 V max DVDD = 3 V
VINH, Input High Voltage 2.5 V min DVDD = 3 V
Input Currents ±10 μA max VIN = DVDD
–70 μA max VIN = DGND, Typically –40 μA at 5 V
and –20 μA at 3 V
Input Capacitance2 10 pF typ All Digital Inputs
LOGIC OUTPUTS (Excluding XTAL2)
VOH, Output High Voltage2 DVDD – 0.6 V min DVDD = 3 V, ISOURCE = 100 μA
VOL, Output Low Voltage2 0.4 V max DVDD = 3 V, ISINK = 100 μA
VOH, Output High Voltage2 4 V min DVDD = 5 V, ISOURCE = 200 μA
VOL, Output Low Voltage 2 0.4 V max DVDD = 5 V, ISINK = 1.6 mA
Floating-State Leakage Current ±10 μA max
Floating-State Output Capacitance ±10 pF typ
Data Output Coding Binary Unipolar Mode
Offset Binary Bipolar Mode
I/O PORT11 I/O Port Voltages Are with Respect to
AVDD and AGND
VINL, Input Low Voltage2 0.8 V max AVDD = 5 V
0.4 V max AVDD = 3 V
VINH, Input High Voltage2 2.0 V min AVDD = 3 V or 5 V
Input Currents ±10 μA max VIN = AVDD
–70 μA max VIN = AGND, Typically –40 μA at AVDD = 5 V
and –20 μA at AVDD = 3 V
Input Capacitance 10 pF typ All Digital Inputs
VOH, Output High Voltage2 AVDD – 0.6 V min AVDD = 3 V, ISOURCE = 100 μA
VOL, Output Low Voltage2 0.4 V max AVDD = 3 V, ISINK = 100 μA
VOH, Output High Voltage2 4 V min AVDD = 5 V, ISOURCE = 200 μA
VOL, Output Low Voltage2 0.4 V max AVDD = 5 V, ISINK = 1.6 mA
Floating-State Output Leakage Current ±10 μA max
Floating-State Output Capacitance ±10 pF typ
START-UP TIME
From Power-On 300 ms typ
From Idle Mode 1 ms typ
From Power-Down Mode 1 ms typ Osc. Active in Power-Down
300 ms typ Osc. Powered Down
POWER REQUIREMENTS
Power Supply Voltages
AVDD – AGND 2.7/3.6 V min/max AVDD = 3 V nom
4.75/5.25 V min/max AVDD = 5 V nom
DVDD – DGND 2.7/3.6 V min/max DVDD = 3 V nom
4.75/5.25 V min DVDD = 5 V nom
Power Supply Currents
DIDD Current (Normal Mode)12 0.6 mA max DVDD = 3 V, 0.5 mA typ
0.75 mA max DVDD = 5 V, 0.6 mA typ
REV. A
AD7719
–6–
Parameter AD7719B Unit Test Conditions
Power Supply Currents (Continued)
AIDD Current (Main ADC) 1.1 mA max AVDD = 3 V or 5 V, Buffered Mode,
0.85 mA typ
0.55 mA max AVDD = 3 V or 5 V, Unbuffered Mode,
0.45 mA typ
AIDD Current (Aux ADC) 0.3 mA max AVDD = 3 V or 5 V, 0.25 mA typ
AIDD Current (Main and Aux ADC) 1.25 mA max AVDD = 3 V or 5 V, Main ADC Buffered,
1 mA typ
DIDD (ADC Disable Mode)13 0.35 mA max DVDD = 3 V, 0.25 mA typ
0.4 mA max DVDD = 5 V, 0.3 mA typ
AIDD (ADC Disable Mode) 0.15 mA max AVDD = 3 V or 5 V
DIDD (Power-Down Mode) 10 μA max DVDD = 3 V, 32.768 kHz Osc. Running
2 μA max DVDD = 3 V, Oscillator Powered Down
30 μA max DVDD = 5 V, 32.768 kHz Osc. Running
8 μA max DVDD = 5 V, Oscillator Powered Down
AIDD (Power-Down Mode) 1 μA max AVDD = 3 V or 5 V
NOTES
1Temperature range –40°C to +85°C.
2Guaranteed by design and/or characterization data on production release.
3System zero calibration will remove this error.
4A calibration at any temperature will remove this drift error.
5The main ADC is factory-calibrated with AVDD = DVDD = 4 V, TA = 25°C, REFIN1(+) – REFIN1(–) = 2.5 V. If the user power supplies or temperature conditions
are significantly different from these, internal full-scale calibration will restore this error to the published specification. System calibration can be used to reduce this
error to the order of the noise. Full-scale error applies to both positive and negative full scale.
6A system full-scale calibration will remove this error.
7A typical gain error of ±10 μV results following a user self-calibration.
8Simultaneous 50 Hz and 60 Hz rejection is achieved using 19.8 Hz (SF = 69) update rate. Normal mode rejection in this case is 60 dB min.
9After a calibration if the analog input exceeds positive full scale, the converter will output all 1s. If the analog input is less than negative full scale, the device will
output all 0s.
10FS = Full-Scale Input. FS = 1.024 × REFIN1/Gain on the main ADC, where REFIN1 = REFIN1(+) – REFIN1(–). FS = REFIN2 on the aux ADC when ARN = 1
in the aux ADC control register (AD1CON) and REFIN2/2 on the aux ADC when ARN = 0.
11 Input and output levels on the I/O Port are with respect to AVDD and AGND.
12Normal mode refers to the case where both main and aux ADCs are running.
13ADC disable is entered by setting both the AD0EN and AD1EN bits in the main and aux ADC control registers to a 0 and setting the mode bits (MD2, MD1,
MD0) in the mode register to non-0.
Specifications subject to change without notice.
ABSOLUTE MAXIMUM RATINGS1
(TA = 25°C, unless otherwise noted.)
AVDD to AGND . . . . . . . . . . . . . . . . . . . . . . . –0.3 V to +7 V
AVDD to DGND . . . . . . . . . . . . . . . . . . . . . . . –0.3 V to +7 V
DVDD to AGND . . . . . . . . . . . . . . . . . . . . . . . –0.3 V to +7 V
DVDD to DGND . . . . . . . . . . . . . . . . . . . . . . . –0.3 V to +7 V
AGND to DGND2. . . . . . . . . . . . . . . . . . . –20 mV to +20 mV
PWRGND to AGND . . . . . . . . . . . . . . . . –20 mV to +20 mV
AVDD to DVDD . . . . . . . . . . . . . . . . . . . . . . . . . . –5 V to +5 V
Analog Input Voltage to AGND . . . . –0.3 V to AVDD +0.3 V
Reference Input Voltage to AGND . . –0.3 V to AVDD +0.3 V
Total AIN/REFIN Current (Indefinite) . . . . . . . . . . . . 30 mA
Digital Input Voltage to DGND . . . . –0.3 V to DVDD +0.3 V
Digital Output Voltage to DGND . . . –0.3 V to DVDD +0.3 V
Operating Temperature Range . . . . . . . . . . . –40°C to +85°C
Storage Temperature Range . . . . . . . . . . . . –65°C to +150°C
Junction Temperature . . . . . . . . . . . . . . . . . . . . . . . . . . 150°C
SOIC Package
θJA Thermal Impedance . . . . . . . . . . . . . . . . . . . . 71.4°C/W
θJC Thermal Impedance . . . . . . . . . . . . . . . . . . . . . . 23°C/W
TSSOP Package
θJA Thermal Impedance . . . . . . . . . . . . . . . . . . . . 97.9°C/W
θJC Thermal Impedance . . . . . . . . . . . . . . . . . . . . . . 14°C/W
Lead Temperature, Soldering
Vapor Phase (60 sec) . . . . . . . . . . . . . . . . . . . . . . . . . 215°C
Infrared (15 sec) . . . . . . . . . . . . . . . . . . . . . . . . . . . . . 220°C
NOTES
1 Stresses above those listed under Absolute Maximum Ratings may cause permanent
damage to the device. This is a stress rating only; functional operation of the
device at these or any other conditions above those listed in the operational
sections of this specification is not implied. Exposure to absolute maximum rating
conditions for extended periods may affect device reliability.
2 AGND and DGND are connected internally within the AD7719.
ORDERING GUIDE
Model Temperature Range Package Description Package Option
AD7719BR –40°C to +85°C SOIC R-28
AD7719BRU –40°C to +85°C TSSOP RU-28
EVAL-AD7719EB Evaluation Board
REV. A –7–
AD7719
TIMING CHARACTERISTICS1, 2
Limit at TMIN, TMAX
Parameter (B Version) Unit Conditions/Comments
t1 32.768 kHz typ Crystal Oscillator Frequency
t2 50 ns min RESET Pulsewidth
Read Operation
t3 0 ns min RDY to CS Setup Time
t4 0 ns min CS Falling Edge to SCLK Active Edge Setup Time3
t5
4 0 ns min SCLK Active Edge to Data Valid Delay3
60 ns max DVDD = 4.75 V to 5.25 V
80 ns max DVDD = 2.7 V to 3.6 V
t5A
4, 5 0 ns min CS Falling Edge to Data Valid Delay3
60 ns max DVDD = 4.75 V to 5.25 V
80 ns max DVDD = 2.7 V to 3.6 V
t6 100 ns min SCLK High Pulsewidth
t7 100 ns min SCLK Low Pulsewidth
t8 0 ns min CS Rising Edge to SCLK Inactive Edge Hold Time3
t9
6 10 ns min Bus Relinquish Time after SCLK Inactive Edge3
80 ns max
t10 100 ns max SCLK Active Edge to RDY High3, 7
Write Operation
t11 0 ns min CS Falling Edge to SCLK Active Edge Setup Time3
t12 30 ns min Data Valid to SCLK Edge Setup Time
t13 25 ns min Data Valid to SCLK Edge Hold Time
t14 100 ns min SCLK High Pulsewidth
t15 100 ns min SCLK Low Pulsewidth
t16 0 ns min CS Rising Edge to SCLK Edge Hold Time
NOTES
1Sample tested during initial release to ensure compliance. All input signals are specified with t R = tF = 5 ns (10% to 90% of DVDD) and timed from a voltage level of 1.6 V.
2See Figures 2 and 3.
3SCLK active edge is falling edge of SCLK.
4These numbers are measured with the load circuit of Figure 1 and defined as the time required for the output to cross the VOL or VOH limits.
5This specification only comes into play if CS goes low while SCLK is low. It is required primarily for interfacing to DSP machines.
6These numbers are derived from the measured time taken by the data output to change 0.5 V when loaded with the circuit of Figure 1. The measured number is then
extrapolated back to remove effects of charging or discharging the 50 pF capacitor. This means that the times quoted in the Timing Characteristics are the true bus
relinquish times of the part and as such are independent of external bus loading capacitances.
7RDY returns high after a read of both ADCs. The same data can be read again, if required, while RDY is high, although care should be taken that subsequent reads
do not occur close to the next output update.
Specifications subject to change without notice.
(AVDD = 2.7 V to 3.6 V or AVDD = 4.75 V to 5.25 V; DVDD = 2.7 V to 3.6 V or DVDD = 4.75 V to 5.25 V;
AGND = DGND = 0 V; XTAL = 32.768 kHz; Input Logic 0 = 0 V, Logic 1 = DVDD, unless otherwise noted.)
ISINK (1.6mA WITH DVDD = 5V
100A WITH DVDD = 3V)
1.6V
ISOURCE (200A WITH DVDD = 5V
100A WITH DVDD = 3V)
TO OUTPUT
PIN
50pF
Figure 1. Load Circuit for Timing Characterization
CAUTION
ESD (electrostatic discharge) sensitive device. Electrostatic charges as high as 4000 V readily
accumulate on the human body and test equipment and can discharge without detection. Although
the AD7719 features proprietary ESD protection circuitry, permanent damage may occur on
devices subjected to high energy electrostatic discharges. Therefore, proper ESD precautions are
recommended to avoid performance degradation or loss of functionality.
WARNING!
ESD SENSITIVE DEVICE
REV. A
AD7719
–8–
DIGITAL INTERFACE
As previously outlined, the AD7719’s programmable functions are
controlled using a set of on-chip registers. Data is written to these
registers via the part’s serial interface; read access to the on-chip
registers is also provided by this interface. All communications to
the part must start with a write operation to the Communications
register. After power-on or RESET, the device expects a write to
its Communications register. The data written to this register
determines whether the next operation to the part is a read or a
write operation and also determines to which register this read
or write operation occurs. Therefore, write access to any of the
other registers on the part starts with a write operation to the
Communications register followed by a write to the selected
register. A read operation from any other register on the part
(including the output data register) starts with a write operation
to the Communications register followed by a read operation
from the selected register.
The AD7719’s serial interface consists of five signals: CS, SCLK,
DIN, DOUT, and RDY. The DIN line is used for transferring
data into the on-chip registers while the DOUT line is used for
accessing data from the on-chip registers. SCLK is the serial
clock input for the device, and all data transfers (either on DIN
or DOUT) take place with respect to this SCLK signal. The
RDY line is used as a status signal to indicate when data is ready
to be read from the AD7719’s data register. RDY goes low when a
new data-word is available in the output register of either the
main or aux ADCs. It is reset high when a read operation from
the data register is complete. It also goes high prior to the updating
of the output register to indicate when not to read from the device
to ensure that a data read is not attempted while the register is
being updated. CS is used to select the device. It can be used to
decode the AD7719 in systems where a number of parts are
connected to the serial bus.
Figures 2 and 3 show timing diagrams for interfacing to the
AD7719 with CS used to decode the part. Figure 3 is for a read
operation from the AD7719’s output shift register while Figure 2
shows a write operation to the input shift register. It is possible
to read the same data twice from the output register even though
the RDY line returns high after the first read operation. Care must
be taken, however, to ensure that the read operations have been
completed before the next output update is about to take place.
The AD7719 serial interface can operate in 3-wire mode by tying
the CS input low. In this case, the SCLK, DIN, and DOUT lines
are used to communicate with the AD7719, and the status of
RDY bits (RDY0 and RDY1) can be obtained by interrogating
the STATUS register. This scheme is suitable for interfacing to
microcontrollers. If CS is required as a decoding signal, it can
be generated from a port bit. For microcontroller interfaces, it is
recommended that the SCLK idles high between data transfers.
The AD7719 can also be operated with CS used as a frame synchronization
signal. This scheme is suitable for DSP interfaces.
In this case, the first bit (MSB) is effectively clocked out by CS
since CS would normally occur after the falling edge of SCLK
in DSPs. The SCLK can continue to run between data transfers
provided the timing numbers are obeyed.
t12
t13
t14
t15
t11 t16
MSB LSB
CS
SCLK
DIN
Figure 2. Write Cycle Timing Diagram
t5
t5A
t4 t6
t3
t9
MSB LSB
CS
SCLK
t8
t10
t7 t6
DOUT
RDY
Figure 3. Read Cycle Timing Diagram
REV. A
AD7719
–9–
PIN CONFIGURATION
14
13
12
11
10
17
16
15
19
18
20
28
27
26
25
24
23
22
21
9
8
1
2
3
4
7
6
5
TOP VIEW
(Not to Scale)
AD7719
IOUT1
DGND
DVDD
XTAL2
XTAL1
IOUT2
AVDD
AGND
RDY
DOUT
REFIN1(–) DIN
REFIN1(+)
AIN1
AIN2
AIN3
AIN4 RESET
SCLK
CS
AIN5
AIN6
REFIN2
P4
P1/SW1
P3
P2/SW2
PWRGND
PIN FUNCTION DESCRIPTIONS
Pin No. Mnemonic Function
1 IOUT1 Output for Internal 200 μA Excitation Current Source. Current source IEXC1 and/or IEXC2 can be
switched to this output.
2 IOUT2 Output for Internal 200 μA Excitation Current Source. Current source IEXC1 and/or IEXC2 can be
switched to this output.
3 AVDD Analog Supply Voltage.
4 AGND Analog Ground.
5 REFIN1(–) Negative Reference Input for Main ADC Channel. This reference input can lie anywhere between AGND
and AVDD – 1 V.
6 REFIN1(+) Positive Reference Input for Main ADC Channel. REFIN1(+) can lie anywhere between AVDD and
AGND + 1 V. The nominal reference voltage (REFIN1(+) – REFIN1(–)) is 2.5 V, but the part is functional
with a reference range from 1 V to AVDD.
7 AIN1 Analog Input. AIN1 is dedicated to the main channel.
8 AIN2 Analog Input. AIN2 is dedicated to the main channel.
9 AIN3 Analog Input. AIN3 can be multiplexed to either the main or auxiliary channel.
10 AIN4 Analog Input. AIN4 can be multiplexed to either the main or auxiliary channel.
11 AIN5 Analog Input. AIN5 is dedicated to the auxiliary channel and is referenced to AIN6 or AGND.
12 AIN6 Analog Input. AIN6 is dedicated to the auxiliary channel. It forms a differential input pair with AIN5 in
fully differential input mode or is referenced to AGND in pseudodifferential mode.
13 REFIN2 Single-Ended Reference Input for Auxiliary Channel. The nominal input reference is 2.5 V. The auxiliary
channel will function with an input reference range from 1 V to AVDD.
14 P4 General-Purpose I/O Bit. The input and output voltage levels are referenced to AVDD and AGND.
15 P3 General-Purpose I/O Bit. The input and output voltage levels are referenced to AVDD and AGND.
The serial interface can be reset by exercising the RESET input
on the part. It can also be reset by writing a series of 1s on the
DIN input. If a logic 1 is written to the AD7719 DIN line for at
least 32 serial clock cycles, the serial interface is reset. This ensures
that in 3-wire systems, if the interface gets lost, either via a software
error or by some glitch in the system, it can be reset back to a
known state. This state returns the interface to where the AD7719
is expecting a write operation to its Communications register. This
operation resets the contents of all registers to their power-on
reset values.
Some microprocessor or microcontroller serial interfaces have a
single serial data line. In this case, it is possible to connect the
AD7719’s DATA OUT and DATA IN lines together and connect
them to the single data line of the processor. A 10 kΩ pull-up
resistor should be used on this single data line. In this case, if the
interface gets lost, because the read and write operations share
the same line, the procedure to reset it to a known state is
somewhat different than previously described. It requires a read
operation of 24 serial clocks followed by a write operation where
a logic 1 is written for at least 32 serial clock cycles to ensure
that the serial interface is back in a known state.
REV. A
AD7719
–10–
PIN FUNCTION DESCRIPTIONS (continued)
Pin No. Mnemonic Function
16 P2/SW2 Dual-Purpose Pin. It can act as a general-purpose output (P2) bit referenced between AVDD and AGND
or as a low-side power switch (SW2) to PWRGND.
17 PWRGND Ground Point for the Low-Side Power Switches SW2 and SW1. PWRGND must be tied to AGND.
18 P1/SW1 Dual-Purpose Pin. It can act as a general-purpose output (P1) bit referenced between AVDD and AGND
or as a low-side power switch (SW1) to PWRGND.
19 RESET Digital Input Used to Reset the ADC to Its Power-On Reset Status. This pin has a weak pull-up internally
to DVDD.
20 SCLK Serial Clock Input for Data Transfers to and from the ADC. The SCLK has a Schmitt-triggered input,
making the interface suitable for opto-isolated applications. The serial clock can be continuous with all
data transmitted in a continuous train of pulses. Alternatively, it can be a noncontinuous clock with the
information being transmitted to or from the AD7719 in smaller batches of data. A weak pull-up to DVDD
is provided on the SCLK input.
21 CS Chip Select Input. This is an active low logic input used to select the AD7719. CS can be used to select
the AD7719 in systems with more than one device on the serial bus or as a frame synchronization signal in
communicating with the device. CS can be hardwired low, allowing the AD7719 to be operated in 3-wire
mode with SCLK, DIN, and DOUT used to interface with the device. A weak pull-up to DVDD is provided
on the CS input.
22 RDY RDY is a logic low status output from the AD7719. RDY is low if either the main ADC or auxiliary ADC
channel has valid data in its data register. This output returns high on completion of a read operation
from the data register. If data is not read, RDY will return high prior to the next update, indicating to the
user that a read operation should not be initiated. The RDY pin also returns low following the completion
of a calibration cycle. The RDY pin is effectively the digital NOR function of the RDY0 and RDY1 bits in
the Status register. If one of the ADCs is disabled, the RDY pin reflects the active ADC. RDY does not
return high after a calibration until the mode bits are written to, enabling a new conversion or calibration.
Since the RDY pin provides information on both the main and aux ADCs, when either the main or aux
ADC is disabled, it is recommended to immediately read its data register to ensure that its RDY bit goes
inactive and releases the RDY pin to indicate output data updates on the remaining active ADC.
23 DOUT Serial Data Output Accessing the Output Shift Register of the AD7719. The output shift register can
contain data from any of the on-chip data, calibration, or control registers.
24 DIN Serial Data Input Accessing the Input Shift Register on the AD7719. Data in this shift register is transferred
to the calibration or control registers within the ADC depending on the selection bits of the Communications
register. A weak pull-up to DVDD is provided on the DIN input.
25 DGND Ground Reference Point for the Digital Circuitry.
26 DVDD Digital Supply Voltage, 3 V or 5 V Nominal.
27 XTAL2 Output from the 32 kHz Crystal Oscillator Inverter.
28 XTAL1 Input to the 32 kHz Crystal Oscillator Inverter.
REV. A
AD7719
–11–
Typical Performance Characteristics–
READING NO.
8389600
8389400
8388000
0 100 1000
CODE READ
200 300
8389200
400 500 600 700 800 900
8389000
8388800
8388600
8388400
8388200
AVDD = DVDD = 5V
INPUT RANGE = 20mV
REFIN1(+)–REFIN1(–) = 2.5V
UPDATE RATE = 19.79Hz
MAIN ADC IN BUFFERED MODE
RMS NOISE = 0.58V rms
TA = 25C
VREF = 2.5V
TPC 1. Typical Noise Plot on ±20 mV Input Range with
19.79 Hz Update Rate
8
7
0
8388039
8388721
8388687
8388657
8388615
8388579
8388547
8388499
8388449
8388382
8388754
8389110
8389033
8388985
8388941
8388906
8388874
8388841
8388805
8388779
6
5
4
3
2
1
9
TPC 2. Noise Distribution Histogram
2.5
0
1.0 1.5 2.0 2.5 3.0 3.5 4.0 4.5 5.0
2.0
1.5
1.0
0.5
3.0
VREF (V)
RMS NOISE (V)
20mV RANGE
2.56V RANGE
AVDD = DVDD = 5V
VREF = 2.5V
INPUT RANGE = 2.56V
UPDATE RATE = 19.79Hz
TA = 25C
TPC 3. RMS Noise vs. Reference Input
16
0 10 20 30 40 50 60 70 80 90 100
24
22
20
18
26
UPDATE RATE (Hz)
NO MISSING CODES (Min)
110
TPC 4. No-Missing-Codes Performance
TEMPERATURE SENSOR ( C)
1400
1200
0
10 20 30 40 50
HITS
800
600
400
200
1000
THE AMBIENT
TEMPERATURE VARIES
FROM 25C TO 30C
WHILE RECORDING
THE DATA FROM
THE DEVICES.
TPC 5. Temperature Sensor Accuracy
MAIN CAL ACC. @ 4V (V)
1200
0
–20 –10 0 10 20
HITS
800
600
400
200
1000
–30
TPC 6. Full-Scale Error Distribution
REV. A
AD7719
–12–
DUAL-CHANNEL ADC CIRCUIT INFORMATION
Overview
The AD7719 incorporates two independent Σ-Δ ADC channels
(main and auxiliary) with on-chip digital filtering intended for
the measurement of wide dynamic range, low frequency signals
such as those in weigh-scale, strain gage, pressure transducer, or
temperature measurement applications.
Main Channel
This channel is intended to convert the primary sensor input.
This channel can be operated in buffered or unbuffered mode, and
can be programmed to have one of eight input voltage ranges
from ±20 mV to ±2.56 V. This channel can be configured as
either two fully differential inputs (AIN1/AIN2 and AIN3/AIN4)
or three pseudodifferential input channels (AIN1/AIN4, AIN2/
AIN4, and AIN3/AIN4). Buffering the input channel means that
the part can accommodate significant source impedances on the
analog input and that R, C filtering (for noise rejection or RFI
reduction) can be placed on the analog inputs if required. Operating
in unbuffered mode leads to lower power consumption in low
power applications, but care must be exercised in unbuffered mode
because source impedances can introduce gain errors. The main
ADC also features sensor burnout currents that can be switched
on and off. These currents can be used to check that a transducer
is still operational before attempting to take measurements.
The ADC employs a Σ-Δ conversion technique to realize up to
24 bits of no-missing-codes performance. The Σ-Δ modulator
converts the sampled input signal into a digital pulse train whose
duty cycle contains the digital information. A Sinc3 programmable
low-pass filter is then employed to decimate the modulator output
data stream to give a valid data conversion result at programmable
output rates from 5.35 Hz (186.77 ms) to 105.03 Hz
(9.52 ms). A chopping scheme is also employed to minimize
ADC channel offset errors. A block diagram of the main ADC
input channel is shown in Figure 4. The sampling frequency of
the modulator loop is many times higher than the bandwidth of
the input signal. The integrator in the modulator shapes the
quantization noise (which results from the analog-to-digital
conversion) so that the noise is pushed toward one-half of the
modulator frequency. The output of the Σ-Δ modulator feeds
directly into the digital filter. The digital filter then band-limits
the response to a frequency significantly lower than one-half of
the modulator frequency. In this manner, the 1-bit output of the
comparator is translated into a band-limited, low noise output
from the AD7719 ADC. The AD7719 filter is a low-pass, Sinc3,
or (SIN(x)/x)3 filter whose primary function is to remove the
quantization noise introduced at the modulator. The cutoff
frequency and decimated output data rate of the filter are programmable
via the SF word loaded to the filter register.
A chopping scheme is employed where the complete signal chain is
chopped, resulting in excellent dc offset and offset drift specifications,
and is extremely beneficial in applications where drift, noise
rejection, and optimum EMI rejection are important factors.
With chopping, the ADC repeatedly reverses its inputs. The
decimated digital output words from the Sinc3 filters therefore
have a positive offset and negative offset term included. As a result,
a final summing stage is included so that each output word from
the filter is summed and averaged with the previous filter output
to produce a new valid output result to be written to the ADC
data register.
Auxiliary Channel
The Auxiliary (Aux) channel is intended to convert supplementary
inputs such as those from a cold junction diode or thermistor.
This channel is unbuffered and has an input range of ±REFIN2
or ±REFIN2/2, determined by the ARN bit in the auxiliary ADC
control register (AD1CON). AIN3 and AIN4 can be multiplexed
into the auxiliary channel as single-ended inputs with respect to
AGND, while AIN5 and AIN6 can operate as a differential
input pair. With AIN6 tied to AGND, AIN5 can be operated as
an additional single-ended input. A block diagram of the auxiliary
ADC channel is shown in Figure 5.
SINC3 FILTER
MUX BUF PGA
MOD0
ANALOG XOR
INPUT
DIGITAL
OUTPUT
1
8 SF
3 ( ) 3 (8 SF )
12
AIN + VOS
AIN – VOS
fCHOP fIN fMOD fCHOP fADC
-
Figure 4. Main ADC Channel Block Diagram
OSCILLATOR
AVDD = DVDD = 5V
TA = 25C
TIME BASE = 100ms/DIV
TRACE 1 = TRACE 2 = 2V/DIV
VDD
TPC 7. Typical Oscillator Power-Up
REV. A
AD7719
–13–
Both Channels
The operation of the aux channel is identical to the main
channel with the exception that there is no PGA on the aux
channel. The input chopping is incorporated into the input
multiplexer while the output chopping is accomplished by an
XOR gate at the output of the modulator. The chopped modulator
bit stream is applied to a Sinc3 filter. The programming of the
Sinc3 decimation factor is restricted to an 8-bit register SF; the
actual decimation factor is the register value times 8. The
decimated output rate from the Sinc3 filter (and the ADC
conversion rate) will therefore be
f
SF
ADC = × fMOD
×
×
1
3
1
8
where:
fADC is the ADC update rate.
SF is the decimal equivalent of the word loaded to the
filter register.
fMOD is the modulator sampling rate of 32.768 kHz.
Programming the filter register determines the update rate for
both the main and aux ADC. Both ADCs operate with the same
update rate.
The chop rate of the channel is half the output data rate.
The frequency response of the filter H (f) is as follows:
1
8
8 1
2
2
3
SF
SF f f
f f
f f
f f
MOD
MOD
OUT
× OUT
× × × ×
×
× × × ×
×
sin ( / )
sin ( / )
sin ( / )
sin ( / )
π
π
π
π
where:
fMOD = 32,768 Hz
SF = value programmed into SF SFR.
fOUT = fMOD/(SF × 8 × 3)
The following shows plots of the filter frequency response for
the SF words shown in Table I. The overall frequency response
is the product of a Sinc3 and a sinc response. There are Sinc3
notches at integer multiples of 3 × fADC and there are sinc notches
SINC3 FILTER
MUX
-
MOD1
ANALOG XOR
INPUT
DIGITAL
OUTPUT
3 (8 SF )
12
AIN + VOS
AIN – VOS
fCHOP fMOD fCHOP fADC
1
8 SF
3 ( )
Figure 5. Auxiliary ADC Channel Block Diagram
at odd integer multiples of fADC/2. The 3 dB frequency for all
values of SF obeys the following equation:
f (3 dB) = 0.24 × fADC
The signal chain is chopped as shown in Figures 4 and 5. The
chop frequency is
f
f
CHOP
= ADC
2
As shown in the block diagram, the Sinc3 filter outputs alternately
contain +VOS and –VOS, where VOS is the respective channel
offset. This offset is removed by performing a running average
of 2. This average by 2 means that the settling time to any change
in programming of the ADC will be twice the normal conversion
time, while an asynchronous step change on the analog input will
not be fully reflected until the third subsequent output.
t
f
SETTLE t
ADC
= ADC
= × 2
2
The allowable range for SF is 13 to 255, with a default of 69
(0x45). The corresponding conversion rates, conversion times,
and settling times are tabulated in Table I. Note that the conversion
time increases by 0.732 ms for each increment in SF.
Table I. ADC Conversion and Settling Times for Various
SF Words
SF Data Update Rate Settling Time
Word fADC (Hz) tSETTLE (ms)
13 105.3 19.04
69 (Default) 19.79 101.07
255 5.35 373.54
Normal mode rejection is the major function of the digital filter
on the AD7719. The normal mode 50 Hz ± 1 Hz rejection with an
SF word of 82 is typically –100 dB. The 60 Hz ± 1 Hz rejection
with SF = 68 is typically –100 dB. Simultaneous 50 Hz and
60 Hz rejection of better than 60 dB is achieved with an SF of
69. Choosing an SF word of 69 places notches at both 50 Hz
and 60 Hz. Figures 6 to 9 show the filter rejection for a selection
of SF words.
REV. A
AD7719
–14–
MAIN AND AUXILIARY ADC NOISE PERFORMANCE
Tables II to VII show the output rms noise and output peak-topeak
resolution in bits (rounded to the nearest 0.5 LSB) for a
selection of output update rates on both the main and auxiliary
ADCs. The numbers are typical and are generated at a differential
input voltage of 0 V. The output update rate is selected via the
SF7 to SF0 bits in the Filter register. It is important to note that
the peak-to-peak resolution figures represent the resolution for
which there will be no code flicker within a six-sigma limit. The
output noise comes from two sources. The first is the electrical
noise in the semiconductor devices (device noise) used in the
implementation of the modulator. Secondly, when the analog
input is converted into the digital domain, quantization noise is
added. The device noise is at a low level and is independent of
frequency. The quantization noise starts at an even lower level but
rises rapidly with increasing frequency to become the dominant
noise source. The numbers in the tables are given for the bipolar
input ranges. For the unipolar ranges, the rms noise numbers
will be the same as the bipolar range, but the peak-to-peak
resolution is now based on half the signal range, which effectively
means losing one bit of resolution.
FREQUENCY (Hz)
0
–140
–200
0 50 100 150 200 250 300 350 400 450 500 550 600 650 700
ATTENUATION (dB)
–20
–120
–160
–180
–60
–100
–40
–80
SF = 13
OUTPUT DATA RATE = 105Hz
INPUT BANDWIDTH = 25.2Hz
FIRST NOTCH = 52.5Hz
50Hz REJECTION = –23.6dB, 50Hz 1Hz REJECTION = –20.5dB
60Hz REJECTION = –14.6dB, 60Hz 1Hz REJECTION = –13.6dB
Figure 6. Filter Profile with SF = 13
FREQUENCY (Hz)
0
–80
–160
0 10 100
ATTENUATION (dB)
20 30 40 50 60 70 80 90
–20
–40
–120
–140
–60
–100
SF = 82
OUTPUT DATA RATE = 16.65Hz
INPUT BANDWIDTH = 4Hz
50Hz REJECTION = –171dB, 50Hz 1Hz REJECTION = –100dB
60Hz REJECTION = –58dB, 60Hz 1Hz REJECTION = –53dB
Figure 7. Filter Profile with SF = 82
FREQUENCY (Hz)
0
–80
–160
0 10 100
ATTENUATION (dB)
20 30 40 50 60 70 80 90
–20
–40
–120
–140
–60
–100
SF = 69
OUTPUT DATA RATE = 19.8Hz
INPUT BANDWIDTH = 4.74Hz
FIRST NOTCH = 9.9Hz
50Hz REJECTION = –66dB, 50Hz 1Hz REJECTION = –60dB
60Hz REJECTION = –117dB, 60Hz 1Hz REJECTION = –94dB
Figure 8. Filter Profile with Default SF = 69 Giving Filter
Notches at Both 50 Hz and 60 Hz
FREQUENCY (Hz)
0
–80
–160
0 10 100
ATTENUATION (dB)
20 30 40 50 60 70 80 90
–20
–40
–120
–140
–60
–100
SF = 255
OUTPUT DATA RATE = 5.35Hz
INPUT BANDWIDTH = 1.28Hz
50Hz REJECTION = –93dB, 50Hz 1Hz REJECTION = –93dB
60Hz REJECTION = –74dB, 60Hz 1Hz REJECTION = –68dB
Figure 9. Filter Profile with SF = 255
REV. A
AD7719
–15–
Table II. Typical Output RMS Noise vs. Input Range and Update Rate for Main ADC (Buffered Mode) Output RMS
Noise in V
SF Data Update Input Range
Word Rate (Hz) 20 mV 40 mV 80 mV 160 mV 320 mV 640 mV 1.28 V 2.56 V
13 105.3 1.50 1.50 1.60 1.75 3.50 4.50 6.70 11.75
69 19.79 0.60 0.65 0.65 0.65 0.65 0.95 1.40 2.30
255 5.35 0.35 0.35 0.37 0.37 0.37 0.51 0.82 1.25
Table III. Peak-to-Peak Resolution vs. Input Range and Update Rate for Main ADC (Buffered Mode) Peak-to-Peak
Resolution in Bits
SF Data Update Input Range
Word Rate (Hz) 20 mV 40 mV 80 mV 160 mV 320 mV 640 mV 1.28 V 2.56 V
13 105.3 12 13 14 15 15 15.5 16 16
69 19.79 13 14 15 16 17 17.5 18 18.5
255 5.35 14 15 16 17 18 18.5 18.8 19.2
Table IV. Typical Output RMS Noise vs. Input Range and Update Rate for Main ADC (Unbuffered Mode) Output
RMS Noise in V
SF Data Update Input Range
Word Rate (Hz) 20 mV 40 mV 80 mV 160 mV 320 mV 640 mV 1.28 V 2.56 V
13 105.3 1.27 1.27 1.35 1.48 2.95 3.82 5.69 10.2
69 19.79 0.52 0.56 0.56 0.56 0.56 0.82 1.21 2.00
255 5.35 0.30 0.30 0.32 0.32 0.32 0.44 0.71 1.10
Table V. Peak-to-Peak Resolution vs. Input Range and Update Rate for Main ADC (Unbuffered Mode) Peak-to-Peak
Resolution in Bits
SF Data Update Input Range
Word Rate (Hz) 20 mV 40 mV 80 mV 160 mV 320 mV 640 mV 1.28 V 2.56 V
13 105.3 12 13 14 15 15 15.5 16 16
69 19.79 13 14 15 16 17 17.5 18 18.5
255 5.35 14 15 16 17 18 18.5 19 19.5
Table VI. Typical Output RMS Noise vs. Update
Rate for Auxiliary ADC (Unbuffered Mode)
SF Data Update Input Range
Word Rate (Hz) 2.5 V
13 105.3 10.75 μV
69 19.79 2.00 μV
255 5.35 1.15 μV
Table VII. Peak-to-Peak Resolution vs. Update
Rate for Auxiliary ADC (Unbuffered Mode)
SF Data Update Input Range
Word Rate (Hz) 2.5 V
13 105.3 16 Bits
69 19.79 16 Bits
255 5.35 16 Bits
REV. A
AD7719
–16–
DOUT FILTER REGISTER
DIN
DOUT TEST REGISTER
DIN
DOUT ID REGISTER
DOUT AUX ADC GAIN REGISTER
DIN
ADC STATUS REGISTER
DOUT
DOUT MODE REGISTER
DIN
DOUT MAIN ADC CONTROL REGISTER
DIN
DOUT AUX ADC CONTROL REGISTER
DIN
DOUT I/O CONTROL REGISTER
DIN
DOUT MAIN ADC GAIN REGISTER
DIN
DOUT AUX ADC OFFSET REGISTER
DIN
DOUT MAIN ADC OFFSET REGISTER
DIN
DOUT AUX ADC DATA REGISTER
DOUT MAIN ADC DATA REGISTER
WEN R/W 0 0 A3 A2 A1 A0
COMMUNICATIONS REGISTER
DOUT
DIN
REGISTER
SELECT
DECODER
Figure 10. On-Chip Registers
ON-CHIP REGISTERS
Both the main and auxiliary ADC channels are controlled and configured
via a number of on-chip registers as shown in Figure 10 and
described in more detail in the following pages. In the following
descriptions, SET implies a logic 1 state and CLEARED implies
a logic 0 state, unless otherwise stated.
REV. A
AD7719
–17–
Table VIII. Registers—Quick Reference Guide
Power-On/Reset
Register Name Type Size Default Value Function
Communications Write Only 8 Bits Not Applicable All operations to other registers are initiated through
the Communications register. This controls whether
subsequent operations are read or write operations
and also selects the register for that subsequent
operation.
Status Register Read Only 8 Bits 0x00 Provides status information on conversions, calibrations,
error conditions, and the validity of the
reference voltage.
Mode Register Read/Write 8 Bits 0x00 Controls functions such as mode of operation, channel
configuration, and oscillator operation in power-down.
Main ADC (AD0CON)
Control Register Read/Write 8 Bits 0x07 This register is used to enable the main ADC and to
configure the main ADC for range, channel selection,
16-/24-bit operation, and unipolar or bipolar operation.
Aux ADC (AD1CON)
Control Register Read/Write 8 Bits 0x01 This register is used to enable the aux ADC and to
configure the Aux ADC for range, channel selection,
unipolar or bipolar operation, and input range.
I/O (IOCON)
Control Register Read/Write 16 Bits 0x0000 This register is used to control and configure the
various excitation and burnout current source options
available on-chip along with controlling the I/O port.
Filter Register Read/Write 8 Bits 0x45 This register determines the amount of averaging
performed by the sinc filter and consequently determines
the data update rate of the AD7719. The filter
register determines the update rate for both the main
and aux ADCs.
MSB LSB
RDY0 RDY1 CAL NOREF ERR0 ERR1 0 LOCK
MSB LSB
SF7 SF6 SF5 SF4 SF3 SF2 SF1 SF0
MSB
PSW2 PSW1 0 BO I2PIN I1PIN I2EN I1EN
LSB
P4DIR P3DIR P2EN P1EN P4DAT P3DAT P2DAT P1DAT
MSB LSB
WEN R/W 0 0 A3 A2 A1 A0
MSB LSB
0 BUF 0 CHCON OSCPD MD2 MD1 MD0
MSB LSB
AD0EN WL CH1 CH0 U/B RN2 RN1 RN0
MSB LSB
AD1EN ACH2 ACH1 ACH0 U/B 0 0 ARN
REV. A
AD7719
–18–
Power-On/Reset
Register Name Type Size Default Value Function
Main ADC (DATA0)
Data Register Read Only 16 Bits or 24 Bits 0x00 0000 Provides the most up-to-date conversion result from
the main ADC. Main ADC data register length
can be programmed to be 16-bit or 24-bit.
Aux ADC (DATA1)
Data Register Read Only 16 Bits 0x0000 Provides the most up-to-date conversion result from
the auxiliary ADC. Aux ADC data register length is
16 bits.
Main ADC
Offset Register Read/Write 24 Bits 0x80 0000 Contains a 24-bit word that is the offset calibration
coefficient for the part. The contents of this register
are used to provide offset correction on the output
from the digital filter. There are three offset registers
on the part and these are associated with input channel
pairs as outlined in the AD0CON register.
Main ADC
Gain Register Read/Write 24 Bits 0x5X XXX5 Contains a 24-bit word that is the gain calibration
coefficient for the part. The contents of this register
are used to provide gain correction on the output
from the digital filter. There are three Gain registers
on the part, which are associated with input channel
pairs as outlined in the AD0CON register.
Aux ADC
Offset Register Read/Write 16 Bits 0x8000 Contains a 16-bit word that is the offset calibration
coefficient for the part. The contents of this register
are used to provide offset correction on the output
from the digital filter.
Aux ADC
Gain Register Read/Write 24 Bits 0x59XX Contains a 16-bit word that is the gain calibration
coefficient for the part. The contents of this register
are used to provide gain correction on the output
from the digital filter.
ID Register Read 8 Bits 0x0X Contains an 8-bit byte that is the identifier for the part.
Test Registers Read/Write 16 Bits 0x0000 Controls the test modes of the part, which are used
when testing the part. The user is advised not to
change the contents of these registers.
REV. A
AD7719
–19–
CR7 CR6 CR5 CR4 CR3 CR2 CR1 CR0
WEN (0) R/W (0) 0 (0) 0 (0) A3 (0) A2 (0) A1 (0) A0 (0)
Table IX. Communications Register Bit Designations
Bit Bit
Location Name Description
CR7 WEN Write Enable Bit. A 0 must be written to this bit so the write operation to the Communications register
actually takes place. If a 1 is written to this bit, the part will not clock on to subsequent bits in the register.
It will stay at this bit location until a 0 is written to this bit. Once a 0 is written to the WEN bit, the next
seven bits will be loaded to the Communications register.
CR6 R/W A 0 in this bit location indicates that the next operation will be a write to a specified register. A 1 in this
position indicates that the next operation will be a read from the designated register.
CR5 Zero A 0 must be written to this bit position to ensure correct operation of the AD7719.
CR4 Zero A 0 must be written to this bit position to ensure correct operation of the AD7719.
CR3–CR0 A3–A0 Register Address Bits. These address bits are used to select which of the AD7719’s registers is being
accessed during this serial interface communication. A3 is the MSB of the three selection bits.
Communications Register (A3, A2, A1, A0 = 0, 0, 2, 0)
The Communications register is an 8-bit write-only register. All
communications to the part must start with a write operation to
the Communications register. The data written to the Communications
register determines whether the next operation is a read
or write operation, and to which register this operation takes
place. For read or write operations, once the subsequent read or
write operation to the selected register is complete, the interface
returns to where it expects a write operation to the Communications
register. This is the default state of the interface, and on power-up
or after a RESET, the AD7719 is in this default state waiting for a
write operation to the Communications register. In situations where
the interface sequence is lost, a write operation of at least 32 serial
clock cycles with DIN high returns the AD7719 to this default
state by resetting the part. Table IX outlines the bit designations
for the Communications register. CR0 through CR7 indicate the
bit location, with CR denoting that the bits are in the Communications
register. CR7 denotes the first bit of the data stream.
Table X. Register Selection Table
A3 A2 A1 A0 Register
0 0 0 0 Communications Register during a Write Operation
0 0 0 0 Status Register during a Read Operation
0 0 0 1 Mode Register
0 0 1 0 Main ADC Control Register (AD0CON)
0 0 1 1 Aux ADC Control Register (AD1CON)
0 1 0 0 Filter Register
0 1 0 1 Main ADC Data Register
0 1 1 0 Aux ADC Data Register
0 1 1 1 I/O Control Register
1 0 0 0 Main ADC Offset Calibration Register
1 0 0 1 Aux ADC Offset Calibration Register
1 0 1 0 Main ADC Gain Calibration Register
1 0 1 1 Aux ADC Gain Calibration Register
1 1 0 0 Test 1 Register
1 1 0 1 Test 2 Register
1 1 1 0 Undefined
1 1 1 1 ID Register
REV. A
AD7719
–20–
Table XI. Status Register Bit Designations
Bit Bit
Location Name Description
SR7 RDY0 Ready Bit for Main ADC.
Set when data is written to main ADC data registers or on completion of calibration cycle. The RDY0 bit is
cleared automatically after the main ADC data register has been read or after a period of time before the
data register is updated with a new conversion result. This bit is also cleared by a write to the mode bits to
indicate a conversion or calibration.
SR6 RDY1 Ready Bit for Aux ADC.
Set when data is written to aux ADC data registers or on completion of calibration cycle. The RDY1 bit is
cleared automatically after the aux ADC data register has been read or a period of time before the data register
is updated with a new conversion result. This bit is also cleared by a write to the mode bits to indicate a
conversion or calibration.
SR5 CAL Calibration Status Bit.
Set to indicate completion of calibration. It is set at the same time that the RDY0 and/or RDY1 bits
are set high. Cleared by a write to the mode bits to start another ADC conversion or calibration.
SR4 NOXREF No External Reference Bit. (Only active if main ADC is active and applies to REFIN1 only.)
Set to indicate that one or both of the REFIN1 pins is floating or the applied voltage is below a specified
threshold. When Set, conversion results are clamped to all 1s.
Cleared to indicate valid reference applied between REFIN1(+) and REFIN1(–).
SR3 ERR0 Main ADC Error Bit.
Set to indicate that the result written to the main ADC data registers has been clamped to all 0s or all 1s.
After a calibration, this bit also flags error conditions that caused the calibration registers not to be written.
Error sources include Overrange, Underrange, and NOXREF.
Cleared by a write to the mode bits to initiate a conversion or calibration.
SR2 ERR1 Aux ADC Error Bit.
Set to indicate that the result written to the Aux ADC data registers has been clamped to all 0s or all 1s.
After a calibration, this bit also flags error conditions that caused the calibration registers not to be written.
Error sources include Overrange, Underrange, and NOXREF.
Cleared by a write to the mode bits to initiate a conversion or calibration.
SR1 0 Reserved for Future Use.
SR0 LOCK PLL Lock Status Bit.
Set if the PLL has locked onto the 32 kHz crystal oscillator clock. If the user is worried about exact sampling
frequencies, for example, the LOCK bit should be interrogated and the result discarded if the LOCK bit is 0.
Status Register (A3, A2, A1, A0 = 0, 0, 0, 0; Power-On
Reset = 0x00)
The ADC Status register is an 8-bit read-only register. To access
the ADC Status register, the user must write to the Communications
register selecting the next operation to be a read and loading
bits A3 to A0 with 0, 0, 0, 0. Table XI outlines the bit designations
for the Status register. SR0 through SR7 indicate the bit location,
with SR denoting that the bits are in the Status register. SR7
denotes the first bit of the data stream. The number in parentheses
indicates the power-on/reset default status of that bit.
SR7 SR6 SR5 SR4 SR3 SR2 SR1 SR0
RDY0 (0) RDY1 (0) CAL (0) NOXREF (0) ERR0 (0) ERR1 (0) (0) LOCK (0)
REV. A
AD7719
–21–
Mode Register (A3, A2, A1, A0 = 0, 0, 0, 1; Power-On
Reset = 0x00)
The Mode register is an 8-bit register from which data can be
read or to which data can be written. This register configures the
operating modes of the AD7719. Table XII outlines the bit
designations for the Mode register. MR7 through MR0 indicate
the bit location, with MR denoting the bits are in the Mode register.
MR7 denotes the first bit of the data stream. The number in
parentheses indicates the power-on/reset default status of that bit.
Table XII. MODE Register Bit Designations
Bit Bit
Location Name Description
MR7 0 Reserved for Future Use.
MR6 BUF Configures the main ADC for buffered or unbuffered mode of operation. If set, the main ADC operates in
unbuffered mode, lowering the power consumption of the AD7719. If cleared, the Main ADC operates
in buffered mode, allowing the user to place source impedances on the front end without contributing gain
errors to the system.
MR5 0 Reserved for Future Use.
MR4 CHCON Channel Configure Bit.
If this bit is set, the main ADC operates with three pseudodifferential input channels and the aux ADC
does not have AIN3/AIN4 as an input option. If cleared, the main ADC operates with two fully differential
input channels and the aux channel operates as one fully differential input and two single-ended inputs or
as three single-ended inputs.
MR3 OSCPD Oscillator Power-Down Bit.
If this bit is set, placing the AD7719 in standby mode will stop the crystal oscillator, reducing the power
drawn by the AD7719 to a minimum. The oscillator will require 300 ms to begin oscillating when the
ADC is taken out of standby mode. If this bit is cleared, the oscillator is not shut off when the ADC is put
into standby mode and will not require the 300 ms start-up time when the ADC is taken out of standby.
MR2–MR0 MD2–MD0 Main and Aux ADC Mode Bits.
These bits select the operational mode of the enabled ADC as follows:
MD2 MD1 MD0 Description
0 0 0 Power-Down Mode (Power-On Default).
The current sources, power switches, and PLL are shut off in Power-Down mode.
0 0 1 Idle Mode.
In Idle mode, the ADC filter and modulator are held in a reset state although the modulator clocks are still
provided.
0 1 0 Single Conversion Mode.
In Single Conversion mode, a single conversion is performed on the enabled channels. On completion of the
conversion, the ADC data registers are updated, the relevant flags in the STATUS register are written, and
idle mode is entered with the MD2–MD0 being written accordingly to 001.
0 1 1 Continuous Conversion.
In continuous conversion mode, the ADC data registers are regularly updated at the selected update rate
(see Filter register).
1 0 0 Internal Zero-Scale Calibration.
Internal short automatically connected to the enabled channel(s). Returns to Idle mode (001) when complete.
1 0 1 Internal Full-Scale Calibration.
External VREF is connected automatically to the ADC input for this calibration. Returns to idle mode when complete.
1 1 0 System Zero-Scale Calibration.
User should connect system zero-scale input to the channel input pins as selected by the CH1/CH0 and
ACH1/ACH0 bits in the control registers.
1 1 1 System Full-Scale Calibration.
User should connect system full-scale input to the channel input pins as selected by the CH1/CH0 and
ACH1/ACH0 bits in the control registers.
MR7 MR6 MR5 MR4 MR3 MR2 MR1 MR0
0 (0) BUF (0) 0 (0) CHCON (0) OSCPD (0) MD2 (0) MD1 (0) MD0 (0)
REV. A
AD7719
–22–
Operating Characteristics when Addressing the Mode and
Control Registers
1. Any change to the MD bits will immediately reset both ADCs.
A write to the MD2–0 bits with no change is also treated as a
reset. (See exception to this in Note 3.)
2. If AD0CON is written when AD0EN = 1, or if AD0EN is
changed from 0 to 1, both ADCs are also immediately reset. In
other words, the main ADC is given priority over the aux ADC
and any change requested on main is immediately responded to.
3. On the other hand, if AD1CON is written to, only the aux
ADC is reset. For example, if the main ADC is continuously
converting when the aux ADC change or enable occurs, the
main ADC continues undisturbed. Rather than allow the aux
ADC to operate with a phase difference from the main ADC,
the aux ADC will fall into step with the outputs of the main
ADC. The result is that the first conversion time for the aux
channel will be delayed up to three outputs while the aux ADC
update rate is synchronized to the main ADC.
4. Once the MODE has been written with a calibration mode,
the RDY0/1 bits (STATUS) are immediately reset and the
calibration commences. On completion, the appropriate calibration
registers are written, the relevant bits in STATUS are
written, and the MD2–0 bits are reset to 001 to indicate the
ADC is back in Idle mode.
5. Any calibration request of the aux ADC while the temperature
sensor is selected will fail to complete.
6. Calibrations are performed with the maximum allowable SF
value. SF register is reset to user configuration after calibration.
Main ADC Control Register (AD0CON): (A3, A2, A1, A0 = 0,
0, 1, 0; Power-On Reset = 0x07)
The main ADC control register is an 8-bit register from which data
can be read or to which data can be written. This register is used to
configure the main ADC for range, channel selection, 16-/24-bit
operation, and unipolar or bipolar coding. Table XIII outlines
the bit designations for the main ADC control register. AD0CON7
through AD0CON0 indicate the bit location, AD0CON denoting
the bits are in the main ADC control register. AD0CON7 denotes
the first bit of the data stream. The number in parentheses
indicates the power-on/reset default status of that bit.
Table XIII. Main ADC Control Register (AD0CON) Bit Designations
Bit Location Bit Name Description
AD0CON7 AD0EN Main ADC Enable Bit.
Set by user to enable the main ADC. When set, the main ADC operates according to the MD bits in
the mode register.
Cleared by the user to power down the Main ADC.
AD0CON6 WL 16-/24-Bit Operating Mode.
Set by user to enable 16-bit mode. The conversion results from the main ADC will be rounded to
16 bits and the main ADC data register will be 16 bits wide.
Cleared by user to enable 24-bit mode. The conversion results from the main ADC will be rounded to
24 bits and the main ADC data register will be 24 bits wide.
AD0CON5 CH1 Main ADC Channel Selection Bits.
AD0CON4 CH0 Written by the user to select the differential input pairs used by the main ADC as follows:
(Note: The CHCON bit resides in the Mode register.)
CHCON CH1 CH0 Positive Input Negative Input Calibration Register Pair
0 0 0 AIN1 AIN2 0
0 0 1 AIN3 AIN4 1
0 1 0 AIN2 AIN2 0
0 1 1 AIN3 AIN2 1
1 0 0 AIN1 AIN4 0
1 0 1 AIN3 AIN4 1
1 1 0 AIN4 AIN4 0
1 1 1 AIN2 AIN4 2
AD0CON3 U/B Main ADC Unipolar/Bipolar Bit.
Set by user to enable unipolar coding, i.e., zero differential input will result in 0x00 0000 output and
a full-scale differential input will result in 0xFF FFFF output when operated in 24-bit mode.
Cleared by user to enable bipolar coding, Negative full-scale differential input will result in an output
code of 0x00 0000, zero differential input will result in an output code of 0x80 0000, and a
Positive full-scale differential input will result in an output code of 0xFF FFFF.
AD0CON7 AD0CON6 AD0CON5 AD0CON4 AD0CON3 AD0CON2 AD0CON1 AD0CON0
AD0EN (0) WL (0) CH1 (0) CH0 (0) U/B (0) RN2 (1) RN1 (1) RN0 (1)
REV. A
AD7719
–23–
Table XIII. Main ADC Control Register (AD0CON) Bit Designations (continued)
Bit Location Bit Name Description
AD0CON2 RN2 Main ADC Range Bits.
AD0CON1 RN1 Written by the user to select the main ADC input range as follows.
AD0CON0 RN0 RN2 RN1 RN0 Selected Main ADC Input Range (VREF = 2.5 V)
0 0 0 ±20 mV
0 0 1 ±40 mV
0 1 0 ±80 mV
0 1 1 ±160 mV
1 0 0 ±320 mV
1 0 1 ±640 mV
1 1 0 ±1.28 V
1 1 1 ±2.56 V
Aux ADC Control Registers (AD1CON):
(A3, A2, A1, A0 = 0, 0, 1, 1; Power-On Reset = 0x00)
The aux ADC control register is an 8-bit register from which data
can be read or to which data can be written. This register is used
to configure the aux ADC for range, channel selection, and unipolar
or bipolar coding. Table XIV outlines the bit designations
for the aux ADC control register. AD1CON7 through AD1CON0
indicate the bit location, with AD1CON denoting that the bits
are in the aux ADC control register. AD1CON7 denotes the
first bit of the data stream. The number in parentheses indicates
the power-on/reset default status of that bit.
Table XIV. Aux ADC Control Register (AD1CON) Bit Designations
Bit Location Bit Name Description
AD1CON7 AD1EN Aux ADC Enable Bit.
Set by user to enable the Aux ADC. When set, the aux ADC operates according to the MD bits
in the mode register.
Cleared by the user to power down the aux ADC.
AD1CON6 ACH2 Aux ADC Channel Selection Bits.
AD1CON5 ACH1 Written by the user to select the active input channels used by the aux ADC as follows:
AD1CON4 ACH0
CHCON ACH2 ACH1 ACH0 Positive Input Negative Input
0 0 0 0 AIN3 AGND
0 0 0 1 AIN4 AGND
0 0 1 0 AIN5 AIN6
0 0 1 1 Temp Sensor (Temp Sensor Routed to the ADC Inputs)
0 1 0 0 AGND AGND (Internal Short)
1 0 0 0 AIN5 AGND
1 0 0 1 AIN6 AGND
1 0 1 0 AIN5 AIN6
1 0 1 1 Temp Sensor (Temp Sensor Routed to the ADC Inputs)
1 1 0 0 AGND AGND (Internal Short)
X 1 0 1 Not Defined
X 1 1 0 Not Defined
X 1 1 1 Not Defined
AD1CON3 U/B Aux ADC Unipolar/Bipolar Selection Bit.
Set by user to enable unipolar coding, i.e., zero differential input will result in 0x0000 output.
Cleared by user to enable bipolar coding, zero differential input will result in 0x8000 output.
AD1CON2 0 Must be zero for specified operation.
AD1CON1 0 Must be zero for specified operation.
AD1CON0 ARN Auxiliary Channel Input Range Bit.
When set by the user, the input range is ±REFIN2. When cleared by the user, the input range is ±REFIN2/2.
NOTES
1. When the temperature sensor is selected, the AD7719 automatically selects its internal reference. The temperature sensor is not factory calibrated. Temp sensor
is suitable for relative temperature measurements. The temperature sensor yields conversion results where a conversion result of 0x8000 equates to typically 0°C.
2. A 1°C change in temperature will normally result in a 256 LSB change in the AD1 data register (ADC conversion result).
AD1CON7 AD1CON6 AD1CON5 AD1CON4 AD1CON3 AD1CON2 AD1CON1 AD1CON0
AD1EN (0) ACH2 (0) ACH1 (0) ACH0 (0) U/B (0) 0 (0) 0 (0) ARN (1)
REV. A
AD7719
–24–
FR7 FR6 FR5 FR4 FR3 FR2 FR1 FR0
SF7 (0) SF6 (1) SF5 (0) SF4 (0) SF3 (0) SF2 (1) SF1 (0) SF0 (1)
IOCON15 IOCON14 IOCON13 IOCON12 IOCON11 IOCON10 IOCON9 IOCON8
PSW2 (0) PSW1 (0) 0 (0) BO (0) I2PIN (0) I1PIN (1) I2EN (0) I1EN (0)
IOCON7 IOCON6 IOCON5 IOCON4 IOCON3 IOCON2 IOCON1 IOCON0
P4DIR (0) P3DIR (0) P2EN (0) P1EN (0) P4DAT (0) P3DAT (0) P2DAT (0) P1DAT (0)
Filter Register (A3, A2, A1, A0 = 0, 1, 0, 0; Power-On
Reset = 0x45)
The Filter register is an 8-bit register from which data can be
read or to which data can be written. This register determines
the amount of averaging performed by the sinc filter. Table XV
outlines the bit designations for the Filter register. FR7 through
FR0 indicate the bit location, with FR denoting that the bits are in
the Filter register. FR7 denotes the first bit of the data stream.
The number in parentheses indicates the power-on/reset default
status of that bit. The number in this register is used to set the
decimation factor and thus the output update rate for the main
and aux ADCs. The filter register cannot be written to by the
user while either ADC is active. The update rate is used for both
main and aux ADCs and is calculated as follows:
f
SF
ADC = × fMOD
×
× 1
3
1
8
where:
fADC = ADC output update rate
fMOD = Modulator clock frequency = 32.768 kHz
(main and aux ADC)
SF = Decimal value written to SF register
The allowable range for SF is 13dec to 255dec. Examples of SF
values and corresponding conversion rate (fADC) and time (tADC)
are shown in Table XV. It should also be noted that both ADC
input channels are chopped to minimize offset errors. This
means that the time for a single conversion or the time to the
first conversion result is 2 × tADC.
Table XV. Update Rate vs. SF WORD
SF (dec) SF (Hex) fADC (Hz) tADC (ms)
13 0D 105.3 9.52
69 45 19.79 50.34
255 FF 5.35 186.77
I/O and Current Source Control Register (IOCON): (A3, A2,
A1, A0 = 0, 1, 1, 1; Power-On Reset = 0x0000)
The IOCON register is a 16-bit register from which data can be
read or to which data can be written. This register is used to control
and configure the various excitation and burnout current source
options available on-chip along with controlling the I/O port.
Table XVI outlines the bit designations for this register. IOCON15
through IOCON0 indicate the bit location, with IOCON denoting
that the bits are in the I/O and Current Source control register.
IOCON15 denotes the first bit of the data stream. The number
in parentheses indicates the power-on/reset default status of that
bit. A write to the IOCON register has immediate effect and
does not reset the ADCs. Thus if a current source is switched
while the ADC is converting, the user will have to wait for the
full settling time of the filter before getting a fully settled output.
Since the ADC is chopped, this equates to three outputs.
REV. A
AD7719
–25–
Table XVI. IOCON (I/O and Current Source Control Register) Bit Designations
Bit Bit
Location Name Description
IOCON15 PSW2 Power Switch 2 Control Bit.
Set by user to enable power switch P2 to PWRGND.
Cleared by user to enable use as a standard I/O pin.
When the ADC is in standby mode, the power switches are open.
IOCON14 PSW1 Power Switch 1 Control Bit.
Set by user to enable power switch P1 to PWRGND.
Cleared by user to enable use as a standard I/O pin.
When ADC is in standby mode, the power switches are open.
IOCON13 0 This bit must be zero for correct operation.
IOCON12 BO Burnout Current Enable Bit.
Set by user to enable the 100 nA current sources in the main ADC signal path. A 100 nA current source is
applied to the positive input leg while a 100 nA sink is applied to the negative input.
Cleared by user to disable both transducer burnout current sources.
IOCON11 I2PIN IEXE2, 200 μA Current Source Direction Bit.
Set by user to enable IEXC2 current source to IOUT1.
Cleared by user to enable IEXC2 current source to IOUT2.
IOCON10 I1PIN IEXE1, 200 μA Current Source Direction Bit.
Set by user to enable IEXC1 current source to IOUT2.
Cleared by user to enable IEXC1 current source to IOUT1.
IOCON9 I2EN IEXC2 Current Source Enable Bit.
Set by user to turn on the IEXC2 excitation current source.
Cleared by user to turn off the IEXC2 excitation current source.
IOCON8 I1EN IEXC1 Current Source Enable Bit.
Set by user to turn on the IEXC1 excitation current source.
Cleared by user to turn off the IEXC1 excitation current source.
IOCON7 P4DIR P4, I/O Direction Control Bit.
Set by user to enable P4 as an output.
Cleared by user to enable P4 as an input. There are weak active pull-ups internally when enabled as an input.
IOCON6 P3DIR P3, I/O Direction Control Bit.
Set by user to enable P3 as an output.
Cleared by user to enable P3 as an input. There are weak active pull-ups internally when enabled as an input.
IOCON5 P2EN P2 Digital Output Enable Bit.
Set by user to enable P2 as a regular digital output pin.
Cleared by user to three-state P2 output.
PSW2 takes precedence over P2EN.
IOCON4 P1EN P1 Digital Output Enable Bit.
Set by user to enable P1 as a regular digital output pin.
Cleared by user to three-state P1 output.
PSW1 takes precedence over P1EN.
IOCON3 P4DAT Digital I/O Port Data Bits.
IOCON2 P3DAT The readback values of these bits indicate the status of their respective pin when the I/O port is active as
IOCON1 P2DAT an input.
IOCON0 P1DAT The values written to these data bits appear at the output port when the I/O bits are enabled as outputs.
P2 and P1 are outputs only, so reading P2DAT and P1DAT will return what was last written to these bits.
REV. A
AD7719
–26–
Main ADC Data Result Registers (DATA0): (A3, A2, A1, A0 =
0, 1, 0, 1; Power-On Reset = 0x00 0000)
The conversion results for the main ADC channel are stored in
the main ADC data register (DATA0). This register is either 16
or 24 bits wide, depending on the status of the WL bit in the
main ADC control register (AD0CON). This is a read-only
register. On completion of a read from this register, the RDY0
bit in the status register is cleared.
Aux ADC Data Result Registers (DATA1): (A3, A2, A1, A0 = 0,
1, 1, 0; Power-On Reset = 0x0000)
The conversion results for the aux ADC channel are stored in
the aux ADC data register (DATA1). This register is 16 bits
wide and is a read-only register. On completion of a read from
this register, the RDY1 bit in the status register is cleared.
Main ADC Offset Calibration Coefficient Registers (OF0):
(A3, A2, A1, A0 = 1, 0, 0, 0; Power-On Reset = 0x80 0000)
The offset calibration registers hold the 24-bit data offset
calibration coefficient for the main ADC. There are three
registers associated with the main ADC channel. In fully
differential operating mode, there are two input channels and a
register is dedicated to each input. When operating in
pseudodifferential mode, the main ADC can be configured for
three input channels and there is a dedicated register for each
pseudodifferential input. These registers have a power-on reset
value of 0x80 0000. The channel bits, in association with the
communication register address for the OF0 register, allow
access to these registers. These registers are read/write registers.
The calibration registers can only be written to if the ADC is
inactive (MD bits in the mode register = 000 or 001 or both
AD0EN and AD1EN bits in the control registers are cleared).
Reading of the calibration registers does not clear the RDY0 bit.
Aux ADC Offset Calibration Coefficient Registers (OF1):
(A3, A2, A1, A0 = 1, 0, 0, 1; Power-On Reset = 0x8000)
The offset calibration register OF1 holds the 16-bit data offset
calibration coefficient for the aux ADC. This register has a poweron-
reset value of 0x8000. The channel bits, in association with
the communication register address for the OF1 register, allow
access to these registers. These registers are read/write registers.
The calibration registers can only be written to if the ADC is
inactive (MD bits in the mode register = 000 or 001 or both
AD0EN and AD1EN bits in the control registers are cleared).
Reading of the calibration registers does not clear the RDY1 bit.
Main ADC Gain Calibration Coefficient Registers (GNO):
(A3, A2, A1, A0 = 1, 0, 1, 0; Power-On Reset = 0x5X XXX5)
The gain calibration registers hold the 24-bit data gain calibration
coefficient for the main ADC. These registers are configured at
power-on with factory calculated internal full-scale calibration
coefficients. Every device will have different coefficients. However,
these bytes will be automatically overwritten if an internal or
system full-scale calibration is initiated by the user via MD2–0 bits
in the Mode register. There are three gain calibration registers
associated with the main ADC channel. In fully differential
operating mode, there are two input channels and a register is
dedicated to each input. When operating in pseudodifferential
mode, the main ADC can be configured for three input channels
and there is a dedicated register for each pseudodifferential
input. These registers are read/write registers. The calibration
registers can only be written to if the ADC is inactive (MD bits
in the mode register = 000 or 001 or both AD0EN and AD1EN
bits in the control registers are cleared). Reading of the
calibration registers does not clear the RDY1 bit.
Aux ADC Gain Calibration Coefficient Registers (GN1): (A3,
A2, A1, A0 = 1, 0, 1, 1; Power-On Reset = 0x59XX)
The gain calibration register GN1 holds the 16-bit data gain
calibration coefficient for the aux ADC. This register is configured
at power-on with factory calculated internal zero-scale calibration
coefficients. Every device will have different coefficients. However,
these coefficients will be automatically overwritten if an internal
or system zero-scale calibration is initiated by the user via the
MD2–0 bits in the Mode register. These registers are read/write
registers. The calibration registers can only be written to if the
ADC is inactive (MD bits in the mode register = 000 or 001 or
both AD0EN and AD1EN bits in the control registers are cleared).
Reading of the calibration registers does not clear the RDY1 bit.
ID Register (ID): (A3, A2, A1, A0 = 1, 1, 1, 1; Power-On
Reset = 0x0X)
This register is a read-only 8-bit register. The contents are used
to determine the die revision of the AD7719. Table XVII
indicates the bit locations.
User Nonprogrammable Test Registers
The AD7719 contains two test registers. The bits in this test
register control the test modes of the AD7719, which are used
for the testing of the device. The user is advised not to change the
contents of these registers.
Table XVII. ID Register Bit Designations
ID7 ID6 ID5 ID4 ID3 ID2 ID1 ID0
0 0 0 0 X X X X
REV. A
AD7719
–27–
CONFIGURING THE AD7719
All user-accessible registers on the AD7719 are accessed via the
serial interface. Communication with any of these registers is
initiated by first writing to the Communications register.
Figure 11 outlines a flow diagram of the sequence used to configure
all registers after a power-up or reset on the AD7719. The
flowchart shows two methods of determining when it is valid to
read the data register or determine when a calibration cycle is
complete. The first method is hardware polling of the RDY
pin and the second method involves software interrogation of
bits in the status and mode registers. The flowchart details all the
necessary programming steps required to initialize the ADC and
read data from the main and aux channel following a power-on or
reset. The steps can be broken down as follows:
1. Configure and initialize the microcontroller or microprocessor
serial port.
2. Initialize the AD7719 by configuring the following registers:
a) IOCON to configure the current sources and digital
I/O port.
b) FILTER to configure the update rate for both channels.
c) AD1CON to enable the aux channel, select the analog
input, select unipolar or bipolar operation and input range.
d) AD0CON to enable the main ADC channel and select
16-/24-bit mode, analog input range, and either unipolar
or bipolar operation.
e) MODE to configure the operating mode. Operating mode
consists of calibration or conversion.
All of these operations consist of a write to the communications
register to specify the next operation as a write to a specified
register. Data is then written to this register. When each
sequence is complete, the ADC defaults to waiting for
another write to the Communications register to specify the
next operation.
3. When the operating mode is selected, the user needs to determine
when it is valid to read the data in conversion mode or
when the calibration is complete in calibration mode. This is
accomplished either by polling the RDY pin (hardware polling)
or by interrogating the bits in either the Status or Mode
registers (software polling). Both are shown in Figure 11. It is
assumed that both the main and aux ADCs are being used
and calibration is required. If the AD7719 is operated at
the factory-calibrated conditions, a field calibration will not be
required and these steps can be bypassed.
MICROCOMPUTER/MICROPROCESSOR INTERFACING
The AD7719’s flexible serial interface allows for easy interface
to most microcomputers and microprocessors. The flowchart of
Figure 11 outlines the sequence that should be followed when
interfacing a microcontroller or microprocessor to the AD7719.
Figures 12, 13, and 14 show some typical interface circuits.
The serial interface on the AD7719 is capable of operating from
just three wires and is compatible with SPI interface protocols.
The 3-wire operation makes the part ideal for isolated systems
where minimizing the number of interface lines minimizes the
number of opto-isolators required in the system. The serial
clock input is a Schmitt-triggered input to accommodate slow
edges from optocouplers. The rise and fall times of other digital
inputs to the AD7719 should be no longer than 1 μs.
Most of the registers on the AD7719 are 8-bit registers, which
facilitates easy interfacing to the 8-bit serial ports of microcontrollers.
The main channel data register (AD0) on the AD7719
can be either 16 or 24 bits, the aux ADC data register (AD1) is
16 bits wide and the offset and gain registers are 24-bit registers,
but data transfers to these registers can consist of multiple 8-bit
transfers to the serial port of the microcontroller. DSP processors
and microprocessors generally transfer 16 bits of data in a
serial data operation. Some of these processors, such as the
ADSP-2105, have the facility to program the amount of cycles
in a serial transfer. This allows the user to tailor the number of
bits in any transfer to match the register length of the required
register in the AD7719.
Even though some of the registers on the AD7719 are only eight
bits in length, communicating with two of these registers in
successive write operations can be handled as a single 16-bit
data transfer, if required. For example, if the Filter register is to
be updated, the processor must first write to the Communications
register (saying that the next operation is a write to the
Filter register) and then write eight bits to the Setup register. If
required, this can all be done in a single 16-bit transfer because
once the eight serial clocks of the write operation to the Communications
register have been completed, the part immediately
sets itself up for a write operation to the Setup register.
REV. A
AD7719
–28–
START
POWER-ON/RESET FOR AD7719
CONFIGURE AND INITIALIZE C/P SERIAL PORT
WRITE TO THE COMMUNICATIONS REGISTER
SELECTING NEXT OPERATION TO BE A WRITE
TO THE IOCON REGISTER
WRITE TO THE IOCON REGISTER TO CONFIGURE
THE CURRENT SOURCES, DIGITAL I/O PORT,
AND POWER SWITCHES
WRITE TO COMMUNICATIONS REGISTER SETTING
UP NEXT OPERATION TO BE A WRITE TO THE
FILTER REGISTER
WRITE TO FILTER REGISTER CONFIRMING
THE REQUIRED UPDATE RATE
WRITE TO COMMUNICATIONS REGISTER SETTING
UP NEXT OPERATION TO BE A WRITE TO THE AUX
CHANNEL ADC CONTROL REGISTER (AD1CON)
WRITE TO AD1CON REGISTER ENABLING THE AUX
ADC, SELECT THE INPUT CHANNEL BIPOLAR/
UNIPOLAR OPERATION AND INPUT RANGE
WRITE TO COMMUNICATIONS REGISTER SETTING
UP NEXT OPERATION TO BE A WRITE TO THE MAIN
CHANNEL ADC CONTROL REGISTER (AD0CON)
WRITE TO AD1CON REGISTER ENABLING THE MAIN
ADC, SELECT THE INPUT CHANNEL, WORD LENGTH,
BIPOLAR/UNIPOLAR OPERATION, AND INPUT RANGE
WRITE TO THE COMMUNICATIONS REGISTER
SETTING UP NEXT OPERATION TO BE A WRITE
TO THE MODE REGISTER
WRITE TO MODE REGISTER SELECTING
FULL-SCALE CALIBRATION
HARDWARE
POLLING
SOFTWARE
POLLING
HARDWARE
POLLING
POLL RDY PIN
RDY
LOW?
NO
YES
WRITE TO THE COMMUNICATIONS REGISTER
SETTING UP NEXT OPERATION TO BE A WRITE
TO THE MODE REGISTER
WRITE TO MODE REGISTER SELECTING
ZERO-SCALE CALIBRATION
POLL RDY PIN
RDY
LOW?
NO
YES
WRITE TO THE COMMUNICATIONS REGISTER
SETTING UP NEXT OPERATION TO BE A WRITE
TO THE MODE REGISTER
WRITE TO MODE REGISTER SELECTING
CONTINUOUS CONVERSION MODE
WRITE TO THE COMMUNICATIONS REGISTER SETTING
UP NEXT OPERATION TO BE A READ FROM THE
MAIN ADC DATA REGISTER (AD0)
POLL RDY PIN
RDY
LOW?
NO
YES
READ FROM DATA REGISTER (AD0)
POLL RDY PIN
RDY
LOW?
NO
YES
WRITE TO THE COMMUNICATIONS REGISTER SETTING
UP NEXT OPERATION TO BE A READ FROM THE
AUX ADC DATA REGISTER (AD1)
READ FROM DATA REGISTER (AD0)
SOFTWARE
POLLING
WRITE TO THE COMMUNICATIONS REGISTER
SETTING UP NEXT OPERATION TO BE A
READ FROM THE MODE REGISTER
READ FROM MODE REGISTER
MD BITS
= 001?
NO
YES
WRITE TO THE COMMUNICATIONS REGISTER
SETTING UP NEXT OPERATION TO BE A WRITE
TO THE MODE REGISTER
WRITE TO MODE REGISTER SELECTING
FULL-SCALE CALIBRATION
WRITE TO THE COMMUNICATIONS REGISTER
SETTING UP NEXT OPERATION TO BE A
READ FROM THE MODE REGISTER
READ FROM MODE REGISTER
MD BITS
= 001?
NO
YES
WRITE TO THE COMMUNICATIONS REGISTER
SETTING UP NEXT OPERATION TO BE A WRITE
TO THE MODE REGISTER
WRITE TO MODE REGISTER SELECTING
CONTINUOUS CONVERSION MODE
WRITE TO THE COMMUNICATIONS REGISTER
SETTING UP NEXT OPERATION TO BE A WRITE
TO THE MODE REGISTER
WRITE TO MODE REGISTER SELECTING
CONTINUOUS CONVERSION MODE
WRITE TO THE COMMUNICATIONS REGISTER SETTING
UP NEXT OPERATION TO BE A READ OF
STATUS REGISTER
READ STATUS REGISTER
RDY0 = 1?
NO
YES
WRITE TO THE COMMUNICATIONS REGISTER
SETTING UP NEXT OPERATION TO BE A READ
OF THE MAIN ADC DATA REGISTER (AD0)
READ AD0
WRITE TO THE COMMUNICATIONS REGISTER SETTING
UP NEXT OPERATION TO BE A READ OF
STATUS REGISTER
READ STATUS REGISTER
RDY1 = 1?
NO
YES
WRITE TO THE COMMUNICATIONS REGISTER
SETTING UP NEXT OPERATION TO BE A READ
OF THE AUX ADC DATA REGISTER (AD1)
READ AD1
Figure 11. Flowchart for Initializing, Calibrating, and Reading Data from the AD7719 Main and Aux Channels
REV. A
AD7719
–29–
AD7719-to-68HC11 Interface
Figure 12 shows an interface between the AD7719 and the 68HC11
microcontroller. The diagram shows the minimum (3-wire) interface
with CS on the AD7719 hardwired low. In this scheme, the
RDY bits of the Status register are monitored to determine
when the Data register is updated. RDY0 indicates the status of
the main ADC channel while RDY1 indicates the status of the
aux channel. An alternative scheme, which increases the number
of interface lines to four, is to monitor the RDY output line
from the AD7719. The monitoring of the RDY line can be done in
two ways. First, RDY can be connected to one of the 68HC11’s
port bits (such as PC0), which is configured as an input. This
port bit is then polled to determine the status of RDY. The
second scheme is to use an interrupt driven system, in which case
the RDY output is connected to the IRQ input of the 68HC11. For
interfaces that require control of the CS input on the AD7719,
one of the port bits of the 68HC11 (such as PC1) that is
configured as an output, can be used to drive the CS input.
The 68HC11 is configured in the master mode with its CPOL
bit set to a logic 1 and its CPHA bit set to a logic 1. When the
68HC11 is configured like this, its SCLK line idles high between
data transfers. The AD7719 is not capable of full duplex operation.
If the AD7719 is configured for a write operation, no data appears
on the DOUT lines even when the SCLK input is active. Similarly,
if the AD7719 is configured for a read operation, data
presented to the part on the DIN line is ignored even when
SCLK is active.
68HC11 AD7719
VDD
SS
SCK
MISO
MOSI
RESET
SCLK
CS
DIN
DOUT
VDD
Figure 12. AD7719-to-68HC11 Interface
AD7719-to-8xC51 Interface
An interface circuit between the AD7719 and the 8xC51 microcontroller
is shown in Figure 13. The diagram shows the minimum
number of interface connections with CS on the AD7719 hardwired
low. In the case of the 8xC51 interface, the minimum
number of interconnects is just two. In this scheme, the RDY
bits of the Status register are monitored to determine when the
Data register is updated. The alternative scheme, which increases
the number of interface lines to three, is to monitor the RDY output
line from the AD7719. The monitoring of the RDY line can be
done in two ways. First, RDY can be connected to one of the
8xC51’s port bits (such as P1.0) that is configured as an input.
This port bit is then polled to determine the status of RDY.
DVDD
8xC51 AD7719
P3.0
P3.1
RESET
SCLK
CS
DVDD
10k
DIN
DOUT
Figure 13. AD7719-to-8XC51 Interface
The second scheme is to use an interrupt-driven system, in which
case the RDY output is connected to the INT1 input of the
8xC51. For interfaces that require control of the CS input on
the AD7719, one of the port bits of the 8xC51 (such as P1.1)
that is configured as an output can be used to drive the CS
input. The 8xC51 is configured in its Mode 0 serial interface
mode. Its serial interface contains a single data line. As a result,
the DOUT and DIN pins of the AD7719 should be connected
together with a 10 kΩ pull-up resistor. The serial clock on the
8xC51 idles high between data transfers. The 8xC51 outputs the
LSB first in a write operation, while the AD7719 expects the
MSB first so the data to be transmitted has to be rearranged
before being written to the output serial register. Similarly, the
AD7719 outputs the MSB first during a read operation while
the 8xC51 expects the LSB first. Therefore, the data read into
the serial buffer needs to be rearranged before the correct data
word from the AD7719 is available in the accumulator.
REV. A
AD7719
–30–
AD7719-to-ADSP-2103/ADSP-2105 Interface
Figure 14 shows an interface between the AD7719 and the
ADSP-2103/ADSP-2105 DSP processor. In the interface shown,
the RDY bits of the Status register are again monitored to
determine when the Data register is updated. The alternative
scheme is to use an interrupt-driven system, in which case the
RDY output is connected to the IRQ2 input of the ADSP-2103/
ADSP-2105. The serial interface of the ADSP-2103/ADSP-2105
is set up for alternate framing mode. The RFS and TFS pins
of the ADSP-2103/ADSP-2105 are configured as active low
outputs and the ADSP-2103/ADSP-2105 serial clock line, SCLK,
is also configured as an output. The CS for the AD7719 is active
when either the RFS or TFS outputs from the ADSP-2103/
ADSP-2105 are active. The serial clock rate on the ADSP-2103/
ADSP-2105 should be limited to 3 MHz to ensure correct operation
with the AD7719.
RESET
DVDD
CS
AD7719
SCLK SCLK
DT
DR
RFS
TFS
ADSP-2103 /
ADSP-2105
DIN
DOUT
Figure 14. AD7719-to-ADSP-2103/ADSP-2105 Interface
CIRCUIT DESCRIPTION
The AD7719 is a Σ-Δ A/D converter incorporating two independent
Σ-Δ A/D converters with on-chip digital filtering, intended
for the measurement of wide dynamic range, low frequency
signals such as those in weigh scale, pressure, temperature,
industrial control, or process control applications.
The main ADC is intended to convert the primary sensor input.
The main ADC employs a Σ-Δ conversion technique to realize up
to 24 bits of no-missing-codes performance. The Σ-Δ modulator
converts the sampled input signal into a digital pulse train whose
duty cycle contains the digital information. A Sinc3 programmable
low-pass filter is then employed to decimate the modulator output
data stream to give a valid data conversion result at programmable
output rates from 5.35 Hz (186.77 ms) to 105.03 Hz
(9.52 ms). A Chopping scheme is also employed to minimize
ADC offset and offset and gain drift errors. The analog input
to the main ADC can be operated in buffered or unbuffered
mode and can be programmed for one of eight input ranges from
±20 mV to ±2.56 V. The input channels can be configured for
either fully differential inputs or pseudodifferential input
channels via the CH1 and CH0 bits in the main ADC control
register (AD0CON) and the CHCON bit in the mode register.
When configured for buffered mode (BUF = 0), the input channels
are internally buffered, allowing the part to handle significant
source impedances on the analog input, allowing R/C filtering
(for noise rejection or RFI reduction) to be placed on the analog
inputs if required. When operating in unbuffered mode, care has
to be exercised when selecting front end source impedances so
as not to introduce gain errors. On-chip burnout currents are
available and can be used to check that a transducer on the
selected channel is still operational before attempting to take
measurements.
The second or auxiliary ADC is intended to convert secondary
inputs such as those from a cold junction diode or thermistor.
This ADC is unbuffered and has a fixed input range of 0 V
to REFIN2 (ARN bit = 1) or 0 to REFIN2/2 (ARN bit = 0).
Again, this ADC can be configured for differential or pseudodifferential
inputs via the ACH2, ACH1, and ACH0 bits in the
auxiliary ADC control register (AD1CON). The auxiliary ADC
is specified for 16-bit performance and, since its analog inputs
are unbuffered, care must be exercised when placing filtering on
the front end to avoid introducing gain errors into the measurement
system.
The basic connection diagram for the AD7719 is shown in
Figure 15. This shows both the AVDD and DVDD pins of the
AD7719 being driven from the analog 5 V supply. Some applications
will have AVDD and DVDD driven from separate supplies.
AVDD and DVDD can be operated independently of each other,
allowing the device to be operated with 5 V analog supply and
3 V digital supply or vice versa. An AD780/REF195 precision
2.5 V reference provides the reference source for the part. A
quartz crystal or ceramic resonator provides the 32 kHz master
clock source for the part. In some cases, it will be necessary to
connect capacitors on the crystal or resonator to ensure that it
does not oscillate at overtones of its fundamental operating
frequency. The values of capacitors will vary depending on the
manufacturer’s specifications.
AD780/
REF195
XTAL1
XTAL2
RECEIVE
(READ)
P1/SW1
P2/SW2
P3
P4
0.1F
10F
ANALOG 5V
SUPPLY
GND
VIN VOUT
PWRGND AGND DGND
10F 0.1F 0.1F
AVDD DVDD
IOUT1
IOUT2
AIN1
AIN2
AIN3
AIN4
AIN5
REFIN2
REFIN1(+)
REFIN1(–)
AIN6
RESET
CS
DOUT
DIN
SCLK
5V
CHIP
SELECT
SERIAL
DATA
(WRITE)
SERIAL
CLOCK
32kHz
CRYSTAL
ANALOG 5V
SUPPLY
AD7719
Figure 15. Basic Connection Diagram
REV. A
AD7719
–31–
Analog Input Channels
The main ADC has four associated analog input pins (labeled
AIN1 to AIN4) that can be configured as two fully differential
input channels or three pseudodifferential input channels.
Channel selection bits CH1 and CH0 in the ADC0CON
register, along with the CHCON bit of the mode register,
detail the different configurations.
The auxiliary ADC has four external input pins (labeled AIN3
to AIN6) as well as an internal connection to the internal on-chip
temperature sensor. Channel selection bits ACH2, ACH1, and
ACH0 in the ADC1CON register, along with the CHCON
bit in the mode register, detail the various configurations on
these input channels.
Two input multiplexers (MUX1 and MUX2) switch the selected
input channel to the on-chip buffer amplifier in the case of the
main ADC when operated in buffered mode, and directly to the
Σ-Δ modulator input in the case of the auxiliary ADC and when
the main ADC is operated in unbuffered mode. When the analog
input channel is switched, the settling time of the part must elapse
before a new valid word is available from the ADC.
Figure 16 shows the analog input channel configurations available
to the user when the CHCON bit in the mode register is set to a
zero. In this case, the main ADC can be configured as one or two
fully differential input channels (AIN1/AIN2 and AIN3/AIN4)
and the aux can be configured as two single-ended inputs with
respect to AGND (AIN3/AGND and AIN4/AGND) and one fully
differential input AIN5/AIN6). The aux can also be configured as
three single-ended inputs with respect to AGND (AIN3/AGND,
AIN4/AGND, and AIN5/AGND) by tying AIN6 externally to
AGND. The temp sensor is available as an internal connection.
SINGLEENDED
INPUT
SINGLEENDED
INPUT
FULLY DIFFERENTIAL
FULLY DIFFERENTIAL
FULLY DIFFERENTIAL AIN(+)
AIN(–)
AIN(+)
AIN(–)
AIN1
AIN2
AIN3
AIN4
AIN1
AIN2
AIN3
AIN4
AIN5
AIN6
AIN3
AIN4
AGND
MUX1
(MAIN ADC)
(AUX ADC)
AIN5
AIN6
MAIN CHANNEL
AUX CHANNEL
MUX2
Figure 16. Input Channel Configurations with CHCON = 0
Figure 17 shows the analog input channel configurations available
to the user when the CHCON bit in the mode register is
set to 1. In this case, the main ADC is configured as three
pseudodifferential input channels (AIN1/AIN4, AIN2/AIN4, and
AIN3/AIN4) and the aux can be configured as two single-ended
inputs with respect to AGND (AIN5/AGND and AIN6/AGND)
and one fully differential input (AIN5/AIN6). The temp sensor
is available as an internal connection.
SINGLEENDED
INPUT
FULLY DIFFERENTIAL
AIN(+)
AIN(–)
AIN(+)
AIN(–)
AIN1
AIN2
AIN3
AIN4
AIN1
AIN2
AIN3
AIN4
AIN5
AIN6
AIN3
AIN4
AGND
MUX1
(MAIN ADC)
(AUX ADC)
AIN5
AIN6
SINGLEENDED
INPUT
AIN3/AIN4
AIN2/AIN4
AIN1/AIN4
MUX2
MAIN CHANNEL
AUX CHANNEL
PSEUDODIFFERENTIAL
INPUT
PSEUDODIFFERENTIAL
INPUT
Figure 17. Input Channel Configurations with CHCON = 1
In buffered mode (BUF = 0), the output of the main ADC multiplexer
feeds into a high impedance input stage of the buffer
amplifier. As a result, the main ADC inputs can handle significant
source impedances and are tailored for direct connection to
external resistive-type sensors like strain gages or resistance
temperature detectors (RTDs).
The auxiliary ADC and the main ADC when operated with
BUF = 1, however, are unbuffered, resulting in higher analog
input current. It should be noted that these unbuffered input
paths provide a dynamic load to the driving source. Therefore,
resistor/capacitor combinations on the input pins can cause dc
gain errors, depending on the output impedance of the source
that is driving the ADC inputs. Table XVIII and XIX show the
allowable external resistance/capacitance values for unbuffered
mode such that no gain error at the 16- and 20-bit level,
respectively, is introduced.
The absolute input voltage range on the main ADC when operated
in buffered mode is restricted to a range between AGND +
100 mV and AVDD – 100 mV. Care must be taken in setting up
the common-mode voltage and input voltage range so that these
limits are not exceeded; otherwise there will be a degradation in
linearity and noise performance.
REV. A
AD7719
–32–
The absolute input voltage range on the auxiliary ADC and the
main ADC in unbuffered mode includes the range between
AGND – 30 mV to AVDD + 30 mV as a result of being unbuffered.
The negative absolute input voltage limit does allow
the possibility of monitoring small true bipolar signals with
respect to AGND.
Programmable Gain Amplifier
The output from the buffer on the main ADC is applied to the
input of the on-chip programmable gain amplifier (PGA). The
PGA can be programmed through eight different unipolar and
bipolar ranges. The PGA gain range is programmed via the
range bits in the ADC0CON register. With an external 2.5 V
reference applied, the unipolar ranges are 0 mV to 20 mV, 0 mV
to 40 mV, 0 mV to 80 mV, 0 mV to 160 mV, 0 mV to 320 mV,
0 mV to 640 mV, 0 V to 1.28 V and 0 V to 2.56 V while bipolar
ranges are ±20 mV, ±40 mV, ±80 mV, ±160 mV, ±320 mV,
±640 mV, ±1.28 V, and ±2.56 V. These are the ranges that
should appear at the input to the on-chip PGA. The ADC range
matching specification of 2 μV (typ) across all ranges means that
calibration need only be carried out on a single range and does
not have to be repeated when the PGA range is changed. This is
a significant advantage when compared with similar ADCs
available on the market.
Typical matching across ranges is shown in Figure 18. Here, the
primary ADC is configured in fully differential, bipolar mode
with an external 2.5 V reference, while an analog input voltage
of just greater than 19 mV is forced on its analog inputs. The
ADC continuously converts the dc voltage at an update rate of
5.35 Hz, i.e., SF = 0xFF. In total, 800 conversion results are
gathered. The first 100 results are gathered with the primary ADC
operating in the ±20 mV range. The ADC range is then switched
to ±40 mV and 100 more results are gathered; this continues
until the last 100 samples are gathered with the ADC configured
in the ±2.5 V range. From Figure 18, the variation in the sample
mean through each range, i.e., the range matching, is seen to
be on the order of 2 μV.
The auxiliary ADC does not incorporate an eight range PGA.
The aux ADC operates at a gain of 1 or a gain of 2 as determined
by the ARN bit in the AD1CON register.
0 100 200 300 400 500 600 700 800 SAMPLE COUNT ADC INPUT VOLTAGE (
mV)
19.372
19.371
19.370
19.369
19.368
19.367
19.366
19.365
19.364
ADC RANGE
20mV
40mV
80mV
160mV
320mV
640mV
1.28V
2.56V
Figure 18. Main ADC Range Matching
Bipolar/Unipolar Configuration
The analog inputs on the AD7719 can accept either unipolar or
bipolar input voltage ranges. Bipolar input ranges do not imply that
the part can handle negative voltages with respect to system
AGND. Unipolar and bipolar signals on the AIN(+) input on
the main ADC are referenced to the voltage on the respective
AIN(–) input. AIN(+) and AIN(–) refer to the signals seen by
the modulator that come from the output of the multiplexer, as
shown in Figures 16 and 17.
For example, if AIN(–) is 2.5 V and the main ADC is configured
for an analog input range of 0 mV to 20 mV, the input
voltage range on the AIN(+) input is 2.5 V to 2.52 V. If AIN(–)
is 2.5 V and the AD7719 is configured for an analog input range
of ±1.28 V, the analog input range on the AIN(+) input is 1.22 V
to 3.78 V (i.e., 2.5 V ± 1.28 V). Bipolar or unipolar options are
chosen by programming the main and auxiliary U/B bit in the
ADC0CON and ADC1CON registers, respectively. This programs
the relevant ADC for either unipolar or bipolar operation.
Programming for either unipolar or bipolar operation does not
change any of the input signal conditioning; it simply changes the
data output coding and the points on the transfer function
where calibrations occur.
Table XVIII. Max Resistance for No 16-Bit Gain Error (Unbuffered Mode)
External Capacitance
Gain 0 pF 50 pF 100 pF 500 pF 1000 pF 5000 pF
1 111.3K 27.8K 16.7K 4.5K 2.58K 700
2 53.7K 13.5K 8.1K 2.2K 1.26K 360
4 25.4K 6.4K 3.9K 1.0K 600 170
8–128 10.7K 2.9K 1.7K 480 270 75
Table XIX. Max Resistance for No 20-Bit Gain Error (Unbuffered Mode)
External Capacitance
Gain 0 pF 50 pF 100 pF 500 pF 1000 pF 5000 pF
1 84.9K 21.1K 12.5K 3.2K 1.77K 440
2 42.0K 10.4K 6.1K 1.6K 880 220
4 20.5K 5.0K 2.9K 790K 430 110
8–128 8.8K 2.3K 1.3K 370 195 50
REV. A
AD7719
–33–
Data Output Coding
When the ADC is configured for unipolar operation, the output
coding is natural (straight) binary with a zero differential input
voltage resulting in a code of 000 . . . 000, a midscale voltage
resulting in a code of 100 . . . 000, and a full-scale input voltage
resulting in a code of 111 . . . 111. The output code for any analog
input voltage on the main ADC can be represented as follows:
Code AIN GAIN N V
= ( × × 2 ) (1.024 × REF )
Where AIN is the analog input voltage, GAIN is the PGA gain,
i.e., 1 on the 2.56 V range and 128 on the 20 mV range, and
N = 16 in 16-bit mode and N = 24 in 24-bit mode of operation.
The output code for any analog input voltage on the aux ADC
can be represented as follows:
Code AIN GAIN N V
= ( × × 2 ) REF
Where AIN is the analog input voltage, GAIN is 1 or 2, determined
by the ARN bit in the aux ADC control register, i.e., 1 on
the VREF range and 2 on the VREF/2 range, and N = 16.
When an ADC is configured for bipolar operation, the coding is
offset binary with a negative full-scale voltage resulting in a code
of 000 . . . 000, a zero differential voltage resulting in a code of
100 . . . 000, and a positive full-scale voltage resulting in a code
of 111 . . . 111. The output code from the main ADC for any
analog input voltage can be represented as follows:
Code N AIN GAIN V
= 2 × [( × (1 024 × REF )) + 1] –1 .
Where AIN is the analog input voltage, GAIN is the PGA gain,
i.e., 1 on the ±2.56 V range and 128 on the ±20 mV range,
N = 16 in 16-bit mode, and N = 24 in 24-bit mode of operation.
The output code from the aux ADC for any analog input voltage
can be represented as follows:
Code N AIN GAIN V
= 2 × [( × REF ) + 1] –1
Where AIN is the analog input voltage, GAIN is 1 or 2, determined
by the ARN bit in the aux ADC control register, i.e., 1 on the
±VREF range, 2 on the ±VREF/2 range, and N = 16.
Burnout Currents
The main ADC on the AD7719 contains two 100 nA constant
current generators, one sourcing current from AVDD to AIN(+),
and one sinking current from AIN(–) to AGND. The currents
are switched to the selected analog input pair. Both currents are
either on or off, depending on the Burnout Current Enable (BO)
bit in the IOCON register. These currents can be used to verify
that an external transducer is still operational before attempting
to take measurements on that channel. Once the burnout currents
are turned on, they will flow in the external transducer circuit,
and a measurement of the input voltage on the analog input
channel can be taken. If the resultant voltage measured is full-scale,
the user needs to verify why this is the case. A full-scale reading
could mean that the front end sensor is open circuit; it could
also mean that the front end sensor is overloaded and is justified
in outputting full-scale, or that the reference may be absent and
the NOXREF bit is set, thus clamping the data to all 1s.
When reading all 1s from the output, the user needs to check
these three cases before making a judgment. If the voltage
measured is 0 V, it may indicate that the transducer has short
circuited. For normal operation, these burnout currents are
turned off by writing a 0 to the BO bit in the IOCON register.
The current sources work over the normal absolute input voltage
range specifications with buffers on.
Excitation Currents
The AD7719 also contains two matched, software configurable
200 μA constant current sources. Both source current from
AVDD that is directed to either the IOUT1 or IOUT2 pins of
the device. These current sources are controlled via bits in the
IOCON register. The configuration bits enable the current
sources and can be configured to source 200 μA individually to
both pins or a combination of both currents, i.e., 400 μA to
either of the selected output pins. These current sources can be
used to excite external resistive bridge or RTD sensors.
Crystal Oscillator
The AD7719 is intended for use with a 32.768 kHz watch crystal.
A PLL internally locks onto a multiple of this frequency to
provide a stable 4.194304 MHz clock for the ADC. The modulator
sample rate is the same as the crystal oscillator frequency.
The start-up time associated with 32 kHz crystals is typically
300 ms. The OSPD bit in the mode register can be used to
prevent the oscillator from powering down when the AD7719 is
placed in power-down mode. This avoids having to wait 300 ms
after exiting power-down to start a conversion at the expense of
raising the power-down current.
Reference Input
The AD7719 has a fully differential reference input capability
for the main channel while the auxiliary channel accepts only a
single-ended reference. On the main channel, the reference inputs
REFIN1(+) and REFIN1(–) provide a differential reference
input capability. The common-mode range for these differential
inputs is from AGND to AVDD. The reference input is unbuffered,
and therefore excessive R-C source impedances will introduce
gain errors. The nominal reference voltage, VREF, (REFIN1(+)
– REFIN1(–), for specified operation is 2.5 V, but the AD7719
is functional with reference voltages from 1 V to AVDD. In applications
where the excitation (voltage or current) for the transducer
on the analog input also drives the reference voltage for the part,
the effect of the low frequency noise in the excitation source
will be removed as the application is ratiometric. If the AD7719
is used in a nonratiometric application, a low noise reference
should be used. Recommended reference voltage sources for
the AD7719 include the AD780, REF43, and REF192. It should
also be noted that the reference inputs provide a high impedance,
dynamic load. Because the input impedance of each reference
input is dynamic, resistor/capacitor combinations on these inputs
can cause dc gain errors, depending on the output impedance
of the source that is driving the reference inputs. Reference
voltage sources like those recommended (e.g., AD780) will typically
have low output impedances and are therefore tolerant to
having decoupling capacitors on the REFIN1(+) without
introducing gain errors in the system. Deriving the reference
input voltage across an external resistor, as shown in Figure 19,
will mean that the reference input sees a significant external
source impedance. External decoupling on the REFIN1(+)
and REFIN1(–) pins would not be recommended in this type
of circuit configuration.
The auxiliary channel conversion results are based on the voltage
applied to REFIN2. This is a single-ended reference input
specified for 2.5 V operation but functional with input voltages
from 1 V to AVDD.
REV. A
AD7719
–34–
Reference Detect
The AD7719 includes on-chip circuitry to detect if the part has a
valid reference on the main ADC for conversions or calibrations.
If the voltage between the external REFIN1(+) and REFIN1(–)
pins goes below 0.3 V or either the REFIN1(+) or REFIN1(–)
inputs are open circuit, the AD7719 detects that it no longer
has a valid reference. In this case, the NOXREF bit of the Status
register is set to 1. If the AD7719 is performing normal conversions
and the NOXREF bit becomes active, the conversion results
revert to all 1s. Therefore, it is not necessary to continuously
monitor the status of the NOXREF bit when performing
conversions. It is only necessary to verify its status if the
conversion result read from the ADC data register is all 1s. If the
AD7719 is performing either an offset or gain calibration and
the NOXREF bit becomes active, the updating of the respective
calibration registers is inhibited to avoid loading incorrect
coefficients to these registers, and the ERR0 bit in the Status
register is set. If the user is concerned about verifying that a
valid reference is in place every time a calibration is performed,
the status of the ERR0 bit should be checked at the end of the
calibration cycle.
Reset Input
The RESET input on the AD7719 resets all the logic, the digital
filter, and the analog modulator while all on-chip registers are
reset to their default state. RDY is driven high and the AD7719
ignores all communications to any of its registers while the
RESET input is low. When the RESET input returns high, the
AD7719 operates with its default setup conditions and it is
necessary to set up all registers and carry out a system calibration
if required after a RESET command.
Power-Down Mode
Loading 0, 0, 0 to the MD2, MD1, MD0 bits in the ADC mode
register places the AD7719 in device power-down mode. Device
power-down mode is the default condition for the AD7719 on
power-up. Individual ADCs (main or auxiliary) can be put in
power-down mode using the AD0EN in the main ADC control
register (AD0CON) to power off the main ADC or the AD1EN
in the auxiliary ADC control register (AD1CON) to power off
the auxiliary ADC. The AD7719 retains the contents of all its
on-chip registers (including the data register) while in powerdown
or ADC disable mode.
The device power-down mode does not affect the digital interface,
and it does affect the status of the RDY pin. Putting the
AD7719 into power-down or idle mode will reset the RDY line
high. Placing the part in power-down mode reduces the total
current (AIDD + DIDD) to 31 μA max when the part is operated
at 5 V and the oscillator is allowed to run during power-down
mode. With the oscillator shuts down, the total IDD is 3 μA max
at 3 V and 9 μA max at 5 V.
Idle Mode
The AD7719 also contains an idle mode. The ADC defaults
to this mode on completion of a calibration sequence and on
the completion of a conversion when operating in single conversion
mode. In idle mode, the power consumption of the
AD7719 is not reduced below the normal mode dissipation.
ADC Disable Mode
This mode is entered by setting both the AD0EN and AD1EN
bits in the main and max ADC control registers to 0 and setting
the Mode bits (MD2, MD1, MD0) in the Mode register to non-0.
In this mode, the internal PLL is enabled and the user can
activate the current sources and power switches, but the power
consumption of the ADC is reduced as both ADCs are disabled.
In this mode, the AIDD is reduced to 0.15 mA and the DIDD is
reduced to 0.35 mA max at 3 V and to 0.4 mA max with DVDD = 5 V.
Calibration
The AD7719 provides four calibration modes that can be programmed
via the mode bits in the mode register. One of the
major benefits of the AD7719 is that it is factory-calibrated as
part of the final test process with the generated coefficients
stored within the ADC. At power-on, the factory gain calibration
coefficients are automatically loaded to the gain calibration
registers on the AD7719. Each ADC (primary and auxiliary) has
dedicated calibration register pairs as outlined in the AD0CON
and AD1CON register descriptions. Given that the ADC is
factory-calibrated and a chopping scheme is employed that gives
excellent offset and drift performance, it is envisaged that in the
majority of applications the user will not need to perform any
field calibrations.
However, the factory calibration values in the ADC calibration
registers will be overwritten if any one of the four calibration
options are initiated. Even though an internal offset calibration
mode is described below, it should be recognized that both
ADCs are chopped. This chopping scheme inherently minimizes
offset and means that an internal offset calibration should never
be required. Also, because factory 25°C gain calibration coefficients
are automatically present at power-on, an internal full-scale
calibration will only be required if the part is being operated at
temperatures significantly different from 25°C or away from the
calibration conditions. The AD7719 offers internal or system
calibration facilities. For full calibration to occur on the selected
ADC, the calibration logic must record the modulator output
for two different input conditions. These are zero-scale and fullscale
points derived by performing a conversion on the different
input voltages provided to the input of the modulator during
calibration. The result of the zero-scale calibration conversion is
stored in the offset calibration registers for the appropriate ADC.
The result of the full-scale calibration conversion is stored in the
gain calibration registers for the appropriate ADC. With these
readings, the calibration logic can calculate the offset and the gain
slope for the input-to-output transfer function of the converter.
During an internal zero-scale or full-scale calibration, the respective
zero input and full-scale input are automatically connected
to the ADC input pins internally to the device. A system
calibration, however, expects the system zero-scale and system
full-scale voltages to be applied to the external ADC pins before
the calibration mode is initiated. In this way, external ADC errors
are taken into account and minimized as a result of system
calibration. It should also be noted that to optimize calibration
accuracy, all AD7719 ADC calibrations are automatically
carried out at the slowest update rate.
REV. A
AD7719
–35–
Internally in the AD7719, the coefficients are normalized before
being used to scale the words coming out of the digital filter. The
offset calibration coefficient is subtracted from the result prior
to the multiplication by the gain coefficient.
From an operational point of view, a calibration should be treated
like another ADC conversion. A zero-scale calibration (if required)
should always be carried out before a full-scale calibration. System
software should monitor the relevant ADC RDY0/1 bit in the
Status register to determine end of calibration via a polling
sequence or interrupt driven routine.
Grounding and Layout
Since the analog inputs and reference input on the main ADC
are differential, most of the voltages in the analog modulator are
common-mode voltages. The excellent common-mode rejection
of the part will remove common-mode noise on these inputs.
The analog and digital supplies to the AD7719 are independent
and separately pinned out to minimize coupling between the
analog and digital sections of the device. The AD7719 can be
operated with 5 V analog and 3 V digital supplies, or vice versa.
The digital filter will provide rejection of broadband noise on
the power supplies, except at integer multiples of the modulator
sampling frequency. The digital filter also removes noise from
the analog and reference inputs provided these noise sources do
not saturate the analog modulator. As a result, the AD7719 is
more immune to noise interference than a conventional high
resolution converter. However, because the resolution of the
AD7719 is so high, and the noise levels from the AD7719 are so
low, care must be taken with regard to grounding and layout.
The printed circuit board that houses the AD7719 should be
designed such that the analog and digital sections are separated
and confined to certain areas of the board. This facilitates the
use of ground planes that can be easily separated. A minimum
etch technique is generally best for ground planes as it gives the
best shielding.
Although the AD7719 has separate pins for analog and digital
ground, the AGND and DGND pins are tied together within
the device via the substrate. The user must not tie these pins
external to separate ground planes unless the ground planes are
connected together near the AD7719.
In systems where the AGND and DGND are connected somewhere
else in the system, i.e., the system power supply, they should
not be connected again at the AD7719 as a ground loop will
result. In these situations, it is recommended that the AD7719’s
AGND and DGND pins be tied to the AGND plane. In any
layout, it is important that the user keep in mind the flow of
currents in the system, ensuring that the return paths for all
currents are as close as possible to the paths the currents took to
reach their destinations. Avoid forcing digital currents to flow
through the AGND sections of the layout.
The PWRGND pin is tied internally to AGND on the AD7719.
The PWRGND pad internally has a resistance of less then 50mΩ
to the PWRGND pin, while the resistance back to the AGND
pad is >3 Ω. This means that 19.5 mA of the maximum specified
current (20 mA) will flow to PWRGND with the remaining
0.5 mA flowing to AGND. PWRGND and AGND should be
tied together at the AD7719 and it is important to minimize the
resistance on the ground return lines.
Avoid running digital lines under the device as these will couple
noise onto the die. The analog ground plane should be allowed
to run under the AD7719 to prevent noise coupling. The power
supply lines to the AD7719 should use as wide a trace as possible
to provide low impedance paths and reduce the effects of glitches
on the power supply line. Fast switching signals like clocks
should be shielded with digital ground to avoid radiating noise
to other sections of the board, and clock signals should never be
run near the analog inputs. Avoid crossover of digital and analog
signals. Traces on opposite sides of the board should run at right
angles to each other. This will reduce the effects of feedthrough
through the board. A microstrip technique is by far the best, but is not
always possible with a double-sided board. In this technique, the
component side of the board is dedicated to ground planes while
signals are placed on the solder side.
Good decoupling is important when using high resolution ADCs.
All analog supplies should be decoupled with 10 μF tantalum in
parallel with 0.1 μF capacitors to AGND. To achieve the best from
these decoupling components, they have to be placed as close as
possible to the device, ideally right up against the device. All logic
chips should be decoupled with 0.1 μF ceramic capacitors to
DGND. In systems where a common supply voltage is used to
drive both the AVDD and DVDD of the AD7719, it is recommended
that the system’s AVDD supply be used. This supply
should have the recommended analog supply decoupling capacitors
between the AVDD pin of the AD7719 and AGND, and the
recommended digital supply decoupling capacitor between the
DVDD pin of the AD7719 and DGND.
APPLICATIONS
The AD7719 provides a low cost, high resolution analog-todigital
function. Because the analog-to-digital function is provided
by a Σ-Δ architecture, it makes the part more immune to noisy
environments, making it ideal for use in sensor measurement and
industrial and process control applications. Given the architecture
used in the AD7719, where the signal chain is chopped and
the device is factory-calibrated at final test, field calibration
can be avoided due to the extremely low offset and gain drifts
exhibited by this converter. It also provides a programmable
gain amplifier, a digital filter, and system calibration options. Thus,
it provides far more system-level functionality than off-the-shelf
integrating ADCs without the disadvantage of having to supply a
high quality integrating capacitor. In addition, using the AD7719
in a system allows the system designer to achieve a much higher
level of resolution because noise performance of the AD7719 is
significantly better than that of integrating ADCs.
The on-chip PGA allows the AD7719 to handle an analog input
voltage range as low as 10 mV full-scale with VREF = 1.25 V. The
differential inputs of the part allow this analog input range to
have an absolute value anywhere between AGND + 100 mV and
AVDD – 100 mV. It allows the user to connect the transducer
directly to the input of the AD7719. The programmable gain
front end on the AD7719 allows the part to handle unipolar
analog input ranges from 0 mV to 20 mV to 0 V to 2.5 V and
bipolar inputs of ±20 mV to ±2.5 V. Because the part operates
from a single supply, these bipolar ranges are with respect to a
biased-up differential input. Another key advantage of the AD7719 is
that it contains two Σ-Δ converters operating in parallel; thus the
user does not need to interrupt the main channel when a secondary
measurement on a different variable needs to be performed.
REV. A
AD7719
–36–
Pressure Measurement
One typical application of the AD7719 is pressure measurement.
Figure 19 shows the AD7719 used with a pressure
transducer, the BP01 from Sensym.
The pressure transducer is arranged in a bridge network and
gives a differential output voltage between its OUT(+) and
OUT(–) terminals. With rated full-scale pressure (in this case
300 mmHg) on the transducer, the differential output voltage is
3 mV/V of the input voltage (i.e., the voltage between its IN(+)
and IN(–) terminals).
Assuming a 5 V excitation voltage, the full-scale output range
from the transducer is 15 mV. The excitation voltage for the
bridge can be used to directly provide the reference for the ADC
as the reference input range includes the supplies. Alternatively,
a suitable resistor divider can be implemented that allows the
full dynamic range of the input to be utilized in these application.
This implementation is fully ratiometric, so variations in
the excitation voltage do not introduce errors in the system.
Choosing resistor values of 20 kΩ and 12 kΩ as per Figure 19
give a 1.875 V reference voltage for the AD7719 when the excitation
voltage is 5 V.
AD7719
IN+
OUT+
OUT–
IN–
20k
12k
EXCITATION VOLTAGE = 5V
AVDD DVDD
AIN1
AIN2
REFIN1(+)
P1
PWRGND
DGND
AGND
REFIN2(–)
Figure 19. Pressure Measurement Using AD7719
Using the part with a programmed gain of 128 results in the
full-scale input span of the AD7719 being 15 mV, which corresponds
with the output span from the transducer.
A second key advantage to using the AD7719 in transducer
based applications is that the on-chip low-side power switch can
be fully utilized in low power applications. The low-side power
switch is connected in series with the cold side of the bridge. In
normal operation, the switch is closed and measurements can be
taken from the bridge. In applications where power is of concern,
the AD7719 can be put in low power mode, substantially
reducing the power burned in the application. In addition to
this, the power switch can be opened while in low power mode
thus avoiding the unnecessary burning of power in the front end
transducer. When the AD7719 is taken back out of power-down
and the power switch is closed, the user should ensure that the
front end circuitry is fully settled before attempting a read from
the AD7719.
The circuit in Figure 20 shows a method that utilizes all three
pseudodifferential input channels on the AD7719 main channel
to temperature-compensate a pressure transducer.
5V
OUT(+)
OUT(–)
IN(–)
IN(+)
I1
I2
PRESSURE
BRIDGE
XTAL1
XTAL2
IOUT1
6.25k
AVDD
REFIN(+)
REFIN(–)
AIN2
AIN1
AIN3
AIN4
AGND
AD7719
250
Figure 20. Temperature-Compensating a Pressure
Transducer
In this application, pseudodifferential input channel AIN1/AIN4
is used to measure the bridge output while pseudodifferential
channels AIN2/AIN4 and AIN3/AIN4 measure the voltage
across the bridge. The voltage measured across the bridge will
vary proportionally with temperature, and the delta in this voltage
can be used to temperature-compensate the output of the
pressure bridge.
Temperature Measurement
The AD7719 is also useful in temperature measurement applications;
Figure 21 shows an RTD temperature measurement
application. In this application, the transducer is an RTD (resistive
temperature device), a PT100. The arrangement is a 4-lead RTD
configuration. There are voltage drops across the lead resistances
RL1 and RL4, but these simply shift the common-mode voltage.
There is no voltage drop across lead resistances RL2 and RL3 as
the input current to the AD7719 is very low, looking into a high
input impedance buffer. RCM is included to shift the analog input
voltage to ensure that it lies within the common-mode range
(AGND + 100 mV to AVDD – 100 mV) of the ADC. In the
application shown, the on-chip 200 μA current source provides the
excitation current for the PT100 and also generates the reference
voltage for the AD7719 via the 12.5 kΩ resistor. Variations in
the excitation current do not affect the circuit as both the input
voltage and the reference voltage vary ratiometrically with the
excitation current. However, the 12.5 kΩ resistor must have a low
temperature coefficient to avoid errors in the reference voltage
over temperature.
REV. A
AD7719
–37–
REFIN(–)
IOUT1
5V
12.5k
AVDD
AIN2
AIN1
AD7719
REFIN(+)
CONTROLLER
IOUT2
DVDD
DGND
AGND PWRGND
DRDY
SCLK
DIN
DOUT
CS
XTAL1
XTAL2
RL1 RREF
RL2
RL3
RL4
RCM
RTD
200A
Figure 21. 4-Wire RTD Temperature Measurement
Using the AD7719
Figure 22 shows a further enhancement to the circuit shown in
Figure 21. Generally, dc excitation has been accepted as the
normal method of exciting resistive-based sensors like RTDs
(resistance temperature detectors) in temperature measurement
applications.
With dc excitation, the excitation current through the sensor
must be large enough so that the smallest temperature/resistance
change to be measured results in a voltage change that is larger
than the system noise, offset, and drift of the system. The purpose
of switching the excitation source is to eliminate dc-induced
errors. DC errors (EMF1 and EMF2) due to parasitic thermocouples
produced by differential metal connections (solder and
copper track) within the circuit are also eliminated when using
this switching arrangement. This excitation is a form of synchronous
detection where the sensor is excited with an alternating
excitation source and the ADC only measures information in the
same phase as the excitation source.
REFIN(–)
IOUT1
IOUT2
AVDD
AIN2
AIN1
AIN3
AIN4
AD7719
REFIN(+)
MUX1
RREF
A A
BUF
AND
PGA
200A
I1
EMF1
RESISTIVE
TRANSDUCER
EMF2
Figure 22. Low Resistance Measurement AD7719
The switched polarity current source is developed using the
on-chip current sources and external phase control switches
(A and A) driven from the controller. During the conversion
process, the AD7719 takes two conversion results, one on each
phase. During Phase 1, the on-chip current source is directed to
IOUT1 and flows top to bottom through the sensor and switch
controlled by A. In Phase 2, the current source is directed to
IOUT2 and flows in the opposite direction through the sensor
and through switch controlled by A. In all cases, the current
flows in the same direction through the reference resistor to
develop the reference voltage for the ADC. All measurements are
ratiometrically derived. The results of both conversions are
combined within the microcontroller to produce one output
measurement representing the resistance or temperature of the
transducer. For example, if the RTD output during Phase 1 is
10 mV, a 1 mV circuit-induced dc error exists due to parasitic
thermocouples, and the ADC measures 11 mV. During the
second phase, the excitation current is reversed and the ADC
measures –10 mV from the RTD and again sees 1 mV dc error,
giving an ADC output of –9 mV during this phase. These measurements
are processed in the controller (11 mV – (–9 mV)/2 =
10 mV), thus removing the dc-induced errors within the system.
In the circuit shown in Figure 22, the resistance measurement is
made using ratiometric techniques. Resistor RREF, which develops
the ADC reference, must be stable over temperature to
prevent reference-induced errors in the measurement output.
3-Wire RTD Configurations
To fully optimize a 3-wire RTD configuration, two identically
matched current sources are required. The AD7719, which
contains two well-matched current sources, is ideally suited to
these applications. One possible 3-wire configuration using the
AD7719 is outlined in Figure 23.
REFIN(–)
IOUT1
DGND
AGND
5V
12.5k
AVDD
AIN2
AIN1
AD7719
RL3
RCM
REFIN(+)
CONTROLLER
IOUT2
DVDD
DRDY
SCLK
DIN
DOUT
CS
XTAL1
XTAL2
RL2
RTD
200A
200A
RL1
Figure 23. 3-Wire RTD Configuration Using the AD7719
REV. A
AD7719
–38–
In this 3-wire configuration, the lead resistances will result in
errors if only one current source is used because the 200 μA will
flow through RL1, developing a voltage error between AIN1 and
AIN2. In the scheme outlined below, the second RTD current
source is used to compensate for the error introduced by the
200 μA flowing through RL1. The second RTD current flows
through RL2. Assuming RL1 and RL2 are equal (the leads
would normally be of the same material and of equal length),
and IOUT1 and IOUT2 match, the error voltage across RL2
equals the error voltage across RL1, and no error voltage is
developed between AIN1 and AIN2. Twice the voltage is
developed across RL3, but since this is a common-mode voltage,
it will not introduce errors. RCM is included so the current
flowing through the combination of RL3 and RCM develops enough
voltage that the analog input voltage seen by the AD7719 is
within the common-mode range of the ADC. The reference
voltage for the AD7719 is also generated using one of these
matched current sources. This reference voltage is developed
across the 12.5 kΩ resistor as shown, and applied to the differential
reference inputs of the AD7719. This scheme ensures that the analog
input voltage span remains ratiometric to the reference voltage.
Any errors in the analog input voltage due to the temperature drift
of the RTD current source is compensated for by the variation
in the reference voltage. The typical drift matching between the
two RTD current sources is less than 1 ppm/°C. The voltage on
either IOUT pin can go to within 0.6 V of the AVDD supply.
Smart Transmitters
Smart transmitters are another key design-in area for the AD7719.
The dual Σ-Δ converter, single-supply operation, 3-wire interface
capabilities, and small package size are all of benefit in smart
transmitters. Here, the entire smart transmitter must operate
from the 4 to 20 mA loop. Tolerances in the loop mean that the
amount of current available to power the transmitter is as low as
3.5 mA. Figure 24 shows a block diagram of a smart transmitter,
which includes the AD7719.
Not shown in Figure 24 is the isolated power source required to
power the front end. The advantages of the AD7719 in these
applications is the dual-channel operation, meaning that the
user does not have to interrupt the main channel when measuring
secondary variables, and therefore does not have the latency
associated with the settling times of the digital filter. The fact
that the AD7719 is factory-calibrated means that in the majority
of applications, the user will not have to perform any field calibration
given the excellent offset and gain drift performance of
the device as a result of the signal chain chopping employed in
the signal chain.
MICROCONTROLLER
REF OUT2
REF IN
10F 0.1F
DVDD AVDD
AIN1
AIN2
CS
DOUT
SCLK
DIN
DGND AGND
AIN5
AIN5 COM
REFIN2
REFIN(+)
REFIN(–)
0.1F
REF OUT1
CLOCK
LATCH
DATA
4.7F
COM
C1 C2 C3
VCC
GND
AD7719
AD421
BOOST VCC LV 0.01F
1k
1000pF
LOOP
POWER
10F
3.3V
1.25V
DN25D
0.01F
LOOP
RTN
COMP
DRIVE
MAIN
VARIABLES
SECONDARY
VARIABLES
AIN3
AIN4
Figure 24. Smart Transmitter Employing the AD7719
REV. A
AD7719
–39–
OUTLINE DIMENSIONS
28-Lead Standard Small Outline Package [SOIC]
Wide Body
(R-28)
Dimensions shown in millimeters and (inches)
CONTROLLING DIMENSIONS ARE IN MILLIMETERS; INCH DIMENSIONS
(IN PARENTHESES) ARE ROUNDED-OFF MILLIMETER EQUIVALENTS FOR
REFERENCE ONLY AND ARE NOT APPROPRIATE FOR USE IN DESIGN
COMPLIANT TO JEDEC STANDARDS MS-013AE
0.32 (0.0126)
0.23 (0.0091)
8
0
0.75 (0.0295)
0.25 (0.0098) 45
1.27 (0.0500)
0.40 (0.0157)
SEATING
PLANE
0.30 (0.0118)
0.10 (0.0039)
0.51 (0.0201)
0.33 (0.0130)
2.65 (0.1043)
2.35 (0.0925)
1.27 (0.0500)
BSC
28 15
1 14
18.10 (0.7126)
17.70 (0.6969)
10.65 (0.4193)
10.00 (0.3937)
7.60 (0.2992)
7.40 (0.2913)
COPLANARITY
0.10
28-Lead Thin Shrink Small Outline Package [TSSOP]
(RU-28)
Dimensions shown in millimeters
4.50
4.40
4.30
28 15
1 14
9.80
9.70
9.60
6.40 BSC
PIN 1
SEATING
PLANE
0.15
0.05
0.30
0.19
0.65
BSC
1.20
MAX
0.20
0.09
0.75
0.60
0.45
8
0
COMPLIANT TO JEDEC STANDARDS MS-153AE
COPLANARITY
0.10
–40–
C02460–0–4/03(A)
REV. A
AD7719
Revision History
Location Page
4/03—Data Sheet changed from REV. 0 to REV. A.
Updated format . . . . . . . . . . . . . . . . . . . . . . . . . . . . . . . . . . . . . . . . . . . . . . . . . . . . . . . . . . . . . . . . . . . . . . . . . . . . . . . . . . .Universal
Changes to SPECIFICATIONS . . . . . . . . . . . . . . . . . . . . . . . . . . . . . . . . . . . . . . . . . . . . . . . . . . . . . . . . . . . . . . . . . . . . . . . . . . . . 3
Updated OUTLINE DIMENSIONS . . . . . . . . . . . . . . . . . . . . . . . . . . . . . . . . . . . . . . . . . . . . . . . . . . . . . . . . . . . . . . . . . . . . . . . 39
Input
Preamp
Ladder
Attenuator
Output Amp
Common Mode
Control
Bandwidth
Limiting
Circuitry
Aux Amp
Overvoltage
Clamp
GND
5,8
VCC
3,4
VCM_Aux
16
Serial Peripheral
Interface
12
VDD
Hi Gain
or
Low Gain
10 Step
2 dB/Step
+IN
-IN
6
7
10 9 11
SDIO CS SCLK
13 VCM
15
14
1
2 -OUT Aux
+OUT Aux
-OUT
+OUT
AUXILIARY
OUTPUT
MAIN OUT
72µ$'&¶
LMH6518
50:
50:
50:
50:
Overvoltage
Clamp
Overvoltage
Clamp
LMH6518
www.ti.com SNOSB21C –MAY 2008–REVISED JULY 2013
LMH6518 900 MHz, Digitally Controlled, Variable Gain Amplifier
Check for Samples: LMH6518
1FEATURES DESCRIPTION
The LMH6518 is a digitally controlled variable gain 2• Gain Range 40 dB
• Gain Step Size 2 dB amplifier whose total gain can be varied from −1.16 dB to 38.8 dB for a 40 dB range in 2 dB steps. The
• Combined Gain Resolution with −3 dB bandwidth is 900 MHz at all gains. Gain
Gsample/Second ADC’s 8.5 mdB accuracy at each setting is typically 0.1 dB. When
• Min Gain −1.16 dB used in conjunction with a Texas Instruments
• Max Gain 38.8 dB Gsample/second (Gsps) ADC with adjustable full scale (FS) range, the LMH6518 gain adjustment will
• −3 dB BW 900 MHz accommodate full scale input signals from 6.8 mVPP
• Rise/Fall Time <500 ps to 920 mVPP to get 700 mVPP nominal at the ADC
• Recovery Time <5 ns input. The Auxiliary output (“+OUT Aux” and “−OUT Aux”) follows the Main output and is intended for use
• Propagation Delay Variation 100 ps in Oscilloscope trigger function circuitry but may have
• HD2 @ 100 MHz −50 dBc other uses in other applications.
• HD3 @ 100 MHz −53 dBc The LMH6518 gain is programmed via a SPI-1
• Input-Referred Noise (Max Gain) 0.98 nV/√Hz compatible serial bus. A signal path combined gain
• Over-Voltage Clamps for Fast Recovery resolution of 8.5 mdB can be achieved when the LMH6518’s gain and the Gsps ADC’s FS input are
• Power Consumption — Auxiliary Turned Off both manipulated. Inputs and outputs are DC-
1.1W0.75W coupled. The outputs are differential with individual
Common Mode (CM) voltage control (for Main and
APPLICATIONS Auxiliary outputs) and have a selectable bandwidth
• Oscilloscope Programmable Gain Amplifier limiting circuitry (common to both Main and Auxiliary) of 20, 100, 200, 350, 650, 750 MHz or full bandwidth.
• Differential ADC Drivers
• High Frequency Single-Ended Input to
Differential Conversion
• Precision Gain Control Applications
• Medical Applications
• RF/IF Applications
Functional Block Diagram
1
Please be aware that an important notice concerning availability, standard warranty, and use in critical applications of
Texas Instruments semiconductor products and disclaimers thereto appears at the end of this data sheet.
2All trademarks are the property of their respective owners.
PRODUCTION DATA information is current as of publication date. Copyright © 2008–2013, Texas Instruments Incorporated Products conform to specifications per the terms of the Texas
Instruments standard warranty. Production processing does not
necessarily include testing of all parameters.
LMH6518
SNOSB21C –MAY 2008–REVISED JULY 2013 www.ti.com
These devices have limited built-in ESD protection. The leads should be shorted together or the device placed in conductive foam
during storage or handling to prevent electrostatic damage to the MOS gates.
Absolute Maximum Ratings (1)(2)
ESD Tolerance (3)
Human Body Model 2000V
Machine Model 200V
Charge Device Model 1000V
Supply Voltage
VCC (5V nominal) 5.5V
VDD (3.3V nominal) 3.6V
Differential Input ±1V
Input Common Mode Voltage 1V to 4V
VCM and VCM_Aux 2V
SPI Inputs 3.6V
Maximum Junction Temperature 150°C
Storage Temperature Range −65°C to 150°C
Soldering Information
Infrared or Convection (20 sec.) 235°C
Wave Soldering (10 sec.) 260°C
(1) Absolute Maximum Ratings indicate limits beyond which damage to the device may occur. Operating Ratings indicate conditions for
which the device is intended to be functional, but specific performance is not ensured. For ensured specifications, see the Electrical
Characteristics tables.
(2) If Military/Aerospace specified devices are required, please contact the Texas Instruments Sales Office/Distributors for availability and
specifications.
(3) Human Body Model, applicable std. MIL-STD-883, Method 3015.7. Machine Model, applicable std. JESD22-A115-A (ESD MM std. of
JEDEC) Field-Induced Charge-Device Model, applicable std. JESD22-C101-C (ESD FICDM std. of JEDEC).
Operating Ratings (1)
Supply Voltage VCC = 5V (±5%)
VDD = 3.3V (±5%)
Temperature Range −40°C to 85°C
(1) Absolute Maximum Ratings indicate limits beyond which damage to the device may occur. Operating Ratings indicate conditions for
which the device is intended to be functional, but specific performance is not ensured. For ensured specifications, see the Electrical
Characteristics tables.
Thermal Properties
Temperature Range (1) −40°C to 85°C
Junction-to-Ambient
Thermal Resistance (θJA), WQFN (1) 40°C/W
(1) The maximum power dissipation is a function of TJ(MAX), θJA and TA. The maximum allowable power dissipation at any ambient
temperature is PD = (TJ(MAX) – TA)/ θJA. All numbers apply for package soldered directly into a 2 layer PC board with zero air flow.
Package should be soldered unto a 6.8 mm2 copper area as shown in the “recommended land pattern” shown in the package drawing.
Electrical Characteristics (1)
Unless otherwise specified, all limits are ensured for TA = 25°C, Input CM = 2.5V, VCM = 1.2V, VCM_Aux = 1.2V, Single-ended
input drive, VCC = 5V, VDD = 3.3V, RL = 100Ω differential (both Main & Auxiliary Outputs), VOUT = 0.7 VPP differential (both
Main & Auxiliary Outputs), both Main and Auxiliary Output Specifications, full bandwidth setting, gain = 18.8 dB (Preamp LG,
0 dB ladder attenuation), Full Power setting (2). Electrical Characteristics Definition of Terms and Specifications for
abbreviations used in the datasheet. Boldface limits apply at the temperature extremes.
(1) Electrical Table values apply only for factory testing conditions at the temperature indicated. Factory testing conditions result in very
limited self-heating of the device such that TJ = TA. No specification of parametric performance is indicated in the electrical tables under
conditions of internal self-heating where TJ > TA.
(2) “Full Power” setting is with Auxiliary output turned on.
2 Submit Documentation Feedback Copyright © 2008–2013, Texas Instruments Incorporated
Product Folder Links: LMH6518
LMH6518
www.ti.com SNOSB21C –MAY 2008–REVISED JULY 2013
Electrical Characteristics (1) (continued)
Unless otherwise specified, all limits are ensured for TA = 25°C, Input CM = 2.5V, VCM = 1.2V, VCM_Aux = 1.2V, Single-ended
input drive, VCC = 5V, VDD = 3.3V, RL = 100Ω differential (both Main & Auxiliary Outputs), VOUT = 0.7 VPP differential (both
Main & Auxiliary Outputs), both Main and Auxiliary Output Specifications, full bandwidth setting, gain = 18.8 dB (Preamp LG,
0 dB ladder attenuation), Full Power setting (2). Electrical Characteristics Definition of Terms and Specifications for
abbreviations used in the datasheet. Boldface limits apply at the temperature extremes.
Symbol Parameter Condition Min(3) Typ(4) Max(3) Units
Dynamic Performance
LSBW −3 dB Bandwidth All Gains 900 MHz
Peaking Peaking All Gains 1 dB
GF_0.1 dB ±0.1 dB Gain Flatness All Gains 150 MHz
GF_1 dB ±1 dB Gain Flatness All Gains 400 MHz
TRS Rise Time 460
ps
TRL Fall Time 450
OS Overshoot Main Output 9 %
ts_1 Settling Time Main Output, ±0.5% 10
ns
ts_2 Main Output, ±0.05% 14
t_recover Recovery Time(5) All Gains <5 ns
PD Propagation Delay VOUT = 0.7 VPP, All Gains 1.2 ns
PD_VAR Propagation Delay Variation Gain Varied 100 ps
Noise, Distortion, and RF Specifications
en_1 Input Noise Spectral Density Max Gain, 10 MHz 0.98 nV/√Hz
en_2 Preamp LG and 0 dB Ladder, 4.1
10 MHz
eno_1 RMS Output Noise Max Gain, 100 Hz to 400 MHz 1.7 mV
eno_2 Preamp LG, 0 dB Ladder, 100 Hz 940 μV
to 400 MHz
NF_1 Noise Figure Max Gain, RS = 50Ω each Input, 3.8
10 MHz
dB
NF_2 Preamp LG, 0 dB Ladder, RS = 50Ω 13.5
each Input, 10 MHz
HD2/ HD3_1 2nd/ 3rd Harmonic Distortion(6) Main Output, 100 MHz, All Gains −50/ −53
HD2/ HD3_2 Auxiliary Output, 100 MHz, All Gains −48/ −50
dBc
HD2/ HD3_3 Main Output, 250 MHz, All Gains −44/ −50
HD2/ HD3_4 Auxiliary Output, 250 MHz, All Gains −42/ −42
IMD3 Intermodulation Distortion (6) f = 250 MHz, Main output −65 dBc
OIP3_1 Intermodulation Intercept (6) Main Output, 250 MHz 26 dBm
P_1dB_main −1 dB Compression Main Output, 250 MHz, 0 dB Ladder 1.8
Main Output, 250 MHz, 20 dB Ladder 1.0
P_1dB_aux Auxiliary Output, 250 MHz, 1.65 VPP 0 dB Ladder
Auxiliary Output, 250 MHz, 1.0
20 dB Ladder
Gain Parameters
AV_DIFF_MAX Max Gain 38.1 38.8 39.5 dB
AV_DIFF_MIN Min Gain −1.91 −1.16 −0.40 dB
(3) Limits are 100% production tested at 25°C unless otherwise specified. Limits over the operating temperature range are ensured through
correlation using Statistical Quality Control (SQC) methods.
(4) Typical values represent the most likely parametric norm as determined at the time of characterization. Actual typical values may vary
over time and will also depend on the application and configuration. The typical values are not tested and are not ensured on shipped
production material.
(5) Recovery time” is the slower of the Main and Auxiliary outputs. Output swing of 700 mVPP shifted up or down by 50% (0.35V) by
introducing an offset. Measured values correspond to the time it takes to return to within ±1% of 0.7 VPP (±7 mV).
(6) Distortion data taken under single ended input condition.
Copyright © 2008–2013, Texas Instruments Incorporated Submit Documentation Feedback 3
Product Folder Links: LMH6518
LMH6518
SNOSB21C –MAY 2008–REVISED JULY 2013 www.ti.com
Electrical Characteristics (1) (continued)
Unless otherwise specified, all limits are ensured for TA = 25°C, Input CM = 2.5V, VCM = 1.2V, VCM_Aux = 1.2V, Single-ended
input drive, VCC = 5V, VDD = 3.3V, RL = 100Ω differential (both Main & Auxiliary Outputs), VOUT = 0.7 VPP differential (both
Main & Auxiliary Outputs), both Main and Auxiliary Output Specifications, full bandwidth setting, gain = 18.8 dB (Preamp LG,
0 dB ladder attenuation), Full Power setting (2). Electrical Characteristics Definition of Terms and Specifications for
abbreviations used in the datasheet. Boldface limits apply at the temperature extremes.
Symbol Parameter Condition Min(3) Typ(4) Max(3) Units
Gain_Step Gain Step Size All Gains including Preamp Step 1.8 2 2.2 dB
Gain Step Size with ADC (See ADC FS Adjusted 8.5 mdB
Applications Information)
Gain_Range Gain Range 39 40 41 dB
TC_AV_DIFF Gain Temp Coefficient (7) All Gains −0.8 mdB/°C
Gain_ACC Absolute Gain Accuracy Compared to theoretical from 0.75 — +0.75 dB
Max Gain in 2 dB steps
Matching
Gain_match Gain Matching Main/Auxiliary All Gains ±0.1 ±0.2 dB
BW_match −3 dB Bandwidth Matching All Gains 5 %
Main/Auxiliary
RT_match Rise Time Matching Main/ Auxiliary All Gains 5 %
PD_match Propagation Delay Matching All Gains 100 ps
Main/Auxiliary
Analog I/O
CMRR_1 CM Rejection Ratio (see Table 1) Preamp HG, 0 dB Ladder, 1.9V < 45 86
CMVR < 3.1V
dB
CMRR_2 Preamp LG, 0 dB Ladder, 1.9V < 40 55
CMVR < 3.1V
CMVR_1 Input Common Mode Voltage Range Preamp HG, All Ladder Steps, CMRR 1.9 — 3.1
≥ 45 dB
V
CMVR_2 Preamp LG, All Ladder Steps, CMRR 1.9 — 3.1
≥ 40 dB
|ΔVO_CM|ΔI_CM| All Gains, 2V < CMVR < 3V −60 −100 dB
CMRR_CM CM Rejection Ratio relative to VCM (see Preamp LG, 0 dB 101 dB
Table 1)
Zin_diff Differential Input Impedance All Gains 150||1.5
Z KΩ || in_CM CM Input impedance Preamp HG 420||1.7 pF
Preamp LG 900||1.7
FSOUT1 Full Scale Voltage Swing Main Output, THD @ 100 MHz ≤ 770(8) 800
−40 dBc, All Gains
FSOUT2 Main Output, Clamped, 0 dB Ladder 1800 1960
FSOUT3 Auxiliary Output, THD @ 100 MHz ≤ 770(8) 800 mVPP
−40 dBc All Gains
FSOUT4 Auxiliary Output, Clamped,0 dB 1600 1760
Ladder
VOUT_MAX1 Voltage range at each output pin Main Output, All gains, VCM = 1.2V 0.5 1.8
V (clamped) OUT_MAX2 Auxiliary Output, All Gains, 0.8 2.2
VCM = 1.2V
V
VOUT_MAX3 Main Output, All Gains, VCM = 1.45V 2.05
VOUT_MAX4 Auxiliary output, All gains, 2.45
VCM = 1.45V
ZOUT_DIFF Differential Output Impedance All Gains 92 100 108 Ω
VOOS Output Offset Voltage All Gains ±15 ±40 mV
VOOS_shift1 Output Offset Voltage Shift Preamp LG to Preamp HG 13.7
mV
VOOS_shift2 All Gains, Excluding Preamp Step 12.7
(7) Drift determined by dividing the change in parameter at temperature extremes by the total temperature change.
(8) Specified by design.
4 Submit Documentation Feedback Copyright © 2008–2013, Texas Instruments Incorporated
Product Folder Links: LMH6518
vO_CM
vOUT
250 MHz,
'VO_CM
'VOUT
DC,
LMH6518
www.ti.com SNOSB21C –MAY 2008–REVISED JULY 2013
Electrical Characteristics (1) (continued)
Unless otherwise specified, all limits are ensured for TA = 25°C, Input CM = 2.5V, VCM = 1.2V, VCM_Aux = 1.2V, Single-ended
input drive, VCC = 5V, VDD = 3.3V, RL = 100Ω differential (both Main & Auxiliary Outputs), VOUT = 0.7 VPP differential (both
Main & Auxiliary Outputs), both Main and Auxiliary Output Specifications, full bandwidth setting, gain = 18.8 dB (Preamp LG,
0 dB ladder attenuation), Full Power setting (2). Electrical Characteristics Definition of Terms and Specifications for
abbreviations used in the datasheet. Boldface limits apply at the temperature extremes.
Symbol Parameter Condition Min(3) Typ(4) Max(3) Units
TCVOOS Output Offset Voltage Drift(9) Preamp HG, 0 dB Ladder −24 μV/°C
Preamp LG, 0 dB Ladder −7
IB Input Bias Current(10) +40 +100 +140 μA
VOCM Output CM Voltage Range All Gains 0.95 1.20 1.45 V
VOS_CM Output CM Offset Voltage All Gains ±15 ±30 mV
TC_VOS_CM CM Offset Voltage Temp Coefficient All Gains +55 μV/°C
BAL_Error_DC Output Gain Balance Error −78
dB
BAL_Error_AC −45
PB Phase Balance Error (See Table 1) 250 MHz ±0.8 deg
PSRR Differential Power Supply Rejection(see Preamp HG, 0 dB Ladder −60 −87
Table 1) dB Preamp LG, 0 dB Ladder −50 −70
PSRR_CM CM Power Supply Rejection(see Preamp LG, 0 dB −55 −71 dB
Table 1)
VCM_I VCM Input Bias Current(11) All Gains ±1 ±10
±20
nA
VCM_AUX_I VCM_AUX Input Bias Current(11) All Gains ±1 ±10
±20
(9) Drift determined by dividing the change in parameter at temperature extremes by the total temperature change.
(10) Positive current is current flowing into the device.
(11) Positive current is current flowing into the device.
Copyright © 2008–2013, Texas Instruments Incorporated Submit Documentation Feedback 5
Product Folder Links: LMH6518
LMH6518
SNOSB21C –MAY 2008–REVISED JULY 2013 www.ti.com
Electrical Characteristics (1) (continued)
Unless otherwise specified, all limits are ensured for TA = 25°C, Input CM = 2.5V, VCM = 1.2V, VCM_Aux = 1.2V, Single-ended
input drive, VCC = 5V, VDD = 3.3V, RL = 100Ω differential (both Main & Auxiliary Outputs), VOUT = 0.7 VPP differential (both
Main & Auxiliary Outputs), both Main and Auxiliary Output Specifications, full bandwidth setting, gain = 18.8 dB (Preamp LG,
0 dB ladder attenuation), Full Power setting (2). Electrical Characteristics Definition of Terms and Specifications for
abbreviations used in the datasheet. Boldface limits apply at the temperature extremes.
Symbol Parameter Condition Min(3) Typ(4) Max(3) Units
Digital I/O & Timing
VIH Input Logic High VDD-0.6 V
VIL Input Logic Low 0.5 V
VOH Output Logic High VDD V
VOL Output Logic Low 0 V
RHi_Z Output Resistance High Impedance Mode 5 MΩ
I_in Input Bias Current <1 μA
FSCLK SCLK Rate 10 MHz
FSCLK_DT SCLK Duty Cyle 45 50 55 %
TS SDIO Setup Time 25 ns
TH SDIO Hold Time 25 ns
TCES CS Enable Setup Time From CS asserted to rising edge of 25 ns
SCLK
tCDS CS Disable Setup Time From CS de-asserted to rising edge 25 ns
of SCLK
TIAG Inter-Acess Gap 3 Cycles
of
SCLK
Power Requirements
IS1 Supply Current VCC 195 210 225
230
mA
IS1_off VCC Aux off 150 165
170
IDD VDD 180 350 400 μA
Bandwidth Limiting Filter Specifications
Filter Parameter Condition Min Typ Max Units
20 MHz Pass Band Tolerance (All Gains) −3 dB Bandwidth −0, +20 %
100 MHz Pass Band Tolerance (All Gains) −3 dB Bandwidth −0, +20 %
200 MHz Pass Band Tolerance (All Gains) −3 dB Bandwidth −0, +20 %
350 MHz Pass Band Tolerance (Preamp LG, 0 dB −3 dB Bandwidth ±10
Ladder) %
Pass Band Tolerance (All Gains) ±25
650 MHz Pass Band Tolerance (Preamp LG, 0 dB −3 dB Bandwidth ±10
Ladder) %
Pass Band Tolerance (All Gains) ±25
750 MHz Pass Band Tolerance (Preamp LG, 0 dB −3 dB Bandwidth ±10
Ladder) %
Pass Band Tolerance (All Gains) ±25
6 Submit Documentation Feedback Copyright © 2008–2013, Texas Instruments Incorporated
Product Folder Links: LMH6518
'VO_CM
'VOUT
LMH6518
www.ti.com SNOSB21C –MAY 2008–REVISED JULY 2013
Table 1. Definition of Terms and Specifications
1. AV_CM (dB) Change in output offset voltage (ΔVOOS) with respect to the change in input common mode
voltage (ΔVI_CM)
2. AV_DIFF (dB) Gain with 100Ω differential load
3. CM Common Mode
4. CMRR (dB) Common Mode rejection defined as: AV_DIFF (dB) - AV_CM (dB)
5. CMRR_CM Common Mode rejection relative to VCM defined as:
ΔVOOS /ΔVCM
6. HG Preamp High Gain
7. Ladder Ladder Attenuator setting (0-20 dB)
8. LG Preamp Low Gain
9. Max Gain Gain = 38.8 dB
10. Min Gain Gain = −1.16 dB
11. +Out Positive Main Output
12. −Out Negative Main Output
13. +Out Aux Positive Auxiliary Output
14. −Out Aux Negative Auxiliary Output
15. PB Phase Balance defined as the phase difference between the complimentary outputs relative to
180°
16. PSRR Input referred VOOS shift divided by change in VCC
17. PSRR_CM Output common mode voltage change (ΔVO_CM) with respect to VCC voltage change (ΔVCC)
18. VCM Input pin voltage that sets Main output CM
19. VCM_Aux Input pin voltage that sets Auxiliary output CM
20. VI_CM Input CM voltage (average of +IN and −IN)
21. ΔVIN (V) Differential voltage across device inputs
22. VOOS DC offset voltage. Differential output voltage measured with inputs shorted together to VCC/2
23. VO_CM Output common mode voltage (DC average of V+OUT and V−OUT)
24. VOS_CM CM offset voltage: VO_CM - VCM
25. ΔVO_CM Variation in output common mode voltage (VO_CM)
26. Balance Error. Measure of the output swing balance of “+OUT” and “−OUT”, as reflected on
the output common mode voltage (VO_CM), relative to the differential output swing (VOUT).
Calculated as output common mode voltage change (ΔVO_CM) divided by the output differential
voltage change (ΔVOUT, which is nominally around 700 mVPP)
27. ΔVOUT Change in differential output voltage (Corrected for DC offset (VOOS))
Copyright © 2008–2013, Texas Instruments Incorporated Submit Documentation Feedback 7
Product Folder Links: LMH6518
-OUT AUX
16
15
14
13
10 11 12
8
7
6
5
4 3 2 1
CS
SDIO
SCLK
VDD
VCM
-OUT
+OUT
VCM_AUX
+OUT AUX
VCC
VCC
GND
+IN
-IN
GND
9
LMH6518
SNOSB21C –MAY 2008–REVISED JULY 2013 www.ti.com
PIN OUT
Pin Out Function
P1 = +OUT Aux Auxiliary positive output
P2 = −OUT Aux Auxiliary negative output
P3 = VCC (5V) Analog power supply
P4 = VCC (5V) Analog power supply
P5 = GND Ground, electrically connected to the WQFN heat sink
P6 = +IN Positive Input
P7 = −IN Negative Input
P8 = GND Ground, electrically connected to the WQFN heat sink
P9 = CS SPI interface, Chip Select, Active low
P10 = SDIO SPI interface, Serial Data Input/Output
P11 = SCLK SPI interface, Clock
P12 = VDD (3.3V) Digital power supply
P13 = VCM Input from ADC to control main output CM
P14 = −OUT Main negative output
P15 = +OUT Main positive output
P16 = VCM_Aux Input to control auxiliary output CM
Connection Diagram
Figure 1. 16-Pin-Top View
See Package Number RGH0016A
8 Submit Documentation Feedback Copyright © 2008–2013, Texas Instruments Incorporated
Product Folder Links: LMH6518
1 10 100 1G
FREQUENCY (MHz)
-25
-20
-15
-10
-5
0
5
NORMALIZED GAIN (dB)
Full BW
750 MHz
650 MHz
350 MHz
200 MHz
100 MHz
20 MHz
Response (HG, 0 dB)
VOUT = 0.1 VPP
10 100 1G
FREQUENCY (MHz)
-6
-5
-4
-3
-2
-1
0
1
2
3
NORMALIZED GAIN (dB)
Full BW HG, 0 dB
LG, 0 dB
HG, 20 dB
LG, 20 dB
1 10 100 1G
FREQUENCY (MHz)
-25
-20
-15
-10
-5
0
5
NORMALIZED GAIN (dB)
Full BW
750 MHz
650 MHz
350 MHz
200 MHz
100 MHz
20 MHz
Response (HG, 0 dB)
1 10 100 1G
FREQUENCY (MHz)
-25
-20
-15
-10
-5
0
5
NORMALIZED GAIN (dB)
Full BW
750 MHz
650 MHz
350 MHz
200 MHz
100 MHz
20 MHz
Response (LG, 0 dB)
VOUT = 0.1 VPP
1 10 100 1G
FREQUENCY (MHz)
-25
-20
-15
-10
-5
0
5
NORMALIZED GAIN (dB)
Full BW
750 MHz
650 MHz
350 MHz
200 MHz
100 MHz
20 MHz
Response (LG, 0 dB)
1 10 100 1G
FREQUENCY (MHz)
-300
-250
-200
-150
-100
-50
0
PHASE (°)
Full BW
20 MHz 750 MHz
100 MHz
200 MHz
350 MHz
650 MHz
Phase (LG, 0 dB)
LMH6518
www.ti.com SNOSB21C –MAY 2008–REVISED JULY 2013
Typical Performance Characteristics
Unless otherwise specified, Input CM = 2.5V, VCM = 1.2V, VCM AUX = 1.2V, Single-ended input drive, VCC = 5V, VDD = 3.3V, RL
= 100Ω differential (both Main & Auxiliary Outputs), VOUT = 0.7 VPP differential (both Main and Auxiliary Outputs), Main output
specification (Auxiliary is labeled “Auxiliary”), full bandwidth setting, gain = 18.8 dB (Preamp LG, 0 dB ladder attenuation), Full
Power setting (1).
Response (LG, 0 dB) Phase (LG, 0 dB)
Figure 2. Figure 3.
Response (HG, 0 dB) Small Signal Response (LG, 0 dB)
Figure 4. Figure 5.
Response
vs.
Small Signal Response (HG, 0 dB) Gain
Figure 6. Figure 7.
(1) “Full Power” setting is with Auxiliary output turned on.
Copyright © 2008–2013, Texas Instruments Incorporated Submit Documentation Feedback 9
Product Folder Links: LMH6518
0 2 4 6 8 10 12 14 16 18 20
-3
-2.5
-2
-1.5
-1
-0.5
0
0.5
NORMALIZED GAIN (dB)
FREQUENCY (MHz)
All Gains
20 MHz Filter
0 50 100 150 200 250 300
FREQUENCY (MHz)
-180
-130
-80
-30
20
PHASE (°)
HG, 0 dB, 10 dB, 20 dB
LG, 0 dB, 10 dB, 20 dB
20 MHz Filter
10 100 1G
FREQUENCY (MHz)
-6
-5
-4
-3
-2
-1
0
1
2
NORMALIZED GAIN (dB)
85°C, HG
25°C, HG 85°C, LG
25°C, LG
-40°C, HG
-40°C, LG
10 dB Ladder
10 100 1G
FREQUENCY (MHz)
-6
-5
-4
-3
-2
-1
0
1
2
NORMALIZED GAIN (dB)
-350
-300
-250
-200
-150
-100
-50
0
50
PHASE (°)
Phase
Gain
Aux, HG
Main, LG
Aux, HG Main, HG
Main, LG
Aux, LG
10 dB Ladder
0 200 400 600 800 1000
-300
-250
-200
-150
-100
-50
0
PHASE (°)
FREQUENCY (MHz)
Full BW
LG, 0 dB
LG, 20 dB
HG, 0 dB
HG, 20 dB
10 100 1G
FREQUENCY (MHz)
-6
-5
-4
-3
-2
-1
0
1
2
NORMALIZED GAIN (dB)
85°C, HG
85°C, LG
25°C, HG
25°C, LG
-40°C, HG
-40°C, LG
10 dB Ladder
LMH6518
SNOSB21C –MAY 2008–REVISED JULY 2013 www.ti.com
Typical Performance Characteristics (continued)
Unless otherwise specified, Input CM = 2.5V, VCM = 1.2V, VCM AUX = 1.2V, Single-ended input drive, VCC = 5V, VDD = 3.3V, RL
= 100Ω differential (both Main & Auxiliary Outputs), VOUT = 0.7 VPP differential (both Main and Auxiliary Outputs), Main output
specification (Auxiliary is labeled “Auxiliary”), full bandwidth setting, gain = 18.8 dB (Preamp LG, 0 dB ladder attenuation), Full
Power setting (1).
Phase
vs.
Gain Response Over Temperature
Figure 8. Figure 9.
Main
vs.
Auxiliary Response Over Temperature Auxiliary Response
Figure 10. Figure 11.
Response Phase
vs. vs.
Gain Gain
Figure 12. Figure 13.
10 Submit Documentation Feedback Copyright © 2008–2013, Texas Instruments Incorporated
Product Folder Links: LMH6518
0 2 4 6 8 10 12 14 16 18 20
0.5
0.7
0.9
1.1
1.3
1.5
1.7
1.9
2.1
2.3
2.5
LADDER ATTENUATION (dB)
INPUT REFERRED (nV/ Hz)
0
20
40
60
80
100
OUTPUT REFERRED (nV/ Hz)
Input Referred
Output Referred
f = 10 MHz
Preamp HG
INPUT REFERRED (nV/ Hz)
OUTPUT REFERRED (nV/ Hz)
0 2 4 6 8 10 12 14 16 18 20
0
2
4
6
8
10
12
14
16
18
LADDER ATTENUATION (dB)
0
5
10
15
20
25
30
35
40
45
Input Referred
Output Referred
f = 10 MHz
Preamp LG
1 10 100 1000
FREQUENCY (MHz)
-15
-10
-5
0
5
10
15
PHASE (°)
0
3
6
9
12
15
18
GROUP DELAY (ns)
Linear Phase Deviation
Group Delay
1 10 100 1G
FREQUENCY (MHz)
-90
-80
-70
-60
-50
-40
-30
-20
-10
GAIN (dB)
Phase
Gain
LG, 0 dB
-7
-6
-5
-4
-3
-2
-1
0
1
PHASE (°)
10 100 1G
-6
-5
-4
-3
-2
-1
0
1
NORMALIZED GAIN (dB)
FREQUENCY (MHz)
650 MHz Filter
LG, 0 dB
HG, 20 dB
HG, 0 dB
LG, 20 dB
0 200 400 600 800 1000
-300
-250
-200
-150
-100
-50
0
PHASE (°)
FREQUENCY (MHz)
650 MHz Filter
LG, 0 dB
LG, 20 dB
HG, 0 dB
HG, 20 dB
LMH6518
www.ti.com SNOSB21C –MAY 2008–REVISED JULY 2013
Typical Performance Characteristics (continued)
Unless otherwise specified, Input CM = 2.5V, VCM = 1.2V, VCM AUX = 1.2V, Single-ended input drive, VCC = 5V, VDD = 3.3V, RL
= 100Ω differential (both Main & Auxiliary Outputs), VOUT = 0.7 VPP differential (both Main and Auxiliary Outputs), Main output
specification (Auxiliary is labeled “Auxiliary”), full bandwidth setting, gain = 18.8 dB (Preamp LG, 0 dB ladder attenuation), Full
Power setting (1).
Response Phase
vs. vs.
Gain Gain
Figure 14. Figure 15.
Balance Error Linear Phase Deviation and Group Delay
Figure 16. Figure 17.
Noise Noise
vs. vs.
Ladder Attenuation Ladder Attenuation
Figure 18. Figure 19.
Copyright © 2008–2013, Texas Instruments Incorporated Submit Documentation Feedback 11
Product Folder Links: LMH6518
0 4 8 12 16 20
-30
-35
-40
-45
-50
-55
-60
-65
-70
HD (dBc)
LADDER ATTENUATION (dB)
500 MHz
10 MHz
100 MHz
20 MHz
250 MHz
50 MHz
HG
0 4 8 12 16 20
-50
-55
-60
-65
-70
-75
-80
-85
HD (dBc)
LADDER ATTENUATION (dB)
LG
10 MHz
20 MHz
50 MHz 100 MHz
250 MHz
500 MHz
1 100 10k 1M
FREQUENCY (kHz)
1
10
100
10 1k 100k
CURRENT NOISE (pA/ Hz)
LG
HG
0 4 8 12 16 20
-30
-35
-40
-45
-50
-55
-60
-65
-70
-75
HD (dBc)
LADDER ATTENUATION (dB)
10 MHz
20 MHz
500 MHz
100 MHz
250 MHz
50 MHz
LG
0 5 10 15 20
0
2
4
8
6
10
12
14
16
18
20
22
24
26
28
LADDER ATTENUATION (dB)
NOISE FIGURE (dB)
f = 10 MHz
RS = 50: on each input
Preamp HG
Preamp LG
1 100 10k 1M
FREQUENCY (kHz)
0
1
10
1000
10 1k 100k
100
VOLTAGE NOISE (nV/ Hz)
HG, 20 dB
LG, 0 dB
HG, 0 dB
LG, 20 dB
LMH6518
SNOSB21C –MAY 2008–REVISED JULY 2013 www.ti.com
Typical Performance Characteristics (continued)
Unless otherwise specified, Input CM = 2.5V, VCM = 1.2V, VCM AUX = 1.2V, Single-ended input drive, VCC = 5V, VDD = 3.3V, RL
= 100Ω differential (both Main & Auxiliary Outputs), VOUT = 0.7 VPP differential (both Main and Auxiliary Outputs), Main output
specification (Auxiliary is labeled “Auxiliary”), full bandwidth setting, gain = 18.8 dB (Preamp LG, 0 dB ladder attenuation), Full
Power setting (1).
Noise Figure Input Voltage Noise
vs. vs.
Gain Frequency
Figure 20. Figure 21.
Input Current Noise HD2
vs. vs.
Frequency Ladder Attenuation
Figure 22. Figure 23.
HD3 HD2
vs. vs.
Ladder Attenuation Ladder Attenuation
Figure 24. Figure 25.
12 Submit Documentation Feedback Copyright © 2008–2013, Texas Instruments Incorporated
Product Folder Links: LMH6518
LADDER ATTENUATION (dB)
0 4 8 12 16
-2
2
6
10
14
18
42
GAIN (dB)
20
22
26
30
34
38
HG -40°C to 85°C
LG
-40°C
HG
0 4 8 12 16 20
-0.15
-0.1
-0.05
0
0.05
0.1
0.15
0.2
0.25
GAIN ACCURACY (dB)
LADDER ATTENUATION (dB)
Relative to HG/0 dB @ 25°C
LG
-40°C
25°C
85°C
25°C
85°C
-7 -6 -5 -4 -3 -2 -1 0
-65
-67
-69
-71
-73
-75
-77
-79
-81
-83
-85
HARMONIC DISTORTION (dBc)
OUTPUT POWER (dBFS)
HG, 10 dB
65 MHz
HD2
HD3
0 4 8 12 16 20
-50
-55
-60
-65
-70
-75
-80
-85
-90
-95
HARMONIC DISTORTION (dBc)
LADDER ATTENUATION (dB)
LG, 65 MHz
Aux
HD3
HD2
Aux
Main
0 4 8 12 16 20
-50
-55
-60
-65
-70
-75
-80
-85
HARMONIC DISTORTION (dBc)
LADDER ATTENUATION (dB)
HG, 65 MHz
HD3
HD2
Main
Aux
Aux
0 4 8 12 16 20
-50
-55
-60
-65
-70
-75
HD (dBc)
LADDER ATTENUATION (dB)
10 MHz
20 MHz
50 MHz
100 MHz
250 MHz
500 MHz
HG
LMH6518
www.ti.com SNOSB21C –MAY 2008–REVISED JULY 2013
Typical Performance Characteristics (continued)
Unless otherwise specified, Input CM = 2.5V, VCM = 1.2V, VCM AUX = 1.2V, Single-ended input drive, VCC = 5V, VDD = 3.3V, RL
= 100Ω differential (both Main & Auxiliary Outputs), VOUT = 0.7 VPP differential (both Main and Auxiliary Outputs), Main output
specification (Auxiliary is labeled “Auxiliary”), full bandwidth setting, gain = 18.8 dB (Preamp LG, 0 dB ladder attenuation), Full
Power setting (1).
HD3
vs.
Ladder Attenuation Main and Auxiliary Distortion Comparison
Figure 26. Figure 27.
Distortion
vs.
Main and Auxiliary Distortion Comparison Output Power
Figure 28. Figure 29.
Gain Gain Accuracy
vs. vs.
Ladder Attenuation Ladder Attenuation
Figure 30. Figure 31.
Copyright © 2008–2013, Texas Instruments Incorporated Submit Documentation Feedback 13
Product Folder Links: LMH6518
1.5 5 2.5 3 3.5
-50
-40
-30
-20
-10
0
10
20
VOOS (mV)
VI_CM
LG, 0 dB
LG, 20 dB
HG, 0 dB
HG, 20 dB
85°C
0 4 8 12 16 20
1.0
1.1
1.2
1.3
1.4
1.5
1.6
1.7
1.8
1.9
2.0
VOUT (VPP)
LADDER ATTENUATION (dB)
LG
HG
Aux, HG
Aux, LG
f = 250 MHz
RL = 100
1.5 5 2.5 3 3.5
-50
-40
-30
-20
-10
0
10
20
VOOS (mV)
VI_CM
LG, 0 dB
LG, 20 dB
HG, 0 dB
HG, 20 dB
-40°C
1.5 5 2.5 3 3.5
-50
-40
-30
-20
-10
0
10
20
VOOS (mV)
VI_CM
LG, 0 dB
LG, 20 dB
HG, 0 dB
HG, 20 dB
25°C
-40°C
0 4 8 12 16 20
-0.15
-0.1
-0.05
0
0.05
0.1
0.15
0.2
0.25
GAIN ACCURACY (dB)
LADDER ATTENUATION (dB)
Relative to HG/0 dB @ 25°C
LG
-40°C
25°C
85°C
25°C
85°C
HG
0 4 8 12 16 20
-0.094
-0.093
-0.092
-0.091
-0.09
-0.089
-0.088
GAIN (dB)
LADDER ATTENUATION (dB)
HG
LG
Aux Gain ± Main Gain
LMH6518
SNOSB21C –MAY 2008–REVISED JULY 2013 www.ti.com
Typical Performance Characteristics (continued)
Unless otherwise specified, Input CM = 2.5V, VCM = 1.2V, VCM AUX = 1.2V, Single-ended input drive, VCC = 5V, VDD = 3.3V, RL
= 100Ω differential (both Main & Auxiliary Outputs), VOUT = 0.7 VPP differential (both Main and Auxiliary Outputs), Main output
specification (Auxiliary is labeled “Auxiliary”), full bandwidth setting, gain = 18.8 dB (Preamp LG, 0 dB ladder attenuation), Full
Power setting (1).
Auxiliary Gain Accuracy Gain Matching
vs. vs.
Ladder Attenuation Ladder Attenuation
Figure 32. Figure 33.
AV_CM AV_CM
Figure 34. Figure 35.
−1 dB Compression
vs.
AV_CM Ladder Attenuation
Figure 36. Figure 37.
14 Submit Documentation Feedback Copyright © 2008–2013, Texas Instruments Incorporated
Product Folder Links: LMH6518
HG
-40°C
LG
0 4 8 12 16 20
-20
-15
-10
-5
0
5
10
15
20
VOOS (mV)
LADDER ATTENUATION (dB)
85°C 25°C
-40°C
LG
0 4 8 12 16 20
-20
-15
-10
-5
0
5
10
15
20
VOOS (mV)
LADDER ATTENUATION (dB)
85°C
25°C HG
TIME (1 ns/DIV)
-0.4
-0.3
-0.2
-0.1
0
0.1
0.2
0.3
0.4
VOUT (V)
HI to LO
LO to HI
HI to LO
LO to HI
Input = 0.2V/DIV
LG, 20 dB
Output
Input
TIME (1 ns/DIV)
-0.4
-0.3
-0.2
-0.1
0
0.1
0.2
0.3
0.4
VOUT (V)
HI to LO
LO to HI
HI to LO
LO to HI
Input = 2 mV/DIV
HG, 0 dB
Output
Input
TIME (1 ns/DIV)
-0.4
-0.3
-0.2
-0.1
0
0.1
0.2
0.3
0.4
VOUT (V)
HI to LO
HI to LO LO to HI
LO to HI
Input = 20 mV/DIV
LG, 0 dB
Output
Input
TIME (1 ns/DIV)
-0.4
-0.3
-0.2
-0.1
0
0.1
0.2
0.3
0.4
VOUT (V)
HI to LO
LO to HI
HI to LO
LO to HI
Input = 20 mV/DIV
HG, 20 dB
Output
Input
LMH6518
www.ti.com SNOSB21C –MAY 2008–REVISED JULY 2013
Typical Performance Characteristics (continued)
Unless otherwise specified, Input CM = 2.5V, VCM = 1.2V, VCM AUX = 1.2V, Single-ended input drive, VCC = 5V, VDD = 3.3V, RL
= 100Ω differential (both Main & Auxiliary Outputs), VOUT = 0.7 VPP differential (both Main and Auxiliary Outputs), Main output
specification (Auxiliary is labeled “Auxiliary”), full bandwidth setting, gain = 18.8 dB (Preamp LG, 0 dB ladder attenuation), Full
Power setting (1).
Step Response Step Response
Figure 38. Figure 39.
Step Response Step Response
Figure 40. Figure 41.
Output Offset Voltage (Typical Unit 1) Output Offset Voltage (Typical Unit 2)
Figure 42. Figure 43.
Copyright © 2008–2013, Texas Instruments Incorporated Submit Documentation Feedback 15
Product Folder Links: LMH6518
85°C
1.5 2 2.5 3 3.5
0
0.02
0.04
0.06
0.08
0.1
0.12
0.14
0.16
0.18
0.2
INPUT BIAS CURRENT (mA)
VI_CM (V)
25°C
-40°C
85°C
4.5 4.7 4.9 5.1 5.3 5.5
1000
1200
1400
1600
1800
2000
2200
2400
AUXILIARY VOLTAGE (mV)
VCC (V)
25°C
-40°C
RL = 100:
VCM_Aux = 1.2V
No CM Load
+OUT Aux and -OUT Aux
4.5 4.7 4.9 5.1 5.3 5.5
195
200
205
210
215
220
225
ICC (mA)
VCC (V)
-40°C
85°C
25°C
2.8 2.9 3 3.1 3.2 3.3 3.4 3.5 3.6
VDD (V)
0.1
0.12
0.14
0.16
0.18
0.2
0.22
IDD (mA)
85°C
25°C
-40°C
85°C
-40°C
LG
0 4 8 12 16 20
-20
-15
-10
-5
0
5
10
15
20
VOOS (mV)
LADDER ATTENUATION (dB)
25°C
HG
0.7 0.9 1.1 1.3 1.5 1.7
-15
-13
-11
-9
-7
-5
-3
-1
1
3
5
VOS_CM (mV)
VCM (V)
85°C
25°C
-40°C
LMH6518
SNOSB21C –MAY 2008–REVISED JULY 2013 www.ti.com
Typical Performance Characteristics (continued)
Unless otherwise specified, Input CM = 2.5V, VCM = 1.2V, VCM AUX = 1.2V, Single-ended input drive, VCC = 5V, VDD = 3.3V, RL
= 100Ω differential (both Main & Auxiliary Outputs), VOUT = 0.7 VPP differential (both Main and Auxiliary Outputs), Main output
specification (Auxiliary is labeled “Auxiliary”), full bandwidth setting, gain = 18.8 dB (Preamp LG, 0 dB ladder attenuation), Full
Power setting (1).
VOS_CM vs.
Output Offset Voltage (Typical Unit 3) VCM
Figure 44. Figure 45.
Supply Current Supply Current
vs. vs.
Supply Voltage Supply Voltage
Figure 46. Figure 47.
Input Bias Current
vs.
Input CM Auxiliary Output Voltage (Hi-Z Mode)
Figure 48. Figure 49.
16 Submit Documentation Feedback Copyright © 2008–2013, Texas Instruments Incorporated
Product Folder Links: LMH6518
0 100 200 300 400 500
TIME (ns)
-1.5
-1.0
-0.5
0
0.5
1.0
1.5
ERROR (%)
0 dB Ladder Attenuation
50% Overdrive
Preamp HG or LG
-10 -6 -2 2 6 10
600
800
1000
1200
1400
1600
1800
OUTPUT VOLTAGE (V)
DELTA-VIN (mV)
+OUT
-OUT
-40°C
85°C
25°C
-40°C
25°C, 85°C
Main or Auxiliary Output
HG, 0 dB
-100 -60 -20 20 60 100
800
900
1000
1100
1200
1300
1400
1500
1600
OUTPUT VOLTAGE (V)
DELTA-VIN (mV)
+OUT
-OUT
-40°C
25°C, 85°C
25°C, 85°C
-40°C
Main or Auxiliary Output
HG, 20 dB
-100 -60 -20 20 60 100
600
800
1000
1200
1400
1600
1800
OUTPUT VOLTAGE (V)
DELTA-VIN (mV)
+OUT
-OUT
-40°C
85°C
25°C
-40°C
25°C, 85°C
Main or Auxiliary Output
LG, 0 dB
-1 -0.6 -0.2 0.2 0.6 1
800
900
1000
1100
1200
1300
1400
1500
1600
OUTPUT VOLTAGE (V)
DELTA-VIN (V)
+OUT
25°C, 85°C -OUT
-40°C
25°C, 85°C
-40°C Main or Auxiliary Output
LG, 20 dB
-5 0 5 10 15 20 25 30 35 40
-10
-5
0
5
10
15
20
25
ERROR from NOMINAL FILTER BW (%)
GAIN (dB)
LG
HG
350 MHz
650 MHz
750 MHz
750 MHz
350 MHz
650 MHz
LMH6518
www.ti.com SNOSB21C –MAY 2008–REVISED JULY 2013
Typical Performance Characteristics (continued)
Unless otherwise specified, Input CM = 2.5V, VCM = 1.2V, VCM AUX = 1.2V, Single-ended input drive, VCC = 5V, VDD = 3.3V, RL
= 100Ω differential (both Main & Auxiliary Outputs), VOUT = 0.7 VPP differential (both Main and Auxiliary Outputs), Main output
specification (Auxiliary is labeled “Auxiliary”), full bandwidth setting, gain = 18.8 dB (Preamp LG, 0 dB ladder attenuation), Full
Power setting (1).
Filter BW Output
vs. vs.
Gain Input
Figure 50. Figure 51.
Output Output
vs. vs.
Input Input
Figure 52. Figure 53.
Output
vs.
Input Overdrive Recovery Time (Return to Zero)
Figure 54. Figure 55.
Copyright © 2008–2013, Texas Instruments Incorporated Submit Documentation Feedback 17
Product Folder Links: LMH6518
0 100 200 300 400 500
TIME (ns)
-1.5
-1.0
-0.5
0
0.5
1.0
1.5
ERROR (%)
20 dB Ladder Attenuation
50% Overdrive
Preamp HG or LG
LMH6518
SNOSB21C –MAY 2008–REVISED JULY 2013 www.ti.com
Typical Performance Characteristics (continued)
Unless otherwise specified, Input CM = 2.5V, VCM = 1.2V, VCM AUX = 1.2V, Single-ended input drive, VCC = 5V, VDD = 3.3V, RL
= 100Ω differential (both Main & Auxiliary Outputs), VOUT = 0.7 VPP differential (both Main and Auxiliary Outputs), Main output
specification (Auxiliary is labeled “Auxiliary”), full bandwidth setting, gain = 18.8 dB (Preamp LG, 0 dB ladder attenuation), Full
Power setting (1).
Overdrive Recovery Time (Return to Zero)
Figure 56.
18 Submit Documentation Feedback Copyright © 2008–2013, Texas Instruments Incorporated
Product Folder Links: LMH6518
) to +1.41 dB
700 mV
(= 20 x log
805 mV
)
700 mV
(= 20 x log
595 mV
= 8.5 mdB
0.56 ±
2 x 512
0.84 ± 0.56
0.56 +
2 x 512
0.84 ± 0.56
¨
¨
©
§
¨ ¨
©
§
¨ ¨
©
§
¨ ¨
©
§
Gain Resolution = 20 log
= 42.6 dB)
920 mVPP
(20 x log
6.8 mVPP
Ladder Attenuator
10 Steps, 2 dB/ Step
0 to -20 dB
Pre-amp
10 dB or 30 dB
Output
Amp
8.86 dB
+Out
-Out
+In
-In
50:
50:
LMH6518
www.ti.com SNOSB21C –MAY 2008–REVISED JULY 2013
APPLICATIONS INFORMATION
FUNCTIONAL DESCRIPTION AND DYNAMIC RANGE IN OSCILLOSCOPE APPLICATIONS
Here is a block diagram of the LMH6518’s Main Output signal path:
Figure 57. LMH6518 Signal Path Block Diagram
The Auxiliary output (not shown) uses another but similar Output Amp that taps into the Ladder Attenuator
output. In this document, Preamp gain of 30 dB is referred to as “Preamp HG” (High Gain) and Preamp gain of
10 dB as “Preamp LG” (Low Gain).
The LMH6518’s 2 dB/step gain resolution and 40 dB adjustment range (from −1.16 dB to 38.8 dB) allows this
device to be used with the TI GSample/second ADCs which have Full Scale, FS, adjustment (through their
Extended Control Mode or ECM) to provide near-continuous variability (8.5 mdB resolution) to cover a 42.6 dB
(1)
FS input range. The Texas Instruments GSample/second ECM control allows the ADC FS to be set using the
ADC SPI bus. The ADC FS voltage range is from 560 mV to 840 mV with 9 bits of FS voltage control.
The ADC ECM gain resolution can be calculated as follows:
(2)
The recommended ADC FS operating range is, however, narrower and it is from 595 mV to 805 mV with 700
mVPP as the mid-point. Raising the value of ADC FS voltage is tantamount to reducing the signal path gain to
accommodate a larger input and vice versa, thus providing a method of gain fine-adjust. The ADC ECM gain
adjustment is −1.21 dB
(3)
Because the ADC FS fine-adjust range of 2.62 dB (= 1.41 dB + 1.21 dB) is larger than the LMH6518’s 2 dB/step
resolution, there is always at least one LMH6518 gain setting to accommodate any FS signal from 6.8 mVPP to
920 mVPP, at the LMH6518 input, with 0.62 dB (= 2.62-2) overlap.
Copyright © 2008–2013, Texas Instruments Incorporated Submit Documentation Feedback 19
Product Folder Links: LMH6518
(20 x log = 3.5 dB)
200 MHz
450 MHz
Attenuation (dB) = 20 x log
FSMAX (VPP)
800 mVPP
Maximum LMH6518 FS Input =
0.7 VPP
10
(-1.16 ± 1.21) dB
20
= 920 mVPP
Minimum LMH6518 FS Input =
0.7 VPP
10
(38.8 + 1.41) dB
20
= 6.8 mVPP
LMH6518
SNOSB21C –MAY 2008–REVISED JULY 2013 www.ti.com
Assuming a nominal 0.7VPP output, the LMH6518’s minimum FS input swing is limited by the maximum signal
path gain possible and vice versa:
(4)
(or 8 mVPP with no ADC fine adjust)
(5)
(or 800 mVPP with no ADC FS adjust)
To accommodate a higher FS input, an additional attenuator is needed before the LMH6518. This front-end
attenuator is shown in the Figure 62 with its details shown in Figure 71. The highest minimum attenuation level is
determined by the largest FS input signal (FSmax):
(6)
So, to accommodate 80 VPP, 40 dB minimum attenuation is needed before the LMH6518.
In a typical oscilloscope application, the voltage range encountered is from 1 mV/DIV to 10 V/DIV with 8 vertical
divisions visible on the screen. One of the primary concerns in a digital oscilloscope is SNR which translates to
display trace width/ thickness. Typically, oscilloscope manufacturers need the noise level to be low enough so
that the “no-input” visible trace width is less than 1% of FS. Experience has shown that this corresponds to a
minimum SNR of 52 dB.
The factors that influence SNR are:
• Scope front end noise (Front-end attenuator + scope probe Hi-Z buffer which is discussed later in this
document and shown in Figure 62)
• LMH6518
• ADC
LMH6518 related SNR factors are:
• Bandwidth
• Preamp used (Preamp High Gain or Low Gain)
• Ladder Attenuation
• Signal level
SNR increases with the inverse square root of the bandwidth. So, reducing bandwidth from 450 MHz to 200
MHz, for example, improves SNR by 3.5 dB
(7)
The other factors listed above, preamp and ladder attenuation, depend on the signal level and also impact SNR.
The combined effect of these factors is summarized in Figure 58 where SNR is plotted as a function of the
LMH6518 FS input voltage (assuming scope bandwidth of 200 MHz) and not including the ADC and the front end
noise:
20 Submit Documentation Feedback Copyright © 2008–2013, Texas Instruments Incorporated
Product Folder Links: LMH6518
100x (= )
80 VPP
0.8 VPP
)
0.8 VPP
24 mVPP
(= 20 x log
0.001 1
INPUT FS (V)
38
46
54
62
SNR (dBFS)
0.01 0.1
58
50
42
40
44
48
52
56
60
6
14
18
10
2
0
4
8
12
16
20
LADDER ATTENUATION (dB)
Preamp HG
Preamp LG
200 MHz Filter
22
-2
LMH6518
www.ti.com SNOSB21C –MAY 2008–REVISED JULY 2013
Figure 58. LMH6518 SNR & Ladder Attenuation used vs. Input
As can be seen from Figure 58, SNR of at least 52 dB is maintained for FS inputs above 24 mVPP (3 mV/DIV on
a scope) assuming the LMH6518’s internal 200 MHz filter is enabled. Most oscilloscope manufacturers relax the
SNR specifications to 40 dB for the highest gain (lowest scope voltage setting). From Figure 58, LMH6518’s
minimum SNR is 43.5 dB, thereby meeting the relaxed SNR specification for the lower range of scope front panel
voltages.
In Figure 58, the step-change in SNR near Input FS of 90 mVPP is the transition point from Preamp LG to
Preamp HG with a subsequent 3 dB difference due to the Preamp HG/ 20 dB ladder attenuation’s lower output
noise compared to Preamp LG/ 2 dB ladder attenuation’s noise. Judicious choice of front end attenuators can
ensure that the 52 dB SNR specification is maintained for scope FS inputs ≥ 24 mVPP by confining the LMH6518
gain range to the lower 30.5 dB
(8)
from the total range of 40 dB (= 38.8 - (−1.16)) possible.
Here is an example:
To cover the range of 1 mV/DIV to 10 V/DIV (80 dB range), here is a configuration which affords good SNR:
Table 2. Oscilloscope Example Including Front-End Attenuators
Row Scope FS Input “S”, Scope Vertical Preamp Ladder Attenuation “A”, Front-end Minimum SNR (dB)
(VPP) Scale (V/DIV) Range (dB) attenuation (V/V) with 200 MHz filter
1 8m-24m 1m-3m HG 0-10 1 44
2 24m-80m 3m-10m HG 10-20 1 52.0
3 80m-0.8 10m-0.1 LG 0-20 1 53.4
4 0.8-8 0.1-1 LG 0-20 10 53.4
5 8-80 1-10 LG 0-20 100 53.4
In Table 2, the highest FS input in Row 5, Column 2 (80 VPP), and the LMH6518’s highest FS input allowed (0.8
VPP) set the
(9)
front-end attenuator value. The 100x attenuator will allow high SNR operation to 30.5 dB down, as explained
earlier, or 2.4 VPP at scope input. In that same table, Rows 1-3 with no front-end attenuation (1x) cover the scope
FS input range from 8 mVPP-800 mVPP. That leaves the scope FS input range of 0.8 VPP-2.4 VPP. If the 100x
attenuator were used for the entire scope FS range of 0.8 VPP-80 VPP, SNR would dip below 52 dB for a portion
of that range. Another attenuation level is thus required to maintain the SNR specification requirement of 52 dB.
Copyright © 2008–2013, Texas Instruments Incorporated Submit Documentation Feedback 21
Product Folder Links: LMH6518
x 1.05 x 1020
G
FSE =
S x 8
A
10
110 mV
oK = -21.6 + 20 x log = 17.57 dB
K = 20 x log =
0.95 x 700 mVPP
8 x S(V/div)
A
-21.6 + 20 x log
A
S(V/div)
LMH6518
SNOSB21C –MAY 2008–REVISED JULY 2013 www.ti.com
One possible attenuation partitioning is to select the additional attenuator value to cover a 20 dB range above 0.8
VPP FS (to 8 VPP) with the 100x attenuator covering the remaining 20 dB range from 8 VPP to 80 VPP. Mapping 8
VPP FS scope input to 0.8 VPP at LMH6518 input means the additional attenuator is 10x, as shown in Table 2,
Row 4. The remaining scope input range of 8 VPP-80 VPP would then be covered by the 100x front-end
attenuator derived earlier. The entire scope input range is now covered with SNR maintained about 52 dB for
scope FS input ≥ 24 mVPP, as shown in Table 2.
SETTINGS AND ADC SPI CODE (ECM)
Covering the range from 1 mV/DIV to 10 V/DIV requires the following to be adjusted within the digital
oscilloscope:
• Front-End Attenuator
• LMH6518 Preamp
• LMH6518 Ladder Attenuation
• ADC FS Value (ECM)
The LMH6518 Product Folder contains a spreadsheet which allows one to calculate the front-end attenuator,
LMH6518 Preamp gain (HG or LG) and ladder attenuation, and ADC FS setting based on the scope vertical
scale (S in V/DIV).
Here is the step by step procedure that explains the operations performed by the said spreadsheet based on the
scope vertical scale setting (S in V/div) and front-end attenuation “A” (from Table 2). A numerical example is also
worked out for more clarification:
1. Determine the required signal path gain, K:
(10)
assuming the full scale signal occupies 95% of the 0.7 VPP FS (for 5% overhead) which occupies 8 vertical
scope divisions).
Required condition: −2.37 dB ≤ K ≤ 40.3 dB
Example: With S = 110 mV/DIV, Table 2 shows that A = 10 V/V:
(11)
2. Determine the LMH6518 gain, G:
– G is the closest LMH6518 gain, to the value of K where:
– G = (38.8 – 2n)dB; n = 0, 1, 2, …, 20
– For this example, the closest G to K = 17.57 dB is 16.8 dB (with n = 11). The next LMH6518 gain, 18.8
dB (with n = 10) would be incorrect as 16.8 is closer. If 18.8 dB were mistakenly chosen, the ADC FS
setting would be out of range.
– Therefore: G = 16.8 dB
3. Determine Preamp (HG or LG) & Ladder Attenuation:
– If G ≥ 18.8 dB → Preamp is HG and Ladder Attenuation = 38.8 - G
– If G < 18.8 dB → Preamp is LG and Ladder Attenuation = 18.8 - G
– For this example, with G = 16.8 → Preamp LG and Ladder Attenuation = 2 dB (= 18.8-16.8).
4. Determine the required ADC FS voltage, FSE:
(12)
22 Submit Documentation Feedback Copyright © 2008–2013, Texas Instruments Incorporated
Product Folder Links: LMH6518
ECM (ratio) =
0.6393 - 0.56
0.28
= 0.283
ECM (ratio) =
FSE - 0.56
0.28
16.8
FSE = = 639.3 mV
S x 8
10
x 1.05 x 1020
LMH6518
www.ti.com SNOSB21C –MAY 2008–REVISED JULY 2013
The “1.05” factor is to add 5% FS overhead margin to avoid ADC overdrive.
(13)
Required condition: 0.56V ≤ FSE ≤ 0.84V
Recommend condition: 0.595V ≤ FSE ≤ 0.805V for optimum ADC FS
5. Determine the ADC ECM code ratio:
where
• 0.28V= (0.84-0.56)V
• 0.56V is the lower end of the ADC FS adjustability
• For this example:
(14)
– Required condition: 0 ≤ ECM (ratio) ≤ 1
6. Determine the ECM binary code to be sent on ADC SPI bus:
– Convert the ECM value represented by the ratio calculated above, to binary:
– ECM (binary) = DEC2BIN{ECM(ratio)* 511, 9}
– Where “DEC2BIN” is a spreadsheet function which converts the decimal ECM ratio, from step 5 above,
multiplied by 511 distinct levels, into binary 9 bits.
NOTE
The Web based spreadsheet computes ECM without the use of “DEC2BIN” function to
ease usage by all spreadsheet users who may not have this function installed.
– For this example: ECM (binary) = DEC2BIN(0.283*511, 9) = 010010000. This would be the number to be
sent to the ADC on the SPI bus to program the ADC to the proper FS voltage.
INPUT/OUTPUT CONSIDERATIONS
The LMH6518’s ideal Input/Output Conditions, considered individually, are listed below:
Table 3. LMH6518's Ideal Input/Output Conditions
Impedance from Common Mode Differential Input Load Impedance (Ω) Differential Output Common Mode
each input to Input (V) (VPP) (V) Output (V)
ground (Ω)
≤50 1.5 to 3.1 <0.8 100 (differential)/ 50 <0.77 0.95-1.45
(single ended)
In addition to the individual conditions listed in Table 3, the Input/Output terminal conditions should match
differentially (i.e. +IN to −IN and +OUT to −OUT), as well, for best performance.
The input is differential but can be driven single-ended as long as the conditions of Table 3 are met and there is
good matching between the driven and the undriven inputs from DC to the highest frequency of interest. If not,
there could be a settling time impact among other possible performance degradations. The datasheet
specifications are with single-ended input, unless specified. Here is the recommended bench-test schematic to
drive one input and to bias the other input with good matching in mind:
Copyright © 2008–2013, Texas Instruments Incorporated Submit Documentation Feedback 23
Product Folder Links: LMH6518
LMH6518
-IN
+IN
R
63.4
2
W
R
82.5
3
W
J1
Input (from 50W source)
(Ground Referenced)
+5V
R
76.8
5
W
R
76.8
4
W
R
63.4
1
W
V
(-3.3V)
EE
LMH6518
-IN
+IN
C1
1 nF
R1
100:
R3
24.9:
R2
R5 49.9:
200:
R4
200:
J1
Input (from 50: source)
(2.5V CM)
+5V
C2
1 nF
LMH6518
SNOSB21C –MAY 2008–REVISED JULY 2013 www.ti.com
Figure 59. Recommended Single-Ended Bench-Test Input Drive from 50Ω Source
With the schematic of Figure 59, each LMH6518 input sees 25Ω to ground at the higher frequencies when the
capacitors look like shorts. This impedance increases to 125Ω at DC for both inputs, thereby preserving the
required matching at any frequency. This configuration, using properly selected R’s and C’s, allows four times
less biasing power dissipation than when the undriven input is biased with an effective 25Ω from the LMH6518
input to ground.
It is possible to drive the LMH6518 input from a ground referenced 50Ω source by providing level shift circuitry
on the driven input. Figure 60 shows a circuit where ½ the input signal reaches the LMH6518 input while the
negative supply voltage (VEE) ensures that the 50Ω source at J1 does not experience any biasing current while
providing 50Ω termination to the source. The driven input (+IN) is biased to 2.5V (VCC/2):
Figure 60. LMH6518 Driven by a Ground Referenced Source
In the schematic of Figure 60, the equivalent impedance from each LMH6518 input to ground is around 38Ω.
This configuration’s power consumption of ∼0.5W (in R1 - R5) is higher than that of Figure 59 because of
additional power dissipated to perform the level shifting. Additional 50Ω attenuators can be placed between J1
and R2/R3 junction in Figure 60 in order to accommodate higher input voltages.
It is also possible to shift the LMH6518 output common mode level using a level shift approach similar to that of
Figure 60. The circuit in Figure 61 shows an implementation where the LMH6518’s nominal 1.2V CM output, set
by a 1.2V on VCM input from the Gsample/s ADC, is shifted lower for proper interface to different ADC's which
require VCM = 0V and have high input impedance:
24 Submit Documentation Feedback Copyright © 2008–2013, Texas Instruments Incorporated
Product Folder Links: LMH6518
50:
50:
VOUT
-5V
R1
0.35 VPP, 0V DC
+5V
Vx R2
+5V -5V
R3
R3
131.3:
R1
172.7:
R2
41.4:
0.7 VPP, 1.2V DC
0.43 VPP, 1.2V DC
+OUT
-OUT
To ADC
LMH6518
LMH6518
www.ti.com SNOSB21C –MAY 2008–REVISED JULY 2013
Figure 61. Output CM Shift Scheme
With the scheme of Figure 61, Vx is kept at 1.2V, by proper selection of external resistor values, so that the
LMH6518 outputs are not CM-loaded. As was the case with input level shifting, this output level shifting also
consumes additional power (0.58W).
Output Swing, Clamping, and Operation Beyond Full Scale
One of the major concerns in interfacing to low voltage ADC’s (such as the Gsample/s ADC’s that the LMH6518
is intended to drive) is ensuring that the ADC input is not violated with excessive drive. For this reason, plus the
very important requirement of an oscilloscope to recover quickly and gracefully from an overdrive condition, the
LMH6518 is fitted with three overvoltage clamps; one at the Preamp output and one at Main and Auxiliary
outputs each. The Preamp clamp is responsible for preventing the Preamp from saturation (to minimize recovery
time) with large ladder attenuation when Preamp output swing is at its highest. On the other hand, the output
clamps, perform this function when the Ladder attenuation is lower and hence the output amplifier is closer to
saturation, and prolonged recovery, if not properly clamped. The combination of these clamps results in
Figure 51, Figure 52, Figure 53, and Figure 54 where it is possible to observe where output limiting starts due to
the clamp action. LMH6518 owes its fast recovery time (< 5 ns) from 50% overdrive to the said clamps.
Figure 51, Figure 52, Figure 53, and Figure 54, in Typical Performance Characteristics, can be used to determine
the LMH6518 linear swing beyond full scale. This information sets the overdrive limit for both oscilloscope
waveform capture and for signal triggering. The Preamp clamp is set tighter than the output clamp, evidenced by
lower output swing with 20 dB Ladder attenuation than with 0 dB. With high ladder attenuation (20 dB) defining
the limit, the graphs show that the “+Out” and “−Out” difference of 0.4V is well inside the clamp range, thereby
ensuring 0.8 VPP of unhindered output swing. This corresponds to an overdrive capability of approximately ±7%
beyond full scale.
Copyright © 2008–2013, Texas Instruments Incorporated Submit Documentation Feedback 25
Product Folder Links: LMH6518
Switch > J1
Oscilloscope
Input
900 k:
90 k:
50: 10 k:
LNA
FPGA
or
MPU
DAC
+IN
-IN
SPI
+OUT
-OUT
VCM
+OUT Aux
-OUT Aux
VCM_Aux
LMH6518
U1
Trigger
Circuit
+IN 1
-IN 1
VCMO
Gsample/sec
8-Bit ADC
SPI (Full Scale
Voltage Control)
Attenuation = 100x
Channel 1
JFET Lo-Noise
Amp
Attenuation = 1x
Attenuation = 10x
Fine Gain Adjust
VCC
Hi-Z/50:
Switch
Attenuator
Block
VCC
200:
1 nF 200:
LMH6518
SNOSB21C –MAY 2008–REVISED JULY 2013 www.ti.com
Here is a block diagram for how the LMH6518 is used in an oscilloscope:
Figure 62. Digital Oscilloscope Front-End
From Figure 62, the signal path consists of the input impedance switch, the attenuator switch, Low Noise
Amplifier (LNA, JFET amplifier) to drive the LMH6518 input (+IN), and the DAC to provide offset adjust. The LNA
must have the following characteristics:
• Set U1’s common mode level to VCC/2 (∼2.5V)
• Very low drift (1 mV shift at LNA output could translate into 88 mV shift at LMH6518 output at max gain, or
∼13% of FS).
• Low output impedance (≤ 50Ω) to drive U1, for good settling behavior
• Low Noise (<0.98 nV/√Hz) to reduce the impact on the LMH6518 Noise Figure. Note that Figure 62 does not
show the necessary capacitors across the resistors in the front-end attenuators (see Figure 71). These
capacitors provide frequency response compensation and limit the noise contribution from the resistors so
that they do not impact the signal path noise. For more information about front-end attenuator design,
including frequency compensation, see REFERENCE for additional resources.
• Gain of 1 V/V (or very close to 1 V/V)
• Excellent frequency response flatness from DC to > 500-800 MHz to not impact the time domain performance
The undriven input (−IN) is biased to VCC/2 using a voltage driver. The impedance driving the LMH6518’s −IN
should be closely matched to the LNA’s output impedance for good settling time performance.
APPENDIX A shows one possible implementation of the LNA buffer along with performance data.
When the LMH6518’s Auxiliary output is not used, it is possible to disable this output using SPI-1 (see LOGIC
FUNCTIONS for SPI register map). Electrical Characteristics shows that by doing so, device power dissipation
decreases by the reduction in supply current of about 60 mA. As can be seen in Figure 63, in the absence of
heavy common loading, the Auxiliary output will be at a voltage close to 1.7V (VCC = 5V). With higher supply
voltages, the Auxiliary voltage will also increase and it is important to make sure any circuitry tied to this output is
capable of handling the 2.3V possible under VCC worst case condition of 5.5V.
26 Submit Documentation Feedback Copyright © 2008–2013, Texas Instruments Incorporated
Product Folder Links: LMH6518
85°C
4.5 4.7 4.9 5.1 5.3 5.5
1000
1200
1400
1600
1800
2000
2200
2400
AUXILIARY VOLTAGE (mV)
VCC (V)
25°C
-40°C
RL = 100:
VCM_Aux = 1.2V
No CM Load
+OUT Aux and -OUT Aux
LMH6518
www.ti.com SNOSB21C –MAY 2008–REVISED JULY 2013
Figure 63. Auxiliary Output Voltage as a Function of VCC
LOGIC FUNCTIONS
The following LMH6518 functions are controlled using the SPI-1 compatible bus:
• Filters (20, 100, 200, 350, 650, 750 MHz or full bandwidth)
• Power Mode (Full Power or Auxiliary Hi-Z (high impedance)
• Preamp (HG or LG)
• Attenuation Ladder (0-20 dB, 10 states)
• LMH6518 state “Write” or “Read” back
The SPI-1 bus uses 3.3V logic. “SDIO” is the serial digital input-output which can write to the LMH6518 or read
back from it. “SCLK” is the bus clock with chip select function controlled by “CS”
SPI-1 PIN DESCRIPTIONS
Pin Name Type Function and Connection
CS Input Serial Chip Select: While this signal is asserted SCLK is used to accept serial data
present on SDIO and to source serial data on SDIO. When this signal is de-asserted,
SDIO is ignored and SDIO is in TRI-STATE mode.
SCLK Input Serial Clock: Serial data are shifted into and out of the device synchronous with this
clock signal. SCLK transitions with CS de-asserted are ignored. SCLK to be stopped
when not needed to minimize digital crosstalk.
SDIO Input-Output Serial Data-In or Data-out: Serial data are shifted into the device (8 bit Command and 16
bit Data) on this pin while CS signal is asserted during Write operation. Serial data are
shifted out of the device on this pin during a read operation while CS signal is asserted.
At other times, and after one complete Access Cycle (24 bits, see Figure 64 and
Figure 65), this input is ignored. This output is in TRI-STATE mode when CS is deasserted.
This pin is bi-directional.
Copyright © 2008–2013, Texas Instruments Incorporated Submit Documentation Feedback 27
Product Folder Links: LMH6518
SCLK
Valid
SDIO Data
Tsu
Th
SCLK
Valid
Data
Valid
Data
Tod
SDIO
Inter-Access Gap
XXX 0 X X X X X X X XXX
C7 C6 C5 C4 C3 C2 C1 C0
1 2 3 4
Command Field Data Field
16 bits LMH6518 Bus
in Tri-State
MSB LSB
DI5 D1 D0
8 9 24 25
Single Access Cycle
SCLK
LMH6518 Bus
in Tri-State
SDIO
CS
Inter-Access Gap
XXX 1 X X X X X X X XXX
C7 C6 C5 C4 C3 C2 C1 C0
1 2
Command Field Data Field
MSB 16 bits LSB
D15 D1 D0
8 9 24 25
Single Access Cycle
SCLK
LMH6518 Bus
in Tri-State
SDIO
CS
3 4
LMH6518 Bus
in Tri-State
LMH6518
SNOSB21C –MAY 2008–REVISED JULY 2013 www.ti.com
Figure 64. Serial Interface Protocol- Read Operation
Figure 65. Serial Interface Protocol- Write Operation
Figure 66. Read Timing
Figure 67. Write Timing
28 Submit Documentation Feedback Copyright © 2008–2013, Texas Instruments Incorporated
Product Folder Links: LMH6518
LMH6518
www.ti.com SNOSB21C –MAY 2008–REVISED JULY 2013
Table 4. Data Field
Filter Pre-amp Ladder Attenuation
D15 D14 D13 D12 D11 D10 D9 D8 D7 D6 D5 D4 D3 D2 D1 D0
(MSB) (LSB)
X 0 0 0 0 0=Full Power 0 See Table 6 0 0=LG See Table 7
1=Aux Hi-Z 1=HG
NOTE
Bits D5, D9, D11-D14 must be “0”. Otherwise, device operation is undefined and
specifications are not ensured.
Table 5. Default Power-On Reset Condition
Filter Pre-amp Ladder Attenuation
D15 D14 D13 D12 D11 D10 D9 D8 D7 D6 D5 D4 D3 D2 D1 D0
(MSB) (LSB)
0 0 0 0 0 0 0 0 0 0 0 0 1 0 1 0
Table 6. Filer Selection Data Field
Filter Filter BW
D8 D7 D6 (MHz)
0 0 0 Full
0 0 1 20
0 1 0 100
0 1 1 200
1 0 0 350
1 0 1 650
1 1 0 750
1 1 1 Unallowed
NOTE
All filters are low pass single pole roll-off and operate on both Main and Auxiliary outputs.
These filters are intended as signal path bandwidth and/ or noise limiting.
Table 7. Ladder Attenuation Data Field
Ladder Attenuation Ladder Attenuation (dB)
D3 D2 D1 D0
0 0 0 0 0
0 0 0 1 −2
0 0 1 0 −4
0 0 1 1 −6
0 1 0 0 −8
0 1 0 1 −10
0 1 1 0 −12
0 1 1 1 −14
1 0 0 0 −16
1 0 0 1 −18
1 0 1 0 −20
1 0 1 1 Unallowed
1 1 0 0 Unallowed
1 1 0 1 Unallowed
1 1 1 0 Unallowed
Copyright © 2008–2013, Texas Instruments Incorporated Submit Documentation Feedback 29
Product Folder Links: LMH6518
x 100°C = 1.45 mV {5.9 LSB)
PV
(9.5 mV + 50
°C
)
2.5V
(=
210
LMH6518
SNOSB21C –MAY 2008–REVISED JULY 2013 www.ti.com
Table 7. Ladder Attenuation Data Field (continued)
Ladder Attenuation Ladder Attenuation (dB)
1 1 1 1 Unallowed
NOTE
An “Unallowed” SPI-1 state may result in undefined operation where device behavior is
not ensured.
OSCILLOSCOPE TRIGGER APPLICATIONS
With the Auxiliary output of the LMH6518 offering a second output that follows the Main one (except for a slightly
reduced distortion performance), the oscilloscope trigger function can be implemented by tapping this output. The
“VCM_Aux” input of the LMH6518 allows the Auxiliary common mode to be set. The trigger function can be
physically located at a distance from the main signal path, if need be, by taking advantage of the differential
Auxiliary output and rejecting any board related common mode interference pick-up at the receive end.
If Trigger circuitry is physically close to the LMH6518, the circuit diagram shown in Figure 68 allows operation
using only one of two Auxiliary outputs. The unused output does need to be terminated properly using R1, R11
combination. U3 (DAC101C085) generates a 0- 2.5V trigger level, with 2.4 mV resolution
(15)
or 0.7% (= 2.4 mV x 100/0.35 VPP) of FS, which is compared to the LMH6518 “+Out Aux” by using an ultra-fast
comparator, U2 (LMH7220). U2’s complimentary LVDS output is terminated in the required 100Ω load (R10), for
best performance, where the LVDS Trigger output is available. The LMH7220’s offset voltage (±9.5 mV) and
offset voltage drift (±50 μV/°C) error will be 5.9 LSB
(16)
of the Trigger DAC (U3). The offset voltage related portion of this error can be nulled-out, if necessary, during the
oscilloscope initial calibration. To do so, the LMH6518 input is terminated properly with no input applied and U3
output is adjusted around VCM_Aux voltage (1.2V ±10 mV) while looking for U2’s output transition. U3’s output,
relative to VCM_Aux at transition corresponds to U2’s offset error which can be factored into the Trigger readings
and thus eliminated, leaving only the Offset voltage temperature drift component (= 2 LSB).
30 Submit Documentation Feedback Copyright © 2008–2013, Texas Instruments Incorporated
Product Folder Links: LMH6518
(= 50 mV x 100)
0.7V
2
U1
LMH6518
+5V
3,4
5,8
16
+5V
VCM_Aux
R3
3.83 k:
1%
R4
1.20 k:
1%
1
2
+OUT Aux
-OUT Aux
R11
237:
R12
237:
R1
75:
R2
75:
-
+
U2
LMH7220
U3
DAC101
C085
U4
LP3985
10 bit DAC
(I
2
C)
SDA
SCL
+5V
2.5V
VA
VOUT
VREF
0-2.5V
R10
100:
Trigger Output (LVDS)
2
3
4
5
+5V
6
1
+5V
LMH6518
www.ti.com SNOSB21C –MAY 2008–REVISED JULY 2013
Figure 68. Single-Ended Trigger from LMH6518 Auxiliary Output
U2’s minimum Toggle Rate specification of 750 Mb/s with ±50 mV overdrive allow the oscilloscope to trigger on
repetitive waveforms well above the 500 MHz oscilloscope bandwidth applications, when the input signal is at
least 14.3% of FS swing
(17)
The worst case single event minimum discernable pulse width is set by the LMH7220’s propagation delay
specification of 3.63 ns (20 mV overdrive).
Both the Main and the Auxiliary outputs can recover gracefully and quickly from a 50% overdrive condition as
tabulated in Electrical Characteristics under overdrive Recovery Time. Overdrive conditions beyond 50%,
however, could result in longer recovery times due to the interaction between an internal clamp and the common
mode feedback loop that sets the output common mode voltage. This may have an impact on both the displayed
waveform and the oscilloscope Trigger. The result could be a loss of Trigger pulse and/or visual distortion of the
displayed waveform. To avoid this scenario, the oscilloscope should detect an excessive overdrive and go into
trigger-loss mode. Done this way, the oscilloscope display would show the last waveform that did not violate the
overdrive condition. Preferably there would be a visual indicator on the screen that alerts the user of the situation
so that he can correct the excessive condition to return to normal display.
Copyright © 2008–2013, Texas Instruments Incorporated Submit Documentation Feedback 31
Product Folder Links: LMH6518
R14
R14 + R21
1 +
R5
R1 || R2
Gain (DC) = ¨
¨
©
§
¨ ¨
©
§
#1 V/V
Scope Input
Input Attenuators
Not Shown
C6
5 pF
R21
678 k:
R14
322 k: -
+
½ U1
LMV842
C7
20 nF
C3
100 nF
R22
1 M:
R15
678 k:
R11
322 k:
LMH6518 +IN
Q0
BFQ67
J8
MMBF5486
R20
500:
J10
MMBF5486
R16
20:
-
+
½ U1
LMV842
-5V
+5V
LMH6518 -IN
R5 +5V
500 k:
R1
500 k:
R3
500 k:
R4
500 k:
R0
500 k:
R9
200:
R6
200:
-10V
+10V
R2
Adjust R2 for gain matching
between DC and AC
C0
1 nF
C5
1 nF
R17
100:
R49
15:
R50
15:
Offset Control
DAC
R8
0:
LMH6518
SNOSB21C –MAY 2008–REVISED JULY 2013 www.ti.com
APPENDIX A
Here is the schematic drawing for a possible implementation of the LNA buffer shown in Figure 62:
Figure 69. JFET LNA Implementation
CIRCUIT OPERATION
This circuit uses an N-Channel JFET (J10) in Source-Follower configuration, to buffer the input signal, with J8
acting as a constant current source. This buffer presents a fixed input impedance (1 MΩ||10 pF) with a gain close
to 1 V/V.
The signal path is AC coupled through C7 with DC (and low frequency) at LMH6518 +IN maintained through the
action of U1. NPN transistor Q0 is an emitter follower which isolates the buffer from the load (LMH6518 input and
board traces).
The undriven input of the LMH6518, −IN, is biased to 2.5V by R6, R9 voltage divider. The Lower ½ of U1 inverts
this voltage and the upper ½ of U1 compares it to the combination of the driven output level at LMH6518 +IN and
the scaled version of scope input at R14, R21 junction, and adjusts J10 Gate accordingly to set the LMH6518 +IN.
This control loop has a frequency response that covers DC to a few Hz, limited by the roll-off capacitor C3 and
R15 combination (1st order approximation). DC and low frequency gain is given by:
(18)
With the values in Figure 69 → R2 ≈ 452 kΩ:
32 Submit Documentation Feedback Copyright © 2008–2013, Texas Instruments Incorporated
Product Folder Links: LMH6518
R21
R14
=
R15
R11
LMH6518
www.ti.com SNOSB21C –MAY 2008–REVISED JULY 2013
For a flat frequency response, the DC (low frequency) gain needs to be lowered to match the less-than-1 V/V AC
(high frequency) path gain through the JFETs. This can be done by increasing the value of R2.
By choosing the values of R15 and R11 so that
(19)
the frequency response at J10 Gate (and consequently the output) will remain flat when C7 starts to conduct.
Offset correction is done by varying the voltage at R4, using a DAC or equivalent as shown, in order to shift the
LMH6518 +IN voltage relative to −IN. The result is a circuit which shifts the ground referenced scope input to
2.5V (VCC/2) CM with adjustable offset and without any JFET or BJT related offsets.
Note that the front-end attenuator (not shown) lower leg resistance should be increased for proper divider-ratio to
account for the 1 MΩ shunt due to the series combination of R21 and R14. For example, a 10:1 front-end
attenuator could be formed by a series 900 kΩ and a shunt 111 kΩ for a scope BNC input impedance of 1 MΩ (=
900K + (111K || 1M)).
Table 8 lists other possible JFET candidates that fall in the range of speed (ft) and low noise needed:
Table 8. Suitable JFET Candidates Specifications
Company Part Number VP (V) Idss gm (mS) Input C noise (1) Break Calculated ft
(mA) (pF) (nV/RtHz) down (V) (MHz)
Interfet IF140 −2.2 10 5.5 2.3 4 −20 380
Interfet IF142 −2.2 10 5.5 2.3 4 −25 380
Interfet 2N5397/8 −2.5 13 8 5 2.5 −25 254
Interfet 2N5911/2 −2.5 13 8 5 2.5 254
Interfet J308/9/10 −2.3 21 17 5.8 −25 466
Philips BF513 -3 15 10 5 318
Fairchild MMBF5486 −4 14 7 4 2.5 −25 278
Vishay
Siliconix SST441 −3.5 13 6 3.5 4 −35 272
(1) Noise data at ∼ Idss/2
The LNA noise could degrade the scope’s SNR if it is comparable to the input referred noise of the LMH6518.
LNA noise is influenced by the following operating conditions:
a. JFET equivalent input noise
b. BJT Base current
Reducing either “a” or “b” above, or both, reduces noise. One way to reduce “a” is to increase R8 (currently set to
0Ω). This will reduce the noise impact of J8 but requires a JFET which has a higher Idss rating in order to
maintain the operating current of J10 so that J10’s noise contribution is minimized. Reducing the BJT Base
current can be accomplished with increasing R20 at the expenses of higher rise/fall times. A higher β will also
reduce the Base current (keep in mind that β and ft at the operating Collector current is what matters).
Figure 70 shows the impact of the JFET buffer noise on SNR, compared to SNR in Figure 58, assuming either 3
nV/√Hz or 1.5 nV/√Hz buffer noise for comparison:
Copyright © 2008–2013, Texas Instruments Incorporated Submit Documentation Feedback 33
Product Folder Links: LMH6518
R1
900 k:
C5
2-5 pF
R2
111 k:
C1
8 pF
C2
65 pF
R3
990 k:
C6
2-5 pF
R4
10.1 k:
C3
8 pF
C4
780 pF
10:1
100:1 R_LNA
1 M:
C_LNA
10 pF
JFET LNA
1:1
10:1
100:1
1:1
-2 2 6 10 14 18 22 26 30 34 38
0
2
4
6
8
10
12
SNR IMPACT (dB)
GAIN (dB)
42
LNA Noise = 1.5 nV/
LNA Noise = 3 nV/ Hz
Hz
LMH6518
SNOSB21C –MAY 2008–REVISED JULY 2013 www.ti.com
Figure 70. LNA Buffer SNR Impact
ATTENUATOR DESIGN
Figure 71 shows a front-end attenuator designed to work with the JFET LNA of Figure 69.
Figure 71. Front End Attenuator for Figure 69 JFET LNA
R_LNA” and “C_LNA” are the input impedance components of the JFET LNA. The 10:1 and 100:1 attenuators
bottom resistors (R2 and R4) are adjusted higher to compensate for the LNA’s 1 MΩ input impedance, compared
to the case where a high-input-impedance LNA is used. The two switches used on the input and output of the
attenuator block must be low capacitance, high isolation switches in order to reduce any speed or crosstalk
impact. C1-C4 provide the proper frequency response (and step response) by creating “zeros” that flatten the
response for wide-band operation. For the 10:1 attenuator, R1C1 = R2C2. The same applies to the 100:1
attenuator. The shunt capacitors C1-C4 have a very important other benefit in that they roll-off the resistor thermal
noise at a low frequency (low pass response, −3 dB down at ∼20 kHz) thereby eliminating any significant noise
contribution from the attenuation resistors. Otherwise, the channel noise would be dominated by the attenuator
resistor thermal noise. C2 and C6 trimmer capacitors can be adjusted to match the input capacitance regardless
of attenuator used.
REFERENCE
1. Wideband amplifiers by Peter Staric and Erik Margan, published by Springer in 2006. (Section 5.2).
34 Submit Documentation Feedback Copyright © 2008–2013, Texas Instruments Incorporated
Product Folder Links: LMH6518
LMH6518
www.ti.com SNOSB21C –MAY 2008–REVISED JULY 2013
REVISION HISTORY
Hooman: Corrected PSRR condition from "HG" to "LG" per CMS C1305178.
Changes from Revision A (March 2013) to Revision B Page
• Changed layout of National Data Sheet to TI format .......................................................................................................... 34
Copyright © 2008–2013, Texas Instruments Incorporated Submit Documentation Feedback 35
Product Folder Links: LMH6518
PACKAGE OPTION ADDENDUM
www.ti.com 24-Jul-2013
Addendum-Page 1
PACKAGING INFORMATION
Orderable Device Status
(1)
Package Type Package
Drawing
Pins Package
Qty
Eco Plan
(2)
Lead/Ball Finish MSL Peak Temp
(3)
Op Temp (°C) Device Marking
(4/5)
Samples
LMH6518SQ/NOPB ACTIVE WQFN RGH 16 1000 Green (RoHS
& no Sb/Br)
CU SN Level-3-260C-168 HR -40 to 85 L6518SQ
LMH6518SQE/NOPB ACTIVE WQFN RGH 16 250 Green (RoHS
& no Sb/Br)
CU SN Level-3-260C-168 HR -40 to 85 L6518SQ
LMH6518SQX/NOPB ACTIVE WQFN RGH 16 4500 Green (RoHS
& no Sb/Br)
CU SN Level-3-260C-168 HR -40 to 85 L6518SQ
(1) The marketing status values are defined as follows:
ACTIVE: Product device recommended for new designs.
LIFEBUY: TI has announced that the device will be discontinued, and a lifetime-buy period is in effect.
NRND: Not recommended for new designs. Device is in production to support existing customers, but TI does not recommend using this part in a new design.
PREVIEW: Device has been announced but is not in production. Samples may or may not be available.
OBSOLETE: TI has discontinued the production of the device.
(2) Eco Plan - The planned eco-friendly classification: Pb-Free (RoHS), Pb-Free (RoHS Exempt), or Green (RoHS & no Sb/Br) - please check http://www.ti.com/productcontent for the latest availability
information and additional product content details.
TBD: The Pb-Free/Green conversion plan has not been defined.
Pb-Free (RoHS): TI's terms "Lead-Free" or "Pb-Free" mean semiconductor products that are compatible with the current RoHS requirements for all 6 substances, including the requirement that
lead not exceed 0.1% by weight in homogeneous materials. Where designed to be soldered at high temperatures, TI Pb-Free products are suitable for use in specified lead-free processes.
Pb-Free (RoHS Exempt): This component has a RoHS exemption for either 1) lead-based flip-chip solder bumps used between the die and package, or 2) lead-based die adhesive used between
the die and leadframe. The component is otherwise considered Pb-Free (RoHS compatible) as defined above.
Green (RoHS & no Sb/Br): TI defines "Green" to mean Pb-Free (RoHS compatible), and free of Bromine (Br) and Antimony (Sb) based flame retardants (Br or Sb do not exceed 0.1% by weight
in homogeneous material)
(3) MSL, Peak Temp. -- The Moisture Sensitivity Level rating according to the JEDEC industry standard classifications, and peak solder temperature.
(4) There may be additional marking, which relates to the logo, the lot trace code information, or the environmental category on the device.
(5) Multiple Device Markings will be inside parentheses. Only one Device Marking contained in parentheses and separated by a "~" will appear on a device. If a line is indented then it is a continuation
of the previous line and the two combined represent the entire Device Marking for that device.
Important Information and Disclaimer:The information provided on this page represents TI's knowledge and belief as of the date that it is provided. TI bases its knowledge and belief on information
provided by third parties, and makes no representation or warranty as to the accuracy of such information. Efforts are underway to better integrate information from third parties. TI has taken and
continues to take reasonable steps to provide representative and accurate information but may not have conducted destructive testing or chemical analysis on incoming materials and chemicals.
TI and TI suppliers consider certain information to be proprietary, and thus CAS numbers and other limited information may not be available for release.
PACKAGE OPTION ADDENDUM
www.ti.com 24-Jul-2013
Addendum-Page 2
In no event shall TI's liability arising out of such information exceed the total purchase price of the TI part(s) at issue in this document sold by TI to Customer on an annual basis.
TAPE AND REEL INFORMATION
*All dimensions are nominal
Device Package
Type
Package
Drawing
Pins SPQ Reel
Diameter
(mm)
Reel
Width
W1 (mm)
A0
(mm)
B0
(mm)
K0
(mm)
P1
(mm)
W
(mm)
Pin1
Quadrant
LMH6518SQ/NOPB WQFN RGH 16 1000 178.0 12.4 4.3 4.3 1.3 8.0 12.0 Q1
LMH6518SQE/NOPB WQFN RGH 16 250 178.0 12.4 4.3 4.3 1.3 8.0 12.0 Q1
LMH6518SQX/NOPB WQFN RGH 16 4500 330.0 12.4 4.3 4.3 1.3 8.0 12.0 Q1
PACKAGE MATERIALS INFORMATION
www.ti.com 24-Jul-2013
Pack Materials-Page 1
*All dimensions are nominal
Device Package Type Package Drawing Pins SPQ Length (mm) Width (mm) Height (mm)
LMH6518SQ/NOPB WQFN RGH 16 1000 213.0 191.0 55.0
LMH6518SQE/NOPB WQFN RGH 16 250 213.0 191.0 55.0
LMH6518SQX/NOPB WQFN RGH 16 4500 367.0 367.0 35.0
PACKAGE MATERIALS INFORMATION
www.ti.com 24-Jul-2013
Pack Materials-Page 2
MECHANICAL DATA
RGH0016A
www.ti.com
SQA16A (Rev A)
IMPORTANT NOTICE
Texas Instruments Incorporated and its subsidiaries (TI) reserve the right to make corrections, enhancements, improvements and other
changes to its semiconductor products and services per JESD46, latest issue, and to discontinue any product or service per JESD48, latest
issue. Buyers should obtain the latest relevant information before placing orders and should verify that such information is current and
complete. All semiconductor products (also referred to herein as “components”) are sold subject to TI’s terms and conditions of sale
supplied at the time of order acknowledgment.
TI warrants performance of its components to the specifications applicable at the time of sale, in accordance with the warranty in TI’s terms
and conditions of sale of semiconductor products. Testing and other quality control techniques are used to the extent TI deems necessary
to support this warranty. Except where mandated by applicable law, testing of all parameters of each component is not necessarily
performed.
TI assumes no liability for applications assistance or the design of Buyers’ products. Buyers are responsible for their products and
applications using TI components. To minimize the risks associated with Buyers’ products and applications, Buyers should provide
adequate design and operating safeguards.
TI does not warrant or represent that any license, either express or implied, is granted under any patent right, copyright, mask work right, or
other intellectual property right relating to any combination, machine, or process in which TI components or services are used. Information
published by TI regarding third-party products or services does not constitute a license to use such products or services or a warranty or
endorsement thereof. Use of such information may require a license from a third party under the patents or other intellectual property of the
third party, or a license from TI under the patents or other intellectual property of TI.
Reproduction of significant portions of TI information in TI data books or data sheets is permissible only if reproduction is without alteration
and is accompanied by all associated warranties, conditions, limitations, and notices. TI is not responsible or liable for such altered
documentation. Information of third parties may be subject to additional restrictions.
Resale of TI components or services with statements different from or beyond the parameters stated by TI for that component or service
voids all express and any implied warranties for the associated TI component or service and is an unfair and deceptive business practice.
TI is not responsible or liable for any such statements.
Buyer acknowledges and agrees that it is solely responsible for compliance with all legal, regulatory and safety-related requirements
concerning its products, and any use of TI components in its applications, notwithstanding any applications-related information or support
that may be provided by TI. Buyer represents and agrees that it has all the necessary expertise to create and implement safeguards which
anticipate dangerous consequences of failures, monitor failures and their consequences, lessen the likelihood of failures that might cause
harm and take appropriate remedial actions. Buyer will fully indemnify TI and its representatives against any damages arising out of the use
of any TI components in safety-critical applications.
In some cases, TI components may be promoted specifically to facilitate safety-related applications. With such components, TI’s goal is to
help enable customers to design and create their own end-product solutions that meet applicable functional safety standards and
requirements. Nonetheless, such components are subject to these terms.
No TI components are authorized for use in FDA Class III (or similar life-critical medical equipment) unless authorized officers of the parties
have executed a special agreement specifically governing such use.
Only those TI components which TI has specifically designated as military grade or “enhanced plastic” are designed and intended for use in
military/aerospace applications or environments. Buyer acknowledges and agrees that any military or aerospace use of TI components
which have not been so designated is solely at the Buyer's risk, and that Buyer is solely responsible for compliance with all legal and
regulatory requirements in connection with such use.
TI has specifically designated certain components as meeting ISO/TS16949 requirements, mainly for automotive use. In any case of use of
non-designated products, TI will not be responsible for any failure to meet ISO/TS16949.
Products Applications
Audio www.ti.com/audio Automotive and Transportation www.ti.com/automotive
Amplifiers amplifier.ti.com Communications and Telecom www.ti.com/communications
Data Converters dataconverter.ti.com Computers and Peripherals www.ti.com/computers
DLP® Products www.dlp.com Consumer Electronics www.ti.com/consumer-apps
DSP dsp.ti.com Energy and Lighting www.ti.com/energy
Clocks and Timers www.ti.com/clocks Industrial www.ti.com/industrial
Interface interface.ti.com Medical www.ti.com/medical
Logic logic.ti.com Security www.ti.com/security
Power Mgmt power.ti.com Space, Avionics and Defense www.ti.com/space-avionics-defense
Microcontrollers microcontroller.ti.com Video and Imaging www.ti.com/video
RFID www.ti-rfid.com
OMAP Applications Processors www.ti.com/omap TI E2E Community e2e.ti.com
Wireless Connectivity www.ti.com/wirelessconnectivity
Mailing Address: Texas Instruments, Post Office Box 655303, Dallas, Texas 75265
Copyright © 2013, Texas Instruments Incorporated
1/7
STPS10H100CT/CG/CR/CFP ®
July 2003 - Ed: 3F
HIGH VOLTAGE POWER SCHOTTKY RECTIFIER
IF(AV) 2 x 5 A
VRRM 100 V
Tj 175°C
VF (max) 0.61 V
MAIN PRODUCT CHARACTERISTICS
n HIGH JUNCTION TEMPERATURE CAPABILITY
FOR CONVERTERS LOCATED IN CONFINED
ENVIRONMENT
n LOW LEAKAGE CURRENT AT HIGH
TEMPERATURE
n LOW STATIC AND DYNAMIC LOSSES AS A
RESULT OF THE SCHOTTKY BARRIER
n AVALANCHE CAPABILITY SPECIFIED
FEATURES AND BENEFITS
Schottky barrier rectifier designed for high
frequency miniature Switched Mode Power
Supplies such as adaptators and on board
DC/DC converters. Packaged in TO-220AB,
TO-220FPAB, D2PAK and I2PAK.
DESCRIPTION
TO-220AB
STPS10H100CT
A1
A2
K
Symbol Parameter Value Unit
VRRM Repetitive peak reverse voltage 100 V
IF(RMS) RMS forward current 10 A
IF(AV) Average forward
current d = 0.5
TO-220AB
D2PAK / I2PAK
Tc = 165°C per diode
per device
5
10
A
TO-220FPAB Tc = 160°C
IFSM Surge non repetitive forward current tp = 10 ms sinusoidal 180 A
IRRM Repetitive peak reverse current tp = 2 μs square F = 1kHz 1 A
PARM Repetitive peak avalanche power tp = 1μs Tj = 25°C 7200 W
Tstg Storage temperature range - 65 to + 175 °C
Tj Maximum operating junction temperature * 175 °C
dV/dt Critical rate of rise of reverse voltage 10000 V/μs
ABSOLUTE RATINGS (limiting values, per diode)
A1
A2
K
A1
A2
K
D2PAK
STPS10H100CG
K
A1
A2
K
I2PAK
STPS10H100CR
* :
dPtot
dTj Rth j a
<
-
1
( )
thermal runaway condition for a diode on its own heatsink
A1
A2
K
TO-220FPAB
STPS10H100CFP
STPS10H100CT/CG/CR/CFP
2/7
Symbol Parameter Value Unit
Rth (j-c) Junction to case D2PAK / I2PAK
TO-220AB
Per diode 2.2 °C/W
Total 1.3
Rth (c) Coupling 0.3
Rth (j-c) Junction to case TO-220FPAB Per diode 4.5 °C/W
Total 3.5
Rth (c) Coupling 2.5
When the diodes 1 and 2 are used simultaneously :
D Tj(diode 1) = P(diode1) x Rth(j-c)(Per diode) + P(diode 2) x Rth(c)
THERMAL RESISTANCES
Symbol Parameter Tests conditions Min. Typ. Max. Unit
IR * Reverse leakage current Tj = 25°C VR = VRRM 3.5 μA
Tj = 125°C 1.3 4.5 mA
VF ** Forward voltage drop Tj = 25°C IF = 5 A 0.73 V
Tj = 125°C 0.57 0.61
Tj = 25°C IF = 10 A 0.85
Tj = 125°C 0.66 0.71
Pulse test : * tp = 5 ms, d < 2%
** tp = 380 μs, d < 2%
To evaluate the maximum conduction losses use the following equation :
P = 0.51 x IF(AV) + 0.02 x IF
2
(RMS)
STATIC ELECTRICAL CHARACTERISTICS (per diode)
0.0 0.5 1.0 1.5 2.0 2.5 3.0 3.5 4.0 4.5 5.0 5.5 6.0
0.0
0.5
1.0
1.5
2.0
2.5
3.0
3.5
4.0
PF(av)(W)
IF(av) (A)
T
d=tp/T tp
d = 0.2 d = 0.5
d = 1
d = 0.05
d = 0.1
Fig. 1: Average forward power dissipation versus
average forward current (per diode).
0 25 50 75 100 125 150 175
0
1
2
3
4
5
6
Tamb(°C)
IF(av)(A)
Rth(j-a)=Rth(j-c)
Rth(j-a)=15°C/W
D²PAK/I²PAK/TO-220AB
TO-220FPAB
Fig. 2: Average forward current versus ambient
temperature (d=0.5, per diode).
STPS10H100CT/CG/CR/CFP
3/7
1E-3 1E-2 1E-1 1E+0
0
20
40
60
80
100
120
IM(A)
Tc=50°C
Tc=75°C
Tc=125°C
t(s)
IM
t
d=0.5
Fig. 5-1: Non repetitive surge peak forward
current versus overload duration (maximum
values, per diode)
1E-3 1E-2 1E-1 1E+0
0.0
0.2
0.4
0.6
0.8
1.0
Zth(j-c)/Rth(j-c)
tp(s)
T
d=tp/T tp
Single pulse
d = 0.1
d = 0.2
d = 0.5
Fig. 6-1: Relative variation of thermal impedance
junction to case versus pulse duration (per diode).
1E-3 1E-2 1E-1 1E+0
0
10
20
30
40
50
60
70
80
t(s)
IM(A)
Tc=50°C
Tc=75°C
Tc=125°C
IM
t
d=0.5
Fig. 5-2: Non repetitive surge peak forward current
versus overload duration (maximum values, per
diode)(TO-220FPAB)
1E-3 1E-2 1E-1 1E+0 1E+1
0.0
0.2
0.4
0.6
0.8
1.0
tp(s)
Zth(j-c)/Rth(j-c)
T
d=tp/T tp Single pulse
d = 0.1
d = 0.2
d = 0.5
Fig. 6-2: Relative variation of thermal impedance
junction to case versus pulse duration (per
diode).(TO-220FPAB)
0
0.2
0.4
0.6
0.8
1
1.2
0 25 50 75 100 125 150
Tj(°C)
P (t)
P (25°C)
ARM p
ARM
Fig. 4: Normalized avalanche power derating
versus junction temperature.
0.001
0.01
0.01 0.1 1
0.1
10 100 1000
1
tp(μs)
P (t)
P (1μs)
ARM p
ARM
Fig. 3: Normalized avalanche power derating versus
pulse duration.
STPS10H100CT/CG/CR/CFP
4/7
0.0 0.2 0.4 0.6 0.8 1.0 1.2 1.4
0.1
1.0
10.0
100.0
IFM(A)
Tj=125°C
Typical values
Tj=125°C
Tj=25°C
Tj=150°C
Typical values
VFM(V)
Fig. 9: Forward voltage drop versus forward
current (maximum values, per diode).
0 2 4 6 8 10 12 14 16 18 20
0
10
20
30
40
50
60
70
80
Rth(j-a) (°C/W)
S(Cu) (cm²)
Fig. 10: Thermal resistance junction to ambient
versus copper surface under tab (Epoxy printed
circuit board FR4, copper thickness: 35μm)
0 10 20 30 40 50 60 70 80 90 100
1E-2
1E-1
1E+0
1E+1
1E+2
1E+3
1E+4
IR(μA)
Tj=125°C
Tj=25°C
Tj=150°C
Tj=100°C
VR(V)
Fig. 7: Reverse leakage current versus reverse
voltage applied (typical values, per diode).
1 2 5 10 20 50 100
10
100
1000
C(pF)
F=1MHz
Tj=25°C
VR(V)
Fig. 8: Junction capacitance versus reverse
voltage applied (typical values, per diode).
5/7
STPS10H100CT/CG/CR/CFP
PACKAGE MECHANICAL DATA
D2PAK
A
C2
D
R
A2
M
V2
C
A1
G
L
L3
L2
B
B2
E
*
* FLAT ZONE NO LESS THAN 2mm
REF.
DIMENSIONS
Millimeters Inches
Min. Max. Min. Max.
A 4.40 4.60 0.173 0.181
A1 2.49 2.69 0.098 0.106
A2 0.03 0.23 0.001 0.009
B 0.70 0.93 0.027 0.037
B2 1.14 1.70 0.045 0.067
C 0.45 0.60 0.017 0.024
C2 1.23 1.36 0.048 0.054
D 8.95 9.35 0.352 0.368
E 10.00 10.40 0.393 0.409
G 4.88 5.28 0.192 0.208
L 15.00 15.85 0.590 0.624
L2 1.27 1.40 0.050 0.055
L3 1.40 1.75 0.055 0.069
M 2.40 3.20 0.094 0.126
R 0.40 typ. 0.016 typ.
V2 0° 8° 0° 8°
FOOT PRINT in millimeters
8.90
3.70
1.30
5.08
16.90
10.30
6/7
STPS10H100CT/CG/CR/CFP
PACKAGE MECHANICAL DATA
I2PAK
e
D
L
L1
L2
b1
b
b2
E
A
c2
A1
c
REF.
DIMENSIONS
Millimeters Inches
Min. Max. Min. Max.
A 4.40 4.60 0.173 0.181
A1 2.49 2.69 0.098 0.106
b 0.70 0.93 0.028 0.037
b1 1.14 1.17 0.044 0.046
b2 1.14 1.17 0.044 0.046
c 0.45 0.60 0.018 0.024
c2 1.23 1.36 0.048 0.054
D 8.95 9.35 0.352 0.368
e 2.40 2.70 0.094 0.106
E 10.0 10.4 0.394 0.409
L 13.1 13.6 0.516 0.535
L1 3.48 3.78 0.137 0.149
L2 1.27 1.40 0.050 0.055
PACKAGE MECHANICAL DATA
TO-220FPAB
H
L3
L2
L4
L6
G
G1
F
F1
L5
D
E
L7
A
B
Dia
F2
REF. DIMENSIONS
Millimeters Inches
Min. Max. Min. Max.
A 4.4 4.6 0.173 0.181
B 2.5 2.7 0.098 0.106
D 2.5 2.75 0.098 0.108
E 0.45 0.70 0.018 0.027
F 0.75 1 0.030 0.039
F1 1.15 1.70 0.045 0.067
F2 1.15 1.70 0.045 0.067
G 4.95 5.20 0.195 0.205
G1 2.4 2.7 0.094 0.106
H 10 10.4 0.393 0.409
L2 16 Typ. 0.63 Typ.
L3 28.6 30.6 1.126 1.205
L4 9.8 10.6 0.386 0.417
L5 2.9 3.6 0.114 0.142
L6 15.9 16.4 0.626 0.646
L7 9.00 9.30 0.354 0.366
Dia. 3.00 3.20 0.118 0.126
7/7
STPS10H100CT/CG/CR/CFP
Information furnished is believed to be accurate and reliable. However, STMicroelectronics assumes no responsibility for the consequences of
use of such information nor for any infringement of patents or other rights of third parties which may result from its use. No license is granted by
implication or otherwise under any patent or patent rights of STMicroelectronics. Specifications mentioned in this publication are subject to
change without notice. This publication supersedes and replaces all information previously supplied.
STMicroelectronics products are not authorized for use as critical components in life support devices or systems without express written
approval of STMicroelectronics.
The ST logo is a registered trademark of STMicroelectronics
© 2003 STMicroelectronics - Printed in Italy - All rights reserved.
STMicroelectronics GROUP OF COMPANIES
Australia - Brazil - Canada - China - Finland - France - Germany
Hong Kong - India - Israel - Italy - Japan - Malaysia - Malta - Morocco - Singapore
Spain - Sweden - Switzerland - United Kingdom - United States.
http://www.st.com
Ordering type Marking Package Weight Base qty Delivery mode
STPS10H100CT STPS10H100CT TO-220AB 2.20g 50 Tube
STPS10H100CFP STPS10H100CFP TO-220FPAB 2.0 g 50 Tube
STPS10H100CG STPS10H100CG D2PAK 1.48g 50 Tube
STPS10H100CG-TR STPS10H100CG D2PAK 1.48g 1000 Tape and reel
STPS10H100CR STPS10H100CR I2PAK 1.49g 50 Tube
n Epoxy meets UL94,V0
PACKAGE MECHANICAL DATA
TO-220AB
A
C
D
L7
Dia
L5
L6
L9
L4
F
H2
G
G1
L2
F2
F1
E
M
REF. DIMENSIONS
Millimeters Inches
Min. Max. Min. Max.
A 4.40 4.60 0.173 0.181
C 1.23 1.32 0.048 0.051
D 2.40 2.72 0.094 0.107
E 0.49 0.70 0.019 0.027
F 0.61 0.88 0.024 0.034
F1 1.14 1.70 0.044 0.066
F2 1.14 1.70 0.044 0.066
G 4.95 5.15 0.194 0.202
G1 2.40 2.70 0.094 0.106
H2 10 10.40 0.393 0.409
L2 16.4 typ. 0.645 typ.
L4 13 14 0.511 0.551
L5 2.65 2.95 0.104 0.116
L6 15.25 15.75 0.600 0.620
L7 6.20 6.60 0.244 0.259
L9 3.50 3.93 0.137 0.154
M 2.6 typ. 0.102 typ.
Diam. 3.75 3.85 0.147 0.151
n Cooling method: C.
n Recommended torque value: 0.55 m.N
n Maximum torque value 0.70 m.N
LM19
www.ti.com SNIS122E –MAY 2001–REVISED MARCH 2013
LM19 2.4V, 10μA, TO-92 Temperature Sensor
Check for Samples: LM19
1FEATURES DESCRIPTION
The LM19 is a precision analog output CMOS 2• Rated for Full −55°C to +130°C Range
integrated-circuit temperature sensor that operates
• Available in a TO-92 Package over a −55°C to +130°C temperature range. The
• Predictable Curvature Error power supply operating range is +2.4 V to +5.5 V.
• Suitable for Remote Applications The transfer function of LM19 is predominately linear,
• UL Recognized Component yet has a slight predictable parabolic curvature. The accuracy of the LM19 when specified to a parabolic
transfer function is ±2.5°C at an ambient temperature APPLICATIONS of +30°C. The temperature error increases linearly
• Cellular Phones and reaches a maximum of ±3.8°C at the
• Computers temperature range extremes. The temperature range
is affected by the power supply voltage. At a power
• Power Supply Modules supply voltage of 2.7 V to 5.5 V the temperature
• Battery Management range extremes are +130°C and −55°C. Decreasing
• FAX Machines the power supply voltage to 2.4 V changes the
negative extreme to −30°C, while the positive
• Printers remains at +130°C.
• HVAC
The LM19's quiescent current is less than 10 μA.
• Disk Drives Therefore, self-heating is less than 0.02°C in still air.
• Appliances Shutdown capability for the LM19 is intrinsic because
its inherent low power consumption allows it to be
KEY SPECIFICATIONS powered directly from the output of many logic gates
or does not necessitate shutdown at all.
• Accuracy at +30°C ±2.5 °C (max)
• Accuracy at +130°C & −55°C ±3.5 to ±3.8 °C
(max)
• Power Supply Voltage Range +2.4V to +5.5V
• Current Drain 10 μA (max)
• Nonlinearity ±0.4 % (typ)
• Output Impedance 160 Ω (max)
• Load Regulation
– 0μA < IL< +16 μA
1
Please be aware that an important notice concerning availability, standard warranty, and use in critical applications of
Texas Instruments semiconductor products and disclaimers thereto appears at the end of this data sheet.
2All trademarks are the property of their respective owners.
PRODUCTION DATA information is current as of publication date. Copyright © 2001–2013, Texas Instruments Incorporated
Products conform to specifications per the terms of the Texas
Instruments standard warranty. Production processing does not
necessarily include testing of all parameters.
LM19
SNIS122E –MAY 2001–REVISED MARCH 2013 www.ti.com
Typical Application
Output Voltage vs Temperature
VO = (−3.88×10−6×T2) + (−1.15×10−2×T) + 1.8639
or
where:
T is temperature, and VO is the measured output voltage of the
LM19.
Figure 1. Full-Range Celsius (Centigrade) Temperature Sensor (−55°C to +130°C) Operating from a
Single Li-Ion Battery Cell
Temperature (T) Typical VO
+130°C +303 mV
+100°C +675 mV
+80°C +919 mV
+30°C +1515 mV
+25°C +1574 mV
0°C +1863.9 mV
−30°C +2205 mV
−40°C +2318 mV
−55°C +2485 mV
Connection Diagram
Figure 2. TO-92
Package Number LP
These devices have limited built-in ESD protection. The leads should be shorted together or the device placed in conductive foam
during storage or handling to prevent electrostatic damage to the MOS gates.
2 Submit Documentation Feedback Copyright © 2001–2013, Texas Instruments Incorporated
Product Folder Links: LM19
LM19
www.ti.com SNIS122E –MAY 2001–REVISED MARCH 2013
Absolute Maximum Ratings(1)
Supply Voltage +6.5V to −0.2V
Output Voltage (V+ + 0.6 V) to −0.6 V
Output Current 10 mA
Input Current at any pin(2) 5 mA
Storage Temperature −65°C to +150°C
Maximum Junction Temperature (TJMAX) +150°C
ESD Susceptibility(3) Human Body Model 2500 V
Machine Model 250 V
Lead Temperature TO-92 Package Soldering (3 seconds dwell) +240°C
(1) Absolute Maximum Ratings indicate limits beyond which damage to the device may occur. Operating Ratings indicate conditions for
which the device is functional, but do not ensure specific performance limits. For ensured specifications and test conditions, see the
Electrical Characteristics. The specified specifications apply only for the test conditions listed. Some performance characteristics may
degrade when the device is not operated under the listed test conditions.
(2) When the input voltage (VI) at any pin exceeds power supplies (VI < GND or VI > V+), the current at that pin should be limited to 5 mA.
(3) The human body model is a 100 pF capacitor discharged through a 1.5 kΩ resistor into each pin. The machine model is a 200 pF
capacitor discharged directly into each pin.
Operating Ratings(1)
Specified Temperature Range TMIN ≤ TA ≤ TMAX
2.4 V ≤ V+≤ 2.7 V −30°C ≤ TA ≤ +130°C
2.7 V ≤ V+≤ 5.5 V −55°C ≤ TA ≤ +130°C
Supply Voltage Range (V+) +2.4 V to +5.5 V
Thermal Resistance, θJA
(2) TO-92 150°C/W
(1) Absolute Maximum Ratings indicate limits beyond which damage to the device may occur. Operating Ratings indicate conditions for
which the device is functional, but do not ensure specific performance limits. For ensured specifications and test conditions, see the
Electrical Characteristics. The specified specifications apply only for the test conditions listed. Some performance characteristics may
degrade when the device is not operated under the listed test conditions.
(2) The junction to ambient thermal resistance (θJA) is specified without a heat sink in still air.
Copyright © 2001–2013, Texas Instruments Incorporated Submit Documentation Feedback 3
Product Folder Links: LM19
LM19
SNIS122E –MAY 2001–REVISED MARCH 2013 www.ti.com
Electrical Characteristics
Unless otherwise noted, these specifications apply for V+ = +2.7 VDC. Boldface limits apply for TA = TJ = TMIN to TMAX ; all
other limits TA = TJ = 25°C; Unless otherwise noted.
Parameter Conditions Typical(1) LM19C Units
Limits(2) (Limit)
Temperature to Voltage Error TA = +25°C to +30°C ±2.5 °C (max)
VO = (−3.88×10−6×T2) TA = +130°C ±3.5 °C (max)
+ (−1.15×10−2×T) + 1.8639V(3)
TA = +125°C ±3.5 °C (max)
TA = +100°C ±3.2 °C (max)
TA = +85°C ±3.1 °C (max)
TA = +80°C ±3.0 °C (max)
TA = 0°C ±2.9 °C (max)
TA = −30°C ±3.3 °C (min)
TA = −40°C ±3.5 °C (max)
TA = −55°C ±3.8 °C (max)
Output Voltage at 0°C +1.8639 V
Variance from Curve ±1.0 °C
Non-Linearity(4) −20°C ≤ TA ≤ +80°C ±0.4 %
Sensor Gain (Temperature Sensitivity −30°C ≤ TA ≤ +100°C −11.77 −11.0 mV/°C (min)
or Average Slope) to equation: −12.6 mV/°C (max)
VO=−11.77 mV/°C×T+1.860V
Output Impedance 0 μA ≤ IL ≤ +16 μA(5) (6) 160 Ω (max)
Load Regulation(7) 0 μA ≤ IL ≤ +16 μA(5) (6) −2.5 mV (max)
Line Regulation(8) +2. 4 V ≤ V+ ≤ +5.0V +3.7 mV/V (max)
+5.0 V ≤ V+ ≤ +5.5 V +11 mV (max)
Quiescent Current +2. 4 V ≤ V+ ≤ +5.0V 4.5 7 μA (max)
+5.0V ≤ V+ ≤ +5.5V 4.5 9 μA (max)
+2. 4 V ≤ V+ ≤ +5.0V 4.5 10 μA (max)
Change of Quiescent Current +2. 4 V ≤ V+ ≤ +5.5V +0.7 μA
Temperature Coefficient of Quiescent −11 nA/°C Current
Shutdown Current V+ ≤ +0.8 V 0.02 μA
(1) Typicals are at TJ = TA = 25°C and represent most likely parametric norm.
(2) Limits are ensured to AOQL (Average Outgoing Quality Level).
(3) Accuracy is defined as the error between the measured and calculated output voltage at the specified conditions of voltage, current, and
temperature (expressed in°C).
(4) Non-Linearity is defined as the deviation of the calculated output-voltage-versus-temperature curve from the best-fit straight line, over
the temperature range specified.
(5) Negative currents are flowing into the LM19. Positive currents are flowing out of the LM19. Using this convention the LM19 can at most
sink −1 μA and source +16 μA.
(6) Load regulation or output impedance specifications apply over the supply voltage range of +2.4V to +5.5V.
(7) Regulation is measured at constant junction temperature, using pulse testing with a low duty cycle. Changes in output due to heating
effects can be computed by multiplying the internal dissipation by the thermal resistance.
(8) Line regulation is calculated by subtracting the output voltage at the highest supply input voltage from the output voltage at the lowest
supply input voltage.
4 Submit Documentation Feedback Copyright © 2001–2013, Texas Instruments Incorporated
Product Folder Links: LM19
MAX Limit
MIN Limit
Typical
-100 -50 0 50 100 150
-5
-4
-3
-2
-1
0
1
2
3
4
5
ERROR ( ºC)
TEMPERATURE (ºC)
LM19
www.ti.com SNIS122E –MAY 2001–REVISED MARCH 2013
Typical Performance Characteristics
Temperature Error vs. Temperature Thermal Response in Still Air
LM19 TRANSFER FUNCTION
The LM19's transfer function can be described in different ways with varying levels of precision. A simple linear
transfer function, with good accuracy near 25°C, is
VO= −11.69 mV/°C × T + 1.8663 V (1)
Over the full operating temperature range of −55°C to +130°C, best accuracy can be obtained by using the
parabolic transfer function
VO = (−3.88×10−6×T2) + (−1.15×10−2×T) + 1.8639 (2)
solving for T:
(3)
A linear transfer function can be used over a limited temperature range by calculating a slope and offset that give
best results over that range. A linear transfer function can be calculated from the parabolic transfer function of
the LM19. The slope of the linear transfer function can be calculated using the following equation:
m = −7.76 × 10−6× T − 0.0115
where
• T is the middle of the temperature range of interest and m is in V/°C. (4)
For example for the temperature range of Tmin = −30 to Tmax = +100°C:
T = 35°C
and
m = −11.77 mV/°C
The offset of the linear transfer function can be calculated using the following equation:
b = (VOP(Tmax) + VOP(T) − m × (Tmax+T))/2
where
• VOP(Tmax) is the calculated output voltage at Tmax using the parabolic transfer function for VO.
• VOP(T) is the calculated output voltage at T using the parabolic transfer function for VO. (5)
Using this procedure the best fit linear transfer function for many popular temperature ranges was calculated in
Table 1. As shown in Table 1 the error that is introduced by the linear transfer function increases with wider
temperature ranges.
Copyright © 2001–2013, Texas Instruments Incorporated Submit Documentation Feedback 5
Product Folder Links: LM19
LM19
SNIS122E –MAY 2001–REVISED MARCH 2013 www.ti.com
Table 1. First Order Equations Optimized For Different Temperature Ranges
Temperature Range Linear Equation Maximum Deviation of Linear Equation from
T VO= Parabolic Equation (°C) min (°C) Tmax (°C)
−55 +130 −11.79 mV/°C × T + 1.8528 V ±1.41
−40 +110 −11.77 mV/°C × T + 1.8577 V ±0.93
−30 +100 −11.77 mV/°C × T + 1.8605 V ±0.70
-40 +85 −11.67 mV/°C × T + 1.8583 V ±0.65
−10 +65 −11.71 mV/°C × T + 1.8641 V ±0.23
+35 +45 −11.81 mV/°C × T + 1.8701 V ±0.004
+20 +30 −11.69 mV/°C × T + 1.8663 V ±0.004
Mounting
The LM19 can be applied easily in the same way as other integrated-circuit temperature sensors. It can be glued
or cemented to a surface. The temperature that the LM19 is sensing will be within about +0.02°C of the surface
temperature to which the LM19's leads are attached.
This presumes that the ambient air temperature is almost the same as the surface temperature; if the air
temperature were much higher or lower than the surface temperature, the actual temperature measured would
be at an intermediate temperature between the surface temperature and the air temperature.
To ensure good thermal conductivity the backside of the LM19 die is directly attached to the GND pin. The
tempertures of the lands and traces to the other leads of the LM19 will also affect the temperature that is being
sensed.
Alternatively, the LM19 can be mounted inside a sealed-end metal tube, and can then be dipped into a bath or
screwed into a threaded hole in a tank. As with any IC, the LM19 and accompanying wiring and circuits must be
kept insulated and dry, to avoid leakage and corrosion. This is especially true if the circuit may operate at cold
temperatures where condensation can occur. Printed-circuit coatings and varnishes such as Humiseal and epoxy
paints or dips are often used to ensure that moisture cannot corrode the LM19 or its connections.
The thermal resistance junction to ambient (θJA) is the parameter used to calculate the rise of a device junction
temperature due to its power dissipation. For the LM19 the equation used to calculate the rise in the die
temperature is as follows:
TJ = TA + θJA [(V+ IQ) + (V+ − VO) IL]
where
• IQ is the quiescent current and ILis the load current on the output. (6)
Since the LM19's junction temperature is the actual temperature being measured care should be taken to
minimize the load current that the LM19 is required to drive.
Table 2 summarizes the rise in die temperature of the LM19 without any loading, and the thermal resistance for
different conditions.
Table 2. Temperature Rise of LM19 Due to Self-Heating and Thermal Resistance (θJA)
TO-92 TO-92
no heat sink small heat fin
θJA TJ − TA θJA TJ − TA
(°C/W) (°C) (°C/W) (°C)
Still air 150 TBD TBD TBD
Moving air TBD TBD TBD TBD
6 Submit Documentation Feedback Copyright © 2001–2013, Texas Instruments Incorporated
Product Folder Links: LM19
LM19
www.ti.com SNIS122E –MAY 2001–REVISED MARCH 2013
Capacitive Loads
The LM19 handles capacitive loading well. Without any precautions, the LM19 can drive any capacitive load less
than 300 pF as shown in Figure 3. Over the specified temperature range the LM19 has a maximum output
impedance of 160 Ω. In an extremely noisy environment it may be necessary to add some filtering to minimize
noise pickup. It is recommended that 0.1 μF be added from V+ to GND to bypass the power supply voltage, as
shown in Figure 4. In a noisy environment it may even be necessary to add a capacitor from the output to ground
with a series resistor as shown in Figure 4. A 1 μF output capacitor with the 160 Ω maximum output impedance
and a 200 Ω series resistor will form a 442 Hz lowpass filter. Since the thermal time constant of the LM19 is
much slower, the overall response time of the LM19 will not be significantly affected.
Figure 3. LM19 No Decoupling Required for Capacitive Loads Less than 300 pF
Table 3. LM19 with Filter for Noisy Environment
and Capacitive Loading greater than 300 pF
R (Ω) C (μF)
200 1
470 0.1
680 0.01
1 k 0.001
Either placement of resistor as shown
above is just as effective.
Figure 4. LM19 with Filter for Noisy Environment
and Capacitive Loading greater than 300 pF
Copyright © 2001–2013, Texas Instruments Incorporated Submit Documentation Feedback 7
Product Folder Links: LM19
4.1V R1
R3
R2
LM4040 U3 0.1 PF
R4
VOUT
V+
VT
VTemp
+
-
U1
V+ LM19
U2
(High = overtemp alarm)
VT1
VT2
VTEMP
VOUT
VT1 =
R1 + R2||R3
(4.1)R2
VT2 =
R2 + R1||R3
(4.1)R2||R3
LM7211
LM19
SNIS122E –MAY 2001–REVISED MARCH 2013 www.ti.com
Applications Circuits
Figure 5. Centigrade Thermostat
Figure 6. Conserving Power Dissipation with Shutdown
Figure 7. Suggested Connection to a Sampling Analog to Digital Converter Input Stage
Most CMOS ADCs found in ASICs have a sampled data comparator input structure that is notorious for causing
grief to analog output devices such as the LM19 and many op amps. The cause of this grief is the requirement of
instantaneous charge of the input sampling capacitor in the ADC. This requirement is easily accommodated by
the addition of a capacitor. Since not all ADCs have identical input stages, the charge requirements will vary
necessitating a different value of compensating capacitor. This ADC is shown as an example only. If a digital
output temperature is required please refer to devices such as the LM74.
8 Submit Documentation Feedback Copyright © 2001–2013, Texas Instruments Incorporated
Product Folder Links: LM19
LM19
www.ti.com SNIS122E –MAY 2001–REVISED MARCH 2013
REVISION HISTORY
Changes from Revision D (March 2013) to Revision E Page
• Changed layout of National Data Sheet to TI format ............................................................................................................ 8
Copyright © 2001–2013, Texas Instruments Incorporated Submit Documentation Feedback 9
Product Folder Links: LM19
PACKAGE OPTION ADDENDUM
www.ti.com 18-Oct-2013
Addendum-Page 1
PACKAGING INFORMATION
Orderable Device Status
(1)
Package Type Package
Drawing
Pins Package
Qty
Eco Plan
(2)
Lead/Ball Finish
(6)
MSL Peak Temp
(3)
Op Temp (°C) Device Marking
(4/5)
Samples
LM19CIZ/LFT4 ACTIVE TO-92 LP 3 2000 Green (RoHS
& no Sb/Br)
SN | CU SN N / A for Pkg Type LM19
CIZ
LM19CIZ/NOPB ACTIVE TO-92 LP 3 1800 Green (RoHS
& no Sb/Br)
SN | CU SN N / A for Pkg Type -55 to 130 LM19
CIZ
(1) The marketing status values are defined as follows:
ACTIVE: Product device recommended for new designs.
LIFEBUY: TI has announced that the device will be discontinued, and a lifetime-buy period is in effect.
NRND: Not recommended for new designs. Device is in production to support existing customers, but TI does not recommend using this part in a new design.
PREVIEW: Device has been announced but is not in production. Samples may or may not be available.
OBSOLETE: TI has discontinued the production of the device.
(2) Eco Plan - The planned eco-friendly classification: Pb-Free (RoHS), Pb-Free (RoHS Exempt), or Green (RoHS & no Sb/Br) - please check http://www.ti.com/productcontent for the latest availability
information and additional product content details.
TBD: The Pb-Free/Green conversion plan has not been defined.
Pb-Free (RoHS): TI's terms "Lead-Free" or "Pb-Free" mean semiconductor products that are compatible with the current RoHS requirements for all 6 substances, including the requirement that
lead not exceed 0.1% by weight in homogeneous materials. Where designed to be soldered at high temperatures, TI Pb-Free products are suitable for use in specified lead-free processes.
Pb-Free (RoHS Exempt): This component has a RoHS exemption for either 1) lead-based flip-chip solder bumps used between the die and package, or 2) lead-based die adhesive used between
the die and leadframe. The component is otherwise considered Pb-Free (RoHS compatible) as defined above.
Green (RoHS & no Sb/Br): TI defines "Green" to mean Pb-Free (RoHS compatible), and free of Bromine (Br) and Antimony (Sb) based flame retardants (Br or Sb do not exceed 0.1% by weight
in homogeneous material)
(3) MSL, Peak Temp. - The Moisture Sensitivity Level rating according to the JEDEC industry standard classifications, and peak solder temperature.
(4) There may be additional marking, which relates to the logo, the lot trace code information, or the environmental category on the device.
(5) Multiple Device Markings will be inside parentheses. Only one Device Marking contained in parentheses and separated by a "~" will appear on a device. If a line is indented then it is a continuation
of the previous line and the two combined represent the entire Device Marking for that device.
(6) Lead/Ball Finish - Orderable Devices may have multiple material finish options. Finish options are separated by a vertical ruled line. Lead/Ball Finish values may wrap to two lines if the finish
value exceeds the maximum column width.
Important Information and Disclaimer:The information provided on this page represents TI's knowledge and belief as of the date that it is provided. TI bases its knowledge and belief on information
provided by third parties, and makes no representation or warranty as to the accuracy of such information. Efforts are underway to better integrate information from third parties. TI has taken and
continues to take reasonable steps to provide representative and accurate information but may not have conducted destructive testing or chemical analysis on incoming materials and chemicals.
TI and TI suppliers consider certain information to be proprietary, and thus CAS numbers and other limited information may not be available for release.
PACKAGE OPTION ADDENDUM
www.ti.com 18-Oct-2013
Addendum-Page 2
In no event shall TI's liability arising out of such information exceed the total purchase price of the TI part(s) at issue in this document sold by TI to Customer on an annual basis.
IMPORTANT NOTICE
Texas Instruments Incorporated and its subsidiaries (TI) reserve the right to make corrections, enhancements, improvements and other
changes to its semiconductor products and services per JESD46, latest issue, and to discontinue any product or service per JESD48, latest
issue. Buyers should obtain the latest relevant information before placing orders and should verify that such information is current and
complete. All semiconductor products (also referred to herein as “components”) are sold subject to TI’s terms and conditions of sale
supplied at the time of order acknowledgment.
TI warrants performance of its components to the specifications applicable at the time of sale, in accordance with the warranty in TI’s terms
and conditions of sale of semiconductor products. Testing and other quality control techniques are used to the extent TI deems necessary
to support this warranty. Except where mandated by applicable law, testing of all parameters of each component is not necessarily
performed.
TI assumes no liability for applications assistance or the design of Buyers’ products. Buyers are responsible for their products and
applications using TI components. To minimize the risks associated with Buyers’ products and applications, Buyers should provide
adequate design and operating safeguards.
TI does not warrant or represent that any license, either express or implied, is granted under any patent right, copyright, mask work right, or
other intellectual property right relating to any combination, machine, or process in which TI components or services are used. Information
published by TI regarding third-party products or services does not constitute a license to use such products or services or a warranty or
endorsement thereof. Use of such information may require a license from a third party under the patents or other intellectual property of the
third party, or a license from TI under the patents or other intellectual property of TI.
Reproduction of significant portions of TI information in TI data books or data sheets is permissible only if reproduction is without alteration
and is accompanied by all associated warranties, conditions, limitations, and notices. TI is not responsible or liable for such altered
documentation. Information of third parties may be subject to additional restrictions.
Resale of TI components or services with statements different from or beyond the parameters stated by TI for that component or service
voids all express and any implied warranties for the associated TI component or service and is an unfair and deceptive business practice.
TI is not responsible or liable for any such statements.
Buyer acknowledges and agrees that it is solely responsible for compliance with all legal, regulatory and safety-related requirements
concerning its products, and any use of TI components in its applications, notwithstanding any applications-related information or support
that may be provided by TI. Buyer represents and agrees that it has all the necessary expertise to create and implement safeguards which
anticipate dangerous consequences of failures, monitor failures and their consequences, lessen the likelihood of failures that might cause
harm and take appropriate remedial actions. Buyer will fully indemnify TI and its representatives against any damages arising out of the use
of any TI components in safety-critical applications.
In some cases, TI components may be promoted specifically to facilitate safety-related applications. With such components, TI’s goal is to
help enable customers to design and create their own end-product solutions that meet applicable functional safety standards and
requirements. Nonetheless, such components are subject to these terms.
No TI components are authorized for use in FDA Class III (or similar life-critical medical equipment) unless authorized officers of the parties
have executed a special agreement specifically governing such use.
Only those TI components which TI has specifically designated as military grade or “enhanced plastic” are designed and intended for use in
military/aerospace applications or environments. Buyer acknowledges and agrees that any military or aerospace use of TI components
which have not been so designated is solely at the Buyer's risk, and that Buyer is solely responsible for compliance with all legal and
regulatory requirements in connection with such use.
TI has specifically designated certain components as meeting ISO/TS16949 requirements, mainly for automotive use. In any case of use of
non-designated products, TI will not be responsible for any failure to meet ISO/TS16949.
Products Applications
Audio www.ti.com/audio Automotive and Transportation www.ti.com/automotive
Amplifiers amplifier.ti.com Communications and Telecom www.ti.com/communications
Data Converters dataconverter.ti.com Computers and Peripherals www.ti.com/computers
DLP® Products www.dlp.com Consumer Electronics www.ti.com/consumer-apps
DSP dsp.ti.com Energy and Lighting www.ti.com/energy
Clocks and Timers www.ti.com/clocks Industrial www.ti.com/industrial
Interface interface.ti.com Medical www.ti.com/medical
Logic logic.ti.com Security www.ti.com/security
Power Mgmt power.ti.com Space, Avionics and Defense www.ti.com/space-avionics-defense
Microcontrollers microcontroller.ti.com Video and Imaging www.ti.com/video
RFID www.ti-rfid.com
OMAP Applications Processors www.ti.com/omap TI E2E Community e2e.ti.com
Wireless Connectivity www.ti.com/wirelessconnectivity
Mailing Address: Texas Instruments, Post Office Box 655303, Dallas, Texas 75265
Copyright © 2013, Texas Instruments Incorporated
© 2006 Microchip Technology Inc. DS22003B-page 1
MCP3421
Features
• 18-bit ΔΣ ADC in a SOT-23-6 package
• Differential input operation
• Self calibration of Internal Offset and Gain per
each conversion
• On-board Voltage Reference:
- Accuracy: 2.048V ± 0.05%
- Drift: 5 ppm/°C
• On-board Programmable Gain Amplifier (PGA):
- Gains of 1,2,4 or 8
• On-board Oscillator
• INL: 10 ppm of FSR (FSR = 4.096V/PGA)
• Programmable Data Rate Options:
- 3.75 SPS (18 bits)
- 15 SPS (16 bits)
- 60 SPS (14 bits)
- 240 SPS (12 bits)
• One-Shot or Continuous Conversion Options
• Low current consumption:
- 145 μA typical
(VDD= 3V, Continuous Conversion)
- 39 μA typical
(VDD= 3V, One-Shot Conversion with 1 SPS)
• Supports I2C Serial Interface:
- Standard, Fast and High Speed Modes
• Single Supply Operation: 2.7V to 5.5V
• Extended Temperature Range: -40°C to 125°C
Typical Applications
• Portable Instrumentation
• Weigh Scales and Fuel Gauges
• Temperature Sensing with RTD, Thermistor, and
Thermocouple
• Bridge Sensing for Pressure, Strain, and Force.
Package Types
Description
The MCP3421 is a single channel low-noise, high
accuracy ΔΣ A/D converter with differential inputs and
up to 18 bits of resolution in a small SOT-23-6 package.
The on-board precision 2.048V reference voltage
enables an input range of ±2.048V differentially
(Δ voltage = 4.096V). The device uses a two-wire I2C
compatible serial interface and operates from a single
2.7V to 5.5V power supply.
The MCP3421 device performs conversion at rates of
3.75, 15, 60, or 240 samples per second (SPS)
depending on the user controllable configuration bit
settings using the two-wire I2C serial interface. This
device has an on-board programmable gain amplifier
(PGA). The user can select the PGA gain of x1, x2, x4,
or x8 before the analog-to-digital conversion takes
place. This allows the MCP3421 device to convert a
smaller input signal with high resolution. The device
has two conversion modes: (a) Continuous mode and
(b) One-Shot mode. In One-Shot mode, the device
enters a low current standby mode automatically after
one conversion. This reduces current consumption
greatly during idle periods.
The MCP3421 device can be used for various high
accuracy analog-to-digital data conversion applications
where design simplicity, low power, and small footprint
are major considerations.
Block Diagram
1
2
3 4
5
VIN+ 6
VSS
SCL
VINVDD
SDA
SOT-23-6 Top View
VSS VDD
VIN+
VINSCL
SDA
Voltage Reference
Clock
(2.048V)
I2C Interface
Gain = 1, 2, 4, or 8 VREF
ΔΣ ADC
PGA Converter Oscillator
18-Bit Analog-to-Digital Converter
with I2C Interface and On-Board Reference
MCP3421
DS22003B-page 2 © 2006 Microchip Technology Inc.
1.0 ELECTRICAL
CHARACTERISTICS
Absolute Maximum Ratings†
VDD...................................................................................7.0V
All inputs and outputs w.r.t VSS ............... –0.3V to VDD+0.3V
Differential Input Voltage ...................................... |VDD - VSS|
Output Short Circuit Current .................................Continuous
Current at Input Pins ....................................................±2 mA
Current at Output and Supply Pins ............................±10 mA
Storage Temperature.....................................-65°C to +150°C
Ambient Temp. with power applied ...............-55°C to +125°C
ESD protection on all pins ................ ≥ 6 kV HBM, ≥ 400V MM
Maximum Junction Temperature (TJ) ..........................+150°C
†Notice: Stresses above those listed under “Maximum Ratings”
may cause permanent damage to the device. This is a
stress rating only and functional operation of the device at
those or any other conditions above those indicated in the
operational listings of this specification is not implied.
Exposure to maximum rating conditions for extended periods
may affect device reliability.
ELECTRICAL CHARACTERISTICS
Electrical Specifications: Unless otherwise specified, all parameters apply for TA = -40°C to +85°C, VDD = +5.0V, VSS = 0V,
VIN+ = VIN- = VREF/2. All ppm units use 2*VREF as full-scale range.
Parameters Sym Min Typ Max Units Conditions
Analog Inputs
Differential Input Range — ±2.048/PGA — V VIN = VIN+ - VINCommon-
Mode Voltage Range
(absolute) (Note 1)
VSS-0.3 — VDD+0.3 V
Differential Input Impedance
(Note 2)
ZIND (f) — 2.25/PGA — MΩ During normal mode operation
Common Mode input
Impedance
ZINC (f) — 25 — MΩ PGA = 1, 2, 4, 8
System Performance
Resolution and No Missing
Codes (Note 8)
12 — — Bits DR = 240 SPS
14 — — Bits DR = 60 SPS
16 — — Bits DR = 15 SPS
18 — — Bits DR = 3.75 SPS
Data Rate (Note 3) DR 176 240 328 SPS S1,S0 = ‘00’, (12 bits mode)
44 60 82 SPS S1,S0 = ‘01’, (14 bits mode)
11 15 20.5 SPS S1,S0 = ‘10’, (16 bits mode)
2.75 3.75 5.1 SPS S1,S0 = ‘11’, (18 bits mode)
Output Noise — 1.5 — μVRMS TA = 25°C, DR = 3.75 SPS,
PGA = 1, VIN = 0
Note 1: Any input voltage below or greater than this voltage causes leakage current through the ESD diodes at the input pins.
This parameter is ensured by characterization and not 100% tested.
2: This input impedance is due to 3.2 pF internal input sampling capacitor.
3: The total conversion speed includes auto-calibration of offset and gain.
4: INL is the difference between the endpoints line and the measured code at the center of the quantization band.
5: Includes all errors from on-board PGA and VREF.
6: Full Scale Range (FSR) = 2 x 2.048/PGA = 4.096/PGA.
7: This parameter is ensured by characterization and not 100% tested.
8: This parameter is ensured by design and not 100% tested.
© 2006 Microchip Technology Inc. DS22003B-page 3
MCP3421
Integral Nonlinearity (Note 4) INL — 10 35 ppm of
FSR
DR = 3.75 SPS
(Note 6)
Internal Reference Voltage VREF — 2.048 — V
Gain Error (Note 5) — 0.05 0.35 % PGA = 1, DR = 3.75 SPS
PGA Gain Error Match (Note 5) — 0.1 — % Between any 2 PGA gains
Gain Error Drift (Note 5) — 5 40 ppm/°C PGA=1, DR=3.75 SPS
Offset Error VOS — 15 40 μV Tested at PGA = 1
VDD = 5.0V and DR = 3.75 SPS
Offset Drift vs. Temperature — 50 — nV/°C VDD = 5.0V
Common-Mode Rejection — 105 — dB at DC and PGA =1,
— 110 — dB at DC and PGA =8,
TA = +25°C
Gain vs. VDD — 5 — ppm/V TA = +25°C, VDD = 2.7V to 5.5V,
PGA = 1
Power Supply Rejection at DC — 100 — dB TA = +25°C, VDD = 2.7V to 5.5V,
PGA = 1
Power Requirements
Voltage Range VDD 2.7 — 5.5 V
Supply Current during
Conversion
IDDA — 155 190 μA VDD = 5.0V
— 145 — μA VDD = 3.0V
Supply Current during Standby
Mode
IDDS — 0.1 0.5 μA
I2C Digital Inputs and Digital Outputs
High level input voltage VIH 0.7 VDD — VDD V
Low level input voltage VIL — — 0.3VDD V
Low level output voltage VOL — — 0.4 V IOL = 3 mA, VDD = +5.0V
Hysteresis of Schmitt Trigger
for inputs (Note 7)
VHYST 0.05VDD — — V fSCL = 100 kHz
Supply Current when I2C bus
line is active
IDDB — — 10 μA
Input Leakage Current IILH — — 1 μA VIH = 5.5V
IILL -1 — — μA VIL = GND
Pin Capacitance and I2C Bus Capacitance
Pin capacitance CPIN — — 10 pF
I2C Bus Capacitance Cb — — 400 pF
Thermal Characteristics
Specified Temperature Range TA -40 — +85 °C
Operating Temperature Range TA -40 — +125 °C
Storage Temperature Range TA -65 — +150 °C
ELECTRICAL CHARACTERISTICS (CONTINUED)
Electrical Specifications: Unless otherwise specified, all parameters apply for TA = -40°C to +85°C, VDD = +5.0V, VSS = 0V,
VIN+ = VIN- = VREF/2. All ppm units use 2*VREF as full-scale range.
Parameters Sym Min Typ Max Units Conditions
Note 1: Any input voltage below or greater than this voltage causes leakage current through the ESD diodes at the input pins.
This parameter is ensured by characterization and not 100% tested.
2: This input impedance is due to 3.2 pF internal input sampling capacitor.
3: The total conversion speed includes auto-calibration of offset and gain.
4: INL is the difference between the endpoints line and the measured code at the center of the quantization band.
5: Includes all errors from on-board PGA and VREF.
6: Full Scale Range (FSR) = 2 x 2.048/PGA = 4.096/PGA.
7: This parameter is ensured by characterization and not 100% tested.
8: This parameter is ensured by design and not 100% tested.
MCP3421
DS22003B-page 4 © 2006 Microchip Technology Inc.
2.0 TYPICAL PERFORMANCE CURVES
Note: Unless otherwise indicated, TA = -40°C to +85°C, VDD = +5.0V, VSS = 0V, VIN+ = VIN- = VREF/2.
FIGURE 2-1: INL vs. Supply Voltage
(VDD).
FIGURE 2-2: INL vs. Temperature.
FIGURE 2-3: Offset Error vs. Temperature.
FIGURE 2-4: Noise vs. Input Voltage.
FIGURE 2-5: Total Error vs. Input Voltage.
FIGURE 2-6: Gain Error vs. Temperature.
Note: The graphs and tables provided following this note are a statistical summary based on a limited number of
samples and are provided for informational purposes only. The performance characteristics listed herein
are not tested or guaranteed. In some graphs or tables, the data presented may be outside the specified
operating range (e.g., outside specified power supply range) and therefore outside the warranted range.
.000
.001
.002
.003
.004
.005
2.5 3 3.5 4 4.5 5 5.5
VDD (V)
PGA = 1
PGA = 2
PGA = 8
PGA = 4
Integral Nonlinearity (% of FSR)
0
0.001
0.002
0.003
-60 -40 -20 0 20 40 60 80 100 120 140
Temperature (oC)
Integral Nonlinearity
(% of FSR)
VDD = 5 V
VDD = 2.7V
PGA = 1
-20
-15
-10
-5
0
5
10
15
20
-60 -40 -20 0 20 40 60 80 100 120 140
Temperature (°C)
Offset Error (μV)
VDD = 5V
PGA = 1
PGA = 2
PGA = 4 PGA = 8
0.0
2.5
5.0
7.5
10.0
-100 -75 -50 -25 0 25 50 75 100
Input Voltage (% of Full-Scale)
Noise (μV, rms)
PGA = 1
PGA = 2
PGA = 8
PGA = 4
TA = +25°C
VDD = 5V
-3.0
-2.0
-1.0
0.0
1.0
2.0
3.0
-100 -75 -50 -25 0 25 50 75 100
Input Voltage (% of Full-Scale)
Total Error (mV)
PGA = 1
PGA = 2
PGA = 8
PGA = 4
-0.4
-0.3
-0.2
-0.1
0
0.1
0.2
0.3
0.4
-60 -40 -20 0 20 40 60 80 100 120 140
Temperature (°C)
Gain Error (% of FSR)
VDD = 5.0V
PGA = 1
PGA = 2
PGA = 8
PGA = 4
© 2006 Microchip Technology Inc. DS22003B-page 5
MCP3421
Note: Unless otherwise indicated, TA = -40°C to +85°C, VDD = +5.0V, VSS = 0V, VIN+ = VIN- = VREF/2.
FIGURE 2-7: IDDA vs. Temperature.
FIGURE 2-8: IDDS vs. Temperature.
FIGURE 2-9: IDDB vs. Temperature.
FIGURE 2-10: OSC Drift vs. Temperature.
FIGURE 2-11: Frequency Response.
100
120
140
160
180
200
220
-60 -40 -20 0 20 40 60 80 100 120 140
Temperature (oC)
IDDA (μA)
VDD = 5V
VDD = 2.7V
0
100
200
300
400
500
600
-60 -40 -20 0 20 40 60 80 100 120 140
Temperature (oC)
IDDS (nA)
VDD = 2.7V
VDD = 5V
0
1
2
3
4
5
6
7
8
9
-60 -40 -20 0 20 40 60 80 100 120 140
Temperature (oC)
IDDB (A)
VDD = 5V
VDD = 4.5V
VDD = 3.3V
VDD = 2.7V
-1
0
1
2
3
4
5
-60 -40 -20 0 20 40 60 80 100 120 140
Temperature (°C)
Oscillator Drift (%)
VDD = 5.0V
VDD = 2.7V
Data Rate = 3.75 SPS
-120
-110
-100
-90
-80
-70
-60
-50
-40
-30
-20
-10
0
0.1 1 10 100 1000 10000
Input Signal Frequency (Hz)
Magnitude (dB)
0.1 1 10 100 1k 10k
MCP3421
DS22003B-page 6 © 2006 Microchip Technology Inc.
3.0 PIN DESCRIPTIONS
TABLE 3-1: PIN FUNCTION TABLE
3.1 Analog Inputs (VIN+, VIN-)
VIN+ and VIN- are differential signal input pins. The
MCP3421 device accepts a fully differential analog
input signal which is connected on the VIN+ and VINinput
pins. The differential voltage that is converted is
defined by VIN = (VIN+ - VIN-) where VIN+ is the voltage
applied at the VIN+ pin and VIN- is the voltage applied
at the VIN- pin. The input signal level is amplified by the
programmable gain amplifier (PGA) before the
conversion. The differential input voltage should not
exceed an absolute of (2* VREF/PGA) for accurate
measurement, where VREF is the internal reference
voltage (2.048V) and PGA is the PGA gain setting. The
converter output code will saturate if the input range
exceeds (2* VREF/PGA).
The absolute voltage range on each of the differential
input pins is from VSS-0.3V to VDD+0.3V. Any voltage
above or below this range will cause leakage currents
through the Electrostatic Discharge (ESD) diodes at
the input pins. This ESD current can cause unexpected
performance of the device. The common mode of the
analog inputs should be chosen such that both the
differential analog input range and the absolute voltage
range on each pin are within the specified operating
range defined in Section 1.0 “Electrical
Characteristics” and Section 4.0 “Description of
Device Operation”.
3.2 Supply Voltage (VDD, VSS)
VDD is the power supply pin for the device. This pin
requires an appropriate bypass capacitor of about
0.1 μF (ceramic) to ground. An additional 10 μF
capacitor (tantalum) in parallel is also recommended
to further attenuate high frequency noise present in
some application boards. The supply voltage (VDD)
must be maintained in the 2.7V to 5.5V range for specified
operation.
VSS is the ground pin and the current return path of the
device. The user must connect the VSS pin to a ground
plane through a low impedance connection. If an
analog ground path is available in the application PCB
(printed circuit board), it is highly recommended that
the VSS pin be tied to the analog ground path or
isolated within an analog ground plane of the circuit
board.
3.3 Serial Clock Pin (SCL)
SCL is the serial clock pin of the I2C interface. The
MCP3421 acts only as a slave and the SCL pin
accepts only external serial clocks. The input data
from the Master device is shifted into the SDA pin on
the rising edges of the SCL clock and output from the
MCP3421 occurs at the falling edges of the SCL clock.
The SCL pin is an open-drain N-channel driver.
Therefore, it needs a pull-up resistor from the VDD line
to the SCL pin. Refer to Section 5.3 “I2C Serial Communications”
for more details of I2C Serial Interface
communication.
3.4 Serial Data Pin (SDA)
SDA is the serial data pin of the I2C interface. The SDA
pin is used for input and output data. In read mode, the
conversion result is read from the SDA pin (output). In
write mode, the device configuration bits are written
(input) though the SDA pin. The SDA pin is an opendrain
N-channel driver. Therefore, it needs a pull-up
resistor from the VDD line to the SDA pin. Except for
start and stop conditions, the data on the SDA pin must
be stable during the high period of the clock. The high
or low state of the SDA pin can only change when the
clock signal on the SCL pin is low. Refer to Section 5.3
“I2C Serial Communications” for more details of I2C
Serial Interface communication.
Pin No Sym Function
1 VIN+ Non-Inverting Analog Input Pin
2 VSS Ground Pin
3 SCL Serial Clock Input Pin of the I2C Interface
4 SDA Bidirectional Serial Data Pin of the I2C Interface
5 VDD Positive Supply Voltage Pin
6 VIN- Inverting Analog Input Pin
© 2006 Microchip Technology Inc. DS22003B-page 7
MCP3421
4.0 DESCRIPTION OF DEVICE
OPERATION
4.1 General Overview
The MCP3421 is a low-power, 18-Bit Delta-Sigma A/D
converter with an I2C serial interface. The device
contains an on-board voltage reference (2.048V),
programmable gain amplifier (PGA), and internal
oscillator. The user can select 12, 14, 16, or 18 bit
conversion by setting the configuration register bits.
The device can be operated in Continuous Conversion
or One-Shot Conversion mode. In the Continuous Conversion
mode, the device converts the inputs
continuously. While in the One-Shot Conversion mode,
the device converts the input one time and stays in the
low-power standby mode until it receives another
command for a new conversion. During the standby
mode, the device consumes less than 0.1 μA typical.
4.2 Power-On-Reset (POR)
The device contains an internal Power-On-Reset
(POR) circuit that monitors power supply voltage (VDD)
during operation. This circuit ensures correct device
start-up at system power-up and power-down events.
The POR has built-in hysteresis and a timer to give a
high degree of immunity to potential ripples and noises
on the power supply. A 0.1 μF decoupling capacitor
should be mounted as close as possible to the VDD pin
for additional transient immunity.
The threshold voltage is set at 2.2V with a tolerance of
approximately ±5%. If the supply voltage falls below
this threshold, the device will be held in a reset
condition. The typical hysteresis value is approximately
200 mV.
The POR circuit is shut-down during the low-power
standby mode. Once a power-up event has occurred,
the device requires additional delay time (approximately
300 μs) before a conversion can take place.
During this time, all internal analog circuitries are
settled before the first conversion occurs. Figure 4-1
illustrates the conditions for power-up and power-down
events under typical start-up conditions.
When the device powers up, it automatically resets
and sets the configuration bits to default settings. The
default configuration bit conditions are a PGA gain of
1 V/V and a conversion speed of 240 SPS in
Continuous Conversion mode. When the device
receives an I2C General Call Reset command, it
performs an internal reset similar to a Power-On-Reset
event.
FIGURE 4-1: POR Operation.
4.3 Internal Voltage Reference
The device contains an on-board 2.048V voltage
reference. This reference voltage is for internal use
only and not directly measurable. The specifications of
the reference voltage are part of the device’s gain and
drift specifications. Therefore, there is no separate
specification for the on-board reference.
4.4 Analog Input Channel
The differential analog input channel has a switched
capacitor structure. The internal sampling capacitor
(3.2 pF) is charged and discharged to process a
conversion. The charging and discharging of the input
sampling capacitor creates dynamic input currents at
the VIN+ and VIN- input pins, which is inversely
proportional to the internal sampling capacitor and
internal frequency. The current is also a function of the
differential input voltages. Care must be taken in setting
the common-mode voltage and input voltage ranges so
that the input limits do not exceed the ranges specified
in Section 1.0 “Electrical Characteristics”.
4.5 Digital Output Code
The digital output code produced by the MCP3421 is a
function of PGA gain, input signal, and internal
reference voltage. In a fixed setting, the digital output
code is proportional to the voltage difference between
the two analog inputs.
The output data format is a binary two’s complement.
With this code scheme, the MSB can be considered a
sign indicator. When the MSB is a logic ‘0’, it indicates
a positive value. When the MSB is a logic ‘1’, it
indicates a negative value. The following is an example
of the output code:
(a) for a negative full-scale input voltage: 100...000
(b) for a zero differential input voltage: 000...000
(c) for a positive full-scale input voltage: 011...111.
The MSB is always transmitted first through the serial
port. The number of data bits for each conversion is 18,
16, 14, or 12 bits depending on the conversion mode
selection.
VDD
2.2V
2.0V
300 μS
Reset Start-up Normal Operation Reset
Time
MCP3421
DS22003B-page 8 © 2006 Microchip Technology Inc.
The output codes will not roll-over if the input voltage
exceeds the maximum input range. In this case, the
code will be locked at 0111...11 for all voltages
greater than +(VREF - 1 LSB) and 1000...00 for
voltages less than -VREF. Table 4-2 shows an example
of output codes of various input levels using 18 bit
conversion mode. Table 4-3 shows an example of
minimum and maximum codes for each data rate
option.
The output code is given by:
EQUATION 4-1:
The LSB of the code is given by:
EQUATION 4-2:
TABLE 4-1: LSB SIZE OF VARIOUS BIT
CONVERSION MODES
TABLE 4-2: EXAMPLE OF OUTPUT CODE
FOR 18 BITS
TABLE 4-3: MINIMUM AND MAXIMUM
CODES
4.6 Self-Calibration
The device performs a self-calibration of offset and
gain for each conversion. This provides reliable
conversion results from conversion-to-conversion over
variations in temperature as well as power supply
fluctuations.
4.7 Input Impedance
The MCP3421 uses a switched-capacitor input stage
using a 3.2 pF sampling capacitor. This capacitor is
switched (charged and discharged) at a rate of the
sampling frequency that is generated by the on-board
clock. The differential mode impedance varies with the
PGA settings. The typical differential input impedance
during a normal mode operation is given by:
Since the sampling capacitor is only switching to the
input pins during a conversion process, the above input
impedance is only valid during conversion periods. In a
low power standby mode, the above impedance is not
presented at the input pins. Therefore, only a leakage
current due to ESD diode is presented at the input pins.
The conversion accuracy can be affected by the input
signal source impedance when any external circuit is
connected to the input pins. The source impedance
adds to the internal impedance and directly affects the
time required to charge the internal sampling capacitor.
Therefore, a large input source impedance connected
to the input pins can increase the system performance
errors such as offset, gain, and integral nonlinearity
(INL) errors. Ideally, the input source impedance
should be zero. This can be achievable by using an
operational amplifier with a closed-loop output
impedance of tens of ohms.
Bit Resolutions LSB (V)
12 bits 1 mV
14 bits 250 μV
16 bits 62.5 μV
18 bits 15.625 μV
Input Voltage (V) Digital Code
≥ VREF 011111111111111111
VREF - 1 LSB 011111111111111111
2 LSB 000000000000000010
1 LSB 000000000000000001
0 000000000000000000
-1 LSB 111111111111111111
-2 LSB 111111111111111110
- VREF 100000000000000000
< -VREF 100000000000000000
Output Code (Max Code + 1)
(VIN+ – VIN-)
2.048V
= × --------------------------------------
LSB 2 × 2.048V
2N = --------------------------
Where:
N = the number of bits
Number
of Bits Data Rate Minimum
Code
Maximum
Code
12 240 SPS -2048 2047
14 60 SPS -8192 8191
16 15 SPS -32768 32767
18 3.75 SPS -131072 131071
Note: Maximum n-bit code = 2n-1 - 1
Minimum n-bit code = -1 x 2n-1
ZIN(f) = 2.25 MΩ/PGA
© 2006 Microchip Technology Inc. DS22003B-page 9
MCP3421
4.8 Aliasing and Anti-aliasing Filter
Aliasing occurs when the input signal contains timevarying
signal components with frequency greater than
half the sample rate. In the aliasing conditions, the
device can output unexpected output codes. For
applications that are operating in electrical noise
environments, the time-varying signal noise or high
frequency interference components can be easily
added to the input signals and cause aliasing. Although
the MCP3421 device has an internal first order sinc
filter, its’ filter response may not give enough
attenuation to all aliasing signal components. To avoid
the aliasing, an external anti-aliasing filter, which can
be accomplished with a simple RC low-pass filter, is
typically used at the input pins. The low-pass filter cuts
off the high frequency noise components and provides
a band-limited input signal to the MCP3421 input pins.
MCP3421
DS22003B-page 10 © 2006 Microchip Technology Inc.
5.0 USING THE MCP3421 DEVICE
5.1 Operating Modes
The user operates the device by setting up the device
configuration register and reads the conversion data
using serial I2C interface commands. The MCP3421
operates in two modes: (a) Continuous Conversion
Mode or (b) One-Shot Conversion Mode (single
conversion). The selection is made by setting the O/C
bit in the Configuration Register. Refer to Section 5.2
“Configuration Register” for more information.
5.1.1 CONTINUOUS CONVERSION
MODE (O/C BIT = 1)
The MCP3421 device performs a Continuous
Conversion if the O/C bit is set to logic “high”. Once the
conversion is completed, the result is placed at the
output data register. The device immediately begins
another conversion and overwrites the output data
register with the most recent data.
The device also clears the data ready flag (RDY bit = 0)
when the conversion is completed. The device sets the
ready flag bit (RDY bit = 1), if the latest conversion
result has been read by the Master.
5.1.2 ONE-SHOT CONVERSION MODE
(O/C BIT = 0)
Once the One-Shot Conversion (single conversion)
Mode is selected, the device performs a conversion,
updates the Output Data register, clears the data ready
flag (RDY = 0), and then enters a low power standby
mode. A new One-Shot Conversion is started again
when the device receives a new write command with
RDY = 1.
This One-Shot Conversion Mode is recommended for
low power operating applications. During the low
current standby mode, the device consumes less than
1 μA typical. For example, if user collects 18 bit
conversion data once a second in One-Shot Conversion
mode, the device draws only about one fourth of
its total operating current. In this example, the device
consumes approximately 39 μA (= ~145 μA/3.75 SPS),
if the device performs only one conversion per second
(1 SPS) in 18-bit conversion mode with 3V power
supply.
© 2006 Microchip Technology Inc. DS22003B-page 11
MCP3421
5.2 Configuration Register
The MCP3421 has an 8-bit wide configuration register
to select for: PGA gain, conversion rate, and conversion
mode. This register allows the user to change the
operating condition of the device and check the status
of the device operation. The user can rewrite the
configuration byte any time during the device
operation. Register 5-1 shows the configuration
register bits.
REGISTER 5-1: CONFIGURATION REGISTER
R/W-1 R/W-0 R/W-0 R/W-1 R/W-0 R/W-0 R/W-0 R/W-0
RDY C1 C0 O/C S1 S0 G1 G0
1 * 0 * 0 * 1 * 0 * 0 * 0 * 0 *
bit 7 bit 0
* Default Configuration after Power-On Reset
Legend:
R = Readable bit W = Writable bit U = Unimplemented bit, read as ‘0’
-n = Value at POR ‘1’ = Bit is set ‘0’ = Bit is cleared x = Bit is unknown
bit 7 RDY: Ready Bit
This bit is the data ready flag. In read mode, this bit indicates if the output register has been updated
with a new conversion. In One-Shot Conversion mode, writing this bit to “1” initiates a new conversion.
Reading RDY bit with the read command:
1 = Output register has not been updated.
0 = Output register has been updated with the latest conversion data.
Writing RDY bit with the write command:
Continuous Conversion mode: No effect
One-Shot Conversion mode:
1 = Initiate a new conversion.
0 = No effect.
bit 6-5 C1-C0: Channel Selection Bits
These are the Channel Selection bits, but not used in the MCP3421 device.
bit 4 O/C: Conversion Mode Bit
1 = Continuous Conversion Mode. Once this bit is selected, the device performs data conversions
continuously.
0 = One-Shot Conversion Mode. The device performs a single conversion and enters a low power
standby mode until it receives another write/read command.
bit 3-2 S1-S0: Sample Rate Selection Bit
00 = 240 SPS (12 bits),
01 = 60 SPS (14 bits),
10 = 15 SPS (16 bits),
11 = 3.75 SPS (18 bits)
bit 1-0 G1-G0: PGA Gain Selector Bits
00 = 1 V/V,
01 = 2 V/V,
10 = 4 V/V,
11 = 8 V/V
MCP3421
DS22003B-page 12 © 2006 Microchip Technology Inc.
In read mode, the RDY bit in the configuration byte
indicates the state of the conversion: (a) RDY = 1
indicates that the data bytes that have just been read
were not updated from the previous conversion. (b)
RDY = 0 indicates that the data bytes that have just
been read were updated.
If the configuration byte is read repeatedly by clocking
continuously after the first read (i.e., after the 5th byte
in the 18-bit conversion mode), the state of the RDY bit
indicates whether the device is ready with new
conversion data. See Figure 5-2. For example,
RDY = 0 means new conversion data is ready for reading.
In this case, the user can send a stop bit to exit the
current read operation and send a new read command
to read out updated conversion data. See Figures 5-2
and 5-3 for reading conversion data. The user can
rewrite the configuration byte any time for a new
setting. Tables 5-1 and 5-2 show the examples of the
configuration bit operation.
5.3 I2C Serial Communications
The MCP3421 device communicates with Master
(microcontroller) through a serial I2C (Inter-Integrated
Circuit) interface and supports standard
(100 kbits/sec), fast (400 kbits/sec) and high-speed
(3.4 Mbits/sec) modes. The serial I2C is a bidirectional
2-wire data bus communication protocol using opendrain
SCL and SDA lines.
The MCP3421 can only be addressed as a slave. Once
addressed, it can receive configuration bits or transmit
the latest conversion results. The serial clock pin (SCL)
is an input only and the serial data pin (SDA) is
bidirectional. An example of a hardware connection
diagram is shown in Figure 6-1.
The Master starts communication by sending a START
bit and terminates the communication by sending a
STOP bit. The first byte after the START bit is always
the address byte of the device, which includes the
device code, the address bits, and the R/W bit. The
device code for the MCP3421 device is 1101. The
address bits (A2, A1, A0) are pre-programmed at the
factory. In general, the address bits are specified by the
customer when they order the device. The three
address bits are programmed to “000” at the factory, if
they are not specified by the customer. Figure 5-1
shows the details of the MCP3421 address byte.
During a low power standby mode, SDA and SCL pins
remain at a floating condition.
More details of the I2C bus characteristic is described
in Section 5.6 “I2C Bus Characteristics”.
5.3.1 DEVICE ADDRESSING
The address byte is the first byte received following the
START condition from the Master device. The
MCP3421 device code is 1101. The device code is
followed by three address bits (A2, A1, A0) which are
programmed at the factory. The three address bits
allow up to eight MCP3421 devices on the same data
bus line. The (R/W) bit determines if the Master device
wants to read the conversion data or write to the
Configuration register. If the (R/W) bit is set (read
mode), the MCP3421 outputs the conversion data in
the following clocks. If the (R/W) bit is cleared (write
mode), the MCP3421 expects a configuration byte in
the following clocks. When the MCP3421 receives the
correct address byte, it outputs an acknowledge bit
after the R/W bit. Figure 5-1 shows the MCP3421
address byte. See Figures 5-2 and 5-3 for the read and
write operations of the device.
TABLE 5-1: CONFIGURATION BITS FOR
WRITING
R/W O/C RDY Operation
0 0 0 No effect if all other bits remain
the same - operation continues
with the previous settings
0 0 1 Initiate One-Shot Conversion
0 1 0 Initiate Continuous Conversion
0 1 1 Initiate Continuous Conversion
TABLE 5-2: CONFIGURATION BITS FOR
READING
R/W O/C RDY Operation
1 0 0 New conversion data in One-
Shot conversion mode has been
just read. The RDY bit remains
low until set by a new write
command.
1 0 1 One-Shot Conversion is in
progress, The conversion data is
not updated yet. The RDY bit
stays high.
1 1 0 New conversion data in Continuous
Conversion mode has been
just read. The RDY bit changes
to high after this read.
1 1 1 The conversion data in Continuous
Conversion mode was
already read. The latest conversion
data is not ready. The RDY
bit stays high until a new
conversion is completed.
© 2006 Microchip Technology Inc. DS22003B-page 13
MCP3421
FIGURE 5-1: MCP3421 Address Byte.
5.3.2 READING DATA FROM THE DEVICE
When the Master sends a read command (R/W = 1),
the MCP3421 outputs the conversion data bytes and
configuration byte. Each byte consists of 8 bits with
one acknowledge (ACK) bit. The ACK bit after the
address byte is issued by the MCP3421 and the ACK
bits after each conversion data bytes are issued by the
Master.
When the device is configured for 18-bit conversion
mode, the device outputs three data bytes followed by
a configuration byte. The first 7 data bits in the first
data byte are the MSB of the conversion data. The
user can ignore the first 6 data bits, and take the 7th
data bit (D17) as the MSB of the conversion data. The
LSB of the 3rd data byte is the LSB of the conversion
data (D0).
If the device is configured for 12, 14, or 16 bit-mode, the
device outputs two data bytes followed by a
configuration byte. In 16 bit-conversion mode, the MSB
of the first data byte is the MSB (D15) of the conversion
data. In 14-bit conversion mode, the first two bits in the
first data byte can be ignored (they are the MSB of the
conversion data), and the 3rd bit (D13) is the MSB of
the conversion data. In 12-bit conversion mode, the
first four bits can be ignored (they are the MSB of the
conversion data), and the 5th bit (D11) of the byte
represents the MSB of the conversion data. Table 5-3
shows an example of the conversion data output of
each conversion mode.
The configuration byte follows the output data byte.
The device outputs the configuration byte as long as
the SCL pulses are received. The device terminates
the current outputs when it receives a Not-Acknowledge
(NAK), a repeated start or a stop bit at any time
during the output bit stream. It is not required to read
the configuration byte. However, the user may read the
configuration byte to check the RDY bit condition to
confirm whether the just received data bytes are
updated conversion data. The user may continuously
send clock (SCL) to repeatedly read the configuration
bytes to check the RDY bit status.
Figures 5-2 and 5-3 show the timing diagrams of the
reading.
5.3.3 WRITING A CONFIGURATION BYTE
TO THE DEVICE
When the Master sends an address byte with the R/W
bit low (R/W = 0), the MCP3421 expects one
configuration byte following the address. Any byte sent
after this second byte will be ignored. The user can
change the operating mode of the device by writing the
configuration register bits.
If the device receives a write command with a new
configuration setting, the device immediately begins a
new conversion and updates the conversion data.
Start bit Read/Write bit
Address Byte
R/W ACK
1 1 0 1 X X X
Device Code Address Bits (Note 1)
Address
Acknowledge bit
Address
Note 1: Specified by customer and programmed at the
factory. If not specified by the customer,
programmed to ‘000’.
TABLE 5-3: EXAMPLE OF CONVERSION DATA OUTPUT OF EACH CONVERSION MODE
Conversion
Mode Conversion Data Output
18-bits MMMMMMMD16 (1st data byte) - D15 ~ D8 (2nd data byte) - D7 ~ D0 (3rd data byte) - Configuration
byte
16-bits MD14~D8 (1st data byte) - D7 ~ D0 (2nd data byte) - Configuration byte
14-bits MMMD12~D8 (1st data byte) - D7 ~ D0 (2nd data byte) - Configuration byte
12-bits MMMMMD10D9D8 (1st data byte) - D7 ~ D0 (2nd data byte) - Configuration byte
Note: M is MSB of the data byte.
MCP3421
DS22003B-page 14 © 2006 Microchip Technology Inc.
FIGURE 5-2: Timing Diagram For Reading From The MCP3421 With 18-Bit Mode.
1 9 1 9 1 9 1 9 1 9
1 9
1 1 0 1 A2 A1 A0 D
ACK by RDY O/C
MCP3421
7
Start Bit by R/W
Master
Repeat of D17 (MSB)
2nd Byte
Upper Data Byte
(Data on Clocks 1-6th
can be ignored)
ACK by
Master
ACK by
Master
ACK by
Master
ACK by
Master
17
D
16
D
15
D
14
D
13
D
12
D
11
D
10
D9
D8
D7
D6
D5
D4
D3
D2
D1
D0
C1
C0
S1
S0
G1
G0
1st Byte
MCP3421 Address Byte
3rd Byte
Middle Data Byte
4th Byte
Lower Data Byte
5th Byte
Configuration Byte
(Optional)
C1
C0
S1
S0
G1
G0
NAK by
Master
Stop Bit by
Master
(Optional)
Nth Repeated Byte:
Configuration Byte
Note: – MCP3421 device code is 1101.
– Address Bits A2- A0 = 000 are programmed at the factory unless customer requests specific codes.
– Stop bit or NAK bit can be issued any time during reading.
– Data bits on clocks 1 - 6th in 2nd byte are repeated MSB and can be ignored.
SCL
SDA
RDY O/C
© 2006 Microchip Technology Inc. DS22003B-page 15
MCP3421
FIGURE 5-3: Timing Diagram For Reading From The MCP3421 With 12-Bit to 16-Bit Modes.
1 1 0 1 A2 A1 A0
ACK by
MCP3421
Start Bit by
Master
2nd Byte
Middle Data Byte
ACK by
Master
ACK by
Master
ACK by
Master
D
15
D
14
D
13
D
12
D
11
D
10
D9
D8
D7
D6
D5
D4
D3
D2
D1
D0
C1
C0
S1
S0
G1
G0
1st Byte
MCP3421 Address Byte
3rd Byte
Lower Data Byte
4th Byte
Configuration Byte
(Optional)
C1
C0
S1
S0
G1
G0
NAK by
Master
Stop Bit by
Master
(Optional)
Nth Repeated Byte:
Configuration Byte
Note: – MCP3421 device code is 1101.
– Address Bits A2- A0 = 000 are programmed at the factory unless customer requests specific codes.
– Stop bit or NAK bit can be issued any time during reading.
– In 14 - bit mode: D15 and D14 are repeated MSB and can be ignored.
– In 12 - bit mode: D15 - D12 are repeated MSB and can be ignored.
1 9 1 9 1 9 1 9
SCL
SDA
1 9
R/W RDY O/C
RDY O/C
MCP3421
DS22003B-page 16 © 2006 Microchip Technology Inc.
FIGURE 5-4: Timing Diagram For Writing To The MCP3421.
5.4 General Call
The MCP3421 acknowledges the general call address
(0x00 in the first byte). The meaning of the general call
address is always specified in the second byte. Refer
to Figure 5-5. The MCP3421 supports the following
general calls:
5.4.1 GENERAL CALL RESET
The general call reset occurs if the second byte is
‘00000110’ (06h). At the acknowledgement of this
byte, the device will abort current conversion and
perform an internal reset similar to a power-on-reset
(POR).
5.4.2 GENERAL CALL CONVERSION
The general call conversion occurs if the second byte
is ‘00001000’ (08h). All devices on the bus initiate a
conversion simultaneously. For the MCP3421 device,
the configuration will be set to the One-Shot Conversion
mode and a single conversion will be performed.
The PGA and data rate settings are unchanged with
this general call.
FIGURE 5-5: General Call Address
Format.
For more information on the general call, or other I2C
modes, please refer to the Phillips I2C specification.
1 9 1 9
Stop Bit by
1 1 0 1 A2 A1 A0
R/W ACK by
MCP3421
RDY
C1 C0
O/C
S1 S0 G1 G0
1st Byte:
2nd Byte:
Master
ACK by
MCP3421
MCP3421 Address Byte
Configuration Byte
Start Bit by
Master
with Write command
Note: – Stop bit can be issued any time during writing.
– MCP3421 device code is 1101.
– Address Bits A2- A0 = 000 are programmed at factory unless customer requests different codes.
SCL
SDA
Note: The I2C specification does not allow to use
“00000000” (00h) in the second byte.
LSB
First Byte
ACK
0 0 0 0 0 0 0 0 A x x x x x x x x A
(General Call Address)
Second Byte
ACK
© 2006 Microchip Technology Inc. DS22003B-page 17
MCP3421
5.5 High-Speed (HS) Mode
The I2C specification requires that a high-speed mode
device must be ‘activated’ to operate in high-speed
mode. This is done by sending a special address byte
of 00001XXX following the START bit. The XXX bits are
unique to the High-Speed (HS) mode Master. This byte
is referred to as the High-Speed (HS) Master Mode
Code (HSMMC). The MCP3421 device does not
acknowledge this byte. However, upon receiving this
code, the MCP3421 switches on its HS mode filters
and communicates up to 3.4 MHz on SDA and SCL.
The device will switch out of the HS mode on the next
STOP condition.
For more information on the HS mode, or other I2C
modes, please refer to the Phillips I2C specification.
5.6 I2C Bus Characteristics
The I2C specification defines the following bus
protocol:
• Data transfer may be initiated only when the bus
is not busy.
• During data transfer, the data line must remain
stable whenever the clock line is HIGH. Changes
in the data line while the clock line is HIGH will be
interpreted as a START or STOP condition.
Accordingly, the following bus conditions have been
defined using Figure 5-6.
5.6.1 BUS NOT BUSY (A)
Both data and clock lines remain HIGH.
5.6.2 START DATA TRANSFER (B)
A HIGH to LOW transition of the SDA line while the
clock (SCL) is HIGH determines a START condition. All
commands must be preceded by a START condition.
5.6.3 STOP DATA TRANSFER (C)
A LOW to HIGH transition of the SDA line while the
clock (SCL) is HIGH determines a STOP condition. All
operations can be ended with a STOP condition.
5.6.4 DATA VALID (D)
The state of the data line represents valid data when,
after a START condition, the data line is stable for the
duration of the HIGH period of the clock signal.
The data on the line must be changed during the LOW
period of the clock signal. There is one clock pulse per
bit of data.
Each data transfer is initiated with a START condition
and terminated with a STOP condition.
5.6.5 ACKNOWLEDGE
The Master (microcontroller) and the slave (MCP3421)
use an acknowledge pulse as a hand shake of
communication for each byte. The ninth clock pulse of
each byte is used for the acknowledgement. The
acknowledgement is achieved by pulling-down the
SDA line “LOW” during the 9th clock pulse. The clock
pulse is always provided by the Master (microcontroller)
and the acknowledgement is issued by the
receiving device of the byte (Note: The transmitting
device must release the SDA line (“HIGH”) during the
acknowledge pulse.). For example, the slave
(MCP3421) issues the acknowledgement (bring down
the SDA line “LOW”) after the end of each receiving
byte, and the master (microcontroller) issues the
acknowledgement when it reads data from the Slave
(MCP3421).
When the MCP3421 is addressed, it generates an
acknowledge after receiving each byte successfully.
The Master device (microcontroller) must provide an
extra clock pulse (9th pulse of each byte) for the
acknowledgement from the MCP3421 (slave).
The MCP3421 (slave) pulls-down the SDA line during
the acknowledge clock pulse in such a way that the
SDA line is stable low during the high period of the
acknowledge clock pulse.
During reads, the Master (microcontroller) can
terminate the current read operation by not providing
an acknowledge bit on the last byte that has been
clocked out from the MCP3421. In this case, the
MCP3421 releases the SDA line to allow the master
(microcontroller) to generate a STOP or repeated
START condition.
FIGURE 5-6: Data Transfer Sequence on the Serial Bus.
SCL
SDA
(A) (B) (D) (D) (C) (A)
START
CONDITION
ADDRESS OR
ACKNOWLEDGE
VALID
DATA
ALLOWED
TO CHANGE
STOP
CONDITION
MCP3421
DS22003B-page 18 © 2006 Microchip Technology Inc.
TABLE 5-4: I2C SERIAL TIMING SPECIFICATIONS
Electrical Specifications: Unless otherwise specified, all limits are specified for TA = -40 to +85°C, VDD = +2.7V, +3.3V or +5.0V,
VSS = 0V, VIN+ = VIN- = VREF/2.
Parameters Sym Min Typ Max Units Conditions
Standard Mode
Clock frequency fSCL 0 — 100 kHz
Clock high time THIGH 4000 — — ns
Clock low time TLOW 4700 — — ns
SDA and SCL rise time (Note 1) TR — — 1000 ns From VIL to VIH
SDA and SCL fall time (Note 1) TF — — 300 ns From VIH to VIL
START condition hold time THD:STA 4000 — — ns After this period, the first clock
pulse is generated.
Repeated START condition
setup time
TSU:STA 4700 — — ns Only relevant for repeated Start
condition
Data hold time (Note 3) THD:DAT 0 — 3450 ns
Data input setup time TSU:DAT 250 — — ns
STOP condition setup time TSU:STO 4000 — — ns
STOP condition hold time THD:STD 4000 — — ns
Output valid from clock
(Notes 2 and 3)
TAA 0 — 3750 ns
Bus free time TBUF 4700 — — ns Time between START and STOP
conditions.
Fast Mode
Clock frequency TSCL 0 — 400 kHz
Clock high time THIGH 600 — — ns
Clock low time TLOW 1300 — — ns
SDA and SCL rise time (Note 1) TR 20 + 0.1Cb — 300 ns From VIL to VIH
SDA and SCL fall time (Note 1) TF 20 + 0.1Cb — 300 ns From VIH to VIL
START condition hold time THD:STA 600 — — ns After this period, the first clock
pulse is generated
Repeated START condition
setup time
TSU:STA 600 — — ns Only relevant for repeated Start
condition
Data hold time (Note 4) THD:DAT 0 — 900 ns
Data input setup time TSU:DAT 100 — — ns
STOP condition setup time TSU:STO 600 — — ns
STOP condition hold time THD:STD 600 — — ns
Output valid from clock
(Notes 2 and 3)
TAA 0 — 1200 ns
Bus free time TBUF 1300 — — ns Time between START and STOP
conditions.
Input filter spike suppression
(Note 5)
TSP 0 — 50 ns SDA and SCL pins
Note 1: This parameter is ensured by characterization and not 100% tested.
2: This specification is not a part of the I2C specification. This specification is equivalent to the Data Hold Time (THD:DAT)
plus SDA Fall (or rise) time: TAA = THD:DAT + TF (OR TR).
3: If this parameter is too short, it can create an unintended Start or Stop condition to other devices on the bus line. If this
parameter is too long, Clock Low time (TLOW) can be affected.
4: For Data Input: This parameter must be longer than tSP. If this parameter is too long, the Data Input Setup (TSU:DAT) or
Clock Low time (TLOW) can be affected.
For Data Output: This parameter is characterized, and tested indirectly by testing TAA parameter.
5: This parameter is ensured by characterization and not 100% tested. This parameter is not available for Standard Mode.
© 2006 Microchip Technology Inc. DS22003B-page 19
MCP3421
High Speed Mode
Clock frequency fSCL 0 — 3.4
1.7
MHz
MHz
Cb = 100 pF
Cb = 400 pF
Clock high time THIGH 60
120
— — ns
ns
Cb = 100 pF
Cb = 400 pF
Clock low time TLOW 160
320
— — ns Cb = 100 pF
Cb = 400 pF
SCL rise time (Note 1) TR — — 40
80
ns From VIL to VIH,Cb = 100 pF
Cb = 400 pF
SCL fall time (Note 1) TF — — 40
80
ns From VIH to VIL,Cb = 100 pF
Cb = 400 pF
SDA rise time (Note 1) TR: DAT — — 80
160
ns From VIL to VIH,Cb = 100 pF
Cb = 400 pF
SDA fall time (Note 1) TF: DATA — — 80
160
ns From VIH to VIL,Cb = 100 pF
Cb = 400 pF
START condition hold time THD:STA 160 — — ns After this period, the first clock
pulse is generated
Repeated START condition
setup time
TSU:STA 160 — — ns Only relevant for repeated Start
condition
Data hold time (Note 4) THD:DAT 00
— 70
150
ns Cb = 100 pF
Cb = 400 pF
Data input setup time TSU:DAT 10 — — ns
STOP condition setup time TSU:STO 160 — — ns
STOP condition hold time THD:STD 160 — — ns
Output valid from clock
(Notes 2 and 3)
TAA — — 150
310
ns Cb = 100 pF
Cb = 400 pF
Bus free time TBUF 160 — — ns Time between START and STOP
conditions.
Input filter spike suppression
(Note 5)
TSP 0 — 10 ns SDA and SCL pins
TABLE 5-4: I2C SERIAL TIMING SPECIFICATIONS (CONTINUED)
Electrical Specifications: Unless otherwise specified, all limits are specified for TA = -40 to +85°C, VDD = +2.7V, +3.3V or +5.0V,
VSS = 0V, VIN+ = VIN- = VREF/2.
Parameters Sym Min Typ Max Units Conditions
Note 1: This parameter is ensured by characterization and not 100% tested.
2: This specification is not a part of the I2C specification. This specification is equivalent to the Data Hold Time (THD:DAT)
plus SDA Fall (or rise) time: TAA = THD:DAT + TF (OR TR).
3: If this parameter is too short, it can create an unintended Start or Stop condition to other devices on the bus line. If this
parameter is too long, Clock Low time (TLOW) can be affected.
4: For Data Input: This parameter must be longer than tSP. If this parameter is too long, the Data Input Setup (TSU:DAT) or
Clock Low time (TLOW) can be affected.
For Data Output: This parameter is characterized, and tested indirectly by testing TAA parameter.
5: This parameter is ensured by characterization and not 100% tested. This parameter is not available for Standard Mode.
MCP3421
DS22003B-page 20 © 2006 Microchip Technology Inc.
FIGURE 5-7: I2C Bus Timing Data.
TF
SCL
SDA
TSU:STA
TSP
THD:STA
TLOW
THIGH
THD:DAT
TAA
TSU:DAT
TR
TSU:STO
TBUF
© 2006 Microchip Technology Inc. DS22003B-page 21
MCP3421
6.0 BASIC APPLICATION
CONFIGURATION
The MCP3421 device can be used for various precision
analog-to-digital converter applications. The device
operates with very simple connections to the
application circuit. The following sections discuss the
examples of the device connections and applications.
6.1 Connecting to the Application
Circuits
6.1.1 INPUT VOLTAGE RANGE
The fully differential input signals can be connected to
the VIN+ and VIN- input pins. The input range should be
within absolute common mode input voltage range:
VSS - 0.3V to VDD + 0.3V. Outside this limit, the ESD
protection diode at the input pin begins to conduct and
the error due to input leakage current increases rapidly.
Within this limit, the differential input VIN (= VIN+ - VIN-)
is boosted by the PGA before a conversion takes place.
The MCP3421 can not accept negative input voltages
on the input pins. Figures 6-1 and 6-2 show typical connection
examples for differential inputs and a singleended
input, respectively. For the single-ended input,
the input signal is applied to one of the input pins
(typically connected to the VIN+ pin) while the other
input pin (typically VIN- pin) is grounded. The input
signal range of the single-ended configuration is from
0V to 2.048V. All device characteristics hold for the
single-ended configuration, but this configuration loses
one bit resolution because the input can only stand in
positive half scale. Refer to Section 1.0 “Electrical
Characteristics”.
6.1.2 BYPASS CAPACITORS ON VDD PIN
For accurate measurement, the application circuit
needs a clean supply voltage and must block any noise
signal to the MCP3421 device. Figure 6-1 shows an
example of using two bypass capacitors (a 10 μF
tantalum capacitor and a 0.1 μF ceramic capacitor) in
parallel on the VDD line. These capacitors are helpful to
filter out any high frequency noises on the VDD line and
also provide the momentary bursts of extra currents
when the device needs from the supply. These
capacitors should be placed as close to the VDD pin as
possible (within one inch). If the application circuit has
separate digital and analog power supplies, the VDD
and VSS of the MCP3421 should reside on the analog
plane.
6.1.3 CONNECTING TO I2C BUS USING
PULL-UP RESISTORS
The SCL and SDA pins of the MCP3421 are open-drain
configurations. These pins require a pull-up resistor as
shown in Figure 6-1. The value of these pull-up resistors
depends on the operating speed (standard, fast,
and high speed) and loading capacitance of the I2C bus
line. Higher value of pull-up resistor consumes less
power, but increases the signal transition time (higher
RC time constant) on the bus. Therefore, it can limit the
bus operating speed. The lower value of resistor, on the
other hand, consumes higher power, but allows higher
operating speed. If the bus line has higher capacitance
due to long bus line or high number of devices
connected to the bus, a smaller pull-up resistor is
needed to compensate the long RC time constant. The
pull-up resistor is typically chosen between 1 kΩ and
10 kΩ ranges for standard and fast modes, and less
than 1 kΩ for high speed mode in high loading
capacitance environments.
FIGURE 6-1: Typical Connection Example
for Differential Inputs.
FIGURE 6-2: Typical Connection Example
for Single-Ended Input.
The number of devices connected to the bus is limited
only by the maximum bus capacitance of 400 pF. The
bus loading capacitance affects on the bus operating
speed. For example, the highest bus operating speed
for the 400 pF bus capacitance is 1.7 MHz, and
3.4 MHz for 100 pF. Figure 6-3 shows an example of
multiple device connections.
MCP3421
VIN+ VINVSS
VDD
1
2
3 4
5
6
SCL SDL 0.1 μF 10 μF
R R
Input Signals VDD VDD
TO MCU
Note: R is the pull-up resistor. (MASTER)
MCP3421
VIN+ VINVSS
VDD
1
2
3 4
5
6
SCL SDL 0.1 μF 10 μF
R R
Input Signals
VDD VDD
TO MCU
Note: R is the pull-up resistor. (MASTER)
MCP3421
DS22003B-page 22 © 2006 Microchip Technology Inc.
FIGURE 6-3: Example of Multiple Device
Connection on I2C Bus.
6.2 Device Connection Test
The user can test the presence of the MCP3421 on the
I2C bus line without performing an input data conversion.
This test can be achieved by checking an
acknowledge response from the MCP3421 after sending
a read or write command. Here is an example using
Figure 6-4:
(a) Set the R/W bit “HIGH” in the address byte.
(b) The MCP3421 will then acknowledge by pulling
SDA bus LOW during the ACK clock and then release
the bus back to the I2C Master.
(c) A STOP or repeated START bit can then be issued
from the Master and I2C communication can continue.
FIGURE 6-4: I2C Bus Connection Test.
6.3 Application Examples
The MCP3421 device can be used in a broad range of
sensor and data acquisition applications. Figure 6-5,
shows an example of interfacing with a bridge sensor
for pressure measurement.
FIGURE 6-5: Example of Pressure
Measurement.
In this circuit example, the sensor full scale range is
±7.5 mV with a common mode input voltage of VDD / 2.
This configuration will provide a full 14-bit resolution
across the sensor output range. The alternative circuit
for this amount of accuracy would involve an analog
gain stage prior to a 16-bit ADC.
Figure 6-6 shows an example of temperature measurement
using a thermistor. This example can achieve a
linear response over a 50°C temperature range. This
can be implemented using a standard resistor with 1%
tolerance in series with the thermistor. The value of the
resistor is selected to be equal to the thermistor value
at the mid-point of the desired temperature range.
FIGURE 6-6: Example of Temperature
Measurement.
SDASCL
(24LC01)
Microcontroller
EEPROM
MCP3421
(TC74)
Temperature
Sensor
(PIC16F876)
SCL 1 2 3 4 5 6 7 8 9
SDA 1 1 0 1 A2 A1 A0 1
Start
Bit
Address Byte
Device bits Address bits
R/W
Start
Bit
MCP3421
ACK
Response
NPP301
MCP3421
VIN+ VINVSS
VDD
1
2
3 4
5
6
SCL SDL 0.1 μF 10 μF
R R
VDD VDD
TO MCU
(MASTER)
VDD
10 kΩ
Resistor
10 kΩ
Thermistor
MCP3421
VIN+ VINVSS
VDD
1
2
3 4
5
6
SCL SDL 0.1 μF 10 μF
R R
VDD VDD
TO MCU
(MASTER)
VDD
© 2006 Microchip Technology Inc. DS22003B-page 23
MCP3421
7.0 PACKAGING INFORMATION
7.1 Package Marking Information
Legend: XX...X Customer-specific information
Y Year code (last digit of calendar year)
YY Year code (last 2 digits of calendar year)
WW Week code (week of January 1 is week ‘01’)
NNN Alphanumeric traceability code
Pb-free JEDEC designator for Matte Tin (Sn)
* This package is Pb-free. The Pb-free JEDEC designator ( )
can be found on the outer packaging for this package.
Note: In the event the full Microchip part number cannot be marked on one line, it will
be carried over to the next line, thus limiting the number of available
characters for customer-specific information.
e3
e3
2
5
3 1
4 6
6-Lead SOT-23
XXNN
2
5
3 1
4 6
Example
CA25
MCP3421
DS22003B-page 24 © 2006 Microchip Technology Inc.
6-Lead Plastic Small Outline Transistor (OT) (SOT-23)
Note: For the most current package drawings, please see the Microchip Packaging Specification located at
http://www.microchip.com/packaging
1
D
B
n
E
E1
L
c
β
φ
α
A A2
A1
p1
Mold Draft Angle Bottom β 0 5 10 0 5 10
Mold Draft Angle Top α 0 5 10 0 5 10
Lead Width B .014 .017 .020 0.35 0.43 0.50
Lead Thickness c .004 .006 .008 0.09 0.15 0.20
Foot Angle φ 0 5 10 0 5 10
Foot Length L .014 .018 .022 0.35 0.45 0.55
Overall Length D .110 .116 .122 2.80 2.95 3.10
Molded Package Width E1 .059 .064 .069 1.50 1.63 1.75
Overall Width E .102 .110 .118 2.60 2.80 3.00
Standoff A1 .000 .003 .006 0.00 0.08 0.15
Molded Package Thickness A2 .035 .043 .051 0.90 1.10 1.30
Overall Height A .035 .046 .057 0.90 1.18 1.45
Outside lead pitch p1 .075 BSC 1.90 BSC
Pitch p .038 BSC 0.95 BSC
Number of Pins n 6 6
Dimension Limits MIN NOM MAX MIN NOM MAX
Units INCHES* MILLIMETERS
Dimensions D and E1 do not include mold flash or protrusions. Mold flash or protrusions shall not exceed .005" (0.127mm) per side.
Notes:
JEITA (formerly EIAJ) equivalent: SC-74A
* Controlling Parameter
Drawing No. C04-120
BSC: Basic Dimension. Theoretically exact value shown without tolerances.
See ASME Y14.5M
Revised 09-12-05
© 2006 Microchip Technology Inc. DS22003B-page 27
MCP3421
APPENDIX A: REVISION HISTORY
Revision B (December 2006)
• Changes to Electrical Characteristics tables
• Added characterization data
• Changes to I2C Serial Timing Specification table
• Change to Figure 5-7.
Revision A (August 2006)
• Original Release of this Document.
MCP3421
DS22003B-page 28 © 2006 Microchip Technology Inc.
NOTES:
© 2006 Microchip Technology Inc. DS22003B-page 29
MCP3421
PRODUCT IDENTIFICATION SYSTEM
To order or obtain information, e.g., on pricing or delivery, refer to the factory or the listed sales office.
Device: MCP3421T: Single Channel ΔΣ A/D Converter
(Tape and Reel)
Address Options: XX A2 A1 A0
A0 * = 0 0 0
A1 = 0 0 1
A2 = 0 1 0
A3 = 0 1 1
A4 = 1 0 0
A5 = 1 0 1
A6 = 1 1 0
A7 = 1 1 1
* Default option. Contact Microchip factory for other
address options
Temperature Range: E = -40°C to +125°C
Package: OT = Plastic Small Outline Transistor (SOT-23-6),
6-lead
Examples:
a) MCP3421A0T-E/OT: Tape and Reel,
Single Channel ΔΣ A/D
Converter, SOT-23-6
package.
PART NO. XX X
Address Temperature
Range
Device
/XX
Package
Options
MCP3421
DS22003B-page 30 © 2006 Microchip Technology Inc.
NOTES:
© 2006 Microchip Technology Inc. DS22003B-page 31
Information contained in this publication regarding device
applications and the like is provided only for your convenience
and may be superseded by updates. It is your responsibility to
ensure that your application meets with your specifications.
MICROCHIP MAKES NO REPRESENTATIONS OR
WARRANTIES OF ANY KIND WHETHER EXPRESS OR
IMPLIED, WRITTEN OR ORAL, STATUTORY OR
OTHERWISE, RELATED TO THE INFORMATION,
INCLUDING BUT NOT LIMITED TO ITS CONDITION,
QUALITY, PERFORMANCE, MERCHANTABILITY OR
FITNESS FOR PURPOSE. Microchip disclaims all liability
arising from this information and its use. Use of Microchip
devices in life support and/or safety applications is entirely at
the buyer’s risk, and the buyer agrees to defend, indemnify and
hold harmless Microchip from any and all damages, claims,
suits, or expenses resulting from such use. No licenses are
conveyed, implicitly or otherwise, under any Microchip
intellectual property rights.
Trademarks
The Microchip name and logo, the Microchip logo, Accuron,
dsPIC, KEELOQ, microID, MPLAB, PIC, PICmicro, PICSTART,
PRO MATE, PowerSmart, rfPIC, and SmartShunt are
registered trademarks of Microchip Technology Incorporated
in the U.S.A. and other countries.
AmpLab, FilterLab, Migratable Memory, MXDEV, MXLAB,
SEEVAL, SmartSensor and The Embedded Control Solutions
Company are registered trademarks of Microchip Technology
Incorporated in the U.S.A.
Analog-for-the-Digital Age, Application Maestro, CodeGuard,
dsPICDEM, dsPICDEM.net, dsPICworks, ECAN,
ECONOMONITOR, FanSense, FlexROM, fuzzyLAB,
In-Circuit Serial Programming, ICSP, ICEPIC, Linear Active
Thermistor, Mindi, MiWi, MPASM, MPLIB, MPLINK, PICkit,
PICDEM, PICDEM.net, PICLAB, PICtail, PowerCal,
PowerInfo, PowerMate, PowerTool, REAL ICE, rfLAB,
rfPICDEM, Select Mode, Smart Serial, SmartTel, Total
Endurance, UNI/O, WiperLock and ZENA are trademarks of
Microchip Technology Incorporated in the U.S.A. and other
countries.
SQTP is a service mark of Microchip Technology Incorporated
in the U.S.A.
All other trademarks mentioned herein are property of their
respective companies.
© 2006, Microchip Technology Incorporated, Printed in the
U.S.A., All Rights Reserved.
Printed on recycled paper.
Note the following details of the code protection feature on Microchip devices:
• Microchip products meet the specification contained in their particular Microchip Data Sheet.
• Microchip believes that its family of products is one of the most secure families of its kind on the market today, when used in the
intended manner and under normal conditions.
• There are dishonest and possibly illegal methods used to breach the code protection feature. All of these methods, to our
knowledge, require using the Microchip products in a manner outside the operating specifications contained in Microchip’s Data
Sheets. Most likely, the person doing so is engaged in theft of intellectual property.
• Microchip is willing to work with the customer who is concerned about the integrity of their code.
• Neither Microchip nor any other semiconductor manufacturer can guarantee the security of their code. Code protection does not
mean that we are guaranteeing the product as “unbreakable.”
Code protection is constantly evolving. We at Microchip are committed to continuously improving the code protection features of our
products. Attempts to break Microchip’s code protection feature may be a violation of the Digital Millennium Copyright Act. If such acts
allow unauthorized access to your software or other copyrighted work, you may have a right to sue for relief under that Act.
Microchip received ISO/TS-16949:2002 certification for its worldwide
headquarters, design and wafer fabrication facilities in Chandler and
Tempe, Arizona, Gresham, Oregon and Mountain View, California. The
Company’s quality system processes and procedures are for its PIC®
8-bit MCUs, KEELOQ® code hopping devices, Serial EEPROMs,
microperipherals, nonvolatile memory and analog products. In addition,
Microchip’s quality system for the design and manufacture of
development systems is ISO 9001:2000 certified.
DS22003B-page 32 © 2006 Microchip Technology Inc.
AMERICAS
Corporate Office
2355 West Chandler Blvd.
Chandler, AZ 85224-6199
Tel: 480-792-7200
Fax: 480-792-7277
Technical Support:
http://support.microchip.com
Web Address:
www.microchip.com
Atlanta
Alpharetta, GA
Tel: 770-640-0034
Fax: 770-640-0307
Boston
Westborough, MA
Tel: 774-760-0087
Fax: 774-760-0088
Chicago
Itasca, IL
Tel: 630-285-0071
Fax: 630-285-0075
Dallas
Addison, TX
Tel: 972-818-7423
Fax: 972-818-2924
Detroit
Farmington Hills, MI
Tel: 248-538-2250
Fax: 248-538-2260
Kokomo
Kokomo, IN
Tel: 765-864-8360
Fax: 765-864-8387
Los Angeles
Mission Viejo, CA
Tel: 949-462-9523
Fax: 949-462-9608
Santa Clara
Santa Clara, CA
Tel: 408-961-6444
Fax: 408-961-6445
Toronto
Mississauga, Ontario,
Canada
Tel: 905-673-0699
Fax: 905-673-6509
ASIA/PACIFIC
Asia Pacific Office
Suites 3707-14, 37th Floor
Tower 6, The Gateway
Habour City, Kowloon
Hong Kong
Tel: 852-2401-1200
Fax: 852-2401-3431
Australia - Sydney
Tel: 61-2-9868-6733
Fax: 61-2-9868-6755
China - Beijing
Tel: 86-10-8528-2100
Fax: 86-10-8528-2104
China - Chengdu
Tel: 86-28-8665-5511
Fax: 86-28-8665-7889
China - Fuzhou
Tel: 86-591-8750-3506
Fax: 86-591-8750-3521
China - Hong Kong SAR
Tel: 852-2401-1200
Fax: 852-2401-3431
China - Qingdao
Tel: 86-532-8502-7355
Fax: 86-532-8502-7205
China - Shanghai
Tel: 86-21-5407-5533
Fax: 86-21-5407-5066
China - Shenyang
Tel: 86-24-2334-2829
Fax: 86-24-2334-2393
China - Shenzhen
Tel: 86-755-8203-2660
Fax: 86-755-8203-1760
China - Shunde
Tel: 86-757-2839-5507
Fax: 86-757-2839-5571
China - Wuhan
Tel: 86-27-5980-5300
Fax: 86-27-5980-5118
China - Xian
Tel: 86-29-8833-7250
Fax: 86-29-8833-7256
ASIA/PACIFIC
India - Bangalore
Tel: 91-80-4182-8400
Fax: 91-80-4182-8422
India - New Delhi
Tel: 91-11-4160-8631
Fax: 91-11-4160-8632
India - Pune
Tel: 91-20-2566-1512
Fax: 91-20-2566-1513
Japan - Yokohama
Tel: 81-45-471- 6166
Fax: 81-45-471-6122
Korea - Gumi
Tel: 82-54-473-4301
Fax: 82-54-473-4302
Korea - Seoul
Tel: 82-2-554-7200
Fax: 82-2-558-5932 or
82-2-558-5934
Malaysia - Penang
Tel: 60-4-646-8870
Fax: 60-4-646-5086
Philippines - Manila
Tel: 63-2-634-9065
Fax: 63-2-634-9069
Singapore
Tel: 65-6334-8870
Fax: 65-6334-8850
Taiwan - Hsin Chu
Tel: 886-3-572-9526
Fax: 886-3-572-6459
Taiwan - Kaohsiung
Tel: 886-7-536-4818
Fax: 886-7-536-4803
Taiwan - Taipei
Tel: 886-2-2500-6610
Fax: 886-2-2508-0102
Thailand - Bangkok
Tel: 66-2-694-1351
Fax: 66-2-694-1350
EUROPE
Austria - Wels
Tel: 43-7242-2244-39
Fax: 43-7242-2244-393
Denmark - Copenhagen
Tel: 45-4450-2828
Fax: 45-4485-2829
France - Paris
Tel: 33-1-69-53-63-20
Fax: 33-1-69-30-90-79
Germany - Munich
Tel: 49-89-627-144-0
Fax: 49-89-627-144-44
Italy - Milan
Tel: 39-0331-742611
Fax: 39-0331-466781
Netherlands - Drunen
Tel: 31-416-690399
Fax: 31-416-690340
Spain - Madrid
Tel: 34-91-708-08-90
Fax: 34-91-708-08-91
UK - Wokingham
Tel: 44-118-921-5869
Fax: 44-118-921-5820
WORLDWIDE SALES AND SERVICE
10/19/06© 2006 Microchip Technology Inc. DS22003B-page 1
MCP3421
Features
• 18-bit ΔΣ ADC in a SOT-23-6 package
• Differential input operation
• Self calibration of Internal Offset and Gain per
each conversion
• On-board Voltage Reference:
- Accuracy: 2.048V ± 0.05%
- Drift: 5 ppm/°C
• On-board Programmable Gain Amplifier (PGA):
- Gains of 1,2,4 or 8
• On-board Oscillator
• INL: 10 ppm of FSR (FSR = 4.096V/PGA)
• Programmable Data Rate Options:
- 3.75 SPS (18 bits)
- 15 SPS (16 bits)
- 60 SPS (14 bits)
- 240 SPS (12 bits)
• One-Shot or Continuous Conversion Options
• Low current consumption:
- 145 μA typical
(VDD= 3V, Continuous Conversion)
- 39 μA typical
(VDD= 3V, One-Shot Conversion with 1 SPS)
• Supports I2C Serial Interface:
- Standard, Fast and High Speed Modes
• Single Supply Operation: 2.7V to 5.5V
• Extended Temperature Range: -40°C to 125°C
Typical Applications
• Portable Instrumentation
• Weigh Scales and Fuel Gauges
• Temperature Sensing with RTD, Thermistor, and
Thermocouple
• Bridge Sensing for Pressure, Strain, and Force.
Package Types
Description
The MCP3421 is a single channel low-noise, high
accuracy ΔΣ A/D converter with differential inputs and
up to 18 bits of resolution in a small SOT-23-6 package.
The on-board precision 2.048V reference voltage
enables an input range of ±2.048V differentially
(Δ voltage = 4.096V). The device uses a two-wire I2C
compatible serial interface and operates from a single
2.7V to 5.5V power supply.
The MCP3421 device performs conversion at rates of
3.75, 15, 60, or 240 samples per second (SPS)
depending on the user controllable configuration bit
settings using the two-wire I2C serial interface. This
device has an on-board programmable gain amplifier
(PGA). The user can select the PGA gain of x1, x2, x4,
or x8 before the analog-to-digital conversion takes
place. This allows the MCP3421 device to convert a
smaller input signal with high resolution. The device
has two conversion modes: (a) Continuous mode and
(b) One-Shot mode. In One-Shot mode, the device
enters a low current standby mode automatically after
one conversion. This reduces current consumption
greatly during idle periods.
The MCP3421 device can be used for various high
accuracy analog-to-digital data conversion applications
where design simplicity, low power, and small footprint
are major considerations.
Block Diagram
1
2
3 4
5
VIN+ 6
VSS
SCL
VINVDD
SDA
SOT-23-6 Top View
VSS VDD
VIN+
VINSCL
SDA
Voltage Reference
Clock
(2.048V)
I2C Interface
Gain = 1, 2, 4, or 8 VREF
ΔΣ ADC
PGA Converter Oscillator
18-Bit Analog-to-Digital Converter
with I2C Interface and On-Board Reference
MCP3421
DS22003B-page 2 © 2006 Microchip Technology Inc.
1.0 ELECTRICAL
CHARACTERISTICS
Absolute Maximum Ratings†
VDD...................................................................................7.0V
All inputs and outputs w.r.t VSS ............... –0.3V to VDD+0.3V
Differential Input Voltage ...................................... |VDD - VSS|
Output Short Circuit Current .................................Continuous
Current at Input Pins ....................................................±2 mA
Current at Output and Supply Pins ............................±10 mA
Storage Temperature.....................................-65°C to +150°C
Ambient Temp. with power applied ...............-55°C to +125°C
ESD protection on all pins ................ ≥ 6 kV HBM, ≥ 400V MM
Maximum Junction Temperature (TJ) ..........................+150°C
†Notice: Stresses above those listed under “Maximum Ratings”
may cause permanent damage to the device. This is a
stress rating only and functional operation of the device at
those or any other conditions above those indicated in the
operational listings of this specification is not implied.
Exposure to maximum rating conditions for extended periods
may affect device reliability.
ELECTRICAL CHARACTERISTICS
Electrical Specifications: Unless otherwise specified, all parameters apply for TA = -40°C to +85°C, VDD = +5.0V, VSS = 0V,
VIN+ = VIN- = VREF/2. All ppm units use 2*VREF as full-scale range.
Parameters Sym Min Typ Max Units Conditions
Analog Inputs
Differential Input Range — ±2.048/PGA — V VIN = VIN+ - VINCommon-
Mode Voltage Range
(absolute) (Note 1)
VSS-0.3 — VDD+0.3 V
Differential Input Impedance
(Note 2)
ZIND (f) — 2.25/PGA — MΩ During normal mode operation
Common Mode input
Impedance
ZINC (f) — 25 — MΩ PGA = 1, 2, 4, 8
System Performance
Resolution and No Missing
Codes (Note 8)
12 — — Bits DR = 240 SPS
14 — — Bits DR = 60 SPS
16 — — Bits DR = 15 SPS
18 — — Bits DR = 3.75 SPS
Data Rate (Note 3) DR 176 240 328 SPS S1,S0 = ‘00’, (12 bits mode)
44 60 82 SPS S1,S0 = ‘01’, (14 bits mode)
11 15 20.5 SPS S1,S0 = ‘10’, (16 bits mode)
2.75 3.75 5.1 SPS S1,S0 = ‘11’, (18 bits mode)
Output Noise — 1.5 — μVRMS TA = 25°C, DR = 3.75 SPS,
PGA = 1, VIN = 0
Note 1: Any input voltage below or greater than this voltage causes leakage current through the ESD diodes at the input pins.
This parameter is ensured by characterization and not 100% tested.
2: This input impedance is due to 3.2 pF internal input sampling capacitor.
3: The total conversion speed includes auto-calibration of offset and gain.
4: INL is the difference between the endpoints line and the measured code at the center of the quantization band.
5: Includes all errors from on-board PGA and VREF.
6: Full Scale Range (FSR) = 2 x 2.048/PGA = 4.096/PGA.
7: This parameter is ensured by characterization and not 100% tested.
8: This parameter is ensured by design and not 100% tested.
© 2006 Microchip Technology Inc. DS22003B-page 3
MCP3421
Integral Nonlinearity (Note 4) INL — 10 35 ppm of
FSR
DR = 3.75 SPS
(Note 6)
Internal Reference Voltage VREF — 2.048 — V
Gain Error (Note 5) — 0.05 0.35 % PGA = 1, DR = 3.75 SPS
PGA Gain Error Match (Note 5) — 0.1 — % Between any 2 PGA gains
Gain Error Drift (Note 5) — 5 40 ppm/°C PGA=1, DR=3.75 SPS
Offset Error VOS — 15 40 μV Tested at PGA = 1
VDD = 5.0V and DR = 3.75 SPS
Offset Drift vs. Temperature — 50 — nV/°C VDD = 5.0V
Common-Mode Rejection — 105 — dB at DC and PGA =1,
— 110 — dB at DC and PGA =8,
TA = +25°C
Gain vs. VDD — 5 — ppm/V TA = +25°C, VDD = 2.7V to 5.5V,
PGA = 1
Power Supply Rejection at DC — 100 — dB TA = +25°C, VDD = 2.7V to 5.5V,
PGA = 1
Power Requirements
Voltage Range VDD 2.7 — 5.5 V
Supply Current during
Conversion
IDDA — 155 190 μA VDD = 5.0V
— 145 — μA VDD = 3.0V
Supply Current during Standby
Mode
IDDS — 0.1 0.5 μA
I2C Digital Inputs and Digital Outputs
High level input voltage VIH 0.7 VDD — VDD V
Low level input voltage VIL — — 0.3VDD V
Low level output voltage VOL — — 0.4 V IOL = 3 mA, VDD = +5.0V
Hysteresis of Schmitt Trigger
for inputs (Note 7)
VHYST 0.05VDD — — V fSCL = 100 kHz
Supply Current when I2C bus
line is active
IDDB — — 10 μA
Input Leakage Current IILH — — 1 μA VIH = 5.5V
IILL -1 — — μA VIL = GND
Pin Capacitance and I2C Bus Capacitance
Pin capacitance CPIN — — 10 pF
I2C Bus Capacitance Cb — — 400 pF
Thermal Characteristics
Specified Temperature Range TA -40 — +85 °C
Operating Temperature Range TA -40 — +125 °C
Storage Temperature Range TA -65 — +150 °C
ELECTRICAL CHARACTERISTICS (CONTINUED)
Electrical Specifications: Unless otherwise specified, all parameters apply for TA = -40°C to +85°C, VDD = +5.0V, VSS = 0V,
VIN+ = VIN- = VREF/2. All ppm units use 2*VREF as full-scale range.
Parameters Sym Min Typ Max Units Conditions
Note 1: Any input voltage below or greater than this voltage causes leakage current through the ESD diodes at the input pins.
This parameter is ensured by characterization and not 100% tested.
2: This input impedance is due to 3.2 pF internal input sampling capacitor.
3: The total conversion speed includes auto-calibration of offset and gain.
4: INL is the difference between the endpoints line and the measured code at the center of the quantization band.
5: Includes all errors from on-board PGA and VREF.
6: Full Scale Range (FSR) = 2 x 2.048/PGA = 4.096/PGA.
7: This parameter is ensured by characterization and not 100% tested.
8: This parameter is ensured by design and not 100% tested.
MCP3421
DS22003B-page 4 © 2006 Microchip Technology Inc.
2.0 TYPICAL PERFORMANCE CURVES
Note: Unless otherwise indicated, TA = -40°C to +85°C, VDD = +5.0V, VSS = 0V, VIN+ = VIN- = VREF/2.
FIGURE 2-1: INL vs. Supply Voltage
(VDD).
FIGURE 2-2: INL vs. Temperature.
FIGURE 2-3: Offset Error vs. Temperature.
FIGURE 2-4: Noise vs. Input Voltage.
FIGURE 2-5: Total Error vs. Input Voltage.
FIGURE 2-6: Gain Error vs. Temperature.
Note: The graphs and tables provided following this note are a statistical summary based on a limited number of
samples and are provided for informational purposes only. The performance characteristics listed herein
are not tested or guaranteed. In some graphs or tables, the data presented may be outside the specified
operating range (e.g., outside specified power supply range) and therefore outside the warranted range.
.000
.001
.002
.003
.004
.005
2.5 3 3.5 4 4.5 5 5.5
VDD (V)
PGA = 1
PGA = 2
PGA = 8
PGA = 4
Integral Nonlinearity (% of FSR)
0
0.001
0.002
0.003
-60 -40 -20 0 20 40 60 80 100 120 140
Temperature (oC)
Integral Nonlinearity
(% of FSR)
VDD = 5 V
VDD = 2.7V
PGA = 1
-20
-15
-10
-5
0
5
10
15
20
-60 -40 -20 0 20 40 60 80 100 120 140
Temperature (°C)
Offset Error (μV)
VDD = 5V
PGA = 1
PGA = 2
PGA = 4 PGA = 8
0.0
2.5
5.0
7.5
10.0
-100 -75 -50 -25 0 25 50 75 100
Input Voltage (% of Full-Scale)
Noise (μV, rms)
PGA = 1
PGA = 2
PGA = 8
PGA = 4
TA = +25°C
VDD = 5V
-3.0
-2.0
-1.0
0.0
1.0
2.0
3.0
-100 -75 -50 -25 0 25 50 75 100
Input Voltage (% of Full-Scale)
Total Error (mV)
PGA = 1
PGA = 2
PGA = 8
PGA = 4
-0.4
-0.3
-0.2
-0.1
0
0.1
0.2
0.3
0.4
-60 -40 -20 0 20 40 60 80 100 120 140
Temperature (°C)
Gain Error (% of FSR)
VDD = 5.0V
PGA = 1
PGA = 2
PGA = 8
PGA = 4
© 2006 Microchip Technology Inc. DS22003B-page 5
MCP3421
Note: Unless otherwise indicated, TA = -40°C to +85°C, VDD = +5.0V, VSS = 0V, VIN+ = VIN- = VREF/2.
FIGURE 2-7: IDDA vs. Temperature.
FIGURE 2-8: IDDS vs. Temperature.
FIGURE 2-9: IDDB vs. Temperature.
FIGURE 2-10: OSC Drift vs. Temperature.
FIGURE 2-11: Frequency Response.
100
120
140
160
180
200
220
-60 -40 -20 0 20 40 60 80 100 120 140
Temperature (oC)
IDDA (μA)
VDD = 5V
VDD = 2.7V
0
100
200
300
400
500
600
-60 -40 -20 0 20 40 60 80 100 120 140
Temperature (oC)
IDDS (nA)
VDD = 2.7V
VDD = 5V
0
1
2
3
4
5
6
7
8
9
-60 -40 -20 0 20 40 60 80 100 120 140
Temperature (oC)
IDDB (A)
VDD = 5V
VDD = 4.5V
VDD = 3.3V
VDD = 2.7V
-1
0
1
2
3
4
5
-60 -40 -20 0 20 40 60 80 100 120 140
Temperature (°C)
Oscillator Drift (%)
VDD = 5.0V
VDD = 2.7V
Data Rate = 3.75 SPS
-120
-110
-100
-90
-80
-70
-60
-50
-40
-30
-20
-10
0
0.1 1 10 100 1000 10000
Input Signal Frequency (Hz)
Magnitude (dB)
0.1 1 10 100 1k 10k
MCP3421
DS22003B-page 6 © 2006 Microchip Technology Inc.
3.0 PIN DESCRIPTIONS
TABLE 3-1: PIN FUNCTION TABLE
3.1 Analog Inputs (VIN+, VIN-)
VIN+ and VIN- are differential signal input pins. The
MCP3421 device accepts a fully differential analog
input signal which is connected on the VIN+ and VINinput
pins. The differential voltage that is converted is
defined by VIN = (VIN+ - VIN-) where VIN+ is the voltage
applied at the VIN+ pin and VIN- is the voltage applied
at the VIN- pin. The input signal level is amplified by the
programmable gain amplifier (PGA) before the
conversion. The differential input voltage should not
exceed an absolute of (2* VREF/PGA) for accurate
measurement, where VREF is the internal reference
voltage (2.048V) and PGA is the PGA gain setting. The
converter output code will saturate if the input range
exceeds (2* VREF/PGA).
The absolute voltage range on each of the differential
input pins is from VSS-0.3V to VDD+0.3V. Any voltage
above or below this range will cause leakage currents
through the Electrostatic Discharge (ESD) diodes at
the input pins. This ESD current can cause unexpected
performance of the device. The common mode of the
analog inputs should be chosen such that both the
differential analog input range and the absolute voltage
range on each pin are within the specified operating
range defined in Section 1.0 “Electrical
Characteristics” and Section 4.0 “Description of
Device Operation”.
3.2 Supply Voltage (VDD, VSS)
VDD is the power supply pin for the device. This pin
requires an appropriate bypass capacitor of about
0.1 μF (ceramic) to ground. An additional 10 μF
capacitor (tantalum) in parallel is also recommended
to further attenuate high frequency noise present in
some application boards. The supply voltage (VDD)
must be maintained in the 2.7V to 5.5V range for specified
operation.
VSS is the ground pin and the current return path of the
device. The user must connect the VSS pin to a ground
plane through a low impedance connection. If an
analog ground path is available in the application PCB
(printed circuit board), it is highly recommended that
the VSS pin be tied to the analog ground path or
isolated within an analog ground plane of the circuit
board.
3.3 Serial Clock Pin (SCL)
SCL is the serial clock pin of the I2C interface. The
MCP3421 acts only as a slave and the SCL pin
accepts only external serial clocks. The input data
from the Master device is shifted into the SDA pin on
the rising edges of the SCL clock and output from the
MCP3421 occurs at the falling edges of the SCL clock.
The SCL pin is an open-drain N-channel driver.
Therefore, it needs a pull-up resistor from the VDD line
to the SCL pin. Refer to Section 5.3 “I2C Serial Communications”
for more details of I2C Serial Interface
communication.
3.4 Serial Data Pin (SDA)
SDA is the serial data pin of the I2C interface. The SDA
pin is used for input and output data. In read mode, the
conversion result is read from the SDA pin (output). In
write mode, the device configuration bits are written
(input) though the SDA pin. The SDA pin is an opendrain
N-channel driver. Therefore, it needs a pull-up
resistor from the VDD line to the SDA pin. Except for
start and stop conditions, the data on the SDA pin must
be stable during the high period of the clock. The high
or low state of the SDA pin can only change when the
clock signal on the SCL pin is low. Refer to Section 5.3
“I2C Serial Communications” for more details of I2C
Serial Interface communication.
Pin No Sym Function
1 VIN+ Non-Inverting Analog Input Pin
2 VSS Ground Pin
3 SCL Serial Clock Input Pin of the I2C Interface
4 SDA Bidirectional Serial Data Pin of the I2C Interface
5 VDD Positive Supply Voltage Pin
6 VIN- Inverting Analog Input Pin
© 2006 Microchip Technology Inc. DS22003B-page 7
MCP3421
4.0 DESCRIPTION OF DEVICE
OPERATION
4.1 General Overview
The MCP3421 is a low-power, 18-Bit Delta-Sigma A/D
converter with an I2C serial interface. The device
contains an on-board voltage reference (2.048V),
programmable gain amplifier (PGA), and internal
oscillator. The user can select 12, 14, 16, or 18 bit
conversion by setting the configuration register bits.
The device can be operated in Continuous Conversion
or One-Shot Conversion mode. In the Continuous Conversion
mode, the device converts the inputs
continuously. While in the One-Shot Conversion mode,
the device converts the input one time and stays in the
low-power standby mode until it receives another
command for a new conversion. During the standby
mode, the device consumes less than 0.1 μA typical.
4.2 Power-On-Reset (POR)
The device contains an internal Power-On-Reset
(POR) circuit that monitors power supply voltage (VDD)
during operation. This circuit ensures correct device
start-up at system power-up and power-down events.
The POR has built-in hysteresis and a timer to give a
high degree of immunity to potential ripples and noises
on the power supply. A 0.1 μF decoupling capacitor
should be mounted as close as possible to the VDD pin
for additional transient immunity.
The threshold voltage is set at 2.2V with a tolerance of
approximately ±5%. If the supply voltage falls below
this threshold, the device will be held in a reset
condition. The typical hysteresis value is approximately
200 mV.
The POR circuit is shut-down during the low-power
standby mode. Once a power-up event has occurred,
the device requires additional delay time (approximately
300 μs) before a conversion can take place.
During this time, all internal analog circuitries are
settled before the first conversion occurs. Figure 4-1
illustrates the conditions for power-up and power-down
events under typical start-up conditions.
When the device powers up, it automatically resets
and sets the configuration bits to default settings. The
default configuration bit conditions are a PGA gain of
1 V/V and a conversion speed of 240 SPS in
Continuous Conversion mode. When the device
receives an I2C General Call Reset command, it
performs an internal reset similar to a Power-On-Reset
event.
FIGURE 4-1: POR Operation.
4.3 Internal Voltage Reference
The device contains an on-board 2.048V voltage
reference. This reference voltage is for internal use
only and not directly measurable. The specifications of
the reference voltage are part of the device’s gain and
drift specifications. Therefore, there is no separate
specification for the on-board reference.
4.4 Analog Input Channel
The differential analog input channel has a switched
capacitor structure. The internal sampling capacitor
(3.2 pF) is charged and discharged to process a
conversion. The charging and discharging of the input
sampling capacitor creates dynamic input currents at
the VIN+ and VIN- input pins, which is inversely
proportional to the internal sampling capacitor and
internal frequency. The current is also a function of the
differential input voltages. Care must be taken in setting
the common-mode voltage and input voltage ranges so
that the input limits do not exceed the ranges specified
in Section 1.0 “Electrical Characteristics”.
4.5 Digital Output Code
The digital output code produced by the MCP3421 is a
function of PGA gain, input signal, and internal
reference voltage. In a fixed setting, the digital output
code is proportional to the voltage difference between
the two analog inputs.
The output data format is a binary two’s complement.
With this code scheme, the MSB can be considered a
sign indicator. When the MSB is a logic ‘0’, it indicates
a positive value. When the MSB is a logic ‘1’, it
indicates a negative value. The following is an example
of the output code:
(a) for a negative full-scale input voltage: 100...000
(b) for a zero differential input voltage: 000...000
(c) for a positive full-scale input voltage: 011...111.
The MSB is always transmitted first through the serial
port. The number of data bits for each conversion is 18,
16, 14, or 12 bits depending on the conversion mode
selection.
VDD
2.2V
2.0V
300 μS
Reset Start-up Normal Operation Reset
Time
MCP3421
DS22003B-page 8 © 2006 Microchip Technology Inc.
The output codes will not roll-over if the input voltage
exceeds the maximum input range. In this case, the
code will be locked at 0111...11 for all voltages
greater than +(VREF - 1 LSB) and 1000...00 for
voltages less than -VREF. Table 4-2 shows an example
of output codes of various input levels using 18 bit
conversion mode. Table 4-3 shows an example of
minimum and maximum codes for each data rate
option.
The output code is given by:
EQUATION 4-1:
The LSB of the code is given by:
EQUATION 4-2:
TABLE 4-1: LSB SIZE OF VARIOUS BIT
CONVERSION MODES
TABLE 4-2: EXAMPLE OF OUTPUT CODE
FOR 18 BITS
TABLE 4-3: MINIMUM AND MAXIMUM
CODES
4.6 Self-Calibration
The device performs a self-calibration of offset and
gain for each conversion. This provides reliable
conversion results from conversion-to-conversion over
variations in temperature as well as power supply
fluctuations.
4.7 Input Impedance
The MCP3421 uses a switched-capacitor input stage
using a 3.2 pF sampling capacitor. This capacitor is
switched (charged and discharged) at a rate of the
sampling frequency that is generated by the on-board
clock. The differential mode impedance varies with the
PGA settings. The typical differential input impedance
during a normal mode operation is given by:
Since the sampling capacitor is only switching to the
input pins during a conversion process, the above input
impedance is only valid during conversion periods. In a
low power standby mode, the above impedance is not
presented at the input pins. Therefore, only a leakage
current due to ESD diode is presented at the input pins.
The conversion accuracy can be affected by the input
signal source impedance when any external circuit is
connected to the input pins. The source impedance
adds to the internal impedance and directly affects the
time required to charge the internal sampling capacitor.
Therefore, a large input source impedance connected
to the input pins can increase the system performance
errors such as offset, gain, and integral nonlinearity
(INL) errors. Ideally, the input source impedance
should be zero. This can be achievable by using an
operational amplifier with a closed-loop output
impedance of tens of ohms.
Bit Resolutions LSB (V)
12 bits 1 mV
14 bits 250 μV
16 bits 62.5 μV
18 bits 15.625 μV
Input Voltage (V) Digital Code
≥ VREF 011111111111111111
VREF - 1 LSB 011111111111111111
2 LSB 000000000000000010
1 LSB 000000000000000001
0 000000000000000000
-1 LSB 111111111111111111
-2 LSB 111111111111111110
- VREF 100000000000000000
< -VREF 100000000000000000
Output Code (Max Code + 1)
(VIN+ – VIN-)
2.048V
= × --------------------------------------
LSB 2 × 2.048V
2N = --------------------------
Where:
N = the number of bits
Number
of Bits Data Rate Minimum
Code
Maximum
Code
12 240 SPS -2048 2047
14 60 SPS -8192 8191
16 15 SPS -32768 32767
18 3.75 SPS -131072 131071
Note: Maximum n-bit code = 2n-1 - 1
Minimum n-bit code = -1 x 2n-1
ZIN(f) = 2.25 MΩ/PGA
© 2006 Microchip Technology Inc. DS22003B-page 9
MCP3421
4.8 Aliasing and Anti-aliasing Filter
Aliasing occurs when the input signal contains timevarying
signal components with frequency greater than
half the sample rate. In the aliasing conditions, the
device can output unexpected output codes. For
applications that are operating in electrical noise
environments, the time-varying signal noise or high
frequency interference components can be easily
added to the input signals and cause aliasing. Although
the MCP3421 device has an internal first order sinc
filter, its’ filter response may not give enough
attenuation to all aliasing signal components. To avoid
the aliasing, an external anti-aliasing filter, which can
be accomplished with a simple RC low-pass filter, is
typically used at the input pins. The low-pass filter cuts
off the high frequency noise components and provides
a band-limited input signal to the MCP3421 input pins.
MCP3421
DS22003B-page 10 © 2006 Microchip Technology Inc.
5.0 USING THE MCP3421 DEVICE
5.1 Operating Modes
The user operates the device by setting up the device
configuration register and reads the conversion data
using serial I2C interface commands. The MCP3421
operates in two modes: (a) Continuous Conversion
Mode or (b) One-Shot Conversion Mode (single
conversion). The selection is made by setting the O/C
bit in the Configuration Register. Refer to Section 5.2
“Configuration Register” for more information.
5.1.1 CONTINUOUS CONVERSION
MODE (O/C BIT = 1)
The MCP3421 device performs a Continuous
Conversion if the O/C bit is set to logic “high”. Once the
conversion is completed, the result is placed at the
output data register. The device immediately begins
another conversion and overwrites the output data
register with the most recent data.
The device also clears the data ready flag (RDY bit = 0)
when the conversion is completed. The device sets the
ready flag bit (RDY bit = 1), if the latest conversion
result has been read by the Master.
5.1.2 ONE-SHOT CONVERSION MODE
(O/C BIT = 0)
Once the One-Shot Conversion (single conversion)
Mode is selected, the device performs a conversion,
updates the Output Data register, clears the data ready
flag (RDY = 0), and then enters a low power standby
mode. A new One-Shot Conversion is started again
when the device receives a new write command with
RDY = 1.
This One-Shot Conversion Mode is recommended for
low power operating applications. During the low
current standby mode, the device consumes less than
1 μA typical. For example, if user collects 18 bit
conversion data once a second in One-Shot Conversion
mode, the device draws only about one fourth of
its total operating current. In this example, the device
consumes approximately 39 μA (= ~145 μA/3.75 SPS),
if the device performs only one conversion per second
(1 SPS) in 18-bit conversion mode with 3V power
supply.
© 2006 Microchip Technology Inc. DS22003B-page 11
MCP3421
5.2 Configuration Register
The MCP3421 has an 8-bit wide configuration register
to select for: PGA gain, conversion rate, and conversion
mode. This register allows the user to change the
operating condition of the device and check the status
of the device operation. The user can rewrite the
configuration byte any time during the device
operation. Register 5-1 shows the configuration
register bits.
REGISTER 5-1: CONFIGURATION REGISTER
R/W-1 R/W-0 R/W-0 R/W-1 R/W-0 R/W-0 R/W-0 R/W-0
RDY C1 C0 O/C S1 S0 G1 G0
1 * 0 * 0 * 1 * 0 * 0 * 0 * 0 *
bit 7 bit 0
* Default Configuration after Power-On Reset
Legend:
R = Readable bit W = Writable bit U = Unimplemented bit, read as ‘0’
-n = Value at POR ‘1’ = Bit is set ‘0’ = Bit is cleared x = Bit is unknown
bit 7 RDY: Ready Bit
This bit is the data ready flag. In read mode, this bit indicates if the output register has been updated
with a new conversion. In One-Shot Conversion mode, writing this bit to “1” initiates a new conversion.
Reading RDY bit with the read command:
1 = Output register has not been updated.
0 = Output register has been updated with the latest conversion data.
Writing RDY bit with the write command:
Continuous Conversion mode: No effect
One-Shot Conversion mode:
1 = Initiate a new conversion.
0 = No effect.
bit 6-5 C1-C0: Channel Selection Bits
These are the Channel Selection bits, but not used in the MCP3421 device.
bit 4 O/C: Conversion Mode Bit
1 = Continuous Conversion Mode. Once this bit is selected, the device performs data conversions
continuously.
0 = One-Shot Conversion Mode. The device performs a single conversion and enters a low power
standby mode until it receives another write/read command.
bit 3-2 S1-S0: Sample Rate Selection Bit
00 = 240 SPS (12 bits),
01 = 60 SPS (14 bits),
10 = 15 SPS (16 bits),
11 = 3.75 SPS (18 bits)
bit 1-0 G1-G0: PGA Gain Selector Bits
00 = 1 V/V,
01 = 2 V/V,
10 = 4 V/V,
11 = 8 V/V
MCP3421
DS22003B-page 12 © 2006 Microchip Technology Inc.
In read mode, the RDY bit in the configuration byte
indicates the state of the conversion: (a) RDY = 1
indicates that the data bytes that have just been read
were not updated from the previous conversion. (b)
RDY = 0 indicates that the data bytes that have just
been read were updated.
If the configuration byte is read repeatedly by clocking
continuously after the first read (i.e., after the 5th byte
in the 18-bit conversion mode), the state of the RDY bit
indicates whether the device is ready with new
conversion data. See Figure 5-2. For example,
RDY = 0 means new conversion data is ready for reading.
In this case, the user can send a stop bit to exit the
current read operation and send a new read command
to read out updated conversion data. See Figures 5-2
and 5-3 for reading conversion data. The user can
rewrite the configuration byte any time for a new
setting. Tables 5-1 and 5-2 show the examples of the
configuration bit operation.
5.3 I2C Serial Communications
The MCP3421 device communicates with Master
(microcontroller) through a serial I2C (Inter-Integrated
Circuit) interface and supports standard
(100 kbits/sec), fast (400 kbits/sec) and high-speed
(3.4 Mbits/sec) modes. The serial I2C is a bidirectional
2-wire data bus communication protocol using opendrain
SCL and SDA lines.
The MCP3421 can only be addressed as a slave. Once
addressed, it can receive configuration bits or transmit
the latest conversion results. The serial clock pin (SCL)
is an input only and the serial data pin (SDA) is
bidirectional. An example of a hardware connection
diagram is shown in Figure 6-1.
The Master starts communication by sending a START
bit and terminates the communication by sending a
STOP bit. The first byte after the START bit is always
the address byte of the device, which includes the
device code, the address bits, and the R/W bit. The
device code for the MCP3421 device is 1101. The
address bits (A2, A1, A0) are pre-programmed at the
factory. In general, the address bits are specified by the
customer when they order the device. The three
address bits are programmed to “000” at the factory, if
they are not specified by the customer. Figure 5-1
shows the details of the MCP3421 address byte.
During a low power standby mode, SDA and SCL pins
remain at a floating condition.
More details of the I2C bus characteristic is described
in Section 5.6 “I2C Bus Characteristics”.
5.3.1 DEVICE ADDRESSING
The address byte is the first byte received following the
START condition from the Master device. The
MCP3421 device code is 1101. The device code is
followed by three address bits (A2, A1, A0) which are
programmed at the factory. The three address bits
allow up to eight MCP3421 devices on the same data
bus line. The (R/W) bit determines if the Master device
wants to read the conversion data or write to the
Configuration register. If the (R/W) bit is set (read
mode), the MCP3421 outputs the conversion data in
the following clocks. If the (R/W) bit is cleared (write
mode), the MCP3421 expects a configuration byte in
the following clocks. When the MCP3421 receives the
correct address byte, it outputs an acknowledge bit
after the R/W bit. Figure 5-1 shows the MCP3421
address byte. See Figures 5-2 and 5-3 for the read and
write operations of the device.
TABLE 5-1: CONFIGURATION BITS FOR
WRITING
R/W O/C RDY Operation
0 0 0 No effect if all other bits remain
the same - operation continues
with the previous settings
0 0 1 Initiate One-Shot Conversion
0 1 0 Initiate Continuous Conversion
0 1 1 Initiate Continuous Conversion
TABLE 5-2: CONFIGURATION BITS FOR
READING
R/W O/C RDY Operation
1 0 0 New conversion data in One-
Shot conversion mode has been
just read. The RDY bit remains
low until set by a new write
command.
1 0 1 One-Shot Conversion is in
progress, The conversion data is
not updated yet. The RDY bit
stays high.
1 1 0 New conversion data in Continuous
Conversion mode has been
just read. The RDY bit changes
to high after this read.
1 1 1 The conversion data in Continuous
Conversion mode was
already read. The latest conversion
data is not ready. The RDY
bit stays high until a new
conversion is completed.
© 2006 Microchip Technology Inc. DS22003B-page 13
MCP3421
FIGURE 5-1: MCP3421 Address Byte.
5.3.2 READING DATA FROM THE DEVICE
When the Master sends a read command (R/W = 1),
the MCP3421 outputs the conversion data bytes and
configuration byte. Each byte consists of 8 bits with
one acknowledge (ACK) bit. The ACK bit after the
address byte is issued by the MCP3421 and the ACK
bits after each conversion data bytes are issued by the
Master.
When the device is configured for 18-bit conversion
mode, the device outputs three data bytes followed by
a configuration byte. The first 7 data bits in the first
data byte are the MSB of the conversion data. The
user can ignore the first 6 data bits, and take the 7th
data bit (D17) as the MSB of the conversion data. The
LSB of the 3rd data byte is the LSB of the conversion
data (D0).
If the device is configured for 12, 14, or 16 bit-mode, the
device outputs two data bytes followed by a
configuration byte. In 16 bit-conversion mode, the MSB
of the first data byte is the MSB (D15) of the conversion
data. In 14-bit conversion mode, the first two bits in the
first data byte can be ignored (they are the MSB of the
conversion data), and the 3rd bit (D13) is the MSB of
the conversion data. In 12-bit conversion mode, the
first four bits can be ignored (they are the MSB of the
conversion data), and the 5th bit (D11) of the byte
represents the MSB of the conversion data. Table 5-3
shows an example of the conversion data output of
each conversion mode.
The configuration byte follows the output data byte.
The device outputs the configuration byte as long as
the SCL pulses are received. The device terminates
the current outputs when it receives a Not-Acknowledge
(NAK), a repeated start or a stop bit at any time
during the output bit stream. It is not required to read
the configuration byte. However, the user may read the
configuration byte to check the RDY bit condition to
confirm whether the just received data bytes are
updated conversion data. The user may continuously
send clock (SCL) to repeatedly read the configuration
bytes to check the RDY bit status.
Figures 5-2 and 5-3 show the timing diagrams of the
reading.
5.3.3 WRITING A CONFIGURATION BYTE
TO THE DEVICE
When the Master sends an address byte with the R/W
bit low (R/W = 0), the MCP3421 expects one
configuration byte following the address. Any byte sent
after this second byte will be ignored. The user can
change the operating mode of the device by writing the
configuration register bits.
If the device receives a write command with a new
configuration setting, the device immediately begins a
new conversion and updates the conversion data.
Start bit Read/Write bit
Address Byte
R/W ACK
1 1 0 1 X X X
Device Code Address Bits (Note 1)
Address
Acknowledge bit
Address
Note 1: Specified by customer and programmed at the
factory. If not specified by the customer,
programmed to ‘000’.
TABLE 5-3: EXAMPLE OF CONVERSION DATA OUTPUT OF EACH CONVERSION MODE
Conversion
Mode Conversion Data Output
18-bits MMMMMMMD16 (1st data byte) - D15 ~ D8 (2nd data byte) - D7 ~ D0 (3rd data byte) - Configuration
byte
16-bits MD14~D8 (1st data byte) - D7 ~ D0 (2nd data byte) - Configuration byte
14-bits MMMD12~D8 (1st data byte) - D7 ~ D0 (2nd data byte) - Configuration byte
12-bits MMMMMD10D9D8 (1st data byte) - D7 ~ D0 (2nd data byte) - Configuration byte
Note: M is MSB of the data byte.
MCP3421
DS22003B-page 14 © 2006 Microchip Technology Inc.
FIGURE 5-2: Timing Diagram For Reading From The MCP3421 With 18-Bit Mode.
1 9 1 9 1 9 1 9 1 9
1 9
1 1 0 1 A2 A1 A0 D
ACK by RDY O/C
MCP3421
7
Start Bit by R/W
Master
Repeat of D17 (MSB)
2nd Byte
Upper Data Byte
(Data on Clocks 1-6th
can be ignored)
ACK by
Master
ACK by
Master
ACK by
Master
ACK by
Master
17
D
16
D
15
D
14
D
13
D
12
D
11
D
10
D9
D8
D7
D6
D5
D4
D3
D2
D1
D0
C1
C0
S1
S0
G1
G0
1st Byte
MCP3421 Address Byte
3rd Byte
Middle Data Byte
4th Byte
Lower Data Byte
5th Byte
Configuration Byte
(Optional)
C1
C0
S1
S0
G1
G0
NAK by
Master
Stop Bit by
Master
(Optional)
Nth Repeated Byte:
Configuration Byte
Note: – MCP3421 device code is 1101.
– Address Bits A2- A0 = 000 are programmed at the factory unless customer requests specific codes.
– Stop bit or NAK bit can be issued any time during reading.
– Data bits on clocks 1 - 6th in 2nd byte are repeated MSB and can be ignored.
SCL
SDA
RDY O/C
© 2006 Microchip Technology Inc. DS22003B-page 15
MCP3421
FIGURE 5-3: Timing Diagram For Reading From The MCP3421 With 12-Bit to 16-Bit Modes.
1 1 0 1 A2 A1 A0
ACK by
MCP3421
Start Bit by
Master
2nd Byte
Middle Data Byte
ACK by
Master
ACK by
Master
ACK by
Master
D
15
D
14
D
13
D
12
D
11
D
10
D9
D8
D7
D6
D5
D4
D3
D2
D1
D0
C1
C0
S1
S0
G1
G0
1st Byte
MCP3421 Address Byte
3rd Byte
Lower Data Byte
4th Byte
Configuration Byte
(Optional)
C1
C0
S1
S0
G1
G0
NAK by
Master
Stop Bit by
Master
(Optional)
Nth Repeated Byte:
Configuration Byte
Note: – MCP3421 device code is 1101.
– Address Bits A2- A0 = 000 are programmed at the factory unless customer requests specific codes.
– Stop bit or NAK bit can be issued any time during reading.
– In 14 - bit mode: D15 and D14 are repeated MSB and can be ignored.
– In 12 - bit mode: D15 - D12 are repeated MSB and can be ignored.
1 9 1 9 1 9 1 9
SCL
SDA
1 9
R/W RDY O/C
RDY O/C
MCP3421
DS22003B-page 16 © 2006 Microchip Technology Inc.
FIGURE 5-4: Timing Diagram For Writing To The MCP3421.
5.4 General Call
The MCP3421 acknowledges the general call address
(0x00 in the first byte). The meaning of the general call
address is always specified in the second byte. Refer
to Figure 5-5. The MCP3421 supports the following
general calls:
5.4.1 GENERAL CALL RESET
The general call reset occurs if the second byte is
‘00000110’ (06h). At the acknowledgement of this
byte, the device will abort current conversion and
perform an internal reset similar to a power-on-reset
(POR).
5.4.2 GENERAL CALL CONVERSION
The general call conversion occurs if the second byte
is ‘00001000’ (08h). All devices on the bus initiate a
conversion simultaneously. For the MCP3421 device,
the configuration will be set to the One-Shot Conversion
mode and a single conversion will be performed.
The PGA and data rate settings are unchanged with
this general call.
FIGURE 5-5: General Call Address
Format.
For more information on the general call, or other I2C
modes, please refer to the Phillips I2C specification.
1 9 1 9
Stop Bit by
1 1 0 1 A2 A1 A0
R/W ACK by
MCP3421
RDY
C1 C0
O/C
S1 S0 G1 G0
1st Byte:
2nd Byte:
Master
ACK by
MCP3421
MCP3421 Address Byte
Configuration Byte
Start Bit by
Master
with Write command
Note: – Stop bit can be issued any time during writing.
– MCP3421 device code is 1101.
– Address Bits A2- A0 = 000 are programmed at factory unless customer requests different codes.
SCL
SDA
Note: The I2C specification does not allow to use
“00000000” (00h) in the second byte.
LSB
First Byte
ACK
0 0 0 0 0 0 0 0 A x x x x x x x x A
(General Call Address)
Second Byte
ACK
© 2006 Microchip Technology Inc. DS22003B-page 17
MCP3421
5.5 High-Speed (HS) Mode
The I2C specification requires that a high-speed mode
device must be ‘activated’ to operate in high-speed
mode. This is done by sending a special address byte
of 00001XXX following the START bit. The XXX bits are
unique to the High-Speed (HS) mode Master. This byte
is referred to as the High-Speed (HS) Master Mode
Code (HSMMC). The MCP3421 device does not
acknowledge this byte. However, upon receiving this
code, the MCP3421 switches on its HS mode filters
and communicates up to 3.4 MHz on SDA and SCL.
The device will switch out of the HS mode on the next
STOP condition.
For more information on the HS mode, or other I2C
modes, please refer to the Phillips I2C specification.
5.6 I2C Bus Characteristics
The I2C specification defines the following bus
protocol:
• Data transfer may be initiated only when the bus
is not busy.
• During data transfer, the data line must remain
stable whenever the clock line is HIGH. Changes
in the data line while the clock line is HIGH will be
interpreted as a START or STOP condition.
Accordingly, the following bus conditions have been
defined using Figure 5-6.
5.6.1 BUS NOT BUSY (A)
Both data and clock lines remain HIGH.
5.6.2 START DATA TRANSFER (B)
A HIGH to LOW transition of the SDA line while the
clock (SCL) is HIGH determines a START condition. All
commands must be preceded by a START condition.
5.6.3 STOP DATA TRANSFER (C)
A LOW to HIGH transition of the SDA line while the
clock (SCL) is HIGH determines a STOP condition. All
operations can be ended with a STOP condition.
5.6.4 DATA VALID (D)
The state of the data line represents valid data when,
after a START condition, the data line is stable for the
duration of the HIGH period of the clock signal.
The data on the line must be changed during the LOW
period of the clock signal. There is one clock pulse per
bit of data.
Each data transfer is initiated with a START condition
and terminated with a STOP condition.
5.6.5 ACKNOWLEDGE
The Master (microcontroller) and the slave (MCP3421)
use an acknowledge pulse as a hand shake of
communication for each byte. The ninth clock pulse of
each byte is used for the acknowledgement. The
acknowledgement is achieved by pulling-down the
SDA line “LOW” during the 9th clock pulse. The clock
pulse is always provided by the Master (microcontroller)
and the acknowledgement is issued by the
receiving device of the byte (Note: The transmitting
device must release the SDA line (“HIGH”) during the
acknowledge pulse.). For example, the slave
(MCP3421) issues the acknowledgement (bring down
the SDA line “LOW”) after the end of each receiving
byte, and the master (microcontroller) issues the
acknowledgement when it reads data from the Slave
(MCP3421).
When the MCP3421 is addressed, it generates an
acknowledge after receiving each byte successfully.
The Master device (microcontroller) must provide an
extra clock pulse (9th pulse of each byte) for the
acknowledgement from the MCP3421 (slave).
The MCP3421 (slave) pulls-down the SDA line during
the acknowledge clock pulse in such a way that the
SDA line is stable low during the high period of the
acknowledge clock pulse.
During reads, the Master (microcontroller) can
terminate the current read operation by not providing
an acknowledge bit on the last byte that has been
clocked out from the MCP3421. In this case, the
MCP3421 releases the SDA line to allow the master
(microcontroller) to generate a STOP or repeated
START condition.
FIGURE 5-6: Data Transfer Sequence on the Serial Bus.
SCL
SDA
(A) (B) (D) (D) (C) (A)
START
CONDITION
ADDRESS OR
ACKNOWLEDGE
VALID
DATA
ALLOWED
TO CHANGE
STOP
CONDITION
MCP3421
DS22003B-page 18 © 2006 Microchip Technology Inc.
TABLE 5-4: I2C SERIAL TIMING SPECIFICATIONS
Electrical Specifications: Unless otherwise specified, all limits are specified for TA = -40 to +85°C, VDD = +2.7V, +3.3V or +5.0V,
VSS = 0V, VIN+ = VIN- = VREF/2.
Parameters Sym Min Typ Max Units Conditions
Standard Mode
Clock frequency fSCL 0 — 100 kHz
Clock high time THIGH 4000 — — ns
Clock low time TLOW 4700 — — ns
SDA and SCL rise time (Note 1) TR — — 1000 ns From VIL to VIH
SDA and SCL fall time (Note 1) TF — — 300 ns From VIH to VIL
START condition hold time THD:STA 4000 — — ns After this period, the first clock
pulse is generated.
Repeated START condition
setup time
TSU:STA 4700 — — ns Only relevant for repeated Start
condition
Data hold time (Note 3) THD:DAT 0 — 3450 ns
Data input setup time TSU:DAT 250 — — ns
STOP condition setup time TSU:STO 4000 — — ns
STOP condition hold time THD:STD 4000 — — ns
Output valid from clock
(Notes 2 and 3)
TAA 0 — 3750 ns
Bus free time TBUF 4700 — — ns Time between START and STOP
conditions.
Fast Mode
Clock frequency TSCL 0 — 400 kHz
Clock high time THIGH 600 — — ns
Clock low time TLOW 1300 — — ns
SDA and SCL rise time (Note 1) TR 20 + 0.1Cb — 300 ns From VIL to VIH
SDA and SCL fall time (Note 1) TF 20 + 0.1Cb — 300 ns From VIH to VIL
START condition hold time THD:STA 600 — — ns After this period, the first clock
pulse is generated
Repeated START condition
setup time
TSU:STA 600 — — ns Only relevant for repeated Start
condition
Data hold time (Note 4) THD:DAT 0 — 900 ns
Data input setup time TSU:DAT 100 — — ns
STOP condition setup time TSU:STO 600 — — ns
STOP condition hold time THD:STD 600 — — ns
Output valid from clock
(Notes 2 and 3)
TAA 0 — 1200 ns
Bus free time TBUF 1300 — — ns Time between START and STOP
conditions.
Input filter spike suppression
(Note 5)
TSP 0 — 50 ns SDA and SCL pins
Note 1: This parameter is ensured by characterization and not 100% tested.
2: This specification is not a part of the I2C specification. This specification is equivalent to the Data Hold Time (THD:DAT)
plus SDA Fall (or rise) time: TAA = THD:DAT + TF (OR TR).
3: If this parameter is too short, it can create an unintended Start or Stop condition to other devices on the bus line. If this
parameter is too long, Clock Low time (TLOW) can be affected.
4: For Data Input: This parameter must be longer than tSP. If this parameter is too long, the Data Input Setup (TSU:DAT) or
Clock Low time (TLOW) can be affected.
For Data Output: This parameter is characterized, and tested indirectly by testing TAA parameter.
5: This parameter is ensured by characterization and not 100% tested. This parameter is not available for Standard Mode.
© 2006 Microchip Technology Inc. DS22003B-page 19
MCP3421
High Speed Mode
Clock frequency fSCL 0 — 3.4
1.7
MHz
MHz
Cb = 100 pF
Cb = 400 pF
Clock high time THIGH 60
120
— — ns
ns
Cb = 100 pF
Cb = 400 pF
Clock low time TLOW 160
320
— — ns Cb = 100 pF
Cb = 400 pF
SCL rise time (Note 1) TR — — 40
80
ns From VIL to VIH,Cb = 100 pF
Cb = 400 pF
SCL fall time (Note 1) TF — — 40
80
ns From VIH to VIL,Cb = 100 pF
Cb = 400 pF
SDA rise time (Note 1) TR: DAT — — 80
160
ns From VIL to VIH,Cb = 100 pF
Cb = 400 pF
SDA fall time (Note 1) TF: DATA — — 80
160
ns From VIH to VIL,Cb = 100 pF
Cb = 400 pF
START condition hold time THD:STA 160 — — ns After this period, the first clock
pulse is generated
Repeated START condition
setup time
TSU:STA 160 — — ns Only relevant for repeated Start
condition
Data hold time (Note 4) THD:DAT 00
— 70
150
ns Cb = 100 pF
Cb = 400 pF
Data input setup time TSU:DAT 10 — — ns
STOP condition setup time TSU:STO 160 — — ns
STOP condition hold time THD:STD 160 — — ns
Output valid from clock
(Notes 2 and 3)
TAA — — 150
310
ns Cb = 100 pF
Cb = 400 pF
Bus free time TBUF 160 — — ns Time between START and STOP
conditions.
Input filter spike suppression
(Note 5)
TSP 0 — 10 ns SDA and SCL pins
TABLE 5-4: I2C SERIAL TIMING SPECIFICATIONS (CONTINUED)
Electrical Specifications: Unless otherwise specified, all limits are specified for TA = -40 to +85°C, VDD = +2.7V, +3.3V or +5.0V,
VSS = 0V, VIN+ = VIN- = VREF/2.
Parameters Sym Min Typ Max Units Conditions
Note 1: This parameter is ensured by characterization and not 100% tested.
2: This specification is not a part of the I2C specification. This specification is equivalent to the Data Hold Time (THD:DAT)
plus SDA Fall (or rise) time: TAA = THD:DAT + TF (OR TR).
3: If this parameter is too short, it can create an unintended Start or Stop condition to other devices on the bus line. If this
parameter is too long, Clock Low time (TLOW) can be affected.
4: For Data Input: This parameter must be longer than tSP. If this parameter is too long, the Data Input Setup (TSU:DAT) or
Clock Low time (TLOW) can be affected.
For Data Output: This parameter is characterized, and tested indirectly by testing TAA parameter.
5: This parameter is ensured by characterization and not 100% tested. This parameter is not available for Standard Mode.
MCP3421
DS22003B-page 20 © 2006 Microchip Technology Inc.
FIGURE 5-7: I2C Bus Timing Data.
TF
SCL
SDA
TSU:STA
TSP
THD:STA
TLOW
THIGH
THD:DAT
TAA
TSU:DAT
TR
TSU:STO
TBUF
© 2006 Microchip Technology Inc. DS22003B-page 21
MCP3421
6.0 BASIC APPLICATION
CONFIGURATION
The MCP3421 device can be used for various precision
analog-to-digital converter applications. The device
operates with very simple connections to the
application circuit. The following sections discuss the
examples of the device connections and applications.
6.1 Connecting to the Application
Circuits
6.1.1 INPUT VOLTAGE RANGE
The fully differential input signals can be connected to
the VIN+ and VIN- input pins. The input range should be
within absolute common mode input voltage range:
VSS - 0.3V to VDD + 0.3V. Outside this limit, the ESD
protection diode at the input pin begins to conduct and
the error due to input leakage current increases rapidly.
Within this limit, the differential input VIN (= VIN+ - VIN-)
is boosted by the PGA before a conversion takes place.
The MCP3421 can not accept negative input voltages
on the input pins. Figures 6-1 and 6-2 show typical connection
examples for differential inputs and a singleended
input, respectively. For the single-ended input,
the input signal is applied to one of the input pins
(typically connected to the VIN+ pin) while the other
input pin (typically VIN- pin) is grounded. The input
signal range of the single-ended configuration is from
0V to 2.048V. All device characteristics hold for the
single-ended configuration, but this configuration loses
one bit resolution because the input can only stand in
positive half scale. Refer to Section 1.0 “Electrical
Characteristics”.
6.1.2 BYPASS CAPACITORS ON VDD PIN
For accurate measurement, the application circuit
needs a clean supply voltage and must block any noise
signal to the MCP3421 device. Figure 6-1 shows an
example of using two bypass capacitors (a 10 μF
tantalum capacitor and a 0.1 μF ceramic capacitor) in
parallel on the VDD line. These capacitors are helpful to
filter out any high frequency noises on the VDD line and
also provide the momentary bursts of extra currents
when the device needs from the supply. These
capacitors should be placed as close to the VDD pin as
possible (within one inch). If the application circuit has
separate digital and analog power supplies, the VDD
and VSS of the MCP3421 should reside on the analog
plane.
6.1.3 CONNECTING TO I2C BUS USING
PULL-UP RESISTORS
The SCL and SDA pins of the MCP3421 are open-drain
configurations. These pins require a pull-up resistor as
shown in Figure 6-1. The value of these pull-up resistors
depends on the operating speed (standard, fast,
and high speed) and loading capacitance of the I2C bus
line. Higher value of pull-up resistor consumes less
power, but increases the signal transition time (higher
RC time constant) on the bus. Therefore, it can limit the
bus operating speed. The lower value of resistor, on the
other hand, consumes higher power, but allows higher
operating speed. If the bus line has higher capacitance
due to long bus line or high number of devices
connected to the bus, a smaller pull-up resistor is
needed to compensate the long RC time constant. The
pull-up resistor is typically chosen between 1 kΩ and
10 kΩ ranges for standard and fast modes, and less
than 1 kΩ for high speed mode in high loading
capacitance environments.
FIGURE 6-1: Typical Connection Example
for Differential Inputs.
FIGURE 6-2: Typical Connection Example
for Single-Ended Input.
The number of devices connected to the bus is limited
only by the maximum bus capacitance of 400 pF. The
bus loading capacitance affects on the bus operating
speed. For example, the highest bus operating speed
for the 400 pF bus capacitance is 1.7 MHz, and
3.4 MHz for 100 pF. Figure 6-3 shows an example of
multiple device connections.
MCP3421
VIN+ VINVSS
VDD
1
2
3 4
5
6
SCL SDL 0.1 μF 10 μF
R R
Input Signals VDD VDD
TO MCU
Note: R is the pull-up resistor. (MASTER)
MCP3421
VIN+ VINVSS
VDD
1
2
3 4
5
6
SCL SDL 0.1 μF 10 μF
R R
Input Signals
VDD VDD
TO MCU
Note: R is the pull-up resistor. (MASTER)
MCP3421
DS22003B-page 22 © 2006 Microchip Technology Inc.
FIGURE 6-3: Example of Multiple Device
Connection on I2C Bus.
6.2 Device Connection Test
The user can test the presence of the MCP3421 on the
I2C bus line without performing an input data conversion.
This test can be achieved by checking an
acknowledge response from the MCP3421 after sending
a read or write command. Here is an example using
Figure 6-4:
(a) Set the R/W bit “HIGH” in the address byte.
(b) The MCP3421 will then acknowledge by pulling
SDA bus LOW during the ACK clock and then release
the bus back to the I2C Master.
(c) A STOP or repeated START bit can then be issued
from the Master and I2C communication can continue.
FIGURE 6-4: I2C Bus Connection Test.
6.3 Application Examples
The MCP3421 device can be used in a broad range of
sensor and data acquisition applications. Figure 6-5,
shows an example of interfacing with a bridge sensor
for pressure measurement.
FIGURE 6-5: Example of Pressure
Measurement.
In this circuit example, the sensor full scale range is
±7.5 mV with a common mode input voltage of VDD / 2.
This configuration will provide a full 14-bit resolution
across the sensor output range. The alternative circuit
for this amount of accuracy would involve an analog
gain stage prior to a 16-bit ADC.
Figure 6-6 shows an example of temperature measurement
using a thermistor. This example can achieve a
linear response over a 50°C temperature range. This
can be implemented using a standard resistor with 1%
tolerance in series with the thermistor. The value of the
resistor is selected to be equal to the thermistor value
at the mid-point of the desired temperature range.
FIGURE 6-6: Example of Temperature
Measurement.
SDASCL
(24LC01)
Microcontroller
EEPROM
MCP3421
(TC74)
Temperature
Sensor
(PIC16F876)
SCL 1 2 3 4 5 6 7 8 9
SDA 1 1 0 1 A2 A1 A0 1
Start
Bit
Address Byte
Device bits Address bits
R/W
Start
Bit
MCP3421
ACK
Response
NPP301
MCP3421
VIN+ VINVSS
VDD
1
2
3 4
5
6
SCL SDL 0.1 μF 10 μF
R R
VDD VDD
TO MCU
(MASTER)
VDD
10 kΩ
Resistor
10 kΩ
Thermistor
MCP3421
VIN+ VINVSS
VDD
1
2
3 4
5
6
SCL SDL 0.1 μF 10 μF
R R
VDD VDD
TO MCU
(MASTER)
VDD
© 2006 Microchip Technology Inc. DS22003B-page 23
MCP3421
7.0 PACKAGING INFORMATION
7.1 Package Marking Information
Legend: XX...X Customer-specific information
Y Year code (last digit of calendar year)
YY Year code (last 2 digits of calendar year)
WW Week code (week of January 1 is week ‘01’)
NNN Alphanumeric traceability code
Pb-free JEDEC designator for Matte Tin (Sn)
* This package is Pb-free. The Pb-free JEDEC designator ( )
can be found on the outer packaging for this package.
Note: In the event the full Microchip part number cannot be marked on one line, it will
be carried over to the next line, thus limiting the number of available
characters for customer-specific information.
e3
e3
2
5
3 1
4 6
6-Lead SOT-23
XXNN
2
5
3 1
4 6
Example
CA25
MCP3421
DS22003B-page 24 © 2006 Microchip Technology Inc.
6-Lead Plastic Small Outline Transistor (OT) (SOT-23)
Note: For the most current package drawings, please see the Microchip Packaging Specification located at
http://www.microchip.com/packaging
1
D
B
n
E
E1
L
c
β
φ
α
A A2
A1
p1
Mold Draft Angle Bottom β 0 5 10 0 5 10
Mold Draft Angle Top α 0 5 10 0 5 10
Lead Width B .014 .017 .020 0.35 0.43 0.50
Lead Thickness c .004 .006 .008 0.09 0.15 0.20
Foot Angle φ 0 5 10 0 5 10
Foot Length L .014 .018 .022 0.35 0.45 0.55
Overall Length D .110 .116 .122 2.80 2.95 3.10
Molded Package Width E1 .059 .064 .069 1.50 1.63 1.75
Overall Width E .102 .110 .118 2.60 2.80 3.00
Standoff A1 .000 .003 .006 0.00 0.08 0.15
Molded Package Thickness A2 .035 .043 .051 0.90 1.10 1.30
Overall Height A .035 .046 .057 0.90 1.18 1.45
Outside lead pitch p1 .075 BSC 1.90 BSC
Pitch p .038 BSC 0.95 BSC
Number of Pins n 6 6
Dimension Limits MIN NOM MAX MIN NOM MAX
Units INCHES* MILLIMETERS
Dimensions D and E1 do not include mold flash or protrusions. Mold flash or protrusions shall not exceed .005" (0.127mm) per side.
Notes:
JEITA (formerly EIAJ) equivalent: SC-74A
* Controlling Parameter
Drawing No. C04-120
BSC: Basic Dimension. Theoretically exact value shown without tolerances.
See ASME Y14.5M
Revised 09-12-05
© 2006 Microchip Technology Inc. DS22003B-page 27
MCP3421
APPENDIX A: REVISION HISTORY
Revision B (December 2006)
• Changes to Electrical Characteristics tables
• Added characterization data
• Changes to I2C Serial Timing Specification table
• Change to Figure 5-7.
Revision A (August 2006)
• Original Release of this Document.
MCP3421
DS22003B-page 28 © 2006 Microchip Technology Inc.
NOTES:
© 2006 Microchip Technology Inc. DS22003B-page 29
MCP3421
PRODUCT IDENTIFICATION SYSTEM
To order or obtain information, e.g., on pricing or delivery, refer to the factory or the listed sales office.
Device: MCP3421T: Single Channel ΔΣ A/D Converter
(Tape and Reel)
Address Options: XX A2 A1 A0
A0 * = 0 0 0
A1 = 0 0 1
A2 = 0 1 0
A3 = 0 1 1
A4 = 1 0 0
A5 = 1 0 1
A6 = 1 1 0
A7 = 1 1 1
* Default option. Contact Microchip factory for other
address options
Temperature Range: E = -40°C to +125°C
Package: OT = Plastic Small Outline Transistor (SOT-23-6),
6-lead
Examples:
a) MCP3421A0T-E/OT: Tape and Reel,
Single Channel ΔΣ A/D
Converter, SOT-23-6
package.
PART NO. XX X
Address Temperature
Range
Device
/XX
Package
Options
MCP3421
DS22003B-page 30 © 2006 Microchip Technology Inc.
NOTES:
© 2006 Microchip Technology Inc. DS22003B-page 31
Information contained in this publication regarding device
applications and the like is provided only for your convenience
and may be superseded by updates. It is your responsibility to
ensure that your application meets with your specifications.
MICROCHIP MAKES NO REPRESENTATIONS OR
WARRANTIES OF ANY KIND WHETHER EXPRESS OR
IMPLIED, WRITTEN OR ORAL, STATUTORY OR
OTHERWISE, RELATED TO THE INFORMATION,
INCLUDING BUT NOT LIMITED TO ITS CONDITION,
QUALITY, PERFORMANCE, MERCHANTABILITY OR
FITNESS FOR PURPOSE. Microchip disclaims all liability
arising from this information and its use. Use of Microchip
devices in life support and/or safety applications is entirely at
the buyer’s risk, and the buyer agrees to defend, indemnify and
hold harmless Microchip from any and all damages, claims,
suits, or expenses resulting from such use. No licenses are
conveyed, implicitly or otherwise, under any Microchip
intellectual property rights.
Trademarks
The Microchip name and logo, the Microchip logo, Accuron,
dsPIC, KEELOQ, microID, MPLAB, PIC, PICmicro, PICSTART,
PRO MATE, PowerSmart, rfPIC, and SmartShunt are
registered trademarks of Microchip Technology Incorporated
in the U.S.A. and other countries.
AmpLab, FilterLab, Migratable Memory, MXDEV, MXLAB,
SEEVAL, SmartSensor and The Embedded Control Solutions
Company are registered trademarks of Microchip Technology
Incorporated in the U.S.A.
Analog-for-the-Digital Age, Application Maestro, CodeGuard,
dsPICDEM, dsPICDEM.net, dsPICworks, ECAN,
ECONOMONITOR, FanSense, FlexROM, fuzzyLAB,
In-Circuit Serial Programming, ICSP, ICEPIC, Linear Active
Thermistor, Mindi, MiWi, MPASM, MPLIB, MPLINK, PICkit,
PICDEM, PICDEM.net, PICLAB, PICtail, PowerCal,
PowerInfo, PowerMate, PowerTool, REAL ICE, rfLAB,
rfPICDEM, Select Mode, Smart Serial, SmartTel, Total
Endurance, UNI/O, WiperLock and ZENA are trademarks of
Microchip Technology Incorporated in the U.S.A. and other
countries.
SQTP is a service mark of Microchip Technology Incorporated
in the U.S.A.
All other trademarks mentioned herein are property of their
respective companies.
© 2006, Microchip Technology Incorporated, Printed in the
U.S.A., All Rights Reserved.
Printed on recycled paper.
Note the following details of the code protection feature on Microchip devices:
• Microchip products meet the specification contained in their particular Microchip Data Sheet.
• Microchip believes that its family of products is one of the most secure families of its kind on the market today, when used in the
intended manner and under normal conditions.
• There are dishonest and possibly illegal methods used to breach the code protection feature. All of these methods, to our
knowledge, require using the Microchip products in a manner outside the operating specifications contained in Microchip’s Data
Sheets. Most likely, the person doing so is engaged in theft of intellectual property.
• Microchip is willing to work with the customer who is concerned about the integrity of their code.
• Neither Microchip nor any other semiconductor manufacturer can guarantee the security of their code. Code protection does not
mean that we are guaranteeing the product as “unbreakable.”
Code protection is constantly evolving. We at Microchip are committed to continuously improving the code protection features of our
products. Attempts to break Microchip’s code protection feature may be a violation of the Digital Millennium Copyright Act. If such acts
allow unauthorized access to your software or other copyrighted work, you may have a right to sue for relief under that Act.
Microchip received ISO/TS-16949:2002 certification for its worldwide
headquarters, design and wafer fabrication facilities in Chandler and
Tempe, Arizona, Gresham, Oregon and Mountain View, California. The
Company’s quality system processes and procedures are for its PIC®
8-bit MCUs, KEELOQ® code hopping devices, Serial EEPROMs,
microperipherals, nonvolatile memory and analog products. In addition,
Microchip’s quality system for the design and manufacture of
development systems is ISO 9001:2000 certified.
DS22003B-page 32 © 2006 Microchip Technology Inc.
AMERICAS
Corporate Office
2355 West Chandler Blvd.
Chandler, AZ 85224-6199
Tel: 480-792-7200
Fax: 480-792-7277
Technical Support:
http://support.microchip.com
Web Address:
www.microchip.com
Atlanta
Alpharetta, GA
Tel: 770-640-0034
Fax: 770-640-0307
Boston
Westborough, MA
Tel: 774-760-0087
Fax: 774-760-0088
Chicago
Itasca, IL
Tel: 630-285-0071
Fax: 630-285-0075
Dallas
Addison, TX
Tel: 972-818-7423
Fax: 972-818-2924
Detroit
Farmington Hills, MI
Tel: 248-538-2250
Fax: 248-538-2260
Kokomo
Kokomo, IN
Tel: 765-864-8360
Fax: 765-864-8387
Los Angeles
Mission Viejo, CA
Tel: 949-462-9523
Fax: 949-462-9608
Santa Clara
Santa Clara, CA
Tel: 408-961-6444
Fax: 408-961-6445
Toronto
Mississauga, Ontario,
Canada
Tel: 905-673-0699
Fax: 905-673-6509
ASIA/PACIFIC
Asia Pacific Office
Suites 3707-14, 37th Floor
Tower 6, The Gateway
Habour City, Kowloon
Hong Kong
Tel: 852-2401-1200
Fax: 852-2401-3431
Australia - Sydney
Tel: 61-2-9868-6733
Fax: 61-2-9868-6755
China - Beijing
Tel: 86-10-8528-2100
Fax: 86-10-8528-2104
China - Chengdu
Tel: 86-28-8665-5511
Fax: 86-28-8665-7889
China - Fuzhou
Tel: 86-591-8750-3506
Fax: 86-591-8750-3521
China - Hong Kong SAR
Tel: 852-2401-1200
Fax: 852-2401-3431
China - Qingdao
Tel: 86-532-8502-7355
Fax: 86-532-8502-7205
China - Shanghai
Tel: 86-21-5407-5533
Fax: 86-21-5407-5066
China - Shenyang
Tel: 86-24-2334-2829
Fax: 86-24-2334-2393
China - Shenzhen
Tel: 86-755-8203-2660
Fax: 86-755-8203-1760
China - Shunde
Tel: 86-757-2839-5507
Fax: 86-757-2839-5571
China - Wuhan
Tel: 86-27-5980-5300
Fax: 86-27-5980-5118
China - Xian
Tel: 86-29-8833-7250
Fax: 86-29-8833-7256
ASIA/PACIFIC
India - Bangalore
Tel: 91-80-4182-8400
Fax: 91-80-4182-8422
India - New Delhi
Tel: 91-11-4160-8631
Fax: 91-11-4160-8632
India - Pune
Tel: 91-20-2566-1512
Fax: 91-20-2566-1513
Japan - Yokohama
Tel: 81-45-471- 6166
Fax: 81-45-471-6122
Korea - Gumi
Tel: 82-54-473-4301
Fax: 82-54-473-4302
Korea - Seoul
Tel: 82-2-554-7200
Fax: 82-2-558-5932 or
82-2-558-5934
Malaysia - Penang
Tel: 60-4-646-8870
Fax: 60-4-646-5086
Philippines - Manila
Tel: 63-2-634-9065
Fax: 63-2-634-9069
Singapore
Tel: 65-6334-8870
Fax: 65-6334-8850
Taiwan - Hsin Chu
Tel: 886-3-572-9526
Fax: 886-3-572-6459
Taiwan - Kaohsiung
Tel: 886-7-536-4818
Fax: 886-7-536-4803
Taiwan - Taipei
Tel: 886-2-2500-6610
Fax: 886-2-2508-0102
Thailand - Bangkok
Tel: 66-2-694-1351
Fax: 66-2-694-1350
EUROPE
Austria - Wels
Tel: 43-7242-2244-39
Fax: 43-7242-2244-393
Denmark - Copenhagen
Tel: 45-4450-2828
Fax: 45-4485-2829
France - Paris
Tel: 33-1-69-53-63-20
Fax: 33-1-69-30-90-79
Germany - Munich
Tel: 49-89-627-144-0
Fax: 49-89-627-144-44
Italy - Milan
Tel: 39-0331-742611
Fax: 39-0331-466781
Netherlands - Drunen
Tel: 31-416-690399
Fax: 31-416-690340
Spain - Madrid
Tel: 34-91-708-08-90
Fax: 34-91-708-08-91
UK - Wokingham
Tel: 44-118-921-5869
Fax: 44-118-921-5820
WORLDWIDE SALES AND SERVICE
10/19/06
Features
• High-performance, Low-power AVR® 8-bit Microcontroller
• RISC Architecture
– 118 Powerful Instructions – Most Single Clock Cycle Execution
– 32 x 8 General Purpose Working Registers
– Fully Static Operation
– Up to 16 MIPS Throughput at 16 MHz
• Data and Non-volatile Program Memory
– 2K Bytes of In-System Programmable Program Memory Flash
Endurance: 10,000 Write/Erase Cycles
– 128 Bytes of In-System Programmable EEPROM
Endurance: 100,000 Write/Erase Cycles
– 128 Bytes Internal SRAM
– Programming Lock for Flash Program and EEPROM Data Security
• Peripheral Features
– 8-bit Timer/Counter with Separate Prescaler
– 8-bit High-speed Timer with Separate Prescaler
2 High Frequency PWM Outputs with Separate Output Compare Registers
Non-overlapping Inverted PWM Output Pins
– Universal Serial Interface with Start Condition Detector
– 10-bit ADC
11 Single Ended Channels
8 Differential ADC Channels
7 Differential ADC Channel Pairs with Programmable Gain (1x, 20x)
– On-chip Analog Comparator
– External Interrupt
– Pin Change Interrupt on 11 Pins
– Programmable Watchdog Timer with Separate On-chip Oscillator
• Special Microcontroller Features
– Low Power Idle, Noise Reduction, and Power-down Modes
– Power-on Reset and Programmable Brown-out Detection
– External and Internal Interrupt Sources
– In-System Programmable via SPI Port
– Internal Calibrated RC Oscillator
• I/O and Packages
– 20-lead PDIP/SOIC: 16 Programmable I/O Lines
– 32-lead QFN/MLF: 16 programmable I/O Lines
• Operating Voltages
– 2.7V - 5.5V for ATtiny26L
– 4.5V - 5.5V for ATtiny26
• Speed Grades
– 0 - 8 MHz for ATtiny26L
– 0 - 16 MHz for ATtiny26
• Power Consumption at 1 MHz, 3V and 25°C for ATtiny26L
– Active 16 MHz, 5V and 25°C: Typ 15 mA
– Active 1 MHz, 3V and 25°C: 0.70 mA
– Idle Mode 1 MHz, 3V and 25°C: 0.18 mA
– Power-down Mode: < 1 μA
8-bit
Microcontroller
with 2K Bytes
Flash
ATtiny26
ATtiny26L
Summary
1477KS–AVR–08/10
2
1477KS–AVR–08/10
ATtiny26(L)
Pin
Configuration
Note: The bottom pad under the QFN/MLF package should be soldered to ground.
1
2
3
4
5
6
7
8
9
10
20
19
18
17
16
15
14
13
12
11
(MOSI/DI/SDA/OC1A) PB0
(MISO/DO/OC1A) PB1
(SCK/SCL/OC1B) PB2
(OC1B) PB3
VCC
GND
(ADC7/XTAL1) PB4
(ADC8/XTAL2) PB5
(ADC9/INT0/T0) PB6
(ADC10/RESET) PB7
PA0 (ADC0)
PA1 (ADC1)
PA2 (ADC2)
PA3 (AREF)
GND
AVCC
PA4 (ADC3)
PA5 (ADC4)
PA6 (ADC5/AIN0)
PA7 (ADC6/AIN1)
PDIP/SOIC
1
2
3
4
5
6
7
8
24
23
22
21
20
19
18
17
32
31
30
29
28
27
26
25
9
10
11
12
13
14
15
16
MLF Top View
NC
(OC1B) PB3
NC
VCC
GND
NC
(ADC7/XTAL1) PB4
(ADC8/XTAL2) PB5
NC
PA2 (ADC2)
PA3 (AREF)
GND
NC
NC
AVCC
PA4 (ADC3)
NC
(ADC9/INT0/T0) PB6
(ADC10/RESET) PB7
NC
(ADC6/AIN1) PA7
(ADC5/AIN0) PA6
(ADC4) PA5
NC
PB2 (SCK/SCL/OC1B)
PB1 (MISO/DO/OC1A)
PB0 (MOSI/DI/SDA/OC1A)
NC
NC
NC
PA0 (ADC0)
PA1 (ADC1)
3
1477KS–AVR–08/10
ATtiny26(L)
Description The ATtiny26(L) is a low-power CMOS 8-bit microcontroller based on the AVR enhanced RISC
architecture. By executing powerful instructions in a single clock cycle, the ATtiny26(L) achieves
throughputs approaching 1 MIPS per MHz allowing the system designer to optimize power consumption
versus processing speed.
The AVR core combines a rich instruction set with 32 general purpose working registers. All the
32 registers are directly connected to the Arithmetic Logic Unit (ALU), allowing two independent
registers to be accessed in one single instruction executed in one clock cycle. The resulting
architecture is more code efficient while achieving throughputs up to ten times faster than conventional
CISC microcontrollers. The ATtiny26(L) has a high precision ADC with up to 11 single
ended channels and 8 differential channels. Seven differential channels have an optional gain of
20x. Four out of the seven differential channels, which have the optional gain, can be used at the
same time. The ATtiny26(L) also has a high frequency 8-bit PWM module with two independent
outputs. Two of the PWM outputs have inverted non-overlapping output pins ideal for synchronous
rectification. The Universal Serial Interface of the ATtiny26(L) allows efficient software
implementation of TWI (Two-wire Serial Interface) or SM-bus interface. These features allow for
highly integrated battery charger and lighting ballast applications, low-end thermostats, and
firedetectors, among other applications.
The ATtiny26(L) provides 2K bytes of Flash, 128 bytes EEPROM, 128 bytes SRAM, up to 16
general purpose I/O lines, 32 general purpose working registers, two 8-bit Timer/Counters, one
with PWM outputs, internal and external Oscillators, internal and external interrupts, programmable
Watchdog Timer, 11-channel, 10-bit Analog to Digital Converter with two differential
voltage input gain stages, and four software selectable power saving modes. The Idle mode
stops the CPU while allowing the Timer/Counters and interrupt system to continue functioning.
The ATtiny26(L) also has a dedicated ADC Noise Reduction mode for reducing the noise in ADC
conversion. In this sleep mode, only the ADC is functioning. The Power-down mode saves the
register contents but freezes the oscillators, disabling all other chip functions until the next interrupt
or hardware reset. The Standby mode is the same as the Power-down mode, but external
oscillators are enabled. The wakeup or interrupt on pin change features enable the ATtiny26(L)
to be highly responsive to external events, still featuring the lowest power consumption while in
the Power-down mode.
The device is manufactured using Atmel’s high density non-volatile memory technology. By
combining an enhanced RISC 8-bit CPU with Flash on a monolithic chip, the ATtiny26(L) is a
powerful microcontroller that provides a highly flexible and cost effective solution to many
embedded control applications.
The ATtiny26(L) AVR is supported with a full suite of program and system development tools
including: Macro assemblers, program debugger/simulators, In-circuit emulators, and evaluation
kits.
4
1477KS–AVR–08/10
ATtiny26(L)
Block Diagram Figure 1. The ATtiny26(L) Block Diagram
WATCHDOG
TIMER
MCU CONTROL
REGISTER
UNIVERSAL
SERIAL
INTERFACE
TIMER/
COUNTER0
DATA DIR.
REG.PORT A
DATA REGISTER
PORT A
PROGRAMMING
LOGIC
TIMING AND
CONTROL
TIMER/
COUNTER1
MCU STATUS
REGISTER
PORT A DRIVERS
PA0-PA7
VCC
GND
+
-
ANALOG
COMPARATOR
8-BIT DATA BUS
ADC
ISP INTERFACE
INTERRUPT
UNIT
EEPROM
INTERNAL
OSCILLATOR
OSCILLATORS
CALIBRATED
OSCILLATOR
INTERNAL
DATA DIR.
REG.PORT B
DATA REGISTER
PORT B
PORT B DRIVERS
PB0-PB7
PROGRAM
COUNTER
STACK
POINTER
PROGRAM
FLASH SRAM
GENERAL
PURPOSE
REGISTERS
INSTRUCTION
REGISTER
INSTRUCTION
DECODER
STATUS
REGISTER
Z
Y
X
ALU
CONTROL
LINES
AVCC
5
1477KS–AVR–08/10
ATtiny26(L)
Pin Descriptions
VCC Digital supply voltage pin.
GND Digital ground pin.
AVCC AVCC is the supply voltage pin for Port A and the A/D Converter (ADC). It should be externally
connected to VCC, even if the ADC is not used. If the ADC is used, it should be connected to VCC
through a low-pass filter. See page 94 for details on operating of the ADC.
Port A (PA7..PA0) Port A is an 8-bit general purpose I/O port. PA7..PA0 are all I/O pins that can provide internal
pull-ups (selected for each bit). Port A has alternate functions as analog inputs for the ADC and
analog comparator and pin change interrupt as described in “Alternate Port Functions” on page
46.
Port B (PB7..PB0) Port B is an 8-bit general purpose I/O port. PB6..0 are all I/O pins that can provide internal pullups
(selected for each bit). PB7 is an I/O pin if not used as the reset. To use pin PB7 as an I/O
pin, instead of RESET pin, program (“0”) RSTDISBL Fuse. Port B has alternate functions for the
ADC, clocking, timer counters, USI, SPI programming, and pin change interrupt as described in
“Alternate Port Functions” on page 46.
An External Reset is generated by a low level on the PB7/RESET pin. Reset pulses longer than
50 ns will generate a reset, even if the clock is not running. Shorter pulses are not guaranteed to
generate a reset.
XTAL1 Input to the inverting oscillator amplifier and input to the internal clock operating circuit.
XTAL2 Output from the inverting oscillator amplifier.
6
1477KS–AVR–08/10
ATtiny26(L)
General
Information
Resources A comprehensive set of development tools, application notes and datasheets are available for
download on http://www.atmel.com/avr.
Code Examples This datasheet contains simple code examples that briefly show how to use various parts of the
device. These code examples assume that the part specific header file is included before compilation.
Be aware that not all C compiler vendors include bit definitions in the header files and
interrupt handling in C is compiler dependent. Please confirm with the C compiler documentation
for more details.
7
1477KS–AVR–08/10
ATtiny26(L)
Register Summary
Address Name Bit 7 Bit 6 Bit 5 Bit 4 Bit 3 Bit 2 Bit 1 Bit 0 Page
$3F ($5F) SREG I T H S V N Z C 10
$3E ($5E) Reserved
$3D ($5D) SP SP7 SP6 SP5 SP4 SP3 SP2 SP1 SP0 11
$3C ($5C) Reserved
$3B ($5B) GIMSK - INT0 PCIE1 PCIE0 - - - - 58
$3A ($5A) GIFR - INTF0 PCIF - - - - - 59
$39 ($59) TIMSK - OCIE1A OCIE1B - - TOIE1 TOIE0 - 59
$38 ($58) TIFR - OCF1A OCF1B - - TOV1 TOV0 - 60
$37 ($57) Reserved
$36 ($56) Reserved
$35 ($55) MCUCR - PUD SE SM1 SM0 - ISC01 ISC00 37
$34 ($54) MCUSR - - - - WDRF BORF EXTRF PORF 36
$33 ($53) TCCR0 - - - - PSR0 CS02 CS01 CS00 66
$32 ($52) TCNT0 Timer/Counter0 (8-Bit) 67
$31 ($51) OSCCAL Oscillator Calibration Register 29
$30 ($50) TCCR1A COM1A1 COM1A0 COM1B1 COM1B0 FOC1A FOC1B PWM1A PWM1B 70
$2F ($4F) TCCR1B CTC1 PSR1 - - CS13 CS12 CS11 CS10 71
$2E ($4E) TCNT1 Timer/Counter1 (8-Bit) 72
$2D ($4D) OCR1A Timer/Counter1 Output Compare Register A (8-Bit) 72
$2C ($4C) OCR1B Timer/Counter1 Output Compare Register B (8-Bit) 73
$2B ($4B) OCR1C Timer/Counter1 Output Compare Register C (8-Bit) 73
$2A ($4A) Reserved
$29 ($49) PLLCSR - - - - - PCKE PLLE PLOCK
$28 ($48) Reserved
$27 ($47) Reserved
$26 ($46) Reserved
$25 ($45) Reserved
$24 ($44) Reserved
$23 ($43) Reserved
$22 ($42) Reserved
$21 ($41) WDTCR - - - WDCE WDE WDP2 WDP1 WDP0 78
$20 ($40) Reserved
$1F ($3F) Reserved
$1E ($3E) EEAR - EEAR6 EEAR5 EEAR4 EEAR3 EEAR2 EEAR1 EEAR0 18
$1D ($3D) EEDR EEPROM Data Register (8-Bit) 19
$1C ($3C) EECR - - - - EERIE EEMWE EEWE EERE 19
$1B ($3B) PORTA PORTA7 PORTA6 PORTA5 PORTA4 PORTA3 PORTA2 PORTA1 PORTA0
$1A ($3A) DDRA DDA7 DDA6 DDA5 DDA4 DDA3 DDA2 DDA1 DDA0
$19 ($39) PINA PINA7 PINA6 PINA5 PINA4 PINA3 PINA2 PINA1 PINA0
$18 ($38) PORTB PORTB7 PORTB6 PORTB5 PORTB4 PORTB3 PORTB2 PORTB1 PORTB0
$17 ($37) DDRB DDB7 DDB6 DDB5 DDB4 DDB3 DDB2 DDB1 DDB0
$16 ($36) PINB PINB7 PINB6 PINB5 PINB4 PINB3 PINB2 PINB1 PINB0
$15 ($35) Reserved
$14 ($34) Reserved
$13 ($33) Reserved
$12 ($32) Reserved
$11 ($31) Reserved
$10 ($30) Reserved
$0F ($2F) USIDR Universal Serial Interface Data Register (8-Bit) 81
$0E ($2E) USISR USISIF USIOIF USIPF USIDC USICNT3 USICNT2 USICNT1 USICNT0 81
$0D ($2D) USICR USISIE USIOIE USIWM1 USIWM0 USICS1 USICS0 USICLK USITC 82
$0C ($2C) Reserved
$0B ($2)B Reserved
$0A ($2A) Reserved
$09 ($29) Reserved
$08 ($28) ACSR ACD ACBG ACO ACI ACIE ACME ACIS1 ACIS0 91
$07 ($27) ADMUX REFS1 REFS0 ADLAR MUX4 MUX3 MUX2 MUX1 MUX0 101
$06 ($26) ADCSR ADEN ADSC ADFR ADIF ADIE ADPS2 ADPS1 ADPS0 103
$05 ($25) ADCH ADC Data Register High Byte 104
$04 ($24) ADCL ADC Data Register Low Byte 104
… Reserved
$00 ($20) Reserved
8
1477KS–AVR–08/10
ATtiny26(L)
Instruction Set Summary
Mnemonic Operands Description Operation Flags # Clocks
ARITHMETIC AND LOGIC INSTRUCTIONS
ADD Rd, Rr Add Two Registers Rd ← Rd + Rr Z,C,N,V,H 1
ADC Rd, Rr Add with Carry Two Registers Rd ← Rd + Rr + C Z,C,N,V,H 1
ADIW Rdl, K Add Immediate to Word Rdh:Rdl ← Rdh:Rdl + K Z,C,N,V,S 2
SUB Rd, Rr Subtract Two Registers Rd ← Rd - Rr Z,C,N,V,H 1
SUBI Rd, K Subtract Constant from Register Rd ← Rd - K Z,C,N,V,H 1
SBC Rd, Rr Subtract with Carry Two Registers Rd ← Rd - Rr - C Z,C,N,V,H 1
SBCI Rd, K Subtract with Carry Constant from Reg. Rd ← Rd - K - C Z,C,N,V,H 1
SBIW Rdl, K Subtract Immediate from Word Rdh:Rdl ← Rdh:Rdl - K Z,C,N,V,S 2
AND Rd, Rr Logical AND Registers Rd ← Rd • Rr Z,N,V 1
ANDI Rd, K Logical AND Register and Constant Rd ← Rd • K Z,N,V 1
OR Rd, Rr Logical OR Registers Rd ← Rd v Rr Z,N,V 1
ORI Rd, K Logical OR Register and Constant Rd ← Rd v K Z,N,V 1
EOR Rd, Rr Exclusive OR Registers Rd ← Rd ⊕ Rr Z,N,V 1
COM Rd One’s Complement Rd ← $FF - Rd Z,C,N,V 1
NEG Rd Two’s Complement Rd ← $00 - Rd Z,C,N,V,H 1
SBR Rd, K Set Bit(s) in Register Rd ← Rd v K Z,N,V 1
CBR Rd, K Clear Bit(s) in Register Rd ← Rd • ($FF - K) Z,N,V 1
INC Rd Increment Rd ← Rd + 1 Z,N,V 1
DEC Rd Decrement Rd ← Rd - 1 Z,N,V 1
TST Rd Test for Zero or Minus Rd ← Rd • Rd Z,N,V 1
CLR Rd Clear Register Rd ← Rd ⊕ Rd Z,N,V 1
SER Rd Set Register Rd ← $FF None 1
BRANCH INSTRUCTIONS
RJMP k Relative Jump PC ← PC + k + 1 None 2
IJMP Indirect Jump to (Z) PC ← Z None 2
RCALL k Relative Subroutine Call PC ← PC + k + 1 None 3
ICALL Indirect Call to (Z) PC ← Z None 3
RET Subroutine Return PC ← STACK None 4
RETI Interrupt Return PC ← STACK I 4
CPSE Rd, Rr Compare, Skip if Equal if (Rd = Rr) PC ← PC + 2 or 3 None 1/2/3
CP Rd, Rr Compare Rd - Rr Z,N,V,C,H 1
CPC Rd, Rr Compare with Carry Rd - Rr - C Z,N,V,C,H 1
CPI Rd, K Compare Register with Immediate Rd - K Z,N,V,C,H 1
SBRC Rr, b Skip if Bit in Register Cleared if (Rr(b) = 0) PC ← PC + 2 or 3 None 1/2/3
SBRS Rr, b Skip if Bit in Register is Set if (Rr(b) = 1) PC ← PC + 2 or 3 None 1/2/3
SBIC P, b Skip if Bit in I/O Register Cleared if (P(b) = 0) PC ← PC + 2 or 3 None 1/2/3
SBIS P, b Skip if Bit in I/O Register is Set if (P(b) = 1) PC ← PC + 2 or 3 None 1/2/3
BRBS s, k Branch if Status Flag Set if (SREG(s) = 1) then PC ← PC + k + 1 None 1/2
BRBC s, k Branch if Status Flag Cleared if (SREG(s) = 0) then PC ← PC + k + 1 None 1/2
BREQ k Branch if Equal if (Z = 1) then PC ← PC + k + 1 None 1/2
BRNE k Branch if Not Equal if (Z = 0) then PC ← PC + k + 1 None 1/2
BRCS k Branch if Carry Set if (C = 1) then PC ← PC + k + 1 None 1/2
BRCC k Branch if Carry Cleared if (C = 0) then PC ← PC + k + 1 None 1/2
BRSH k Branch if Same or Higher if (C = 0) then PC ← PC + k + 1 None 1/2
BRLO k Branch if Lower if (C = 1) then PC ← PC + k + 1 None 1/2
BRMI k Branch if Minus if (N = 1) then PC ← PC + k + 1 None 1/2
BRPL k Branch if Plus if (N = 0) then PC ← PC + k + 1 None 1/2
BRGE k Branch if Greater or Equal, Signed if (N ⊕ V = 0) then PC ← PC + k + 1 None 1/2
BRLT k Branch if Less than Zero, Signed if (N ⊕ V = 1) then PC ← PC + k + 1 None 1/2
BRHS k Branch if Half-carry Flag Set if (H = 1) then PC ← PC + k + 1 None 1/2
BRHC k Branch if Half-carry Flag Cleared if (H = 0) then PC ← PC + k + 1 None 1/2
BRTS k Branch if T-flag Set if (T = 1) then PC ← PC + k + 1 None 1/2
BRTC k Branch if T-flag Cleared if (T = 0) then PC ← PC + k + 1 None 1/2
BRVS k Branch if Overflow Flag is Set if (V = 1) then PC ← PC + k + 1 None 1/2
BRVC k Branch if Overflow Flag is Cleared if (V = 0) then PC ← PC + k + 1 None 1/2
BRIE k Branch if Interrupt Enabled if (I = 1) then PC ← PC + k + 1 None 1/2
BRID k Branch if Interrupt Disabled if (I = 0) then PC ← PC + k + 1 None 1/2
DATA TRANSFER INSTRUCTIONS
MOV Rd, Rr Move between Registers Rd ← Rr None 1
LDI Rd, K Load Immediate Rd ← K None 1
LD Rd, X Load Indirect Rd ← (X) None 2
LD Rd, X+ Load Indirect and Post-inc. Rd ← (X), X ← X + 1 None 2
LD Rd, -X Load Indirect and Pre-dec. X ← X - 1, Rd ← (X) None 2
9
1477KS–AVR–08/10
ATtiny26(L)
LD Rd, Y Load Indirect Rd ← (Y) None 2
LD Rd, Y+ Load Indirect and Post-inc. Rd ← (Y), Y ← Y + 1 None 2
LD Rd, -Y Load Indirect and Pre-dec. Y ← Y - 1, Rd ← (Y) None 2
LDD Rd,Y+q Load Indirect with Displacement Rd ← (Y + q) None 2
LD Rd, Z Load Indirect Rd ← (Z) None 2
LD Rd, Z+ Load Indirect and Post-inc. Rd ← (Z), Z ← Z + 1 None 2
LD Rd, -Z Load Indirect and Pre-dec. Z ← Z - 1, Rd ← (Z) None 2
LDD Rd, Z+q Load Indirect with Displacement Rd ← (Z + q) None 2
LDS Rd, k Load Direct from SRAM Rd ← (k) None 2
ST X, Rr Store Indirect (X) ← Rr None 2
ST X+, Rr Store Indirect and Post-inc. (X) ← Rr, X ← X + 1 None 2
ST -X, Rr Store Indirect and Pre-dec. X ← X - 1, (X) ← Rr None 2
ST Y, Rr Store Indirect (Y) ← Rr None 2
ST Y+, Rr Store Indirect and Post-inc. (Y) ← Rr, Y ← Y + 1 None 2
ST -Y, Rr Store Indirect and Pre-dec. Y ← Y - 1, (Y) ← Rr None 2
STD Y+q, Rr Store Indirect with Displacement (Y + q) ← Rr None 2
ST Z, Rr Store Indirect (Z) ← Rr None 2
ST Z+, Rr Store Indirect and Post-inc. (Z) ← Rr, Z ← Z + 1 None 2
ST -Z, Rr Store Indirect and Pre-dec. Z ← Z - 1, (Z) ← Rr None 2
STD Z+q, Rr Store Indirect with Displacement (Z + q) ← Rr None 2
STS k, Rr Store Direct to SRAM (k) ← Rr None 2
LPM Load Program Memory R0 ← (Z) None 3
LPM Rd, Z Load Program Memory Rd ← (Z) None 3
IN Rd, P In Port Rd ← P None 1
OUT P, Rr Out Port P ← Rr None 1
PUSH Rr Push Register on Stack STACK ← Rr None 2
POP Rd Pop Register from Stack Rd ← STACK None 2
BIT AND BIT-TEST INSTRUCTIONS
SBI P, b Set Bit in I/O Register I/O(P,b) ← 1 None 2
CBI P, b Clear Bit in I/O Register I/O(P,b) ← 0 None 2
LSL Rd Logical Shift Left Rd(n+1) ← Rd(n), Rd(0) ← 0 Z,C,N,V 1
LSR Rd Logical Shift Right Rd(n) ← Rd(n+1), Rd(7) ← 0 Z,C,N,V 1
ROL Rd Rotate Left through Carry Rd(0) ← C, Rd(n+1) ← Rd(n), C ← Rd(7) Z,C,N,V 1
ROR Rd Rotate Right through Carry Rd(7) ← C, Rd(n) ← Rd(n+1), C ← Rd(0) Z,C,N,V 1
ASR Rd Arithmetic Shift Right Rd(n) ← Rd(n+1), n = 0..6 Z,C,N,V 1
SWAP Rd Swap Nibbles Rd(3..0) ← Rd(7..4), Rd(7..4) ← Rd(3..0) None 1
BSET s Flag Set SREG(s) ← 1 SREG(s) 1
BCLR s Flag Clear SREG(s) ← 0 SREG(s) 1
BST Rr, b Bit Store from Register to T T ← Rr(b) T 1
BLD Rd, b Bit Load from T to Register Rd(b) ← T None 1
SEC Set Carry C ← 1 C 1
CLC Clear Carry C ← 0 C 1
SEN Set Negative Flag N ← 1 N 1
CLN Clear Negative Flag N ← 0 N 1
SEZ Set Zero Flag Z ← 1 Z 1
CLZ Clear Zero Flag Z ← 0 Z 1
SEI Global Interrupt Enable I ← 1 I 1
CLI Global Interrupt Disable I ← 0 I 1
SES Set Signed Test Flag S ← 1 S 1
CLS Clear Signed Test Flag S ← 0 S 1
SEV Set Two’s Complement Overflow V ← 1 V 1
CLV Clear Two’s Complement Overflow V ← 0 V 1
SET Set T in SREG T ← 1 T 1
CLT Clear T in SREG T ← 0 T 1
SEH Set Half-carry Flag in SREG H ← 1 H 1
CLH Clear Half-carry Flag in SREG H ← 0 H 1
NOP No Operation None 1
SLEEP Sleep (see specific descr. for Sleep function) None 1
WDR Watchdog Reset (see specific descr. for WDR/timer) None 1
Instruction Set Summary (Continued)
Mnemonic Operands Description Operation Flags # Clocks
10
1477KS–AVR–08/10
ATtiny26(L)
Notes: 1. This device can also be supplied in wafer form. Please contact your local Atmel sales office for detailed ordering information
and minimum quantities.
2. Pb-free packaging alternative, complies to the European Directive for Restriction of Hazardous Substances (RoHS directive).
Also Halide free and fully Green.
3. Code Indicators:
– U: matte tin
– R: tape & reel
Ordering Information
Speed (MHz) Power Supply (V) Ordering Code(2) Package(2) Operational Range
8 2.7 - 5.5
ATtiny26L-8PU
ATtiny26L-8SU
ATtiny26L-8SUR
ATtiny26L-8MU
ATtiny26L-8MUR
20P3
20S
20S
32M1-A
32M1-A
Industrial
(-40°C to +85°C)(1)
16 4.5 - 5.5
ATtiny26-16PU
ATtiny26-16SU
ATtiny26-16SUR
ATtiny26-16MU
ATtiny26-16MUR
20P3
20S
20S
32M1-A
32M1-A
Industrial
(-40°C to +85°C)(1)
Package Type
20P3 20-lead, 0.300" Wide, Plastic Dual Inline Package (PDIP)
20S 20-lead, 0.300" Wide, Plastic Gull Wing Small Outline (SOIC)
32M1-A 32-pad, 5 x 5 x 1.0 body, Lead Pitch 0.50 mm Quad Flat No-Lead/Micro Lead Frame Package (QFN/MLF)
11
1477KS–AVR–08/10
ATtiny26(L)
Packaging Information
20P3
2325 Orchard Parkway
San Jose, CA 95131
TITLE DRAWING NO.
R
REV.
20P3, 20-lead (0.300"/7.62 mm Wide) Plastic Dual
Inline Package (PDIP) 20P3 C
1/12/04
PIN
1
E1
A1
B
E
B1
C
L
SEATING PLANE
A
D
e
eB
eC
COMMON DIMENSIONS
(Unit of Measure = mm)
SYMBOL MIN NOM MAX NOTE
A – – 5.334
A1 0.381 – –
D 25.493 – 25.984 Note 2
E 7.620 – 8.255
E1 6.096 – 7.112 Note 2
B 0.356 – 0.559
B1 1.270 – 1.551
L 2.921 – 3.810
C 0.203 – 0.356
eB – – 10.922
eC 0.000 – 1.524
e 2.540 TYP
Notes: 1. This package conforms to JEDEC reference MS-001, Variation AD.
2. Dimensions D and E1 do not include mold Flash or Protrusion.
Mold Flash or Protrusion shall not exceed 0.25 mm (0.010").
12
1477KS–AVR–08/10
ATtiny26(L)
20S
13
1477KS–AVR–08/10
ATtiny26(L)
32M1-A
2325 Orchard Parkway
San Jose, CA 95131
TITLE DRAWING NO.
R
REV.
32M1-A, 32-pad, 5 x 5 x 1.0 mm Body, Lead Pitch 0.50 mm, 32M1-A E
5/25/06
3.10 mm Exposed Pad, Micro Lead Frame Package (MLF)
COMMON DIMENSIONS
(Unit of Measure = mm)
SYMBOL MIN NOM MAX NOTE
D1
D
E1 E
b e
A3
A2
A1
A
D2
E2
0.08 C
L
1
2
3
P
P
0
1
2
3
A 0.80 0.90 1.00
A1 – 0.02 0.05
A2 – 0.65 1.00
A3 0.20 REF
b 0.18 0.23 0.30
D
D1
D2 2.95 3.10 3.25
4.90 5.00 5.10
4.70 4.75 4.80
4.70 4.75 4.80
4.90 5.00 5.10
E
E1
E2 2.95 3.10 3.25
e 0.50 BSC
L 0.30 0.40 0.50
P – – 0.60
– – 12o
Note: JEDEC Standard MO-220, Fig. 2 (Anvil Singulation), VHHD-2.
TOP VIEW
SIDE VIEW
BOTTOM VIEW
0
Pin 1 ID
Pin #1 Notch
(0.20 R)
K 0.20 – –
K
K
14
1477KS–AVR–08/10
ATtiny26(L)
Errata The revision letter refers to the revision of the device.
ATtiny26 Rev.
B/C/D
• First Analog Comparator conversion may be delayed
1. First Analog Comparator conversion may be delayed
If the device is powered by a slow rising VCC, the first Analog Comparator conversion will
take longer than expected on some devices.
Problem Fix/Workaround
When the device has been powered or reset, disable then enable the Analog Comparator
before the first conversion.
15
1477KS–AVR–08/10
ATtiny26(L)
Datasheet
Revision
History
Please note that the referring page numbers in this section refer to the complete document.
Rev. 1477K-08/10 Added tape and reel part numbers in “Ordering Information” on page 171. Removed text
“Not recommended for new design” from cover page. Updated last page.
Rev. 1477J-06/07 1. “Not recommended for new design”
Rev. 1477I-05/06 1. Updated “Errata” on page 175
Rev. 1477H-04/06 1. Updated typos.
2. Added “Resources” on page 6.
3. Updated features in “System Control and Reset” on page 32.
4. Updated “Prescaling and Conversion Timing” on page 96.
5. Updated algorithm for “Enter Programming Mode” on page 112.
Rev. 1477G-03/05 1. MLF-package alternative changed to “Quad Flat No-Lead/Micro Lead Frame Package
QFN/MLF”.
2. Updated “Electrical Characteristics” on page 126
3. Updated “Ordering Information” on page 171
Rev. 1477F-12/04 1. Updated Table 16 on page 33, Table 9 on page 28, and Table 29 on page 57.
2. Added Table 20 on page 40.
3. Added “Changing Channel or Reference Selection” on page 98.
4. Updated “Offset Compensation Schemes” on page 105.
5. Updated “Electrical Characteristics” on page 126.
6. Updated package information for “20P3” on page 172.
7. Rearranged some sections in the datasheet.
Rev. 1477E-10/03 1. Removed Preliminary references.
2. Updated “Features” on page 1.
3. Removed SSOP package reference from “Pin Configuration” on page 2.
4. Updated VRST and tRST in Table 16 on page 33.
5. Updated “Calibrated Internal RC Oscillator” on page 29.
16
1477KS–AVR–08/10
ATtiny26(L)
6. Updated DC Characteristics for VOL, IIL, IIH, ICC Power Down and VACIO in “Electrical
Characteristics” on page 126.
7. Updated VINT, INL and Gain Error in “ADC Characteristics” on page 129 and page 130.
Fixed typo in “Absolute Accuracy” on page 130.
8. Added Figure 106 in “Pin Driver Strength” on page 146, Figure 120, Figure 121 and
Figure 122 in “BOD Thresholds and Analog Comparator Offset” on page 155. Updated
Figure 117 and Figure 118.
9. Removed LPM Rd, Z+ from “Instruction Set Summary” on page 169. This instruction
is not supported in ATtiny26.
Rev. 1477D-05/03 1. Updated “Packaging Information” on page 172.
2. Removed ADHSM from “ADC Characteristics” on page 129.
3. Added section “EEPROM Write During Power-down Sleep Mode” on page 20.
4. Added section “Default Clock Source” on page 26.
5. Corrected PLL Lock value in the “Bit 0 – PLOCK: PLL Lock Detector” on page 73.
6. Added information about conversion time when selecting differential channels on
page 97.
7. Corrected {DDxn, PORTxn} value on page 42.
8. Added section “Unconnected Pins” on page 46.
9. Added note for RSTDISBL Fuse in Table 50 on page 108.
10. Corrected DATA value in Figure 61 on page 116.
11. Added WD_FUSE period in Table 60 on page 123.
12. Updated “ADC Characteristics” on page 129 and added Table 66, “ADC Characteristics,
Differential Channels, TA = -40°C to +85°C,” on page 130.
13. Updated “ATtiny26 Typical Characteristics” on page 131.
14. Added LPM Rd, Z and LPM Rd, Z+ in “Instruction Set Summary” on page 169.
Rev. 1477C-09/02 1. Changed the Endurance on the Flash to 10,000 Write/Erase Cycles.
Rev. 1477B-04/02 1. Removed all references to Power Save sleep mode in the section “System Clock and
Clock Options” on page 23.
2. Updated the section “Analog to Digital Converter” on page 94 with more details on
how to read the conversion result for both differential and single-ended conversion.
3. Updated “Ordering Information” on page 171 and added QFN/MLF package
information.
Rev. 1477A-03/02 1. Initial version.
17
1477KS–AVR–08/10
ATtiny26(L)
1477KS–AVR–08/10
Headquarters International
Atmel Corporation
2325 Orchard Parkway
San Jose, CA 95131
USA
Tel: 1(408) 441-0311
Fax: 1(408) 487-2600
Atmel Asia
Unit 1-5 & 16, 19/F
BEA Tower, Millennium City 5
418 Kwun Tong Road
Kwun Tong, Kowloon
Hong Kong
Tel: (852) 2245-6100
Fax: (852) 2722-1369
Atmel Europe
Le Krebs
8, Rue Jean-Pierre Timbaud
BP 309
78054 Saint-Quentin-en-
Yvelines Cedex
France
Tel: (33) 1-30-60-70-00
Fax: (33) 1-30-60-71-11
Atmel Japan
9F, Tonetsu Shinkawa Bldg.
1-24-8 Shinkawa
Chuo-ku, Tokyo 104-0033
Japan
Tel: (81) 3-3523-3551
Fax: (81) 3-3523-7581
Product Contact
Web Site
www.atmel.com
Technical Support
Enter Product Line E-mail
Sales Contact
www.atmel.com/contacts
Literature Requests
www.atmel.com/literature
Disclaimer: The information in this document is provided in connection with Atmel products. No license, express or implied, by estoppel or otherwise, to any
intellectual property right is granted by this document or in connection with the sale of Atmel products. EXCEPT AS SET FORTH IN ATMEL’S TERMS AND CONDITIONS
OF SALE LOCATED ON ATMEL’S WEB SITE, ATMEL ASSUMES NO LIABILITY WHATSOEVER AND DISCLAIMS ANY EXPRESS, IMPLIED OR STATUTORY
WARRANTY RELATING TO ITS PRODUCTS INCLUDING, BUT NOT LIMITED TO, THE IMPLIED WARRANTY OF MERCHANTABILITY, FITNESS FOR A PARTICULAR
PURPOSE, OR NON-INFRINGEMENT. IN NO EVENT SHALL ATMEL BE LIABLE FOR ANY DIRECT, INDIRECT, CONSEQUENTIAL, PUNITIVE, SPECIAL OR INCIDENTAL
DAMAGES (INCLUDING, WITHOUT LIMITATION, DAMAGES FOR LOSS OF PROFITS, BUSINESS INTERRUPTION, OR LOSS OF INFORMATION) ARISING OUT
OF THE USE OR INABILITY TO USE THIS DOCUMENT, EVEN IF ATMEL HAS BEEN ADVISED OF THE POSSIBILITY OF SUCH DAMAGES. Atmel makes no
representations or warranties with respect to the accuracy or completeness of the contents of this document and reserves the right to make changes to specifications
and product descriptions at any time without notice. Atmel does not make any commitment to update the information contained herein. Unless specifically provided
otherwise, Atmel products are not suitable for, and shall not be used in, automotive applications. Atmel’s products are not intended, authorized, or warranted for use
as components in applications intended to support or sustain life.
© 2010 Atmel Corporation. All rights reserved. Atmel®, logo and combinations thereof, AVR® and others are registered trademarks or trademarks
of Atmel Corporation or its subsidiaries. Other terms and product names may be trademarks of others.
Un design innovant pour une utilisation efficace et confortable
Un boîtier léger et compact et une interface utilisateur conviviale
Un afficheur d’une dimension et d’une lisibilité inégalées
Une calibration 100% numérique pour une précision et un coût maîtrisés
Des versions entièrement programmables respectant la norme SCPI
Nouvelle technologie d’affichage intelligent (Smart Persistence Oscilloscope)
Des atouts qui séduiront l’Enseignement et l’Industrie
Si intelligents que vous pouvez les choisir
pour leurs courbes !
Série MTX COMPACT
« Une gamme complète et performante d’instruments de laboratoire »
MTX 3252
MTX 3352
MTX 3354
MTX 3240
MTX 3250
MTX 3250 Multimètre-Analyseur
MTX 3240 Générateur BF-Mesureur
MTX 3252 - MTX 3352 - MTX 3354 Oscilloscopes-Analyseurs
Tout comme le générateur de la même
famille, le multimètre MTX est un appareil
surdoué, multifonction. Grâce à son analyse du
signal il évite à l’utilisateur la mise en oeuvre
d’autres instruments (oscilloscope par exemple),
pour contrôler la validité des mesures réalisées.
Impossible de commettre les, si fréquentes
et souvent ignorées, erreurs dues à un facteur
de crête trop élevé. En effet, le MTX 3250
mesure en permanence les crêtes rapides à
500 μs et vous alerte en cas d’anomalie.
Mieux encore, en validant alors le “Mode
AUTO PEAK”, le multimètre commutera automatiquement
sur une gamme adaptée à la
nature du signal mesuré. L’affichage du facteur
de crête vous permettra aussi d’établir un
premier diagnostic qualitatif sur vos signaux.
Le MTX 3250, c’est encore la rationalisation de
l’investissement réalisé, puisqu’il est également
fréquencemètre, thermomètre et même
enregistreur, vous évitant ainsi l’achat d’un
instrument d’usage ponctuel. Ainsi, pour les
enregistrements en laboratoire jusqu’à 4 voies
et 12 paramètres, la version “acquisition de
données” et son logiciel PC associé rendront
un service performant à partir d’un instrument
polyvalent.
La température est directement mesurée à
partir de Pt 100 ou Pt 1000, de même que la
fréquence, jusqu’à 1 MHz, avec période et
rapport cyclique.
Et pour mieux répondre à vos attentes dans
le cadre de systèmes automatisés, cet
instrument existe en version programmable
à 100 % via une liaison optique RS232 à
57600 bauds, compatible SCPI.
Un multimètre
à la pointe
des modes
50 000 points 500 mV - 500 mV - 600 V 500 μA - 500 mA & 10 A 5 μA – 500 mA & 10A 500 Ω - 50 MΩ 170 x 270 x 190 mm optique RS232
LCD 50 x 140 mm 500 V & 0,5%L + 3D 0,2%L + 3D 0,5%L + 3D 0,1%L + 3D Masse : 2,3 kg 57 600 bauds
Rétro-éclairage 1000 V (50 000 pts) 10 kHz
Aff. triple 0,08%L+3D 100 kHz
CARACTÉRISTIQUES :
Affichage Gammes et Gammes et Gammes et Gammes et Gammes et Dimensions Interface
précision précision VAC de base précision IDC de base précision IAC précision de base H x L x P MTX 3250-P
de base VDC Bande passante Bande passante Ohm MTX 3250-A
Autres mesures : test continuité, test diode capacités 50 nF – 50 mF, fréquence 1 Hz - 1 MHz,
rapport cyclique 0,01% à 100%, température - 200 à + 800°C, pt 100 et pt 1000.
Fonction PEAK HOLD : Pk+/ -500 μs sur I & V, facteur de crête
Fonctions complémentaires : SURV = MIN/MAX datés / MATH = dB, dBm, ax+b / OFFSET
(Offset, nul, delta%) / Data HOLD & Auto HOLD
Fonction supplémentaire sur MTX 3250-P :
PRINT, cadence 0,5 s à 10 h, horloge et calendrier, pilotage RS232 optique
Fonction supplémentaire sur MTX 3250-A :
DATA LOGGER avec 1500 mesures stockées, 1 ou 3 valeurs simultanées.
Normes : sécurité selon IEC 61010-1, 2001 et CEM selon NF 61326-1, 1998
Garantie : 3 ans
Le multimètre MTX 3250 est fourni avec 1 câble d’alimentation secteur, 1 jeu de cordons de mesure, une notice de fonctionnement
et une présentation interactive de l’instrument sur CD-Rom.
Pour commander
MTX3250 Multimètre de Table 50 000 pts
MTX3250-P Multimètre de Table 50 000 pts + RS232
Fourni avec un cordon de liaison optique RS232, un manuel de programmation et les drivers Labwindows / Labview sur CD-Rom.
MTX3250-A Multimètre de Table 50 000 pts + Acquisition
Fourni avec un cordon de liaison optique RS232, un manuel de programmation, les drivers Labwindows / Labview et le logiciel
d’acquisition de données SX-DMM sur CD-Rom.
Tout commence avec une connexion réduite à
3 bornes qui limite erreurs et manipulations,
permettant un “AUTORANGING” courant complet
de 50 μA à 20 A.
Le MTX 3250 offre ensuite, grâce à son affichage
triple, les combinaisons de mesures qui
répondent simplement et efficacement à vos
applications courantes, comme, par exemple,
la mesure de bande passante (affichage de l’atténuation
en dB et de la fréquence).
Accessoires et informations pour commander
Pour la maîtrise métrologique, le “Mode
SPEC” calcule et affiche les incertitudes de
l’instrument en fonction des gammes et de la
valeur mesurée
Le Mode offre la lecture directe
de la grandeur mesurée, ainsi que l’unité
physique correspondante
Le “Mode Surveillance” enregistre
les minima et les maxima afin de piéger et
de dater vos défauts
L’association
fonctionnelle
Le “Mode RELATIF” exprimé en absolu,
pourcentage ou dB (ratio), permet une
exploitation directe
MTX 3250 : multimètre-analyseur intégré
Asservissement et affichage de la fréquence
Contrôle et affichage de l’AMPLITUDE VCC
(crête/crête) et de l’OFFSET VDC
Contrôle et affichage du rapport cyclique
Le MTX 3240 c’est aussi la rationalisation de
l’investissement réalisé, puisqu’il est aussi un
fréquencemètre 100 MHz (Cat. I, 300 V), vous
évitant ainsi l’achat d’un instrument
d’usage souvent ponctuel.
Et pour répondre enfin de manière économique
à vos attentes dans le cadre de systèmes automatisés,
ce générateur existe en version
programmable à 100 % via une liaison rapide,
compatible SCPI.
Sa technologie permet à chacun de bénéficier
de fonctions nouvelles, indispensables :
Réglage de la fréquence, garantie stable
au digit près, et accélérateur intelligent
avec changement de gammes automatique
pour la fréquence
Changement de gammes automatique
optimisé pour l’amplitude
“LEVEL et OFFSET”
Rapport cyclique réglable sans variation
ni division de la fréquence
Fonction “LOGIC”
pour une réponse simple et rapide à la
génération de signaux logiques à seuils
directement ajustables
Un générateur robuste, avec des sorties
protégées 60 VDC / 40 VAC
L’association fonctionnelle
MTX 3240 : générateur-mesureur autonome
Un générateur
doté de caractéristiques
innovantes
LCD 50 x 140 mm 0,1 Hz à 5,1 MHz Sinus, carré, 1) Principale : LIN ou LOG 0,1 Hz à 100 MHz 115 V - 230 V - 170x270x190 mm optique
Afficheur principal 7 gammes + triangle, jusqu’à 20 VCC CONTINU, Précision : 0,05 % 240 V Masse : 2,8 kg RS232
20 mm réglage fin au digit impulsion, circuit ouvert, 1 : 50 Min Entrée 300 V, Cat. I 50 / 60 Hz
4 grandeurs près + accélérateur rampe, TTL, gamme automatique De 10 ms à 10 s Sensibilité 300 V, Cat.II
simultanées Précision : 0,05 % LOGIC 2) TTL Interne ou externe automatique
Distorsion Protection : overload
< 0,5 % 60 VDC / 40 VAC
CARACTÉRISTIQUES :
Affichage Gamme Formes Sorties Balayage Fréquencemètre Alimentation Dimensions Interface
de fréquence de signaux externe H x L x P (MTX 3240-P)
Normes : sécurité selon IEC 61010-1, 2001 et CEM selon NF 61326-1, 1998
Garantie : 3 ans
Le générateur MTX 3240 est fourni avec un câble d’alimentation secteur, une notice de fonctionnement et une présentation interactive
de l’instrument sur CD-Rom.
Pour commander
MTX3240 Générateur de Fonctions 5,1 MHz
MTX3240-P Générateur de Fonctions 5,1 MHz + RS232
Fourni avec un cordon de liaison optique RS232, un manuel de programmation et les drivers Labwindows / Labview sur CD-Rom.
Accessoires et informations pour commander
Autre apport de l’innovation pour l’utilisateur : une fonctionnalité complète pour l’investissement
réalisé. En effet, l’association fonctionnelle du MX 3240 permet sa mise en oeuvre autonome,
ce qui évite, par exemple, l’utilisation systématique d’un oscilloscope ou d‘un multimètre,
simplement pour en contrôler les réglages.
MTX 3240
avec fréquencemètre
intégré
Interface Homme-Machine,
la simplicité au service
de la performance
L’instrument peut être piloté par la souris
ou le clavier
Les réglages sont simplifiés et conviviaux
grâce aux 21 touches d’accès direct de la
face avant et à l’environnement
« Windows-Like »
Une aide en ligne détaillée et en 5 langues
est disponible à tout moment grâce à la
touche
Léger, compact et pourvu d'une poignée,
il dispose d’un « Pack Terrain » qui permet
la mise en oeuvre de l ‘oscilloscope sans le
sortir de sa sacoche
Affichage
Des curseurs peuvent être placés à tout
moment sur les signaux pour réaliser des
mesures précises
Grâce à une profondeur mémoire de
50.000 points, le zoom de trace horizontal,
« Winzoom », peut aller jusqu’à un facteur
x100 en affichant des vrais points
acquis .
Une dynamique verticale exceptionnelle
de 2,5 mV à 100 V par div.
La fonction « full trace » permet de diviser
l’écran en deux afin d’optimiser la lisibilité
des courbes
Affichage jusqu’à 4 courbes à l’écran et
possibilité d’établir des comparaisons
entre deux courbes.
Pour plus de simplicité et un gain de
temps conséquent, l’utilisateur peut
sélectionner et afficher 2 mesures automatiques
parmi 19.
MTX 3252, MTX 3352 et MTX 3354 :
Oscilloscopes – analyseurs 2 ou 4 voies, de 60 à 150 MHz !
Experts en communication
Équipés d’une liaison RS232, de l’interface
Centronics et d’une liaison USB
indispensables à la communication vers
un PC ou une imprimante
Gestion à distance grâce à la liaison
Ethernet et au serveur HTML présent
dans chaque instrument
Des fonctions complexes d’analyse sont
accessibles en mode « avancé » , elles
sont masquées en mode « standard »
dans un soucis de simplification.
Enregistrement,
une mémoire infinie …,
Les oscilloscopes de la gamme MTX
COMPACT disposent d’un écran LCD
couleur 5"7 avec rétro éclairage pour une
excellente précision de lecture
Le choix est large puisque MTX COMPACT
propose trois appareils de 2 et 4
voies de bande passante 60 MHz,
100 MHz ou 150 MHz qui séduiront aussi
bien l'Enseignement Technique que
l’Industrie
Le déclenchement dispose de 5 modes
différents : Pulse, déclenchement sur largeur
d'impulsion, Retard, déclenchement
sur fronts avec retardateur, TV, déclenchement
sur un signal TV, Comptage,
déclenchement sur font avec comptage
d'événements et Secteur, déclenchement
sur le front ascendant ou descendant
de la tension réseau 50/60 Hz
Outre ces multiples modes de paramétrage,
le Holdoff est disponible sur la majorité
de ces fonctions de déclenchement
, technologie d’affichage
de type « analogique
» qui permet de faire
apparaître les évolutions
du signal (modulations, jitters
etc.) et les phénomènes
uniques (transitoires,
glitchs, etc.)
La profondeur mémoire de 50.000 points
est une référence dans cette catégorie
d’oscilloscopes
Durée d’enregistrement et fréquence d’échantillonnage
20 fois plus élevées qu’un
oscilloscope traditionnel
Les oscilloscopes de la gamme MTX
COMPACT dispose d’une résolution
exceptionnelle de 100 Gé/s en mode
répétitif et 200 Mé/s en mode monocoup,
ce qui permet d’avoir des calibres de base
de temps allant de 200 s/div à 1ns/div.
Enregistrement de courbes et rappel à l’écran
Possibilité de sauvegarder des fichiers
dans l’instrument, de les imprimer ou de
les exporter vers un PC en vue d’une
exploitation ultérieure dans les applications
« Windows » (rapports, tableurs,
impression, images …)
Les traces et les fichiers enregistrés sont
horodatés
Les fichiers sont générés dans des formats
standards : .gif, .pcl, .txt, .bmp, .eps,
.prn, etc.
Des instruments intégrés
pour un « outil global »
L’ensemble des oscilloscopes sont pourvus
de la fonction analyse FFT temps réel
et multivoie du signal
Pour les utilisateurs du domaine de
l’Electrotechnique, nous proposons en
option l’analyse d’harmoniques multivoies
31 rangs
Enfin, pour tous ceux qui doivent surveiller
dans le temps les variations de phénomènes
physiques ou mécaniques , un enregistreur
numérique rapide est intégrable
dans l’instrument, sous forme d’un module
software
MTX 3252, MTX 3352 et MTX 3354 :
Oscilloscopes – analyseurs 2 ou 4 voies, de 60 à 150 MHz !
Ergonomie
La technologie " " permet de faire persister les acquisitions pendant une durée
paramétrée pour observer un cumul de traces. L’intensité lumineuse ou la couleur, affectée
au point à l’écran, va décroître si celui-ci n’est pas renouvelé lors d’une nouvelle
acquisition.
L’acquisition se fait donc en trois dimensions :
- le temps
- l’amplitude
- l’occurrence
Grâce à sa profondeur mémoire de 50.000 points, l’oscilloscope acquiert et traite
l’information en parallèle.
Le nombre d’acquisitions à la seconde peut-être multiplié par un facteur supérieur à
1000, ainsi le temps mort entre deux acquisitions est considérablement réduit.
Représentation à l’écran des 50.000 points acquis par un système de compression
intelligente.
L’occurrence apporte une dimension statistique à la répartition des échantillons. La
couleur ou l’intensité lumineuse met en évidence les irrégularités du signal.
Durées d’affichage des points acquis : 100ms, 200ms, 500ms, 1s, 2s, 5s, 10s et infini.
, Smart Persistence Oscilloscope :
L’outil indispensable de visualisation intelligente !
La technologie SPO
La nouvelle génération d’oscilloscopes MTX COMPACT est dotée de l'affichage " " (Smart Persistence Oscilloscope) qui permet, comme
en analogique, de faire apparaître les évolutions du signal dans le temps, les jitters, les modulations et les phénomènes instables.
Par ailleurs, ce mode d’affichage permet aussi la mise en évidence des phénomènes uniques tels que les transitoires ou les glitchs.
Acquisition
Traitement
rapide Affichage
Parallèle
N acquisitions = 1 affichage
Modulation AM ou FM
Évènements uniques
ou transitoires
Caractérisation du bruit
Signal vidéo
Applications
- MTX3354E-C : oscilloscope numérique
4x150MHz, couleur, Ethernet
- MTX3354E-CK : MTX3354E-C
+ SX-METRO/P
- MTX3252BE-C : oscilloscope numérique
2x60MHz, couleur, Ethernet
- MTX3352BE-C : oscilloscope numérique
2x100MHz, couleur, Ethernet
- MTX3252BED : MTX3252BE-C
+ sonde différentielle MTX1032-B
- MTX3352BED : MTX3352BE-C
+ sonde différentielle MTX1032-C
Pour commander
MTX 3252, MTX 3352 & MTX 3354 :
Oscilloscopes – analyseurs 60, 100 et 150 MHz
Interface Homme-Machine
Affichage LCD couleur 5” 7 (115x86mm) - LCD monochrome ou couleur 5” 7 (115x86mm)
320 x 240 + rétro éclairage CCFL - 320 x 240 + rétro éclairage CCFL
Nombre de courbes à l’écran 4 courbes + 4 références
Commandes 21 touches de raccourcis directs + 1 encodeur + 1 touche « aide »
Menu « Windows-like » - 100% des commandes accessibles via la souris
Choix des langues par le menu (FRA/ANG/ESP/ITA/ALL)
Déviation Verticale
Bande passante 150 MHz 100 MHz 60 MHz
(limiteur de BP 15 MHz (limiteur de BP (limiteur de BP
1,5 MHz et 5 kHz) 15 MHz) 15 MHz)
Nombre voies 4 voies classe 1 – Cat. II 300 V 2 voies classe 1 – Cat. II 300 V
Sensibilité 2,5 mV – 100 V/div + expansion verticale 2,5 mV – 100 V/div
"Winzoom" jusqu'à un facteur 10 + expansion verticale « Winzoom »
(sensibilité maximum 250 μV/div) jusqu’à un facteur 10
Temps de montée < 3 ns < 3,5 ns
Déviation Horizontale
Vitesse de balayage 1 ns à 200 s/div - winzoom graphique jusqu'à un facteur 100
Déclenchement
Modes Auto, Normal, Monocoup, Auto 50%
Types Front, Largeur d’impulsion, Retard, Comptage d’évènements, Compteur de lignes TV, Hold-off
Sources CH1, CH2, CH3, CH4, Secteur CH1, CH2, EXT et Secteur
Mémoire Numérique
Échantillonnage maxi. Répétitif = 100 Gé/s
Monocoup = 200 Mé/s (2 voies),
convertisseur 9 bits
Profondeur mémoire 50.000 points – 4 références + 16 courbes de 50 kpts
Modes d’affichage Glitch, Enveloppe, Moyennage, XY Numérique
SPO (Smart Persistence Oscilloscope)
Durée 100 ms, 200 ms, 500 ms, 1 s, 2 s, 5 s 100 s et Infini
Représentation Monochrome ou couleur
Vitesse d’acquisition 50 kwaveform/s max. par voie – 19 Mé traités par s et par voie
Mode Enregistreur
Cadence d’acquisition De 40 μs à 54 μs d’intervalle d’échantillonnage (2 s à 31 jours d’enregistrement)
Exploitation Horodatage direct, conversion et unités des grandeurs physiques,
mesures par curseurs et recherche d’événement, fichiers exploitables sur tableur standard
Mode Analyseur d’Harmoniques
Étendue d’analyse 31 rangs simultanément sur 1 à 4 voies
Exploitation Affichage permanent : valeur RMS totale THD – Rang sélectionné : %F, phase, freq, Vrms
Interface RS232, Centronics, USB, Ethernet avec serveur HTML
Caractéristiques Générales
Boîtier 210 x 177 x 200 – 2,5 Kg – IP30
Alimentation 100 à 240 VAC – 47 à 63 Hz
Sécurité IEC 1010-1 (2001) – Surtension de l’alimentation CAT II 240 V –
Surtension des entrées de mesure CAT II 300V
CEM NF EN 61326-1 07/97 + A1 10/98
CARACTÉRISTIQUES :
MTX 3354 MTX 3352 MTX 3252
AD.COM- Code : 906210055 - Ed.5 - 09/2007 - Caractéristiques sous réserve de modifications liées à l’évolution de la technologie
L’efficacité s’affiche avec élégance
Le design moderne et séduisant des appareils de la famille MTX permet, grâce à une forte
compacité, leur parfaite intégration dans votre cadre de travail.
Posés directement sur la paillasse, l’espace libéré devant eux est déjà très
appréciable, de plus, leur hauteur est calculée pour pouvoir les glisser
sans peine sous les demi-étagères. Leur faible profondeur et leur largeur
standard vous permettent aussi de les placer
sur ces mêmes demi-étagères ou de
les poser sur un autre instrument.
Le déplacement et le transport sont
très aisés grâce à leur poignée
intégrée et à leur légèreté.
Une architecture et des formes
avantageuses qui vous font gagner
de la place
Même à distance ou dans des conditions d’éclairage difficiles (soleil, néons), les mesures sont
parfaitement lisibles, grâce notamment à un afficheur négatif de grandes dimensions
(50 x 140 mm), à un rétro-éclairage à matrice de leds ajustable, ainsi qu’à une hauteur
exceptionnelle de 20 mm de l’afficheur principal (MTX 3240 et MTX 3250).
Monochrome ou couleur, l’écran LCD réglable et orientable des MTX 3252 et MTX 3352
vous garantira, lui aussi, une lisibilité en toutes circonstances.
Les zones fonctionnelles de l’ensemble des MTX Compact sont vastes, cohérentes
et hiérarchisées, et les connexions mesure facilement accessibles, puisque situées
en façade. Sur le générateur et le multimètre, la sélection des fonctions primaires
s’établit directement au moyen de touches avec leds de validation intégrées. Un
encodeur performant permet de réaliser efficacement les réglages et des touches
contextuelles en bord d’écran indiquent clairement la configuration.
Pour les oscilloscopes, en plus des accès directs aux fonctions essentielles par clavier et aux
réglages par encodeur, le pilotage via la souris, sous environnement “Windows-like”, est totalement
innovant dans cette catégorie d’appareil.
Une technologie de leader,
l’innovation jusqu’au bout
des doigts
Une lisibilité privilégiée,
une Interface Homme-Machine conviviale (I.H.M.)
Les qualités des MTX ne se limitent pas à
leur physique. Ces instruments ont une
tête bien faite, grâce notamment à leurs
micro-processeurs 16 ou 32 bits de
dernière génération, aux logiciels téléchargeables
et à la calibration 100%
numérique. Du point de vue de la sécurité,
une protection électronique réarmable a
permis la suppression du fusible secteur
sur certains modèles.
Tous les modèles de la famille MTX
Compact peuvent disposer d’interfaces
de communication performantes et
du langage standard SCPI.
Même le clavier de sélection est
à la pointe de la technologie
grâce à ses contacts à microswitches
qui assurent une
durabilité exceptionnelle de
plus de 100 000 manoeuvres.
Les touches bénéficient,
quant à elles, d’une gravure laser inaltérable.
Avec la famille MTX Compact, Metrix permet
à chaque professionnel d’accéder à
des Instruments “de haute couture”, dont
vous ne pourrez plus vous passer
Pour informations et commandes
FRANCE
Chauvin-Arnoux
190, rue Championnet
75876 PARIS Cedex 18
Tél : (33) 01 44 85 44 58
Fax : (33) 01 46 27 07 48
info@metrix.fr
www.metrix.fr
SUISSE
Chauvin Arnoux AG
Einsiedlerstraße 535 -
8810 HORGEN
Tél : 01/727 75 55
Fax : 01/727 75 56
info@chauvin-arnoux.ch
www.chauvin-arnoux.ch
MOYEN-ORIENT
Chauvin Arnoux Middle East
Ain El Zalka, Immeuble Zalka 686
ZALKA (Beyrouth)
Tél : +961 1 890 425
Fax : +961 1 890 424
camie@chauvin-arnoux.com
www.chauvin-arnoux.com
Cube 3D Printer
2nd generation
®
User Guide
See inside for use and safety information.
Table of Contents
Introduction 2
Important safety information
EN 3
DE 4
ES 5
FR 6
IT 7
DU 8
DA 9
JP . 10
Cube 3D Printer features . 11
MTX 3250
Mull tt iimètt rre de Tablle 50 000 ptts
mull tt ii ffonctt iion
Multimètre-Analyseur MTX : la précision et le contrôle... !
• Très vaste affichage triple 50 000 pts pour une meilleure efficacité
• Précision de base 0,08%, bande-passante 100 kHz
• 3 bornes de mesure et «AUTORANGING» complet en courant
• Fonction « SPEC » permettant de visualiser directement les incertitudes
• Avec le mode « AUTOPEAK » plus de limitation du facteur de crête
• Mesures fréquentielles jusqu’à 1 MHz, de la période et du rapport cyclique
• Mesures de température à partir de sondes Pt 100 / Pt 1000
• Mode «MATH» pour une lecture directe (conversion & unité physique)
• Un modèle programmable via RS232 selon protocole SCPI
• Un modèle « Acquisition de données » avec enregistreur horodaté
MTX Compactt
Caractéristiques techniques MTX 3250
Tensions DC, AC et AC+DC
Gammes 500 mV 5 V 50 V 500 V 600 V
Résolution 10 μV 100 μV 1 mV 10 mV 100 mV
Précision DC 0,08% L+3D 0,08% L+3D 0,08% L+3D 0,1% L+3D 0,1% L+3D
Bande passante AC / AC+DC 40 Hz à 100 kHz/DC à 100 kHz
Précision de base AC et AC+DC 0,5% L+30D (0,5% L+40D/gamme 500 mV) pour DC à 1 kHz
Impédance d’entrée 10 MΩ/1 GΩ 11 MΩ 10 MΩ 10 MΩ 10 MΩ
Protection / Surcharge admissible Protection réarmable 600 Vrms permanents/1000 VDC ou 700 VAC (1min max.)
Courants DC, AC et AC+DC
Gammes 500 μA 5 mA 50 mA 500 mA 10A
Résolution 10 nA 100 nA 1 μA 10 μA 1mA
Précision DC 0,2% L+5D 0,2% L+3D 0,2% L+3D 0,2% L+5D 0,5% L+5D
Bande passante AC / AC+DC 40 Hz à 10 kHz/DC à 10 kHz
Précision de base AC et AC+DC 0,5% L+30D (2,5% L+30D/gamme 10A) pour DC à 1 kHz
Protection / Surcharge admissible Fusible HPC 10 A, 600 V/50 kA/20 ADC ou 20 Arms (30 s max.)
Mesures Fréquentielles
Fréquence (tension ou courant) Plage de mesure 1 Hz à 1 MHz - 7 gammes de 5,0000 Hz à 1,0000 MHz - Précision 0,03% L+2D jusqu’à 50 kHz
Rapport Cyclique Résolution 0,01% - Période du signal de 10 μs à 0,8 s
Résistances & Continuité
Gammes 500 Ω 5 kΩ 50 kΩ 500 kΩ 5 MΩ 50 MΩ
Résolution 10 mΩ 100 mΩ 1 Ω 10 Ω 100 Ω 1 kΩ
Précision de base 0,1% L+5 D 0,1% L+3 D 0,1% L+3 D 0,1% L+3 D 0,5% L+3 D 1% L+5 D
Protection Protection réarmable 600 Vrms
Détection en continuité sonore Gamme 500 Ω - Seuil 10 Ω à 15 Ω - Temps de réponse 1ms
Test de diode
Mesures de tension de diode De 0 à 4,5 V - Précision 0,2% L+3D - courant de mesure 1 mA env. - Protection réarmable 600 Vrms
Capacités
Performances 7 Gammes de 50,00 nF à 50,00 mF - Précision de base 1%L+ 3D - Temps de mesure 1 s jusqu’à 50 μF
Protection Protection réarmable 600 Vrms
Température ( sondes Pt 100 / Pt 1000 )
Performances Plage de mesure –125,0 °C à +800,0 °C - Précision 0,5 °C (de –125 °C à 75 °C) - Protection réarmable 600 Vrms
Autres Mesures
Capture de pics rapides >500 μs Valide sur toutes les gammes - Erreur additionnelle en Tension 3% L+10D, en Courant 4% L+10D
Mesure en dBm Résolution 0,01 dBm - Référence ajustable de 1 Ω à 9999 Ω - Protection réarmable 600 Vrms
Puissance Résistive U2/R ou R I2 Résolution 100 μW - Référence ajustable de 1 Ω à 9999 Ω - Protection réarmable 600 Vrms
Autres fonctions
Fonction AUTOPEAK Surveillance permanente du facteur de crête (FC) et gestion automatique des gammes pour respecter le FC spécifié
Fonction SPEC Calcul de la tolérance de mesure sous forme x% L+x D ou Min et Max sur les afficheurs 2 et 3
Fonction HOLD & AUTOHOLD Maintien manuel de l’affichage (HOLD) ou automatique sur mesure stable (AUTOHOLD)
Fonction REL / fonction dB Mode triple affichage : valeur relative, valeur absolue, écart en % / écart en dB
Fonction SURV Surveillance et mémorisation automatique des «MIN» et «MAX» avec horodatage des événements
Fonction MATH Mise à l’échelle et à l’unité pour les grandeurs physiques (fonction y = Ax+B et unité définissables)
Fonction STORE Acquisition de données (jusqu’à 3 mesures à la fois) - Cadence 0,5 s à 10 h - 10 mémoires & env. 1500 mesures
Fonction PRINT Envoi direct sur imprimante série des mesures horodatées - Cadence 0,5 s à 10 h
* choix de l’utilisateur à la mise sous tension
Caractéristiques générales MTX 3250
Affichage Affichage triple 50 000 points - Dimensions utiles : 130 x 50 mm - Hauteur des chiffres 20 mm et 10 mm
Cadence de Mesure 2 mesures par seconde (mode 50 000 pts)
Rétroéclairage Affichage positif rétroéclairé par matrice de LED’s - Contraste réglable
Température Référence 23°C ±5° (après 1 heure de chauffe) - Fonctionnement 0°C à +45°C - Stockage -20°C à +70°C
Humidité Relative < 80 % à 40°C
CEM / Sécurité Emission et immunité selon NF EN 61326-1, 1998 / IEC 61010, 2001
Alimentation Secteur 230 V ±10% ou 110 V ±10% (50 Hz – 60 Hz) - Cat.II/300V
Alimentation Batterie (Option) Accumulateur 9,6 V - Autonomie 10 à 12 H environ (suivant les fonctions)
Interface RS232 Optique (Option) Trame : 8 bits de données, 1 bit de stop, pas de parité - Vitesses sélectionnables 9600 & 57600 bauds
Boîtier ABS V0 - Dimensions H/L/P : 170 x 270 x 195 mm - Masse : 2,8 kg - Indice de protection IP30
Caractéristiques sous réserve de modifications liées à l’évolution de la technologie
190, rue Championnet
75876 PARIS cedex 18
Tél. : 01 44 85 44 58
Fax : 01 46 27 07 48
Filiale suisse :
Einsiedlerstrasse 535
8810 HORGEN
Tél. : 01 / 727 75 55 - Fax : 01 / 727 75 56
Agences :
Lille 03 20 55 96 41
Lyon 04 72 65 77 60
Nancy 03 83 92 19 21
Nantes 02 40 84 01 16
Paris 01 44 85 45 70
Rennes 02 99 22 80 80
Toulouse 05 62 74 50 30
Code : 906210045 - Ed.1-03/02
MTX 3250 : Multimètre 50 000 pts multifonction
Pour commander :
Etat de livraison :
1multimètre de table MTX 3250, 1 câble d’alimentation
secteur, 1 jeu de cordons de mesure et 1 notice de
fonctionnement.
MTX3250 : Multimètre de table 50 000 pts MTX 3250
MTX3250-P : Multimètre de table 50 000 pts MTX 3250
+ RS232
MTX3250-A : Multimètre de table 50 000 pts MTX 3250
+ acquisitionAt a glance . . . . . . . . . . . . . . . . . . . . . . . . . . . . . . . . . . . . . . . . . . . . . . . . . . . . . . . . . . . . . . . . . . . . . . . . . . . . . . . . . . . . . . . 12
Requirements for your Cube . 13
Unpacking and setting up your Cube 14
Link your Cube to your Cubify account 15
Unlock your Cube 16
Download and install Cube Software for Windows 16
Download and install Cube Software for Mac OSX . 17
Download your free creations 17
Cubify Software overview 18
WI-FI set-up . 20
Non-wireless computer set-up (without WI-FI options) 20
Update Cube firmware . . . . . . . . . . . . . . . . . . . . . . . . . . . . . . . . . . . . . . . . . . . . . . . . . . . . . . . . . . . . . . . . . . . . . . . . . . . . . . 20
Setting Print Jet gap 21
Material Cartridge installation 22
Printing preparation . 23
Printing your first creation 24-25
Replacing Material Cartridge . 26
Cloud printing from Cubify.com . 27
Finishing your creation 27
Maintaining your Cube . . . . . . . . . . . . . . . . . . . . . . . . . . . . . . . . . . . . . . . . . . . . . . . . . . . . . . . . . . . . . . . . . . . . . . . . . . . . . . 28
Print Pad leveling instructions . 29
Thank you for purchasing the “Cube®” 3D Printer. This printer is portable with a plug and print design that enables everybody in the family to express their creativity like never before. With ten different material colors to choose from, enjoy the freedom to print in your true colors or to mix it up.Cube 3D Printers ready-to-print technology provides a new dimension to your imagination and helps you share your creations with others in the Cubify community at Cubify.com.
At Cubify.com you can:
• Upload your creations for sale
• Purchase creations from others
• Get your creations 3D printed and shipped to you
• Buy the Cube 3D Printer and Cube Cartridges
• Engage with other creative partners
COPYRIGHT INFORMATION
© 2013 by 3D Systems, Inc. All rights reserved. This document is subject to change without notice. This document is copyrighted and contains proprietary information that is the property of 3D Systems, Inc. Cubify, Cube, and the 3D Systems logo are registered trademarks of 3D Systems, Inc. Use of the Cubify.com website constitutes acceptance of its Terms of Service and Privacy Policy.
FCC NOTICE
This equipment has been tested and found to comply with the limits for a class “B” digital device, pursuant to Part 15 of the FCC Rules. These limits are designed to provide reasonable protection against harmful interference. This equipment generates, uses, and can radiate radio frequency energy and, if not installed and used in accordance with the instruction manual, may cause harmful interference to radio communications. Operation of this equipment in a residential area is likely to cause harmful interference in which case the user will be required to correct the interference at their expense.
WARRANTY
3D Systems warrants that the Cube 3D Printer will be free from defects in materials and workmanship, during the applicable warranty period, when used under the normal conditions described in the documentation provided to you, including this user’s guide. 3D Systems will promptly repair or replace the Cube 3D Printer, if required, to make it free of defects during the warranty period. This warranty excludes (i) normal consumable or expendable parts (such as Material Cartridges, Print Pads, and CubeStick), (ii) repairs required during the warranty period because of abnormal use or conditions (such as riots, floods, misuse, neglect or improper service by anyone except 3D Systems or its authorized service provider), and (iii) repairs required during the warranty period because of the use of non-integrated, non-approved or non-licensed materials in the Cube 3D Printer. The warranty period for the Cube 3D Printer is ninety (90) days and shall start on the date your Cube 3D printer is activated. For consumers who are covered by consumer protection laws or regulations in their country of residence, the benefits conferred by our ninety (90) day warranty are in addition to, and operate concurrently with, all rights and remedies conveyed by such consumers protection laws and regulations, including but not limited to these additional rights.
THIS WARRANTY IS THE ONLY WARRANTY PROVIDED FOR THE CUBE 3D PRINTER. TO THE MAXIMUM EXTENT PERMITTED BY LAW, 3D SYSTEMS EXPRESSLY DISCLAIMS ALL OTHER WARRANTIES FOR THE CUBE 3D PRINTER AND EACH OF ITS COMPONENTS, WHETHER THOSE WARRANTIES ARE EXPRESS, IMPLIED OR STATUTORY INCLUDING WARRANTIES OF MERCHANTABILITY AND FITNESS FOR INTENDED OR PARTICULAR PURPOSES.
LIMITATION OF LIABILITY
3D SYSTEMS WILL NOT BE RESPONSIBLE FOR CONSEQUENTIAL, EXEMPLARY OR INCIDENTAL DAMAGES (SUCH AS LOSS OF PROFIT OR EMPLOYEE’S TIME) REGARDLESS OF THE REASON. IN NO EVENT SHALL THE LIABILITY AND/OR OBLIGATIONS OF 3D SYSTEMS ARISING OUT OF THE PURCHASE, LEASE, LICENSE AND/OR USE OF THE EQUIPMENT BY YOU OR OTHERS EXCEED THE PURCHASE PRICE OF THE CUBE 3D PRINTER.
2
1
INTRODUCTION
SAFETY SYMBOLS AND DEFINITIONS
SAFETY GUIDELINES
• Follow all safety rules in this section and observe all cautions and warnings in this guide.
• Do not modify any safety features or make modifications to the Cube. Doing so is prohibited and voids warranty.
• Use of print materials, or 3D prints other than 3D Systems print materials and genuine 3D Systems components may void warranty.
• Adult supervision is required; observe children closely and intervene as necessary to prevent potential safety problems and ensure the Cube’s appropriate use. Ensure small 3D prints are not accessible to young children. These 3D prints are potential choking hazards for young children.
• When the Cube is operating, the print tip on the Print Jet becomes hot; avoid touching this area until it has cooled down.
• Do not change color of material during printing; doing so may damage the Cube.
!
Hot Surface Hazard: A hot surface is accessible in the vicinity of this sign or at the Print Jet; avoid contact. Hot surfaces can cause severe burns.
Caution: Indicates something may happen that could cause loss of data, damage to equipment, or could cause personal injury.
Caution: Indicates a pinch point hazard that could cause person injury.
3
2
IMPORTANT SAFETY INFORMATION (EN)
SICHERHEITSSYMBOLE UND DEFINITIONEN
SICHERHEITSHINWEISE
• Befolgen Sie alle Sicherheitsvorschriften in diesem Abschnitt und beachten Sie alle Vorsichtsmaßnahmen und Warnhinweise in diesem Handbuch.
• Modifizieren Sie keine Sicherheitsmerkmale und nehmen Sie keine Änderungen am Cube-Drucker vor. Dies ist verboten und kann zum Erlöschen der Gewährleistung führen.
• Die Verwendung von Druckmaterialien oder 3D-Drucken, die keine Originalteile von 3D Systems sind, kann zum Erlöschen der Gewährleistung führen.
• Die Beaufsichtigung durch Erwachsene ist erforderlich; beobachten Sie Kinder genau und greifen Sie gegebenenfalls ein, um mögliche Sicherheitsprobleme zu vermeiden und um sicherzustellen, dass der Cube-Drucker ordnungsgemäß verwendet wird. Stellen Sie sicher, dass kleine 3D-Drucke für kleine Kinder nicht zugänglich sind. Diese 3D-Drucke bergen eine mögliche Erstickungsgefahr für kleine Kinder.
• Wenn der Cube-Drucker in Betrieb ist, wird die Druckdüse am Print Jet heiß; vermeiden Sie es, diesen Bereich zu berühren, bis er abgekühlt ist.
• Verändern Sie während des Druckens nicht die Farbe des Materials; dadurch kann der Cube-Drucker beschädigt werden.
!
Gefahr durch heiße Oberflächen: Eine heiße Oberfläche ist in der Nähe dieses Zeichens oder am Print Jet zugänglich; vermeiden Sie jeglichen Kontakt. Heiße Oberflächen können schwere Verbrennungen verursachen.
Achtung: Weist darauf hin, dass etwas passieren kann, was zum Verlust von Daten, zu Schäden an den Geräten oder zu Körperverletzungen führen könnte.
Achtung: Weist auf eine Einklemmgefahr hin, die zu Körperverletzungen führen könnte.
4
2A
WICHTIGE SICHERHEITSINFORMATIONEN (DE)
SÍMBOLOS Y DEFINICIONES DE SEGURIDAD
PAUTAS DE SEGURIDAD
• Siga todas las normas de seguridad de esta sección y esté atento a todas las precauciones y advertencias en esta guía.
• No modifique ninguna medida de seguridad ni realice modificaciones a la impresora Cube. Hacerlo está prohibido y anula la garantía.
• El uso de materiales de impresión o de piezas impresas en 3D que no sean componentes auténticos de 3D Systems puede anular la garantía.
• Se requiere la supervisión de un adulto; observe a los niños de cerca e intervenga cada vez que sea necesario para prevenir cualquier posible problema de seguridad y asegurar el uso adecuado de la impresora Cube. Asegúrese de que las piezas pequeñas impresas en 3D no estén al alcance de niños pequeños. Estas piezas impresas en 3D representan un posible peligro de asfixia para los niños pequeños.
• Cuando la impresora Cube está funcionando, la punta de impresión se calienta; evite tocar esta área hasta que se haya enfriado.
• No cambie el color de los materiales durante la impresión; esto puede dañar la impresora Cube.
!
Peligro de superficie caliente: Una superficie caliente se encuentra cerca de esta señal o en la impresora; evite el contacto con esta. Las superficies calientes pueden causar quemaduras graves.
Precaución: Indica que algo puede ocurrir y causar una pérdida de datos, daños al equipo o lesiones.
Precaución: Indica un peligro de punto de pellizco que podría causar lesiones a una persona.
5
2B
INFORMACIÓN IMPORTANTE SOBRE SEGURIDAD (ES)
SYMBOLES DE SÉCURITÉ ET DÉFINITIONS
CONSIGNES DE SÉCURITÉ
• Suivez toutes les consignes de sécurité de cette section et respectez tous les messages d’avertissement du présent manuel.
• Ne pas modifier les dispositifs de sécurité ou apporter des modifications au Cube. Cela est interdit et annule la garantie.
• L’utilisation de matériaux d’impression ou d’imprimés en 3D autres que les composants 3D Systems d’origine peut entraîner l’annulation de la garantie.
• La supervision d’un adulte est requise ; surveillez les enfants en restant à proximité et intervenez si nécessaire pour éviter tout problème de sécurité potentiel et garantir la bonne utilisation du Cube. Assurez-vous que les petits imprimés en 3D restent hors de portée des jeunes enfants. Ces imprimés en 3D présentent un risque d’étouffement pour les jeunes enfants.
• Lorsque le Cube fonctionne, l’extrémité de l’imprimante peut chauffer ; évitez de toucher cette zone jusqu’à ce qu’elle est refroidie.
• Ne pas modifier la couleur du matériau pendant l’impression ; cela peut endommager le Cube.
!
Surface chaude: Une surface chaude est présente à proximité de ce symbole ou sur l’imprimante ; évitez de la toucher. Les surfaces chaudes peuvent causer de graves brûlures.
Attention: Indique que quelque chose pourrait occasionner une perte des données, des dommages sur l’équipement ou des blessures.
Attention: Indique un risque de pincement qui pourrait occasionner une blessure.
6
2C
INFORMATIONS IMPORTANTES RELATIVES À LA SÉCURITÉ (FR)
SIMBOLI E DEFINIZIONI DI SICUREZZA
LINEE GUIDA DI SICUREZZA
• Attenersi a tutte le norme di sicurezza contenute in questa sezione e rispettare le avvertenze e le precauzioni indicate nella guida.
• Non alterare le caratteristiche di sicurezza né apportare modifiche a Cube. Queste operazioni sono vietate e determinano l’annullamento della garanzia.
• L’utilizzo di materiali di stampa o di componenti di stampa 3D non originali di 3D Systems può comportare l’annullamento della garanzia.
• È richiesta la supervisione di un adulto; tenere sotto stretta sorveglianza i bambini e, in caso di necessità, intervenire per evitare eventuali problemi di sicurezza e per garantire l’uso appropriato di Cube. Assicurarsi che i bambini non possano raggiungere i componenti più piccoli delle stampe 3D, poiché questi potrebbero costituire un pericolo di soffocamento.
• Durante l’attività di Cube, la puntina di stampa del getto d’inchiostro si riscalda; evitare il contatto con quest’area fino al completo raffreddamento.
• Non modificare il colore del materiale durante la stampa; quest’operazione potrebbe causare danni a Cube.
!
Pericolo di superficie calda: In prossimità di questo simbolo o del getto d’inchiostro è presente una superficie calda; evitare il contatto. Le superfici calde possono causare ustioni gravi.
Attenzione: Segnala che un determinato evento potrebbe causare la perdita di dati, danni all’impianto o lesioni personali.
Attenzione: Segnala la presenza di una zona ad alto rischio che potrebbe causare lesioni personali.
7
2D
INFORMAZIONI IMPORTANTI SULLA SICUREZZA (IT)
VEILIGHEIDSSYMBOLEN EN DEFINITIES
VEILIGHEIDSVOORSCHRIFTEN
• Volg alle veiligheidsvoorschriften in dit gedeelte en neem alle opmerkingen en waarschuwingen in deze handleiding in acht.
• Breng geen wijzigingen aan de veiligheidsvoorzieningen of de Cube aan. Dit is verboden en de garantie komt hierdoor te vervallen.
• Door gebruik van printmaterialen of 3D-prints anders dan originele 3D Systems-onderdelen kan de garantie komen te vervallen.
• Oezicht door een volwassene is vereist; houd kinderen nauwlettend in het oog en grijp zo nodig in om mogelijke veiligheidsproblemen te voorkomen en het juiste gebruik van de Cube te verzekeren. Zorg dat jonge kinderen niet bij kleine 3D-prints kunnen komen. Deze 3D-prints houden potentieel verstikkingsgevaar in voor jonge kinderen.
• Wanneer de Cube in werking is, wordt de printtip op de Print Jet heet. Raak dit oppervlak niet aan totdat het is afgekoeld.
• Wijzig de kleur van het materiaal niet tijdens het printen, anders kan de Cube worden beschadigd.
!
Gevaar voor hete oppervlakken: Er is een toegankelijk heet oppervlak in de nabijheid van dit waarschuwingsteken of bij de Print Jet. Niet aanraken. Hete oppervlakken kunnen ernstige brandwonden veroorzaken.
Let op: Duidt op iets wat kan gebeuren dat verlies van gegevens, beschadiging van de apparatuur of lichamelijk letsel kan veroorzaken.
Let op: Duidt op een gevaarlijk knelpunt dat lichamelijk letsel kan veroorzaken.
8
2E
BELANGRIJKE VEILIGHEIDSINFORMATIE (DU)
SIKKERHEDSSYMBOLER OG -DEFINITIONER
SIKKERHEDSRETNINGSLINIER
• Følg alle sikkerhedsreglerne i denne sektion og overhold forsigtighedsregler og advarsler i denne vejledning.
• Sikkerhedsfeatures må ikke modificeres og der må ikke foretages andre modifikationer til Cube. Det er forbudt at gøre det og det vil ugyldiggøre garantien.
• Brugen af andre printmaterialer eller 3D prints end de ægte 3D Systems komponenter kan ugyldiggøre garantien.
• Det er påkrævet at en voksen holder opsyn; hold nøje øje med børn og træd ind hvis det er nødvendigt for at undgå potentielle sikkerhedsproblemer og at sikre at Cube bruges rigtigt. Sørg for at små børn ikke har adgang til små 3D prints. Disse 3D prints kan udgøre en kvælningsfare for små børn.
• Når Cube er i drift bliver spidsen på printaggregatet varm, så dette område må ikke røres før det er kølet af.
• Materialets farve må ikke ændres under trykning; det kan beskadige Cube.
!
Fare for varm overflade: Der findes en varm overflade i nærheden af dette skilt eller ved printaggregatet; undgå kontakt. Varme overflader kan forårsage alvorlige forbrændinger.
Forsigtig: Viser at der kunne ske noget som kan resultere i datatab, beskadigelse af udstyr eller personskade.
Forsigtig: Viser at der findes en knusefare, som kan forårsage personskade.
9
2F
VIGTIG SIKKERHEDSINFORMATION (DA)
安全関連シンボルと定義
SIK 安全ガイドライン
• 本項の安全ルールのすべてに従い、また本書のすべての要注意および警告事項を守ってください。
• 安全機能を修正したり、Cube に改変を加えたりしないでください。そうすることは禁じられており、保証は無効になります。
• 純正 3D Systems コンポーネント以外のプリント材料や 3D プリントの使用は、保証を無効にする場合があります。
• 大人の監視が必要です。子供が使用している時には身近で見守り、安全上の問題を未然に防ぎ、Cube が適切に使用されるよう、必要に応じて介入するようにしてください。小型の 3D プリントが幼児の手に届くことのないようにしてください。これらの 3D プリントは幼児にとっては、のどを詰まらせる危険物となります。
• Cube が動作中は、プリントジェット上のプリントチップが熱くなります。その部分が冷めるまでは、触れないようにしてください。
• プリント中に材料の色を変更しないでください。そうすると Cube を損傷させることがあります。
!
高熱面の危険: このシンボルサインの近くまたはプリントジェット部には表面が高熱となっている部分があります。触れないように注意してください。高熱面で火傷することになります。
要注意: データの喪失、機器の損傷、または人身傷害を引き起こすような何かが起こる場合もあることを示します。
要注意: 人身傷害を引き起こす可能性のある危険個所を示します。
10
2G
安全に関する重要情報 (JP)
The Print Jet print tip heats the material and produces a thin flowing material of plastic creating layers that adhere to the Print Pad. After each layer is produced, the Print Pad lowers so that a new layer can be drawn on top of the last. This process continues until the last layer on the top of the creation is jetted.
CUBE 3D PRINTER FEATURES
• Material Cartridge
• Durable, ABS & PLA Plastic
• 25 free 3D print creations
• USB & WI-FI connectivity
CUBE 3D PRINTER PROPERTIES
Technology: Plastic Jet Printing (PJP)
Print Jets: Single jet
Max. Creation Size: 5.5” x 5.5” x 5.5” (140 x 140 x 140 mm)
Material: Tough recyclable plastic
Layer Thickness: 10 mil | 0.01 inches 250 microns | 0.25 mm
Supports: Fully automated; easy to peel off
Cartridge: 1 Cartridge prints 13 to 14 mid-sized creations
Material Colors: See Cubify.com for color choices
11
3
CUBE 3D PRINTER FEATURES
A
Cube Tube
B
Print Jet
C
Print Pad
D
Operator’s Touchscreen
F
Material Cartridge
G
Fuse
H
Power Cord Connection
I
USB Port*
J
USB Connection**
E
ON/OFF Push-Button
Power Switch & Menu Function
RIGHT SIDE
*For firmware update only.
**For loading Cube build files only.
A
B
C
D
E
F
BACK
G
H
I
J
12
4
AT A GLANCE
WEIGHT & DIMENSIONS
• Weight (without cartridge): 4.3 kg (9.5 lbs.)
SOFTWARE
• Complimentary software for Windows and Mac OSX. This application converts your 3D model into layered slices (G-code), ready for printing on your machine.
MINIMUM HARDWARE REQUIREMENTS
• A PC with these minimum requirements will be required to run the Cubify Software
• Processor: Multi-core processor - 2 GHz or faster per core
• System RAM: 2 GB
• Screen Resolution: 1024x768
WINDOWS REQUIREMENTS
• Cubify Software runs on 32 and 64-bit Operating Systems
• Windows XP Professional or Home Edition with Service Pack 3
• Windows 7
• Windows is required for ad-hoc WiFi Print Job submission. NOTE: Ad-Hoc WI-FI connection will not connect to Windows XP operating systems
• If not already installed, the Cubify Software installer will automatically install the Microsoft .NET 4.0 Framework
WIRELESS OPTIONS
• 802.11b/g with: WLAN Infrastructure or Ad hoc Mode.
NON-WIRELESS OPTION
• USB Memory Stick, to transfer print files (supplied with the Cube)
MAC OSX REQUIREMENTS
• Cubify Software runs on Mac OSX 10.8
ELECTRICAL REQUIREMENTS
• Outlet requirements: 100-240 Volts, at 50/60 Hz.
• Cube electrical rating: 24V DC, 3.75 amp.
MATERIAL STORAGE
• All polymers degrade with time. The following conditions ensure the material remains high quality:
• Do not unpack until material is needed.
• Store at room temperature: 16-29° C (60 - 85° F)
OPERATING ENVIRONMENT
• Room Temperature: 16-29° C (60 - 85° F)
• Nozzle- 280°C (536°F)
• Print Pad- 66-77° C (150-170°F)
13
11.25” (28.6 cm)
8” (20.3 cm)
8” (20.3 cm)
8” (20.3 cm)
8” (20.3 cm)
10” (25.4 cm)
13” (33 cm)
5
REQUIREMENTS FOR YOUR CUBE
WALL
Print Pad
USB Cable**
Cube Tube
USB Stick
Quick Start Guide
CubeStick™
Unclog Tool***
The Cube
Power Supply
Material Cartridge*
Power Cord
cubify.com
*Neon green included **For downloading firmware ***For unclogging filament in Print Jet
SETTING UP YOUR CUBE
1. Remove the white plastic inserts from each side of the box by pinching the tabs in and bending the inserts out. Pull to remove both from the box. Lift top of carton up and remove.
2. Lift foam from the top of Cube. Remove foam from the midsection and below the plate by gently pulling out from the front. Remove Cube from box.
3. Lift foam that Cube was sitting on to access power supply and other accessories.
4. Remove the Print Pad from the foam under the Cube and set it aside.
5. Plug round connector of power supply into the back of the Cube.
6. Plug power cord into power supply, then into outlet or power strip.
14
6
UNPACKING AND SETTING UP YOUR CUBE
Creating an account on Cubify.com is easy and gives you access to all of the great designs and collections that will drive and inspire your creativity. Once you have set up an account you will be able to activate your Cube by entering your Cube’s individual serial number in the designated bar under the Activate My Cube tab. An activation code will then be sent to the email account you used to register. This code will be used to unlock your Cube so that you can get your 3D printing underway.
NOTE: If you do not receive an activation code email, please check your spam filter settings.
1. Go to Cubify.com and log in to your account or click Sign Up to create an account.
2. Click on Activate My Cube from My Cubify drop down menu.
3. Enter your 12-character serial number (digits and letters found on the back of your Cube), then click Activate.
4. Your Cube’s serial number is now activated on Cubify.com and your Cube will be assigned to your Cubify account. Your activation code will be displayed, and also sent to you in an e-mail along with links to help you get started creating. Make sure the email doesn’t get caught in your spam filter!
5. Using the links from your activation code email, or the My Cubes section in your account, you can download:
• The Cube Software, which turns your 3D models into a file that the Cube can print. The software must be installed on your computer. Your Cube won’t print without it!
• 25 free, ready-to-print 3D files created by top artists and designers (also on your USB stick)
• Cube 3D Printer User Guide (also on your USB stick)
• Material Safety Data Sheets
6. Now it is time to unlock your Cube so you can begin creating.
NOTE: If you use a PC, you need Microsoft® Windows® 7 or XP (SP3+). If you use a Mac, you need Mac OSX 10.8+
15
7
LINK YOUR CUBE TO YOUR CUBIFY ACCOUNT
Once you’ve linked your Cube to your Cubify account, you can activate your Cube to start printing!
1. Your activation code to unlock your Cube will appear on the “Congratulations” screen after you activate your Cube in your Cubify account. A copy of the code should also be sent to your email.
2. Make sure your Cube is plugged in. Press the button on the front panel to turn it on. It may take a few seconds for it to warm up.
3. Tap on the touchscreen to view the Unlock Cube screen.
4. Enter your activation code and then tap on the check box to unlock your Cube. Your Cube will automatically transition to the main menu touchscreen and is now unlocked and ready to create!
Download the Cube Software and experiment with it to create your own designs.
1. To download Cube Software, go to My Cubify and click on My Accounts. Click on My Downloads and select Cube software on the menu page.
2. Click on Setup.exe in the Cube Client.zip. The next screen will ask you if you would like to open this file. Click Yes.
3. The Cubify Setup Wizard will guide you through the steps to install the software on your computer. Click Next to launch the Setup Wizard.
• It is necessary to download the Cube software in order to be able to import .stl or .creation files and convert them to .cube files.
• The .cube file is a machine-specific file type that is coded for the Cube to read and print.
16
8
UNLOCK YOUR CUBE
9
DOWNLOAD AND INSTALL CUBE SOFTWARE FOR WINDOWS
Download the Cube Software and experiment with it to create your own designs.
1. In the menu, Click on Download Cube Software. Double click on the zip file and the Cubify Mac installer.dmg will appear.
2. Double click Cubify Mac Installer dmg, to make a new window open. Drag to install into the Applications folder.
3. If the “Drag to install” window did not open, locate the Cubify driver in your device panel. Click on Cubify to download software.
NOTE: Safari users will not have to unzip the dmg; it unzips as it downloads.
• It is necessary to download the Cube software in order to be able to import .stl or .creation files and convert them to .cube files.
• The .cube file is a machine-specific file type that is coded for the Cube to read and print.
Follow these simple steps to access your 25 free creations:
1. Click on Download Free Creations to start your immediate download.
2. Click Extract in the Creation zip file and extract it to your computer.
There are a couple of file types involved in 3D printing with your Cube:
• .stl file: A 3-dimensional solid computer-aided design (CAD) file that defines the geometry to be printed. This is the typical file type output of a 3D design program or software tool.
• .creation file: These files represent the pre-made 3D models that are optimized for your Cube and available on Cubify for download. Simply import your .creation file into the Cube software and click Build to prepare your creation for print.
• .cube file: This is the machine code read by the Cube 3D Printer that enables it to build your print.
17
10
DOWNLOAD AND INSTALL CUBE SOFTWARE FOR MAC OSX
11
DOWNLOAD YOUR FREE CREATIONS
Cubify Software allows you manipulate your design before sending it to the Cube for printing. It simulates the Cube’s Print Pad so you can orient, scale, re-size and rotate the 3D print to get the best result when printing. Become familiar with the software and understand the functions before starting your first print.
IMPORT
Select your creation file.
CENTER
Center your creation on the Print Pad; multiple items are auto-positioned.
BUILD
Creates the Cube file by adding support structures; the slicing process will prepare your creation.
PRINT
Transfers your creation to the Cube.
HEAL
Fix your creation.
ORIENT & SCALE
Rotate the X, Y, Z axis; move creation back & forth or side to side; increase or decrease your creation.
These icons permit you to view your creation in various view points. After selecting a particular view, place your cursor on the part and hold the mouse button down; rotate the Print Pad to view different angles of the part.
Isometric
Right
Left
Front
Back
Top
Bottom
COLOR*
Select your background and model color.
SAVE
Saves your creation without printing.
UNLOAD
Deletes your creation from the Cube software.
CONFIGURE
Connect your computer and the Cube via WI-FI using the USB cable. Also, download firmware upgrades.
SETTINGS
Choose your print size; turn rafts & supports ON or OFF.
MODEL INFO
Displays your creation information.
* The color you select will not affect the color of your printed creation
18
12
CUBIFY SOFTWARE OVERVIEW
SET UP A COMPUTER (AD HOC) NETWORK
An ad hoc network is a temporary connection to your Cube through your computer or wireless smart phone.
Ad hoc networks can only be wireless, so you must have a wireless network adapter installed in your computer to setup or join an ad hoc network.
NOTE: Ad hoc will not connect to operating systems running Windows XP.
1. Tap on Setup in the main menu and scroll until you come to the WI-FI Setup screen.
2. Tap WI-FI Setup.
3. Tap on Ad Hoc in the menu screen. The next screen will show Ad Hoc mode enabled meaning it is ready to connect to your computer.
4. Open your Internet Access located in your Network Settings.
NOTE: Always select your Cube from your network settings before opening Cube Software.
5. Select your Cube on the menu. Click on Connect. This may take a few minutes.
7. Open your Cube software and click on “Configure” and select your Cube from the menu. Click on “Connect;” once connected, you may begin to print your creation.
19
13
WI-FI SETUP
SET UP A COMPUTER (WLAN) NETWORK
WLAN (wireless local area network) allow easy establishment of a secure wireless network.
WLAN connection will allow you to connect to your printer through the Cube Software.
1. Tap on Setup in the main menu and scroll until you come to the WI-FI Setup.
2. Tap on WI-FI Setup.
3. Tap on WLAN in the menu screen. “Acquiring networks” screen appears, meaning it is searching for available networks to connect to the printer.
4. Tap the on the touch screen to select your network. If your network is password protected, enter your password and tap on “OK.”
5. After your WI-FI is set up, if needed, the WI-FI firmware may update to the latest version if the latest firmware is not installed. NOTE: This will only occur when you are connecting to the network for the first time and if the WI-FI firmware needs updating. Once it is updated, the network will be successfully connected.
6. If the WI-FI firmware update was necessary, you may get one of two responses: (1) Network connected update successful; (2) Network connected... Update not successful. NOTE: If WiFi firmware update reported unsuccessful, the WLAN WiFi connection should still work. The WiFi firmware will try again with next connection attempt.
7. Click the function button to take you back to the main menu before printing
8. Open your Cube software and click on “Configure” and select your Cube from the menu. Click on “Connect;” once connected, you may begin to print your creation.
9. Open your Internet Access located on your computer’s taskbar.
10. Scroll and select the network on the menu. Click on Connect. This may take a few minutes.
If you are not connected to a network, you can download your creations from your computer and save them to your USB Memory Stick for printing.
• When printing with the USB Memory Stick, the file must be in the main folder located on the memory stick. If it is put into another folder it cannot be accessed from Cube screen.
• Install your USB Memory Stick into your computer’s USB connection and save your creation to your USB Memory Stick.
• When you are ready to print your creation, install your memory stick into the Cube’s USB connection and print your creations.
1. Tap on Setup on the Cube touch screen and scroll to Update Firmware.
2. If your USB Memory Stick is connected to the Cube, please remove the memory stick, (the screen will display the directions to do this). Tap the NEXT arrow on the display; locate the USB cable and connect the cable to the Cube USB port located on the back of the Cube (again, these instructions will be displayed on the screen). Connect the other end of cable to your computer’s USB port.
NOTE: Never leave the USB cable connected except when upgrading the firmware.
3. Tap the NEXT arrow. SETTINGS SAVED will be displayed at the top of the screen. You will have 6 seconds to press and hold the control button until firmware loader appears on the screen. If the button is released before the firmware screen appears, the screen will go blank. Repeat Step 1 to restart the update firmware process.
4. Continue to hold button until Settings Saved and then Cube Firmware Loader appears on the touch screen. You are now in the correct mode to update your firmware. NOTE: Even though the update is unsuccessful, WLAN WI-FI should still work, To try again, simply reconnect to the network,
5. Open Cubify Software and click the Configure icon; click on Load New Cube Firmware. Once you see the pop-up message Firmware Updated Successfully, click OK.
6. Disconnect the USB cable from your Cube and computer; unplug the Cube power cord. Plug power cord back in and press the Cube power button; a message will display on the touchscreen “Setting restored,” click on the check mark to save settings. This does not change your previous settings that you have made. Your new firmware is now installed.
14
NON-WIRELESS COMPUTER SETUP
15
UPDATE CUBE FIRMWARE
SWITCHING FROM AD-HOC TO WLAN CONNECTION (OR VICE VERSA)
1. Close out Cube Software.
2. Disconnect the Cube’s wireless connection through the Internet Access menu on your operating system.
3. Now follow steps for WLAN / ad-hoc set up.
4. Re-open your software after your Cube successfully connects to WLAN / ad-hoc.
There must be an appropriate distance between the print tip and the Print Pad to make sure the first printed layer sticks properly.
When the Cube is operating, the print tip on the Print Jet nozzle becomes hot; avoid touching this area until it has cooled down.
1. Make sure the textured side of the Print Pad is facing upwards. Place the magnetic side of the print pad onto the magnetic print plate.
2. Press Set Up on your Cube’s touchscreen and tap Next until Set Gap appears.
3. Select Set Gap. The Print Jet and Print Pad will move into position to set the gap. Place a standard piece of paper between the print tip and Print Pad. If the paper cannot slide between the tip and pad, tap the down arrow on the touchscreen until you can slide the paper between the tip and pad.
4. Press the Z up key to move the Print Pad toward the print tip until the paper is tight between the Print Pad and print tip. Tap the down key until the paper slides back and forth with no resistance.
5. Tap the check mark to save this setting to the printer’s memory. If the setting is saved, the “Gap” value will be applied for each subsequent print. If you wish to cancel this setting, tap the X box.
6. If you have any trouble printing after the gap setting has been saved, you can use this procedure at any time to change the gap.
7. Once the gap has been set, tap PREV to start the Load Cartridge process.
!
21
16
SETTING PRINT JET GAP
1. Use scissors to remove the cartridge from its packaging.
2. Tap Setup on your Cube’s touchscreen, select Load Cartridge, and press Next.
3. Remove the thumbscrew from the side of the Material Cartridge and remove the blue tape from the material. Save the screw to reinstall into cartridge if material was not fully used during printing. This will prevent filament from unravelling during storage. Press Next to begin cartridge installation.
Do not pull out plastic until the thumbscrew is removed from the cartridge. Failure to remove the thumbscrew will damage the cartridge.
7. Filament will then be drawn into the Print Jet until a small amount comes out of the heated tip at the bottom. Carefully dispose of the filament without touching the hot print tip. Press Next.
(If nothing comes out of the print tip, remove the plastic from the top of the Print Jet and repeat these installation steps.)
WARNING: PRINT TIP BECOMES EXTREMELY HOT DURING SET-UP AND OPERATION. DO NOT TOUCH PRINT TIP.
8. Insert Cube Tube (the clear tube that’s already around the filament at the top) into the Print Jet. Press Next and your cartridge is loaded and ready to go!
4. Tilt cartridge so that bottom of cartridge is resting on cartridge holder.
5. Slide cartridge in the holder ensuring that top of cartridge feeder is seated into the Cube feeder. Do not slide cartridge without tilting, doing so can cause damage to t he Cube Feeder.
6. Insert the filament coming from the tube into the hole at the top of the Print Jet. Press Next, and continue to push filament into the Print Jet until it feeds through on its own.
!
!
22
17
MATERIAL CARTRIDGE INSTALLATION
NOTE: CubeStick must be applied to the Print Pad every time you start a new creation.
CAUTION: Do not use any adhesive other than the CubeStick. Doing so can damage your print tip and the Print Pad. Make sure you use the 2nd generation CubeStick for your 2nd generation Cube.
WARNING: APPLY ADHESIVE JUST PRIOR TO STARTING A PRINT. ADHESIVE WILL DRY IF YOU WAIT TO START A PRINT AFTER APPLICATION. ADHESIVE SHOULD STILL BE WET AT START OF PRINT.
1. Find the CubeStick in the original packaging.
2. Remove Print Pad from the Cube. Apply a thin, even coat of adhesive on the entire top surface of the Print Pad. (Don’t over-apply!) Check the Print Pad after applying the adhesive to make sure there aren’t any gaps where the adhesive was not applied. If you find any gaps, apply adhesive to those areas. Never put glue on side with magnet.
3. Replace CubeStick cap after use.
4. Place Print Pad on the Print Pad platform.
!
!
23
18
PRINTING PREPARATION
NOTE: Your Cube will have printed test creations before leaving the factory. These test creations may have been printed in a different material color than you are using. Therefore, a small amount of material may be remaining in the Print Jet. The start of your first creation may have some of this material color until it transitions over to your material color.
WARNING: PRINT JET NOZZLE TIP BECOMES EXTREMELY HOT DURING SET-UP AND OPERATION. DO NOT TOUCH NOZZLE.
Do not change color of material or cartridge during printing; doing so may damage the Cube.
To get started printing your first creation; the creation files that were downloaded when you activated your account or a .stl file will need to be converted to a .Cube file. This is the machine code file that the Cube printer will read to build your creation.
WI-FI CONNECTION
if you wished to connect to WI-FI via Ad hoc or WLAN connection, please refer to Section 14: WI-FI Set-Up and follow the step by step instructions. Then resume these instructions and follow the steps below to connect to your Cube. These steps apply to both Ad Hoc and WLAN connections.
1. Open Cube Software and click on Configure. Click on your Cube model in the dialog box and click on Connect. Your computer is now connected to your Cube.
2. Click on Import and select a file from the creation files that you downloaded from Cubify.com or a .stl file. The creation that you selected will appear on Print Pad. The software’s Print Pad is a simulation of your Cube Print Pad.
3. In the software you can orient, scale, and manipulate your creation to ensure that you obtain the optimum printing results. Refer to Section 13: Cubify Software Overview to understand the different functions your software provides.
4. To prepare your creation file for printing, click on Build and save your creation as a .cube file.
PRINTING WITH WI-FI CONNECTION
1. If have a WI-FI connection, click on Print and select the Cube file saved from your creation file; click on Open to send your file to the Cube.
2. On the Cube touchscreen, finger tap check box to start the build file or tap the X box to cancel.
3. The Cube will begin the heating process; this will take a few minutes. During the heating process, the print tip and the Print Pad will reach the temperatures that have been preset by the manufacturer. Once these temperatures are reached, your creation will begin to print.
NOTE: During printing operation, please do not place a cover over your Cube. The Cube generates heat during printing and if covered, it can cause damage to the Cube.
!
24
19
PRINTING YOUR FIRST CREATION
PRINTING YOUR FIRST CREATION (continued)
PRINTING FROM THE USB MEMORY STICK
1. If you are using your USB Memory Stick to download your Cube file, please install the USB Memory Stick into the USB port on your computer. Open the file folder where your Cube file is located and save it on the USB Memory Stick. Remove memory stick and install it into the Cube’s USB port.
2. Tap Print on the touchscreen and use left or right arrows until you see your Cube file displayed.
3. Tap on your .cube file; the print touchscreen will appear showing the total time it will take to print your creation and the time left to complete it during printing. It also shows the maximum height of your creation and the current height while printing.
4. The Cube will begin the heating process that will take a few minutes. During the heating process, the print tip and the Print Pad will reach the temperatures that have been preset by the manufacturer. Once these temperatures are reached, your creation will begin to print. Once the creation is finished printing, your touch screen will display Print Finished, press the check box to confirm. Wait until the Print Pad is completely cool before removing your print.
WARNING: PRINT JET NOZZLE TIP BECOMES EXTREMELY HOT DURING SET-UP AND OPERATION. DO NOT TOUCH NOZZLE.
Do not change color of material or cartridge during printing; doing so may damage the Cube.
ABORTING YOUR PRINT
To abort your creation during warm up or printing, finger tap the STOP button on touchscreen. The next screen will read Are you sure you want to abort the print? Finger tap the check box to abort or tap the X box to cancel. Wait until the print tip completely cools before touching.
!
25
Do not change color of material or cartridge during printing; doing so may damage the Cube.
1. After being instructed by the touchscreen, pull Cube Tube away from top of Print Jet. Do not pull the material out until the touch screen instructs you to do so.
2. Pull material out of Print Jet and press Next.
NOTE: After the material is pulled out from the print jet, material debris may be visible at the print tip that could clog the tip. Using the unclog tool, insert the tool’s point into the Print Jet; if debris is present, it will protrude from the print tip.
3. Tilt cartridge down to clear Cube feeder and remove cartridge from cube; remove the Cube Tube from material. Replace thumb screw into cartridge to hold the unused material in place. Refer to Step 18: Material Cartridge Installation for instructions on how to install your new cartridge.
26
20
REPLACING MATERIAL CARTRIDGE
!
1. Click on My Cubify and select My Account.
2. Click on My Downloads.
3. If you would like to download a file to save on your computer or print using Cube software, click on DOWNLOAD. The file will download to the Cube software on your computer to save your creation.
4. Your file can download directly to your Cube by clicking on PRINT ON MY CUBE; the Select Cube Printer screen will appear and will search for available Cube printers on your network.
5. If a Cube was not discovered in your network, you can either save the .cube file to your computer or connect through an ad hoc network (refer to PRINTING WITH WI-FI CONNECTION, page 25).
6. If a Cube is discovered in your network, you have a choice of printing now or decline printing. If you select print now, your print will start printing on the Cube you selected in the network.
REMOVING YOUR CREATION FROM THE PRINT PAD
• Place the Print Pad with your creation in a container (not supplied) filled with regular warm tap water and let it soak approximately five minutes or until your creation eases free from the Print Pad. Clean and dry the Print Pad before reuse.
• To clean your Print Pad, rinse under tap water and dry with a lint-free wipe.
REMOVING RAFTS AND SUPPORTS (IF REQUIRED)
• Use small pliers to remove supports and rafts. In places where the supports are inside your creation and are hard to get to, use small wire snips (not provided) to remove the supports.
27
21
CLOUD PRINTING FORM CUBIFY.COM
22
FINISHING FIRST CREATION
CLEANING THE EXTERIOR
• Clean the Cube’s exterior with a lint free cloth and water. Dampen the cloth with water and wipe the outer surfaces of any debris that is visible.
CLEANING THE PRINT PAD
• Submerge the Print Pad in a container filled with warm tap water. Please do not use well water; it contains certain minerals that may make your creation difficult to remove from the Print Pad.
• Rinse pad under tap water and dry using a lint free wipe.
CLEANING THE PRINT TIP
• Using the small pliers, pull away any plastic debris away from the Print Jet tip (if the debris is stubborn, the Print Jet may need to be heated to make the debris soft enough to remove).
CLEANING THE TOUCHSCREEN
WARNING: PRINT JET NOZZLE TIP BECOMES EXTREMELY HOT DURING SET-UP AND OPERATION. DO NOT TOUCH NOZZLE.
• Wipe the touchscreen with the soft, lint-free cloth. Do not spray cleaners on the touchscreen.
4 AMP FUSE REPLACEMENT
CAUTION: Before replacing the fuse, switch Cube’s power off and unplug power cord.
1. 4 Amp, 250 V fast blow fuse is located on the back left side of your Cube.
2. Using a flat screwdriver, turn screwdriver counterclockwise to remove fuse from the fuse holder.
3. Using a flat screwdriver, turn screwdriver clockwise to install new fuse into the fuse holder. After new fuse is installed, plug power cord into outlet and switch power on.
!
!
28
23
MAINTAINING YOUR CUBE
Your Print Pad may become un-leveled when transporting your Cube. If this occurs, please follow these instructions to level your Print Pad for optimum creation experience. The Print Pad pad can be leveled with the two adjusting bolts located underneath the front and back of the pad.
1. Press the power button on the Cube control panel.
2. Ensure Print Pad is properly installed on print plate.
3. Finger tap SETUP on the touchscreen and scroll through the menu until LEVEL PLATE appears. Press LEVEL PLATE; the Print Pad will move up to Print Jet tip.
4. Using the Up arrow, raise the Print Pad to the Print Jet tip until the tip is near the Print Pad but not touching it.
5. Touch the “clockwise” and “counter-clockwise” buttons to automatically move the Print Jet around the four corners of the Print Pad. During each movement along the sides of the Print Pad, observe any gaps between the pad and the Print Jet. If there are gaps on one side of Print Pad and the Print Jet tip is barely touching the Print Pad on the other side, the Print Pad pad is not level.
NOTE: Please refer to the FRONT TO BACK or SIDE TO SIDE instructions on how to turn the adjusting knob bolts to level the pad properly.
6. Place a standard sheet of paper between the Print Jet tip and the Print Pad where the adjustment needs to be made. If the paper cannot slide between the Print Jet tip and Print Pad, tap the down arrow key on touchscreen.
ROLL SIDE TO SIDE
If the gap between the Print Pad and Print Jet tip is at the right or left side, adjust the pad using the rear adjusting knob bolt (B). If the gap is too low, the pad is too low and will need to be moved up.
1. If the pad needs to be moved up, turn the front adjusting knob bolt (A) clockwise to loosen and then turn the rear adjusting knob bolt (B) clockwise. To move the pad down, turn the rear adjusting knob bolt (B) counterclockwise.
2. While adjusting, slide the paper between the Print Jet tip and Print Pad where the adjustment was needed; the paper should slide back and forth with no resistance. If resistance is felt, the pad is too high. Please repeat the adjustment.
3. Once adjustments are made, tighten the adjusting knob bolts.
TILT FRONT TO BACK
If the gap between the Print Pad and Print Jet tip is at the front or back of pad, adjust the pad using the rear adjusting knob bolt (B). If the gap appears on the side, the pad is too low and will need to be moved up.
1. If the pad needs to be moved up, turn the front adjusting knob bolt (A) clockwise to loosen and then turn the back adjusting knob bolt (B) clockwise. To move the pad down, turn the rear adjusting knob bolt (B) counterclockwise.
2. While adjusting, slide the paper between the Print Jet tip and Print Pad where the adjustment was needed; the paper should slide back and forth with no resistance. If resistance is felt, the pad is too high. Please repeat the adjustment.
3. Once adjustments are made, tighten the adjusting knob bolts.
NOTE: After the print jet is adjusted, you will need to reset the print jet gap; please refer to Section 16: Setting Print Jet Gap.
A
B
29
24
PRINT PAD LEVELING INSTRUCTIONS
3D Systems, Inc.
333 Three D Systems Circle | Rock Hill, SC | 29730
Cubify.com
©2013 3D Systems, Inc. All rights reserved.
The 3D Systems logo, Cube and Cubify are registered trademarks of 3D Systems, Inc.
pn 350341-01, Rev. C
This document was generated on 01/08/2014
PLEASE CHECK WWW.MOLEX.COM FOR LATEST PART INFORMATION
Part Number: 43375-1001
Status: Active
Overview: Sabre™ Power Connector
Description: Sabre™ Crimp Terminal, Female, Double 18 AWG, 4.57mm Max. Insulation Diameter,
Reel Packaged, Tin (Sn) Plated Brass Contact with TPA
Documents:
Drawing (PDF) Product Literature (PDF)
RoHS Certificate of Compliance (PDF)
General
Product Family Crimp Terminals
Series 43375
Application Power
Comments For double crimping of 18 AWG wire in a side-by-side
orientation. Terminal mates to 3.18mm wide x 0.51mm )
thick flat blade PC tab. Allows 44441 receptacle
housings to comply with the UL1977 finger proof access
requirement
Crimp Quality Equipment Yes
MolexKits Yes
Overview Sabre™ Power Connector
Product Literature Order No 987650-5662
Product Name Sabre™
UPC 800754365994
Physical
Durability (mating cycles max) 25
Gender Female
Material - Metal Brass
Material - Plating Mating Tin
Material - Plating Termination Tin
Net Weight 0.360/g
Packaging Type Reel
Plating min - Mating 0.508μm
Plating min - Termination 0.508μm
Termination Interface: Style Crimp or Compression
Wire Insulation Diameter 4.57mm max.
Wire Size AWG 14, 16, 18+18
Wire Size mm² N/A
Electrical
Current - Maximum per Contact 18A
Voltage - Maximum 600V
Material Info
Reference - Drawing Numbers
Product Specification RPSX-44441-001
Sales Drawing SD-43375-1001
Series
image - Reference only
EU RoHS China RoHS
ELV and RoHS
Compliant
REACH SVHC
Contains SVHC: No
Low-Halogen Status
Low-Halogen
Need more information on product
environmental compliance?
Email productcompliance@molex.com
For a multiple part number RoHS Certificate of
Compliance, click here
Please visit the Contact Us section for any
non-product compliance questions.
Search Parts in this Series
43375Series
Mates With
43178 Male Crimp Terminals
Use With
44441 Receptacle Housings
Application Tooling | FAQ
Tooling specifications and manuals are
found by selecting the products below.
Crimp Height Specifications are then
contained in the Application Tooling
Specification document.
Global
Description Product #
Manual Extraction
Tool
0638130500
Terminator Die -
Doubles
0638405200
Hand Crimp Tool
for Flat Blade Crimp
Terminal
0638117300
Extraction Tool 0638132700
Mini-Mac™
Applicator
0638916100
Mini-Mac™
Applicator, For
Narrow Insulation
Crimp
0638917000
This document was generated on 01/08/2014
PLEASE CHECK WWW.MOLEX.COM FOR LATEST PART INFORMATION
This document was generated on 01/22/2014
PLEASE CHECK WWW.MOLEX.COM FOR LATEST PART INFORMATION
Part Number: 43031-0002
Status: Active
Overview: Micro-Fit 3.0™ Connectors
Description: Micro-Fit 3.0™ Crimp Terminal, Male, with Gold (Au) Plated Tin/Brass Alloy Contact,
20-24 AWG, Reel
Documents:
Drawing (PDF) RoHS Certificate of Compliance (PDF)
Product Specification PS-43045 (PDF) Product Literature (PDF)
Test Summary TS-43045-002 (PDF)
General
Product Family Crimp Terminals
Series 43031
Application Power
Crimp Quality Equipment Yes
Overview Micro-Fit 3.0™ Connectors
Packaging Alternative 43031-0008 (Loose)
Product Literature Order No 987650-5984
Product Name Micro-Fit 3.0™
UPC 800754369411
Physical
Gender Male
Material - Metal Phosphor Bronze
Material - Plating Mating Gold
Material - Plating Termination Tin
Net Weight 0.061/g
Packaging Type Reel
Plating min - Mating 0.381μm
Plating min - Termination 2.540μm
Termination Interface: Style Crimp or Compression
Wire Insulation Diameter 1.85mm max.
Wire Size AWG 20, 22, 24
Wire Size mm² N/A
Material Info
Reference - Drawing Numbers
Product Specification PS-43045, RPS-43045-003, RPS-43045-004
Sales Drawing SD-43031-****
Test Summary TS-43045-002
Series
image - Reference only
EU RoHS China RoHS
ELV and RoHS
Compliant
REACH SVHC
Contains SVHC: No
Low-Halogen Status
Low-Halogen
Need more information on product
environmental compliance?
Email productcompliance@molex.com
For a multiple part number RoHS Certificate of
Compliance, click here
Please visit the Contact Us section for any
non-product compliance questions.
Search Parts in this Series
43031Series
Mates With
43030
Application Tooling | FAQ
Tooling specifications and manuals are
found by selecting the products below.
Crimp Height Specifications are then
contained in the Application Tooling
Specification document.
Global
Description Product #
Extraction Tool 0011030043
Insertion Tool for
Crimp Terminal
0638120800
Hand Crimp Tool 0638190000
FineAdjust™
Applicator for
Insulation OD
1.30-1.85mm - 20-24
AWG
0639004500
FineAdjust™
Applicator for
0639018800
Insulation OD
1.10-1.30mm - 20-24
AWG
FineAdjust™
Applicator for
Insulation OD
0.91-1.09mm - 20-24
AWG
0639018900
T2 Terminator™
for insulation OD
1.30-1.85mm - 20-24
AWG
0639104500
T2 Terminator™
for insulation OD
1.10-1.30mm - 20-24
AWG
0639118800
T2 Terminator™
for insulation OD
0.91-1.09mm - 20-24
AWG
0639118900
This document was generated on 01/22/2014
PLEASE CHECK WWW.MOLEX.COM FOR LATEST PART INFORMATION
This document was generated on 04/14/2014
PLEASE CHECK WWW.MOLEX.COM FOR LATEST PART INFORMATION
Part Number: 39-28-8060
Status: Active
Overview: Mini-Fit Jr.™ Power Connectors
Description: Mini-Fit® Jr. Header, Dual Row, Vertical, without Snap-in Plastic Peg PCB Lock, 6
Circuits, PA Polyamide Nylon 6/6 94V-0, Tin (Sn) Plating, without Drain Holes
Documents:
3D Model Packaging Specification PK-5566-003 (PDF)
Drawing (PDF) Test Summary TS-5556-002 (PDF)
Product Specification PS-5556-001 (PDF) RoHS Certificate of Compliance (PDF)
Agency Certification
CSA LR19980
UL E29179
General
Product Family PCB Headers
Series 5566
Application Power, Wire-to-Board
Comments The 5566 header should be used with standard Mini-
Fit® female terminals. If increased amperage of up
to 13A per circuit is needed, please consider using
the Mini-Fit® Plus HCS family 45750 terminals with
46015 headers; . See Molex Product specification
PS-5666-001 for current de-rating information.
Overview Mini-Fit Jr.™ Power Connectors
Product Name Mini-Fit Jr.™
UPC 800753580732
Physical
Breakaway No
Circuits (Loaded) 6
Circuits (maximum) 6
Color - Resin Natural
Durability (mating cycles max) 30
First Mate / Last Break No
Flammability 94V-0
Glow-Wire Compliant No
Guide to Mating Part No
Keying to Mating Part None
Lock to Mating Part Yes
Material - Metal Brass
Material - Plating Mating Tin
Material - Plating Termination Tin
Material - Resin Nylon
Net Weight 1.778/g
Number of Rows 2
Orientation Vertical
PC Tail Length 3.50mm
PCB Locator Yes
PCB Retention None
PCB Thickness - Recommended 1.60mm
Packaging Type Bag
Pitch - Mating Interface 4.20mm
Pitch - Termination Interface 4.20mm
Polarized to Mating Part Yes
Series
image - Reference only
EU RoHS China RoHS
ELV and RoHS
Compliant
REACH SVHC
Contains SVHC: No
Low-Halogen Status
Low-Halogen
Need more information on product
environmental compliance?
Email productcompliance@molex.com
For a multiple part number RoHS Certificate of
Compliance, click here
Please visit the Contact Us section for any
non-product compliance questions.
Search Parts in this Series
5566Series
Mates With
5557 Mini-Fit Jr.™ Receptacle Housing
Polarized to PCB Yes
Shrouded Fully
Stackable No
Surface Mount Compatible (SMC) No
Temperature Range - Operating -40°C to +105°C
Termination Interface: Style Through Hole
Electrical
Current - Maximum per Contact 9A
Voltage - Maximum 600V
Solder Process Data
Duration at Max. Process Temperature (seconds) 5
Lead-free Process Capability Wave Capable (TH only)
Max. Cycles at Max. Process Temperature 1
Process Temperature max. C 260
Material Info
Old Part Number 5566-06A-210
Reference - Drawing Numbers
Packaging Specification PK-5566-003
Product Specification PS-5556-001, RPS-5557-036, RPS-5557-058
Sales Drawing SD-5566-002
Test Summary TS-5556-002
This document was generated on 04/14/2014
PLEASE CHECK WWW.MOLEX.COM FOR LATEST PART INFORMATION
1. General description
The UHF EPCglobal Generation 2 standard allows the commercialized provision of mass
adoption of UHF RFID technology for passive smart tags and labels. Main fields of
applications are supply chain management and logistics for worldwide use with special
consideration of European, US and Chinese frequencies to ensure that operating
distances of several meters can be realized.
The G2X is a dedicated chip for passive, intelligent tags and labels supporting the
EPCglobal Class 1 Generation 2 UHF RFID standard. It is especially suited for
applications where operating distances of several meters and high anti-collision rates are
required.
The G2X is a product out of the NXP Semiconductors UCODE product family. The entire
UCODE product family offers anti-collision and collision arbitration functionality. This
allows a reader to simultaneously operate multiple labels / tags within its antenna field.
A UCODE G2X based label/ tag requires no external power supply.
Its contact-less interface generates the power supply via the antenna circuit by
propagative energy transmission from the interrogator (reader), while the system clock is
generated by an on-chip oscillator. Data transmitted from interrogator to label/tag is
demodulated by the interface, and it also modulates the interrogator’s electromagnetic
field for data transmission from label/tag to interrogator. A label/tag can be operated
without the need for line of sight or battery, as long as it is connected to a dedicated
antenna for the targeted frequency range. When the label/tag is within the interrogator’s
operating range, the high-speed wireless interface allows data transmission in both
directions.
In addition to the EPC specifications the G2X offers an integrated EAS (Electronic Article
Surveillance) feature and read protection of the memory content. On top of the
specification of the G2XL the G2XM offers 512-bit of user memory.
SL3ICS1002/1202
UCODE G2XM and G2XL
Rev. 3.8 — 11 November 2013
139038
Product data sheet
COMPANY PUBLIC
139037 All information provided in this document is subject to legal disclaimers. © NXP B.V. 2013. All rights reserved.
Product data sheet
COMPANY PUBLIC
Rev. 3.8 — 11 November 2013
139038 2 of 48
NXP Semiconductors SL3ICS1002/1202
UCODE G2XM and G2XL
2. Features and benefits
2.1 Key features
512-bit user memory (G2XM only)
240-bit of EPC memory
64-bit tag identifier (TID) including 32-bit unique serial number
Memory read protection
EAS (Electronic Article Surveillance) command
Calibrate command
32-bit kill password to permanently disable the tag
32-bit access password to allow a transition into the secured transmission state
Broad international operating frequency: from 840 MHz to 960 MHz
Long read/write ranges due to extremely low power design
Reliable operation of multiple tags due to advanced anti-collision
Forward link: 40-160 kbit/s
Return link: 40-640 kbit/s
2.2 Key benefits
High sensitivity provides long read range
Low Q-factor for consistent performance on different materials
Improved interference suppression for reliable operation in multi-reader environment
Large input capacitance for ease of assembly and high assembly yield
Highly advanced anti-collision resulting in highest identification speed
Reliable and robust RFID technology suitable for dense reader and noisy
environments
2.3 Custom commands
EAS Alarm
Enables the UHF RFID tag to be used as EAS tag without the need for a backend data
base.
Read Protect
Protects all memory content including CRC16 from unauthorized reading.
Calibrate
Activates permanent back-scatter in order to evaluate the tag-to-reader performance.
139037 All information provided in this document is subject to legal disclaimers. © NXP B.V. 2013. All rights reserved.
Product data sheet
COMPANY PUBLIC
Rev. 3.8 — 11 November 2013
139038 3 of 48
NXP Semiconductors SL3ICS1002/1202
UCODE G2XM and G2XL
3. Applications
Supply chain management
Item level tagging
Asset management
Container identification
Pallet and case tracking
Product authentication
Outside above mentioned applications, please contact NXP Semiconductors for support.
4. Ordering information
Table 1. Ordering information G2XM
Type number Package
Name Description Version
SL3ICS1002FUG/V7AF Wafer Bumped die on sawn wafer -
SL3S1002FTB1 XSON3 plastic extremely thin small outline
package;3 terminals;
body 1 x 1.45 x 0,5 mm
SOT1122
Table 2. Ordering information G2XL
Type number Package
Name Description Version
SL3ICS1202FUG/V7AF Wafer Bumped die on sawn wafer -
SL3S1202FTB1 XSON3 plastic extremely thin small outline
package;3 terminals;
body 1 x 1.45 x 0,5 mm
SOT1122
139037 All information provided in this document is subject to legal disclaimers. © NXP B.V. 2013. All rights reserved.
Product data sheet
COMPANY PUBLIC
Rev. 3.8 — 11 November 2013
139038 4 of 48
NXP Semiconductors SL3ICS1002/1202
UCODE G2XM and G2XL
5. Block diagram
The SL3ICS1002/1202 IC consists of three major blocks:
- Analog RF Interface
- Digital Controller
- EEPROM
The analog part provides stable supply voltage and demodulates data received from the
reader for being processed by the digital part. Further, the modulation transistor of the
analog part transmits data back to the reader.
The digital section includes the state machines, processes the protocol and handles
communication with the EEPROM, which contains the EPC and the user data.
Fig 1. Block diagram of G2X IC
001aai335
MOD
DEMOD
VREG
VDD
data
in
data
out
R/W
ANALOG
RF INTERFACE
PAD
PAD
RECT
DIGITAL CONTROL
ANTENNA
ANTICOLLISION
READ/WRITE
CONTROL
ACCESS CONTROL
EEPROM INTERFACE
CONTROL
RF INTERFACE
CONTROL
EEPROM
MEMORY
SEQUENCER
CHARGE PUMP
139037 All information provided in this document is subject to legal disclaimers. © NXP B.V. 2013. All rights reserved.
Product data sheet
COMPANY PUBLIC
Rev. 3.8 — 11 November 2013
139038 5 of 48
NXP Semiconductors SL3ICS1002/1202
UCODE G2XM and G2XL
6. Wafer layout and pinning information
6.1 Wafer layout
(1) X-scribe line width: 56.4 m
(2) Y-scribe line width: 56.4 m
(3) Chip step, x-length: 488.0 m
(4) Chip step, y-length: 470,0 m
(5) Bump to bump distance X (TP1 - RFN): 351,0 m
(6) Bump to bump distance Y (RFN - RFP): 333,0 m
(7) Distance bump to metal sealring X: 40,3 m
(8) Distance bump to metal sealring Y: 40,3 m
Bump size X x Y: 60 m x 60 m
Fig 2. Wafer layout and pinning information
not to scale! 001aai346
(1)
(7)
(2)
(8)
(5)
(6) (4)
(3)
Y
X
TP2
TP1 RFN
RFP
139037 All information provided in this document is subject to legal disclaimers. © NXP B.V. 2013. All rights reserved.
Product data sheet
COMPANY PUBLIC
Rev. 3.8 — 11 November 2013
139038 6 of 48
NXP Semiconductors SL3ICS1002/1202
UCODE G2XM and G2XL
7. Package outline
Fig 3. Package outline SOT1122
Outline References
version
European
projection
Issue date
IEC JEDEC JEITA
SOT1122 MO-252
sot1122_po
Unit
mm
max
nom
min
0.50 0.04
0.55 0.425
0.30
0.25
0.22
0.35
0.30
0.27
A(1)
Dimensions
Notes
1. Dimension A is including plating thickness.
2. Can be visible in some manufacturing processes.
SOT1122
A1 D
1.50
1.45
1.40
1.05
1.00
0.95
E e e1
0.55
0.50
0.47
0.45
0.40
0.37
b b1 L L1
09-10-09
XSON3: plastic extremely thin small outline package; no leads; 3 terminals; body 1 x 1.45 x 0.5 mm
D
E
e1
e
A1
b1
L1
L
e1
0 1 2 mm
scale
3
1
2
b
4×
(2)
4×
(2)
A
pin 1 indication
type code
terminal 1
index area
139037 All information provided in this document is subject to legal disclaimers. © NXP B.V. 2013. All rights reserved.
Product data sheet
COMPANY PUBLIC
Rev. 3.8 — 11 November 2013
139038 7 of 48
NXP Semiconductors SL3ICS1002/1202
UCODE G2XM and G2XL
Table 3. Pin description of SOT1122
Symbol Pin Description
RFP 1 Ungrouded antenna connector
RFN 2 Grounded antenna connector
n.c. 3 not connected
Table 4. SOT1122 Marking
Type Type code (Marking) Comment
SL3S1202FTB1 UL UCODE G2XL
SL3S1002FTB1 UM UCODE G2XM
139037 All information provided in this document is subject to legal disclaimers. © NXP B.V. 2013. All rights reserved.
Product data sheet
COMPANY PUBLIC
Rev. 3.8 — 11 November 2013
139038 8 of 48
NXP Semiconductors SL3ICS1002/1202
UCODE G2XM and G2XL
8. Mechanical specification
8.1 Wafer specification
See Ref. 20 “Data sheet - Delivery type description – General specification for 8” wafer on
UV-tape with electronic fail die marking, BL-ID document number: 1093**”.
8.1.1 Wafer
• Designation: each wafer is scribed with batch number and
wafer number
• Diameter: 200 mm (8”)
• Thickness: 150 m ± 15 m
• Number of pads 4
• Pad location: non diagonal/ placed in chip corners
• Distance pad to pad RFN-RFP 333.0 μm
• Distance pad to pad TP1-RFN: 351.0 μm
• Process: CMOS 0.14 μm
• Batch size: 25 wafers
• Dies per wafer: 120.000
8.1.2 Wafer backside
• Material: Si
• Treatment: ground and stress release
• Roughness: Ra max. 0.5 m, Rt max. 5 m
8.1.3 Chip dimensions
• Die size without scribe: 0.414 mm x 0.432 mm = 0.178 mm2
• Scribe line width:
x-dimension:56.4 m (width is measured on top metal layer)
y-dimension: 56.4 m (width is measured on top metal layer)
8.1.4 Passivation on front
• Type Sandwich structure
• Material: PE-Nitride (on top)
• Thickness: 1.75 m total thickness of passivation
139037 All information provided in this document is subject to legal disclaimers. © NXP B.V. 2013. All rights reserved.
Product data sheet
COMPANY PUBLIC
Rev. 3.8 — 11 November 2013
139038 9 of 48
NXP Semiconductors SL3ICS1002/1202
UCODE G2XM and G2XL
8.1.5 Au bump
• Bump material: > 99.9% pure Au
• Bump hardness: 35 – 80 HV 0.005
• Bump shear strength: > 70 MPa
• Bump height: 18 m
• Bump height uniformity:
– within a die: ± 2 m
– within a wafer: ± 3 m
– wafer to wafer: ± 4 m
• Bump flatness: ± 1.5 m
• Bump size:
– RFP, RFN 60 x 60 m
– TP1, TP2 60 x 60 m
– Bump size variation: ± 5 m
• Under bump metallization: sputtered TiW
8.1.6 Fail die identification
No inkdots are applied to the wafer.
Electronic wafer mapping (SECS II format) covers the electrical test results and
additionally the results of mechanical/visual inspection.
See Ref. 20 “Data sheet - Delivery type description – General specification for 8” wafer on
UV-tape with electronic fail die marking, BL-ID document number: 1093**”
8.1.7 Map file distribution
See Ref. 20 “Data sheet - Delivery type description – General specification for 8” wafer on
UV-tape with electronic fail die marking, BL-ID document number: 1093**”
139037 All information provided in this document is subject to legal disclaimers. © NXP B.V. 2013. All rights reserved.
Product data sheet
COMPANY PUBLIC
Rev. 3.8 — 11 November 2013
139038 10 of 48
NXP Semiconductors SL3ICS1002/1202
UCODE G2XM and G2XL
9. Limiting values
[1] Stresses above those listed under Absolute Maximum Ratings may cause permanent damage to the
device. This is a stress rating only and functional operation of the device at these or any conditions other
than those described in the Operating Conditions and Electrical Characteristics section of this specification
is not implied.
[2] This product includes circuitry specifically designed for the protection of its internal devices from the
damaging effects of excessive static charge. Nonetheless, it is suggested that conventional precautions be
taken to avoid applying greater than the rated maxima.
[3] For ESD measurement, the die chip has been mounted into a CDIP20 package.
Table 5. Limiting values[1][2]
In accordance with the Absolute Maximum Rating System (IEC 60134)
Voltages are referenced to RFN
Symbol Parameter Conditions Min Max Unit
Die
Tstg storage temperature range -55 +125 C
Toper operating temperature -40 +85 C
VESD electrostatic discharge voltage Human body model [3] - 2 kV
SOT1122
Tstg storage temperature range -55 +125 C
Ptot total power dissipation - 30 mW
Toper operating temperature -40 +85 C
VESD electrostatic discharge voltage Human body model - 2 kV
139037 All information provided in this document is subject to legal disclaimers. © NXP B.V. 2013. All rights reserved.
Product data sheet
COMPANY PUBLIC
Rev. 3.8 — 11 November 2013
139038 11 of 48
NXP Semiconductors SL3ICS1002/1202
UCODE G2XM and G2XL
10. Characteristics
10.1 Wafer characteristics
[1] Power to process a Query command
[2] Measured with a 50 source impedance
[3] At minimum operating power
[4] Values measured for a 40 kHz phase reserval command under matched conditions
10.2 Package characteristics
[1] Measured with network analyzer at 915 MHz; values at 0.5 dBm after peakmax of on-set of die, measured in the center of the pads.
Table 6. Wafer characteristics
Symbol Parameter Conditions Min Typ Max Unit
Memory characteristics
tRET EEPROM data retention Tamb 55 C 50 - - year
NWE EEPROM write endurance Tamb 55 C 100000 - - cycle
Interface characteristics
Ptot total power dissipation - 30 mW
foper operating frequency 840 - 960 MHz
Pmin minimum operating power supply [1][2] - -15 - dBm
Ci input capacitance (parallel) [3] - 0.88 - pF
Q quality factor (Im (Zchip) / Re (Zchip)) [3] - 9 - -
Z impedance (915 MHz) - 22 - j195 -
- modulated jammer suppression 1.0 MHz [4] - - 4 - dB
- unmodulated jammer suppression 1.0 MHz [4] - - 4 - dB
Table 7. Package interface characteristics
Symbol Parameter Conditions Min Typ Max Unit
Interface characteristics SOT1122
Ci input capacitance (parallel) [1] - 1.02 - pF
Z SOT1122 impedance (915 MHz) - 18.6 - j171.2 -
139037 All information provided in this document is subject to legal disclaimers. © NXP B.V. 2013. All rights reserved.
Product data sheet
COMPANY PUBLIC
Rev. 3.8 — 11 November 2013
139038 12 of 48
NXP Semiconductors SL3ICS1002/1202
UCODE G2XM and G2XL
11. Packing information
11.1 Wafer
See Ref. 20 “Data sheet - Delivery type description – General specification for 8” wafer on
UV-tape with electronic fail die marking, BL-ID document number: 1093**”.
11.2 SOT1122
Part orientation T1. For details please refer to
http://www.standardics.nxp.com/packaging/packing/pdf/sot886.t1.t4.pdf.
139037 All information provided in this document is subject to legal disclaimers. © NXP B.V. 2013. All rights reserved.
Product data sheet
COMPANY PUBLIC
Rev. 3.8 — 11 November 2013
139038 13 of 48
NXP Semiconductors SL3ICS1002/1202
UCODE G2XM and G2XL
12. Functional description
12.1 Power transfer
The interrogator provides an RF field that powers the tag, equipped with a UCODE G2X.
The antenna transforms the impedance of free space to the chip input impedance in order
to get the maximum possible power for the G2X on the tag.
The RF field, which is oscillating on the operating frequency provided by the interrogator,
is rectified to provide a smoothed DC voltage to the analog and digital modules of the IC.
The antenna that is attached to the chip may use a DC connection between the two
antenna pads. Therefore the G2X also enables loop antenna design. Possible examples
of supported antenna structures can be found in the reference antenna design guide.
12.2 Data transfer
12.2.1 Reader to G2X Link
An interrogator transmits information to the UCODE G2X by modulating an RF signal in
the 840 MHz - 960 MHz frequency range. The G2X receives both information and
operating energy from this RF signal. Tags are passive, meaning that they receive all of
their operating energy from the interrogator's RF waveform.
An interrogator is using a fixed modulation and data rate for the duration of at least an
inventory round. It communicates to the G2X by modulating an RF carrier using
DSB-ASK, SSB-ASK or PR-ASK with PIE encoding.
For further details refer to Section 17, Ref. 1, section 6.3.1.2. Interrogator-to-tag (R=>T)
communications.
12.2.2 G2X to reader Link
An interrogator receives information from the UCODE G2X by transmitting a
continuous-wave RF signal to the tag; the G2X responds by modulating the reflection
coefficient of its antenna, thereby generating modulated sidebands used to backscatter an
information signal to the interrogator. The system is a reader talks first (RTF) system,
meaning that a G2X modulates its antenna reflection coefficient with an information signal
only after being directed by the interrogator.
G2X backscatter is a combination of ASK and PSK modulation depending on the tuning
and bias point. The backscattered data is either modulated with FM0 baseband or Miller
sub carrier.
For further details refer to Section 17, Ref. 1, section 6.3.1.3. tag-to-interrogator (T=>R)
communications.
139037 All information provided in this document is subject to legal disclaimers. © NXP B.V. 2013. All rights reserved.
Product data sheet
COMPANY PUBLIC
Rev. 3.8 — 11 November 2013
139038 14 of 48
NXP Semiconductors SL3ICS1002/1202
UCODE G2XM and G2XL
12.3 Operating distances
RFID tags based on the UCODE G2X silicon may achieve maximum operating distances
according the following formula:
(1)
(2)
[1] CEPT/ETSI regulations [CEPT1], [ETSI1].
[2] New CEPT/ETSI regulations. [ETSI3].
[3] FCC 47 part 15 regulation [FCC1].
[4] These read distances are maximum values for general tags and labels. Practical usable values may be
lower due to damping by object materials and environmental conditions. A special tag antenna design can
help achieve higher values.
The typical write range is > 50% of the read range.
Table 8. Symbol description
Symbol Description Unit
Ptag minimum required RF power for the tag W
Gtag gain of the tag antenna -
EIRP transmitted RF power m
wavelength m
Rmax maximum achieved operating distance for a /2-dipole m
loss factor assumed to be 0.5 considering matching and
package losses
-
R distance m
Table 9. Operating distances for UCODE G2X based tags and labels in released frequency
bands
Frequency range Region Available
power
Calculated read distance
single antenna [4]
Unit
868.4 to 868.65 MHz (UHF) Europe [1] 0.5 W ERP 3.6 m
865.5 to 867.6 MHz (UHF) Europe [2] 2 W ERP 7.1 m
902 to 928 MHz (UHF) America [3] 4 W EIRP 7.5 m
Ptag EIRP Gtag
4R
----------
2
=
Rmax
EIRP Gtag 2
42Ptag
= ---------------------------------------
139037 All information provided in this document is subject to legal disclaimers. © NXP B.V. 2013. All rights reserved.
Product data sheet
COMPANY PUBLIC
Rev. 3.8 — 11 November 2013
139038 15 of 48
NXP Semiconductors SL3ICS1002/1202
UCODE G2XM and G2XL
12.4 Air interface standards
The G2X is certified according EPCglobal 1.0.9 and fully supports all parts of the
"Specification for RFID Air Interface EPCglobal, EPCTM Radio-Frequency Identity
Protocols, Class-1 Generation-2 UHF RFID, Protocol for Communications at 860 MHz -
960 MHz, Version 1.1.0".
EPCglobal compliance and interoperability certification
139037 All information provided in this document is subject to legal disclaimers. © NXP B.V. 2013. All rights reserved.
Product data sheet
COMPANY PUBLIC
Rev. 3.8 — 11 November 2013
139038 16 of 48
NXP Semiconductors SL3ICS1002/1202
UCODE G2XM and G2XL
13. Physical layer and signaling
13.1 Reader to G2X communication
13.1.1 Physical layer
For interrogator-to-G2X link modulation refer to Section 17, Ref. 1, annex H.1 Baseband
waveforms, modulated RF, and detected waveforms.
13.1.2 Modulation
An interrogator sends information to one or more G2X by modulating an RF carrier using
double-sideband amplitude shift keying (DSB-ASK), single-sideband amplitude shift
keying (SSB-ASK) or phase-reversal amplitude shift keying (PR-ASK) using a
pulse-interval encoding (PIE) format. The G2X receives the operating energy from this
same modulated RF carrier.
Section 17, Ref. 1: Annex H, as well as chapter 6.3.1.2.2.
The G2X is capable of demodulating all three modulation types.
13.1.3 Data encoding
The R=>T link is using PIE. For the definition of the therefore relevant reference time
interval for interrogator-to-chip signaling (Tari) refer to Section 17, Ref. 1, chapter
6.3.1.2.3. The Tari is specified as the duration of a data-0.
13.1.4 Data rates
Interrogators shall communicate using Tari values between 6.25 s and 25 s, inclusive.
For interrogator compliance evaluation the preferred Tari values of 6.25 s, 12.5 s or
25 s should be used. For further details refer to Section 17, Ref. 1, chapter 6.3.1.2.4.
13.1.5 RF envelope for R=>T
A specification of the relevant RF envelope parameters can be found in Section 17,
Ref. 1, chapter 6.3.1.2.5.
13.1.6 Interrogator power-up/down waveform
For a specification of the interrogator power-up and power-down RF envelope and
waveform parameters refer to Section 17, Ref. 1, chapters 6.3.1.2.6 and 6.3.1.2.7.
13.1.7 Preamble and frame-sync
An interrogator shall begin all R=>T signaling with either a preamble or a frame-sync. A
preamble shall precede a Query command and denotes the start of an inventory round.
For a definition and explanation of the relevant R=>T preamble and frame-sync refer to
Section 17, Ref. 1, chapter 6.3.1.2.8.
139037 All information provided in this document is subject to legal disclaimers. © NXP B.V. 2013. All rights reserved.
Product data sheet
COMPANY PUBLIC
Rev. 3.8 — 11 November 2013
139038 17 of 48
NXP Semiconductors SL3ICS1002/1202
UCODE G2XM and G2XL
13.2 G2X to reader communication
An interrogator receives information from a G2X by transmitting an unmodulated RF
carrier and listening for a backscattered reply. The G2X backscatters by switching the
reflection coefficient of its antenna between two states in accordance with the data being
sent. For further details refer to Section 17, Ref. 1, chapter 6.3.1.3.
13.2.1 Modulation
The UCODE G2X communicates information by backscatter-modulating the amplitude
and/or phase of the RF carrier. Interrogators shall be capable of demodulating either
demodulation type.
13.2.2 Data encoding
The encoding format, selected in response to interrogator commands, is either FM0
baseband or Miller-modulated subaltern. The interrogator commands the encoding choice
13.2.2.1 FM0 baseband
FM0 inverts the baseband phase at every symbol boundary; a data-0 has an additional
mid-symbol phase inversion. For details on FM0 and generator state diagram, FM0
symbols and sequences and how FM0 transmissions should be terminated refer to
Section 17, Ref. 1, chapter 6.3.1.3.
13.2.2.2 FM0 Preamble
T=>R FM0 signaling begin with one of two defined preambles, depending on the value of
the TRext bit specified in the Query command that initiated the inventory round. For
further details refer to Section 17, Ref. 1, chapter 6.3.1.3.
13.2.2.3 Miller-modulated sub carrier
Baseband Miller inverts its phase between two data-0s in sequence. Baseband Miller also
places a phase inversion in the middle of a data-1 symbol. For details on Miller-modulated
sub carrier, generator state diagram, sub carrier sequences and terminating sub carrier
transmissions refer to Section 17, Ref. 1, chapter 6.3.1.3.
13.2.2.4 Miller sub carrier preamble
T=>R sub carrier signaling begins with one of the two defined preambles. The choice
depends on the value of the TRext bit specified in the Query command that initiated the
inventory round. For further details refer to Section 17, Ref. 1, chapter 6.3.1.3.
13.2.3 Data rates
The G2X IC supports tag to interrogator data rates and link frequencies as specified in
Section 17, Ref. 1, chapter 6.3.1.3.
139037 All information provided in this document is subject to legal disclaimers. © NXP B.V. 2013. All rights reserved.
Product data sheet
COMPANY PUBLIC
Rev. 3.8 — 11 November 2013
139038 18 of 48
NXP Semiconductors SL3ICS1002/1202
UCODE G2XM and G2XL
13.3 Link timing
For the interrogator interacting with a UCODE G2X equipped tag population exact link and
response timing requirements must be fulfilled, which can be found in Section 17, Ref. 1,
chapter 6.3.1.6.
13.3.1 Regeneration time
The regeneration time is the time required if a G2X is to demodulate the interrogator
signal, measured from the last falling edge of the last bit of the G2X response to the first
falling edge of the interrogator transmission. This time is referred to as T2 and can vary
between 3.0 Tpri and 20 Tpri. For a more detailed description refer to Section 17, Ref. 1,
chapter 6.3.1.6.
13.3.2 Start-up time
For a detailed description refer to Section 17, Ref. 1, chapter 6.3.1.3.4.
13.3.3 Persistence time
An interrogator chooses one of four sessions and inventories tags within that session
(denoted S0, S1, S2, and S3). The interrogator and associated UCODE G2X population
operate in one and only one session for the duration of an inventory round (defined
above). For each session, a corresponding inventoried flag is maintained. Sessions allow
tags to keep track of their inventoried status separately for each of four possible
time-interleaved inventory processes, using an independent inventoried flag for each
process. Two or more interrogators can use sessions to independently inventory a
common UCODE G2X chip population.
A session flag indicates whether a G2X may respond to an interrogator. G2X chips
maintain a separate inventoried flag for each of four sessions; each flag has symmetric A
and B values. Within any given session, interrogators typically inventory tags from A to B
followed by a re-inventory of tags from B back to A (or vice versa).
Additionally, the G2X has implemented a selected flag, SL, which an interrogator may
assert or deassert using a Select command.
For a description of Inventoried flags S0 – S3 refer to Section 17, Ref. 1 chapter 6.3.2.2
and for a description of the Selected flag refer to Section 17, Ref. 1, chapter 6.3.2.3. For
tag flags and respective persistence time refer to Section 17, Ref. 1, table 6.14.
13.4 Bit and byte ordering
The transmission order for all R=>T and T=>R communications respects the following
conventions:
• within each message, the most-significant word is transmitted first, and
• within each word, the most-significant bit (MSB) is transmitted first,
whereas one word is composed of 16 bits.
To represent memory addresses and mask lengths EBV-8 values are used. An extensible
bit vector (EBV) is a data structure with an extensible data range. For a more detailed
explanation refer to Section 17, Ref. 1, Annex A.
139037 All information provided in this document is subject to legal disclaimers. © NXP B.V. 2013. All rights reserved.
Product data sheet
COMPANY PUBLIC
Rev. 3.8 — 11 November 2013
139038 19 of 48
NXP Semiconductors SL3ICS1002/1202
UCODE G2XM and G2XL
13.5 Data integrity
The G2X ignores invalid commands. In general, "invalid" means a command that (1) is
incorrect given the current the G2X state, (2) is unsupported by the G2X, (3) has incorrect
parameters, (4) has a CRC error, (5) specifies an incorrect session, or (6) is in any other
way not recognized or not executable by the G2X. The actual definition of "invalid" is
state-specific and defined, for each G2X state, in n Section 17, Ref. 1 Annex B and
Annex C.
All UCODE G2X backscatter error codes are summarized in Section 17, Ref. 1 Error
codes, Annex I. For a detailed description of the individual backscatter error situations
which are command specific please refer to the Section 17, Ref. 1 individual command
description section 6.3.2.10.
13.6 CRC
A CRC-16 is a cyclic-redundancy check that an interrogator uses when protecting certain
R=>T commands, and the G2X uses when protecting certain backscattered T=>R
sequences. To generate a CRC-16 an interrogator or the G2X first generates the CRC-16
precursor shown in Section 17, Ref. 1 Table 6.11, then take the ones-complement of the
generated precursor to form the CRC-16. For a detailed description of the CRC-16
generation and handling rules refer to Section 17, Ref. 1, chapter 6.3.2.1.
The CRC-5 is only used to protect the Query command (out of the mandatory command
set). It is calculated out of X5 + X3 + 1. For a more detailed CRC-5 description refer to
Section 17, Ref. 1, table 6.12.
For exemplary schematic diagrams for CRC-5 and CRC-16 encoder/decoder refer to
Section 17, Ref. 1, Annex F.
For a CRC calculation example refer to Section 15.1, Table 27 and Table 28.
139037 All information provided in this document is subject to legal disclaimers. © NXP B.V. 2013. All rights reserved.
Product data sheet
COMPANY PUBLIC
Rev. 3.8 — 11 November 2013
139038 20 of 48
NXP Semiconductors SL3ICS1002/1202
UCODE G2XM and G2XL
14. TAG selection, inventory and access
This section contains all information including commands by which a reader selects,
inventories, and accesses a G2X population
An interrogator manages UCODE G2X equipped tag populations using three basic
operations. Each of these operations comprises one or more commands. The operations
are defined as follows
Select: The process by which an interrogator selects a tag population for inventory
and access. Interrogators may use one or more Select commands to select a
particular tag population prior to inventory.
Inventory: The process by which an interrogator identifies UCODE G2X equipped tags.
An interrogator begins an inventory round by transmitting a Query command
in one of four sessions. One or more G2X may reply. The interrogator detects
a single G2X reply and requests the PC, EPC, and CRC-16 from the chip. An
inventory round operates in one and only one session at a time. For an
example of an interrogator inventorying and accessing a single G2X refer to
Section 17, Ref. 1, Annex E.
Access: The process by which an interrogator transacts with (reads from or writes to)
individual G2X. An individual G2X must be uniquely identified prior to access.
Access comprises multiple commands, some of which employ one-time-pad
based cover-coding of the R=>T link.
139037 All information provided in this document is subject to legal disclaimers. © NXP B.V. 2013. All rights reserved.
Product data sheet
COMPANY PUBLIC
Rev. 3.8 — 11 November 2013
139038 21 of 48
NXP Semiconductors SL3ICS1002/1202
UCODE G2XM and G2XL
14.1 G2X Memory
For the general memory layout according to the standard Section 17, Ref. 1, refer to
Figure 6.17. The tag memory is logically subdivided into four distinct banks.
In accordance to the standard Section 17, Ref. 1, section 6.3.2.1. The tag memory of the
SL3ICS1002 G2XM is organized in following 4 memory sections:
The logical address of all memory banks begin at zero (00h).
Table 10. G2X memory sections
Name Size Bank
Reserved memory (32 bit ACCESS and 32 bit KILL password) 64 bit 00b
EPC (excluding 16 bit CRC-16 and 16 bit PC) 240 bit 01b
TID (including unique 32 bit serial number) 64 bit 10b
User memory (G2XM only) 512 bit 11b
Fig 4. G2X TID memory structure
Serial Number Model Number Mask-Designer Identifier Class Identifier
TID
0 31 0 11 0 11 0 7
3Fh 20h 1Fh 14h 13h 08h 07h 00h
0 6 0 4
1Fh 19h 18h 14h
Version Number Sub Version Number
00000001h to FFFFFFFFh 006h E2h
Whenever the 32 bit serial
is exceeded the sub version
is incremented by 1
Addresses 3Fh 00h
Addresses
Addresses
Bits
Bits
LS Byte
LSBit MSBit LSBit MSBit
MS Byte
LSBit MSBit LSBit MSBit
0000010b 00000b
Sub Version Nr Version (Silicon) Nr Model Nr. Mask ID
UCode EPC G2XM 00000b 0000011b 003h 006h
UCode EPC G2XL 00000b 0000100b 004h 006h
002h
139037 All information provided in this document is subject to legal disclaimers. © NXP B.V. 2013. All rights reserved.
Product data sheet
COMPANY PUBLIC
Rev. 3.8 — 11 November 2013
139038 22 of 48
NXP Semiconductors SL3ICS1002/1202
UCODE G2XM and G2XL
14.1.1 Memory map
[1] This is the initial memory content when delivered by NXP Semiconductors
[2] G2XL: HEX 3005 FB63 AC1F 3841 EC88 0467
G2XM: HEX 3005 FB63 AC1F 3681 EC88 0468
[3] only G2XM
Table 11. Memory map
Bank
address
Memory
address
Type Content Initial [1] Remark
Bank 00 00h – 1Fh Reserved kill password:
refer to Section 17, Ref. 1,
chapter 6.3.2.1.1
all 00h unlocked memory
20h – 3Fh Reserved access password:
refer to Section 17, Ref. 1,
chapter 6.3.2.1.1
all 00h unlocked memory
Bank 01 00h – 0Fh EPC CRC-16: refer to Section 17,
Ref. 1, chapter 6.3.2.1.2
memory mapped
calculated CRC
10h – 14h EPC Backscatter length:
refer to Section 17, Ref. 1,
chapter 6.3.2.1.2
00110b unlocked memory
15h EPC Reserved for future use:
refer to Section 17, Ref. 1,
chapter 6.3.2.1.2
0b unlocked memory
16h EPC Reserved for future use:
refer to Section 17, Ref. 1,
chapter 6.3.2.1.2
0b hardwired to 0
17h –1Fh EPC Numbering system indicator:
refer to Section 17, Ref. 1,
chapter 6.3.2.1.2
00h unlocked memory
20h - 10Fh EPC EPC:
refer to Section 17, Ref. 1,
chapter 6.3.2.1.2
[2] unlocked memory
Bank 10 00h – 07h TID allocation class identifier:
refer to Section 17, Ref. 1,
chapter 6.3.2.1.3
1110 0010b locked memory
08h – 13h TID tag mask designer identifier:
refer to Section 17, Ref. 1,
chapter 6.3.2.1.3
0000 0000 0110b locked memory
14h – 1Fh TID tag model number:
refer to Section 17, Ref. 1,
chapter 6.3.2.1.3
TMNR locked memory
20h – 3Fh TID serial number:
refer to [Section 17, Ref. 1,
chapter 6.3.2.1.3
SNR locked memory
Bank 11[3] 00h – 1FFh User user memory:
refer to [Section 17, Ref. 1,
chapter 6.3.2.1.4
undefined unlocked memory
139037 All information provided in this document is subject to legal disclaimers. © NXP B.V. 2013. All rights reserved.
Product data sheet
COMPANY PUBLIC
Rev. 3.8 — 11 November 2013
139038 23 of 48
NXP Semiconductors SL3ICS1002/1202
UCODE G2XM and G2XL
14.1.1.1 User memory (only G2XM)
The User Memory bank contains a sequential block of 512 bits (32 words of 16 bit)
ranging from address 00h to 1Fh. The user memory can be accessed via Select, Read or
Write command and it may be write locked, permanently write locked, unlocked or
permanently unlocked.
In addition reading of not only of the User Memory but of the whole memory including EPC
and TID can be protected by using the custom ReadProtect command.
14.1.1.2 Special behavior of user memory address 1Fh
WRITE or SELECT of user memory address 1Fh will falsely set an error flag. This will
affect the subsequent READ or SELECT.
The following commands will falsely set an internal error flag (without actually
causing an error):
1) WRITE to user memory with WordPtr=1Fh
2) SELECT to user memory with compare mask ending at bitaddress 1FFh
(e.g. Pointer=1FEh, length=1 or Pointer=1FDh, length=2 …)
Note: The error flag is set independent of the chip state (also chips in the e.g. Ready
state are affected).
The falsely set error flag will affect the following sub sequential commands:
A) READ command with WordCount=0 falsely responds with "memory overrun" error
B) SELECT command with Length<>0 falsely assumes non existing memory location
The behavior can be avoided with:
• Turning off the RF carrier to reset the chip (This is what readers typically do!).
• Using the READ command with WordCount<>0.
• Sending other command prior to READ or SELECT (e.g. WRITE to address<>1Fh,
ReqRN) or executing READ or SELECT two times.
Remark: The WRITE operation itself is not affected by this problem i.e. data is written
properly! With commercially available readers this behavior is typically not observed.
14.1.1.3 Supported EPC types
The EPC types are defined in the EPC Tag Standards document from EPCglobal.
These standards define completely that portion of EPC tag data that is standardized,
including how that data is encoded on the EPC tag itself (i.e. the EPC Tag Encodings), as
well as how it is encoded for use in the information systems layers of the EPC Systems
Network (i.e. the EPC URI or Uniform Resource Identifier Encodings).
The EPC Tag Encodings include a Header field followed by one or more Value Fields. The
Header field indicates the length of the Values Fields and contains a numbering system
identifier (NSI). The Value Fields contain a unique EPC Identifier and optional Filter Value
when the latter is judged to be important to encode on the tag itself.
139037 All information provided in this document is subject to legal disclaimers. © NXP B.V. 2013. All rights reserved.
Product data sheet
COMPANY PUBLIC
Rev. 3.8 — 11 November 2013
139038 24 of 48
NXP Semiconductors SL3ICS1002/1202
UCODE G2XM and G2XL
14.2 Sessions, selected and inventoried flags
Session, Selected and Inventory Flags are according the EPCglobal standard. For a
description refer to Section 17, Ref. 1, section 6.3.2.3.
14.2.1 G2X States and slot counter
For a description refer to Section 17, Ref. 1, section 6.3.2.4.
14.2.2 G2X State Diagram
The tag state are according the EPCglobal standard please refer to: Section 17, Ref. 1,
section 6.3.2.4 Tag states and slot counter.
A detailed tag state diagram is shown in Section 17, Ref. 1, figure 6.19. Refer also to
Section 17, Ref. 1, Annex B for the associated state-transition tables and to Section 17,
Ref. 1, Annex C for the associated command-response tables.
14.3 Managing tag populations
For a detailed description on how to manage an UCODE G2X tag populations refer to
Section 17, Ref. 1, chapter 6.3.2.6.
14.4 Selecting tag populations
For a detailed description of the UCODE G2X tag population selection process refer to
Section 17, Ref. 1, section 6.3.2.7.
14.5 Inventorying tag populations
For a detailed description on accessing individual tags based on the UCODE G2X refer to
Section 17, Ref. 1, section 6.3.2.8.
14.6 Accessing individual tags
For a detailed description on accessing individual tags based on the UCODE G2X refer to
Section 17, Ref. 1, section 6.3.2.9.
An example inventory and access of a single UCODE G2X tag is shown in Section 17,
Ref. 1, Annex E.1.
14.7 Interrogator commands and tag replies
For a detailed description refer to Section 17, Ref. 1, section 6.3.2.10.
14.7.1 Commands
An overview of interrogator to tag commands is located in Section 17, Ref. 1, Table 6.16.
Note that all mandatory commands are implemented on the G2X according to the
standard. Additionally the optional command Access is supported by the G2X (for details
refer to Section 14.11 “Optional Access Command”). Besides also custom commands are
implemented on the G2X (for details refer to Section 14.12 “Custom Commands”.
139037 All information provided in this document is subject to legal disclaimers. © NXP B.V. 2013. All rights reserved.
Product data sheet
COMPANY PUBLIC
Rev. 3.8 — 11 November 2013
139038 25 of 48
NXP Semiconductors SL3ICS1002/1202
UCODE G2XM and G2XL
14.7.2 State transition tables
The G2X responses to interrogator commands are defined by State Annex B transition
tables in Section 17, Ref. 1. Following states are implemented on the G2X:
• Ready, for a description refer to Section 17, Ref. 1, Annex B.1.
• Arbitrate, for a description refer to Section 17, Ref. 1, Annex B.2.
• Reply, for a description refer to Section 17, Ref. 1, Annex B.3.
• Acknowledged, for a description refer to Section 17, Ref. 1, Annex B.4.
• Open, for a description refer to Section 17, Ref. 1, Annex B.5.
• Secured, for a description refer to Section 17, Ref. 1, Annex B.6.
• Killed, for a description refer to Section 17, Ref. 1, Annex B.7.
14.7.3 Command response tables
The G2X responses to interrogator commands are described in following Annex C
sections of Section 17, Ref. 1:
• Power-up, for a description refer to Section 17, Ref. 1, Annex C.1.
• Query, for a description refer to Section 17, Ref. 1, Annex C.2.
• QueryRep, for a description refer to Section 17, Ref. 1, Annex C.3.
• QueryAdjust, for a description refer to Section 17, Ref. 1, Annex C.4.
• ACK, for a description refer to Section 17, Ref. 1, Annex C.5.
• NAK, for a description refer to Section 17, Ref. 1, Annex C.6.
• Req_RN, for a description refer to Section 17, Ref. 1, Annex C.7.
• Select, for a description refer to Section 17, Ref. 1, Annex C.8.
• Read, for a description refer to Section 17, Ref. 1, Annex C.9.
• Write, for a description refer to Section 17, Ref. 1, Annex C.10.
• Kill, for a description refer to Section 17, Ref. 1, Annex C.11.
• Lock, for a description refer to Section 17, Ref. 1, Annex C.12.
• Access, for a description refer to Section 17, Ref. 1, Annex C.13.
• T2 time-out, for a description refer to Section 17, Ref. 1, Annex C.17.
• Invalid command, for a description refer to Section 17, Ref. 1, Annex C.18.
14.7.4 Example data-flow exchange
For data flow-exchange examples refer to Section 17, Ref. 1, Annex K:
• K.1 Overview of the data-flow exchange
• K.2 Tag memory contents and lock-field values
• K.3 Data-flow exchange and command sequence
14.8 Mandatory Select Commands
Select commands select a particular UCODE G2X tag population based on user-defined
criteria.
139037 All information provided in this document is subject to legal disclaimers. © NXP B.V. 2013. All rights reserved.
Product data sheet
COMPANY PUBLIC
Rev. 3.8 — 11 November 2013
139038 26 of 48
NXP Semiconductors SL3ICS1002/1202
UCODE G2XM and G2XL
14.8.1 Select
For a detailed description of the mandatory Select command refer to Section 17, Ref. 1,
section 6.3.2.10.
14.9 Mandatory Inventory Commands
Inventory commands are used to run the collision arbitration protocol.
14.9.1 Query
For a detailed description of the mandatory Query command refer to Section 17, Ref. 1,
section 6.3.2.10.
14.9.2 QueryAdjust
For a detailed description of the mandatory QueryAdjust command refer to Section 17,
Ref. 1, section 6.3.2.10.
14.9.3 QueryRep
For a detailed description of the mandatory QueryRep command refer to Section 17,
Ref. 1, section 6.3.2.10.
14.9.4 ACK
For a detailed description of the mandatory ACK command refer to Section 17, Ref. 1,
section 6.3.2.10.
14.9.5 NAK
For a detailed description of the mandatory NAK command refer to Section 17, Ref. 1,
section 6.3.2.10.
139037 All information provided in this document is subject to legal disclaimers. © NXP B.V. 2013. All rights reserved.
Product data sheet
COMPANY PUBLIC
Rev. 3.8 — 11 November 2013
139038 27 of 48
NXP Semiconductors SL3ICS1002/1202
UCODE G2XM and G2XL
14.10 Mandatory Access Commands
Access commands are used to read or write data from or to the G2X memory. For a
detailed description of the mandatory Access command refer to Section 17, Ref. 1,
section 6.3.2.10.
14.10.1 REQ_RN
Access commands are used to read or write data from or to the G2X memory. For a
detailed description of the mandatory Access command refer to Section 17, Ref. 1,
section 6.3.2.10.
14.10.2 READ
For a detailed description of the mandatory Req_RN command refer to Section 17, Ref. 1,
section 6.3.2.10.
14.10.3 WRITE
For a detailed description of the mandatory Write command refer to Section 17, Ref. 1,
section 6.3.2.10.
14.10.4 KILL
For a detailed description of the mandatory Kill command refer to Section 17, Ref. 1,
section 6.3.2.10.
14.10.5 LOCK
For a detailed description of the mandatory Lock command refer to Section 17, Ref. 1,
section 6.3.2.10.
14.11 Optional Access Command
14.11.1 Access
For a detailed description of the optional Access command refer to Section 17, Ref. 1,
section 6.3.2.10.
139037 All information provided in this document is subject to legal disclaimers. © NXP B.V. 2013. All rights reserved.
Product data sheet
COMPANY PUBLIC
Rev. 3.8 — 11 November 2013
139038 28 of 48
NXP Semiconductors SL3ICS1002/1202
UCODE G2XM and G2XL
14.12 Custom Commands
14.12.1 ReadProtect
The G2X ReadProtect custom command enables reliable read protection of the entire
G2X memory. Executing ReadProtect from the Secured state will set the ReadProtect-bit
to '1'. With the ReadProtect-Bit set the G2X will continue to work unaffected but fail its
content.
Following commands will be disabled: Read, Write, Kill, Lock, Access, ReadProtect,
ChangeEAS, EAS Alarm and Calibrate. The G2X will only react upon an anticollision with
Select, Query, QueryRep, QueryAdjust, ACK (no truncated reply), NAK, ReqRN but reply
with zeros as EPC and CRC-16 content (except PC/password). ACK will return zeros
except for the PC.
The read protection can be removed by executing Reset ReadProtect. The
ReadProtect-Bit will than be cleared.
Devices whose access password is zero will ignore the command. A frame-sync must be
prepended the command.
After sending the ReadProtect command an interrogator shall transmit CW for the lesser
of TReply or 20 ms, where TReply is the time between the interrogator's ReadProtect
command and the backscattered reply. An interrogator may observe three possible
responses after sending a ReadProtect, depending on the success or failure of the
operation:
• ReadProtect succeeds: After completing the ReadProtect the G2X shall backscatter
the reply shown in Table 14 comprising a header (a 0-bit), the tag's handle, and a
CRC-16 calculated over the 0-bit and handle. Immediately after this reply the G2X will
render itself to this ReadProtect mode. If the interrogator observes this reply within 20
ms then the ReadProtect completed successfully.
• The G2X encounters an error: The G2X will backscatter an error code during the CW
period rather than the reply shown in the EPCglobal Spec (see Annex I for error-code
definitions and for the reply format).
• ReadProtect does not succeed: If the interrogator does not observe a reply within
20 ms then the ReadProtect did not complete successfully. The interrogator may
issue a Req_RN command (containing the handle) to verify that the G2X is still in the
interrogation zone, and may re-initiate the ReadProtect command.
The G2X reply to the ReadProtect command will use the extended preamble shown in
EPCglobal Spec (Figure 6.11 or Figure 6.15), as appropriate (i.e. a Tag shall reply as if
TRext=1) regardless of the TRext value in the Query that initiated the round.
Table 12. ReadProtect command
Command RN CRC-16
# of bits 16 16 16
description 11100000 00000001 handle -
139037 All information provided in this document is subject to legal disclaimers. © NXP B.V. 2013. All rights reserved.
Product data sheet
COMPANY PUBLIC
Rev. 3.8 — 11 November 2013
139038 29 of 48
NXP Semiconductors SL3ICS1002/1202
UCODE G2XM and G2XL
Table 13. G2X reply to a successful ReadProtect procedure
Header RN CRC-16
# of bits 1 16 16
description 0 handle -
Table 14. ReadProtect command-response table
Starting State Condition Response Next State
ready all – ready
arbitrate, reply,
acknowledged
all – arbitrate
open all - open
secured valid handle & invalid
access password
– arbitrate
valid handle & valid
non zero access
password
Backscatter handle,
when done
secured
invalid handle – secured
killed all – killed
139037 All information provided in this document is subject to legal disclaimers. © NXP B.V. 2013. All rights reserved.
Product data sheet
COMPANY PUBLIC
Rev. 3.8 — 11 November 2013
139038 30 of 48
NXP Semiconductors SL3ICS1002/1202
UCODE G2XM and G2XL
14.12.2 Reset ReadProtect
Reset ReadProtect allows an interrogator to resets the ReadProtect-bit and re-enables
reading of the G2X memory content according the EPCglobal specification.
The G2X will execute Reset ReadProtect from the Open or Secured states.
If a G2X in the Open or Secured states receives a Reset ReadProtect with a valid CRC-16
and a valid handle but an incorrect access password, it will not reply and transit to the
Arbitrate state.
If a G2X in the Open or Secured states receives a Reset ReadProtect with a valid CRC-16
and a valid handle but the ReadProtect-Bit is not set ('0'), it will not change the
ReadProtect-Bit but backscatter the reply shown in Table 17.
If a G2X in the Open or Secured receives a Reset ReadProtect with a valid CRC-16 but
an invalid handle, or it receives a Reset ReadProtect before which the immediately
preceding command was not a Req_RN, it will ignore the Reset ReadProtect and remain
in its current state.
A frame-sync must be prepended the Reset ReadProtect command.
After sending a Reset ReadProtect an interrogator shall transmit CW for the lesser of
TReply or 20 ms, where TReply is the time between the interrogator's Reset ReadProtect
command and the G2X backscattered reply. An interrogator may observe three possible
responses after sending a Reset ReadProtect, depending on the success or failure of the
operation:
• Write succeeds: After completing the Reset ReadProtect a G2X will backscatter the
reply shown in Table 17 comprising a header (a 0-bit), the handle, and a CRC-16
calculated over the 0-bit and handle. If the interrogator observes this reply within
20 ms then the Reset ReadProtect completed successfully.
• The G2X encounters an error: The G2X will backscatter an error code during the CW
period rather than the reply shown in Table 17 (see EPCglobal Spec for error-code
definitions and for the reply format).
• Write does not succeed: If the interrogator does not observe a reply within 20 ms then
the Reset ReadProtect did not complete successfully. The interrogator may issue a
Req_RN command (containing the handle) to verify that the G2X is still in the
interrogation zone, and may reissue the Reset ReadProtect command.
The G2X reply to the Reset ReadProtect command will use the extended preamble shown
in EPCglobal Spec (Figure 6.11 or Figure 6.15), as appropriate (i.e. a G2X will reply as if
TRext=1 regardless of the TRext value in the Query that initiated the round.
The Reset ReadProtect command is structured as following:
• 16 bit command
• Password: 32 bit Access-Password XOR with 2 times current RN16
• 16 bit handle
• CRC-16 calculate over the first command-code bit to the last handle bit
139037 All information provided in this document is subject to legal disclaimers. © NXP B.V. 2013. All rights reserved.
Product data sheet
COMPANY PUBLIC
Rev. 3.8 — 11 November 2013
139038 31 of 48
NXP Semiconductors SL3ICS1002/1202
UCODE G2XM and G2XL
Table 15. Reset ReadProtect command
Command Password RN CRC-16
# of bits 16 32 16 16
description 11100000
00000010
(access
password)
2*RN16
handle -
Table 16. G2X reply to a successful Reset ReadProtect command
Header RN CRC-16
# of bits 1 16 16
description 0 handle -
Table 17. Reset ReadProtect command-response table
Starting State Condition Response Next State
ready all – ready
arbitrate, reply,
acknowledged
all – arbitrate
open ReadProtect bit is set,
valid handle & valid access password
Backscatter handle,
when done
open
ReadProtect bit is set,
valid handle & invalid access password
– arbitrate
ReadProtect bit is set,
invalid handle
– open
ReadProtect bit is reset – open
secured ReadProtect bit is set,
valid handle & valid access password
Backscatter handle,
when done
secured
ReadProtect bit is set,
valid handle & invalid access password
– arbitrate
ReadProtect bit is set,
invalid handle
– secured
ReadProtect bit is reset – secured
killed all – killed
139037 All information provided in this document is subject to legal disclaimers. © NXP B.V. 2013. All rights reserved.
Product data sheet
COMPANY PUBLIC
Rev. 3.8 — 11 November 2013
139038 32 of 48
NXP Semiconductors SL3ICS1002/1202
UCODE G2XM and G2XL
14.12.3 ChangeEAS
A G2X equipped RFID tag can be enhanced by a stand-alone operating EAS alarm
feature. With an EAS-Alarm bit set to '1' the tag will reply to an EAS_Alarm command by
backscattering a 64 bit alarm code without the need of a Select or Query. The EAS is a
built-in solution so no connection to a backend database is required. As it is a custom
command no Select or Query is required to detect the EAS state enabling fast, reliable
and offline article surveillance.
ChangeEAS can be executed from the Secured state only. The command will be ignored
if the Access Password is zero, the command will also be ignored with an invalid CRC-16
or an invalid handle, the G2X will than remain in the current state. The CRC-16 is
calculated from the first command-code bit to the last handle bit. A frame-sync must be
prepended the command.
The G2X reply to a successful ChangeEAS will use the extended preamble, as
appropriate (i.e. a Tag shall reply as if TRext=1) regardless of the TRext value in the
Query that initiated the round.
After sending a ChangeEAS an interrogator shall transmit CW for less than TReply or
20 ms, where TReply is the time between the interrogator's ChangeEAS command and
the G2X backscattered reply. An interrogator may observe three possible responses after
sending a ChangeEAS, depending on the success or failure of the operation
• Write succeeds: After completing the ChangeEAS a G2X will backscatter the reply
shown in Table 20 comprising a header (a 0-bit), the handle, and a CRC-16 calculated
over the 0-bit and handle. If the interrogator observes this reply within
20 ms then the ChangeEAS completed successfully.
• The G2X encounters an error: The G2X will backscatter an error code during the CW
period rather than the reply shown in Table 20 (see EPCglobal Spec for error-code
definitions and for the reply format).
• Write does not succeed: If the interrogator does not observe a reply within 20 ms then
the ChangeEAS did not complete successfully. The interrogator may issue a Req_RN
command (containing the handle) to verify that the G2X is still in the interrogator's
field, and may reissue the ChangeEAS command.
Upon receiving a valid ChangeEAS command a G2X will perform the commanded
set/reset operation of the EAS_Alarm-Bit.
If EAS-Bit is set, the EAS_Alarm command will be available after the next power up and
reply the 64 bit EAS code upon execution. Otherwise the EAS_Alarm command will be
ignored.
Table 18. ChangeEAS command
Command ChangeEas RN CRC-16
# of bits 16 1 16 16
description 11100000
00000011
1 ... set EAS system bit
0 ... reset EAS system bit
handle
139037 All information provided in this document is subject to legal disclaimers. © NXP B.V. 2013. All rights reserved.
Product data sheet
COMPANY PUBLIC
Rev. 3.8 — 11 November 2013
139038 33 of 48
NXP Semiconductors SL3ICS1002/1202
UCODE G2XM and G2XL
Table 19. G2X reply to a successful ChangeEAS command
Header RN CRC-16
# of bits 1 16 16
description 0 handle -
Table 20. ChangeEAS command-response table
Starting State Condition Response Next State
ready all – ready
arbitrate, reply,
acknowledged
all – arbitrate
open all – open
secured valid handle Backscatter handle,
when done
secured
invalid handle – secured
killed all – killed
Starting State Condition Response Next State
139037 All information provided in this document is subject to legal disclaimers. © NXP B.V. 2013. All rights reserved.
Product data sheet
COMPANY PUBLIC
Rev. 3.8 — 11 November 2013
139038 34 of 48
NXP Semiconductors SL3ICS1002/1202
UCODE G2XM and G2XL
14.12.4 EAS_Alarm
EAS_Alarm is a custom command causing the G2X to immediately backscatter an
EAS-Alarmcode, when EAS ALARM bit is set without any delay caused by Select, Query
and without the need for a backend database.
The EAS feature of the G2X is available after enabling it by sending a ChangeEAS
command described in Section 14.12.3 “ChangeEAS”. With an EAS-Alarm bit set to '1' the
G2X will reply to an EAS_Alarm command by backscattering a fixed 64 bit alarm code. A
G2X will reply to an EAS_Alarm command from the ready state only.
If the EAS-Alarm bit is reset ('0') by sending a ChangeEAS command in the password
protected Secure state the G2X will not reply to an EAS_Alarm command.
The EAS_Alarm command is structured as following:
• 16 bit command
• 16 bit inverted command
• DR (TRcal divide ratio) sets the T=>R link frequency as described in EPCglobal Spec.
6.3.1.2.8 and Table 6.9.
• M (cycles per symbol) sets the T=>R data rate and modulation format as shown in
EPCglobal Spec. Table 6.10.
• TRext chooses whether the T=>R preamble is prepended with a pilot tone as
described in EPCglobal Spec. 6.3.1.3.
A preamble must be prepended the EAS_Alarm command according EPCglobal Spec,
6.3.1.2.8.
Upon receiving an EAS_Alarm command the tag loads the CRC5 register with 01001b
and backscatters the 64 bit alarm code accordingly. The reader is now able to calculate
the CRC5 over the backscattered 64 bits received to verify the received code.
Table 21. EAS_Alarm command
Command Inv_Command DR M TRext CRC-16
# of bits 16 16 1 2 1 16
description 11100000
00000100
00011111
11111011
0: DR=8
1: DR=64/3
00: M=1
01: M=2
10: M=4
11: M=8
0: No pilot
tone
1: Use pilot
tone
-
Table 22. G2X reply to a successful EAS_Alarm command
Header EAS Code
# of bits 1 64
description 0 CRC5 (MSB)
139037 All information provided in this document is subject to legal disclaimers. © NXP B.V. 2013. All rights reserved.
Product data sheet
COMPANY PUBLIC
Rev. 3.8 — 11 November 2013
139038 35 of 48
NXP Semiconductors SL3ICS1002/1202
UCODE G2XM and G2XL
Table 23. Eas_Alarm command-response table
Starting State Condition Response Next State
ready EAS-bit is set and
non-zero access
password
Backscatter Alarm
code
ready
arbitrate, reply,
acknowledged
EAS-bit is set and
non-zero access
password
– arbitrate
open EAS-bit is set and
non-zero access
password
open
secured EAS-bit is set and
non-zero access
password
secured
killed EAS-bit is set and
non-zero access
password
– killed
139037 All information provided in this document is subject to legal disclaimers. © NXP B.V. 2013. All rights reserved.
Product data sheet
COMPANY PUBLIC
Rev. 3.8 — 11 November 2013
139038 36 of 48
NXP Semiconductors SL3ICS1002/1202
UCODE G2XM and G2XL
14.12.5 Calibrate
After execution of the custom Calibrate command the G2X will continuously backscatter
the user memory content in an infinite loop. The G2XL will continuously backscatter zeros.
This command can be used for frequency spectrum measurements. Calibrate can only be
executed from the Secure state with an non-zero Access Password set otherwise the
command will be ignored.
The Calibrate command includes a CRC-16 calculated over the whole command, the
handle and a prepended frame-sync.
[1] G2XM
[2] G2XL
Table 24. Calibrate command
Command RN16 CRC-16
# of bits 16 16 16
description 11100000 00000101 handle -
Table 25. G2X reply to a successful Calibrate command
Header Infinite repeat
# of bits 1 512 (looped)
description 0 User memory data[1]
zeros[2]
Table 26. Calibrate command-response table
Starting State Condition Response Next State
ready all – ready
arbitrate, reply,
acknowledged
all – arbitrate
secured nonzero access
password
Backscatter infinite _
access password is
zero
– secured
killed all – killed
139037 All information provided in this document is subject to legal disclaimers. © NXP B.V. 2013. All rights reserved.
Product data sheet
COMPANY PUBLIC
Rev. 3.8 — 11 November 2013
139038 37 of 48
NXP Semiconductors SL3ICS1002/1202
UCODE G2XM and G2XL
15. Support information
15.1 CRC Calculation EXAMPLE
Old RN = 3D5Bh
Table 27. Practical example of CRC calculation for a 'Req_RN' command by the reader
CRC Calculated @ Reader
Cmd Code for Req_RN
F F F F
1 F F F E
1 F F F C
0 E F D 9
0 C F 9 3
0 8 F 0 7
0 0 E 2 F
0 1 C 5 E
1 2 8 9 9
First Byte of RN
0 5 1 3 A
0 A 2 7 4
1 4 4 E 8
1 9 9 F 1
1 3 3 E 2
1 7 7 E 5
0 E F C A
1 D F 9 4
Second Byte of RN
0 A F 0 9
1 5 E 1 2
0 B C 2 4
1 7 8 4 8
1 E 0 B 1
0 D 1 4 3
1 A 2 8 6
1 4 5 0 C -> ones complement: B A F 3
=> Command-Sequence: C1 3D 5B BA F3 hex
139037 All information provided in this document is subject to legal disclaimers. © NXP B.V. 2013. All rights reserved.
Product data sheet
COMPANY PUBLIC
Rev. 3.8 — 11 November 2013
139038 38 of 48
NXP Semiconductors SL3ICS1002/1202
UCODE G2XM and G2XL
Table 28. Practical example of CRC calculation for a 'Req_RN' command by the reader
CRC Calculated @ Tag
Cmd Code for Req_RN
F F F F
1 F F F E
1 F F F C
0 E F D 9
0 C F 9 3
0 8 F 0 7
0 0 E 2 F
0 1 C 5 E
1 2 8 9 9
First Byte of RN
0 5 1 3 A
0 A 2 7 4
1 4 4 E 8
1 9 9 F 1
1 3 3 E 2
1 7 7 E 5
0 E F C A
1 D F 9 4
Second Byte of RN
0 A F 0 9
1 5 E 1 2
0 B C 2 4
1 7 8 4 8
1 E 0 B 1
0 D 1 4 3
1 A 2 8 6
1 4 5 0 C
First Byte of CRC
1 9 A 3 9
0 2 4 5 3
1 5 8 8 7
1 A 1 2 F
1 4 2 5 E
0 8 4 B C
1 0 9 7 8
0 1 2 F 0 Second Byte of CRC
1 3 5 C 1
1 7 B A 3
1 E 7 6 7
1 C E C E
0 8 D B D
0 0 B 5 B
1 0 6 9 7
1 1 D 0 F -> Residue OK
139037 All information provided in this document is subject to legal disclaimers. © NXP B.V. 2013. All rights reserved.
Product data sheet
COMPANY PUBLIC
Rev. 3.8 — 11 November 2013
139038 39 of 48
NXP Semiconductors SL3ICS1002/1202
UCODE G2XM and G2XL
16. Abbreviations
Table 29. Abbreviations
Acronym Description
CRC Cyclic redundancy check
CW Continuos wave
EEPROM Electrically Erasable Programmable Read Only Memory
EPC Electronic Product Code (containing Header, Domain Manager, Object Class
and Serial Number)
FM0 Bi phase space modulation
G2 Generation 2
HBM Human Body Model
IC Integrated Circuit
LSB Least Significant Byte/Bit
MSB Most Significant Byte/Bit
NRZ Non-Return to Zero coding
RF Radio Frequency
RTF Reader Talks First
Tari Type A Reference Interval (ISO 18000-6)
UHF Ultra High Frequency
Xxb Value in binary notation
xxhex Value in hexadecimal notation
139037 All information provided in this document is subject to legal disclaimers. © NXP B.V. 2013. All rights reserved.
Product data sheet
COMPANY PUBLIC
Rev. 3.8 — 11 November 2013
139038 40 of 48
NXP Semiconductors SL3ICS1002/1202
UCODE G2XM and G2XL
17. References
[1] EPCglobal: EPC Radio-Frequency Identity Protocols Class-1 Generation-2 UHF
RFID Protocol for Communications at 860 MHz – 960 MHz, Version 1.1.0
(December 17, 2005)
[2] EPCglobal: EPC Tag Data Standards
[3] EPCglobal (2004): FMCG RFID Physical Requirements Document (draft)
[4] EPCglobal (2004): Class-1 Generation-2 UHF RFID Implementation Reference
(draft)
[5] European Telecommunications Standards Institute (ETSI), EN 302 208:
Electromagnetic compatibility and radio spectrum matters (ERM) – Radio-frequency
identification equipment operating in the band 865 MHz to 868 MHz with power
levels up to 2 W, Part 1 – Technical characteristics and test methods
[6] European Telecommunications Standards Institute (ETSI), EN 302 208:
Electromagnetic compatibility and radio spectrum matters (ERM) – Radio-frequency
identification equipment operating in the band 865 MHz to 868 MHz with power
levels up to 2 W, Part 2 – Harmonized EN under article 3.2 of the R&TTE directive
[7] [CEPT1]: CEPT REC 70-03 Annex 1
[8] [ETSI1]: ETSI EN 330 220-1, 2
[9] [ETSI3]: ETSI EN 302 208-1, 2 V<1.1.1> (2004-09-Electromagnetic compatibility
And Radio spectrum Matters (ERM) Radio Frequency Identification Equipment
operating in the band 865 - MHz to 868 MHz with power levels up to 2 W Part 1:
Technical characteristics and test methods.
[10] [FCC1]: FCC 47 Part 15 Section 247
[11] ISO/IEC Directives, Part 2: Rules for the structure and drafting of International
Standards
[12] ISO/IEC 3309: Information technology – Telecommunications and information
exchange between systems – High-level data link control (HDLC) procedures –
Frame structure
[13] ISO/IEC 15961: Information technology, Automatic identification and data capture –
Radio frequency identification (RFID) for item management – Data protocol:
application interface
[14] ISO/IEC 15962: Information technology, Automatic identification and data capture
techniques – Radio frequency identification (RFID) for item management – Data
protocol: data encoding rules and logical memory functions
[15] ISO/IEC 15963: Information technology — Radio frequency identification for item
management — Unique identification for RF tags
[16] ISO/IEC 18000-1: Information technology — Radio frequency identification for item
management — Part 1: Reference architecture and definition of parameters to be
standardized
[17] ISO/IEC 18000-6: Information technology automatic identification and data capture
techniques — Radio frequency identification for item management air interface —
Part 6: Parameters for air interface communications at 860–960 MHz
[18] ISO/IEC 19762: Information technology AIDC techniques – Harmonized vocabulary
– Part 3: radio-frequency identification (RFID)
139037 All information provided in this document is subject to legal disclaimers. © NXP B.V. 2013. All rights reserved.
Product data sheet
COMPANY PUBLIC
Rev. 3.8 — 11 November 2013
139038 41 of 48
NXP Semiconductors SL3ICS1002/1202
UCODE G2XM and G2XL
[19] U.S. Code of Federal Regulations (CFR), Title 47, Chapter I, Part 15:
Radio-frequency devices, U.S. Federal Communications Commission.
[20] Data sheet - Delivery type description – General specification for 8” wafer on
UV-tape with electronic fail die marking, BL-ID document number: 1093**1
1. ** ... document version number
139037 All information provided in this document is subject to legal disclaimers. © NXP B.V. 2013. All rights reserved.
Product data sheet
COMPANY PUBLIC
Rev. 3.8 — 11 November 2013
139038 42 of 48
NXP Semiconductors SL3ICS1002/1202
UCODE G2XM and G2XL
18. Revision history
Table 30. Revision history
Document ID Release date Data sheet status Change notice Supersedes
SL3ICS1002_1202 v.3.8 20131111 Product data sheet - SL3ICS1002_1202 v.3.7
Modifications: • Update of the delivery form (TSSOP package due to DOD removed)
SL3ICS1002_1202 v.3.7 20121009 Product data sheet - 139036
Modifications: • Update of the delivery form
139036 20110310 Product data sheet 139035
Modifications: • Table 4 “TSSOP8 Marking”: added
• Section 14.1.1.2 “Special behavior of user memory address 1Fh”: added
139035 20091102 Product data sheet 139034
Modifications: • Type SOT1122 added
• Figure 2 “Wafer layout and pinning information”: correction of drawing
139034 20090721 Product data sheet 139033
Modifications: • Table 11 “TSSOP8 characteristics” andTable 7 “Package interface characteristics” :removed
“Memory characteristics”
139033 20090605 Product data sheet - 139032
139132
Modifications: • This data sheet is a combination of data sheets SL3ICS1002 and SL3ICS1202
• New type FCS2 Aluminum, SOT1040AB2 added
• Section 8.1.6 “Fail die identification”: added
• Section 11 “Packing information”: edited
139032 20080716 Product data sheet 139031
Modifications: • rephrasing of Section 2 “Features and benefits” on page 2
• added “calibrate command” in Section 2 “Features and benefits” on page 2
• redesign of Figure 1 “Block diagram of G2X IC” on page 4
• merging of Fig. 2 Pinning and Fig. 3 Wafer layout - see Figure 2 “Wafer layout and pinning
information” on page 5
• added type “FCS2 Polymer Strap - SOT1040AA1” in Section 4 “Ordering information”,
Section 6 “Wafer layout and pinning information”, Section 7 “Package outline”, Section 8
“Mechanical specification”, Section 9 “Limiting values”, Section 10 “Characteristics”
• added Section 11 “Handling information for Flip Chip Strap (FCS2, SOT1040)” on page 19
• added Section 11 “Packing information” on page 12
• added Table 8 “Symbol description” on page 14
• correction of Table 11 “Memory map” on page 22
• removed “ongoing” in 32 bit ongoing in Section 2.1 and Table 10 “G2X memory sections”
139031 20080428 Product data sheet 139030
139037 All information provided in this document is subject to legal disclaimers. © NXP B.V. 2013. All rights reserved.
Product data sheet
COMPANY PUBLIC
Rev. 3.8 — 11 November 2013
139038 43 of 48
NXP Semiconductors SL3ICS1002/1202
UCODE G2XM and G2XL
Modifications: • update of Table 1 “Ordering information” on page 3
• added Section 7 “Package outline” on page 6
• added Section 8.1.7 “Map file distribution” on page 9
• added Table 9 “Limiting values TSSOP8 [1][2]” on page 14
• added room temperature in Table 11 “Memory characteristics” on page 15
• added Section 10.2 “TSSOP8 characteristics” on page 17
• update of the “EPCglobal compliance and interoperability certification” in Section
12.4 “Air interface standards” on page 15
• correction of “(excluding 16 bit CRC-16 and 16 bit PC) in Table 10 “G2X memory sections”
on page 21
• correction of Initials in “tag mask designer” in Table 11 “Memory map” on page 22
• removed the sentence “The ChangeEAS custom command will toggle the state of the
EAS-Alarm bit located in the EEprom” in Section 14.12.3 “ChangeEAS” on page 32.
• added description of ChangeEAS in Table 18 “ChangeEAS command” on page 32
139030 20071221 Product data sheet - 139011
Modifications: • change of product status
• general update
139011 20070910 Objective data sheet - 139010
Modifications: • removed double section Change EAS, EAS Alarm, Chapter 12.11.7
• changed “Reader” to “Tag”
139010 20070612 Objective data sheet - -
• initial version
Table 30. Revision history …continued
Document ID Release date Data sheet status Change notice Supersedes
139037 All information provided in this document is subject to legal disclaimers. © NXP B.V. 2013. All rights reserved.
Product data sheet
COMPANY PUBLIC
Rev. 3.8 — 11 November 2013
139038 44 of 48
NXP Semiconductors SL3ICS1002/1202
UCODE G2XM and G2XL
19. Legal information
19.1 Data sheet status
[1] Please consult the most recently issued document before initiating or completing a design.
[2] The term ‘short data sheet’ is explained in section “Definitions”.
[3] The product status of device(s) described in this document may have changed since this document was published and may differ in case of multiple devices. The latest product status
information is available on the Internet at URL http://www.nxp.com.
19.2 Definitions
Draft — The document is a draft version only. The content is still under
internal review and subject to formal approval, which may result in
modifications or additions. NXP Semiconductors does not give any
representations or warranties as to the accuracy or completeness of
information included herein and shall have no liability for the consequences of
use of such information.
Short data sheet — A short data sheet is an extract from a full data sheet
with the same product type number(s) and title. A short data sheet is intended
for quick reference only and should not be relied upon to contain detailed and
full information. For detailed and full information see the relevant full data
sheet, which is available on request via the local NXP Semiconductors sales
office. In case of any inconsistency or conflict with the short data sheet, the
full data sheet shall prevail.
Product specification — The information and data provided in a Product
data sheet shall define the specification of the product as agreed between
NXP Semiconductors and its customer, unless NXP Semiconductors and
customer have explicitly agreed otherwise in writing. In no event however,
shall an agreement be valid in which the NXP Semiconductors product is
deemed to offer functions and qualities beyond those described in the
Product data sheet.
19.3 Disclaimers
Limited warranty and liability — Information in this document is believed to
be accurate and reliable. However, NXP Semiconductors does not give any
representations or warranties, expressed or implied, as to the accuracy or
completeness of such information and shall have no liability for the
consequences of use of such information. NXP Semiconductors takes no
responsibility for the content in this document if provided by an information
source outside of NXP Semiconductors.
In no event shall NXP Semiconductors be liable for any indirect, incidental,
punitive, special or consequential damages (including - without limitation - lost
profits, lost savings, business interruption, costs related to the removal or
replacement of any products or rework charges) whether or not such
damages are based on tort (including negligence), warranty, breach of
contract or any other legal theory.
Notwithstanding any damages that customer might incur for any reason
whatsoever, NXP Semiconductors’ aggregate and cumulative liability towards
customer for the products described herein shall be limited in accordance
with the Terms and conditions of commercial sale of NXP Semiconductors.
Right to make changes — NXP Semiconductors reserves the right to make
changes to information published in this document, including without
limitation specifications and product descriptions, at any time and without
notice. This document supersedes and replaces all information supplied prior
to the publication hereof.
Suitability for use — NXP Semiconductors products are not designed,
authorized or warranted to be suitable for use in life support, life-critical or
safety-critical systems or equipment, nor in applications where failure or
malfunction of an NXP Semiconductors product can reasonably be expected
to result in personal injury, death or severe property or environmental
damage. NXP Semiconductors and its suppliers accept no liability for
inclusion and/or use of NXP Semiconductors products in such equipment or
applications and therefore such inclusion and/or use is at the customer’s own
risk.
Applications — Applications that are described herein for any of these
products are for illustrative purposes only. NXP Semiconductors makes no
representation or warranty that such applications will be suitable for the
specified use without further testing or modification.
Customers are responsible for the design and operation of their applications
and products using NXP Semiconductors products, and NXP Semiconductors
accepts no liability for any assistance with applications or customer product
design. It is customer’s sole responsibility to determine whether the NXP
Semiconductors product is suitable and fit for the customer’s applications and
products planned, as well as for the planned application and use of
customer’s third party customer(s). Customers should provide appropriate
design and operating safeguards to minimize the risks associated with their
applications and products.
NXP Semiconductors does not accept any liability related to any default,
damage, costs or problem which is based on any weakness or default in the
customer’s applications or products, or the application or use by customer’s
third party customer(s). Customer is responsible for doing all necessary
testing for the customer’s applications and products using NXP
Semiconductors products in order to avoid a default of the applications and
the products or of the application or use by customer’s third party
customer(s). NXP does not accept any liability in this respect.
Limiting values — Stress above one or more limiting values (as defined in
the Absolute Maximum Ratings System of IEC 60134) will cause permanent
damage to the device. Limiting values are stress ratings only and (proper)
operation of the device at these or any other conditions above those given in
the Recommended operating conditions section (if present) or the
Characteristics sections of this document is not warranted. Constant or
repeated exposure to limiting values will permanently and irreversibly affect
the quality and reliability of the device.
Terms and conditions of commercial sale — NXP Semiconductors
products are sold subject to the general terms and conditions of commercial
sale, as published at http://www.nxp.com/profile/terms, unless otherwise
agreed in a valid written individual agreement. In case an individual
agreement is concluded only the terms and conditions of the respective
agreement shall apply. NXP Semiconductors hereby expressly objects to
applying the customer’s general terms and conditions with regard to the
purchase of NXP Semiconductors products by customer.
No offer to sell or license — Nothing in this document may be interpreted or
construed as an offer to sell products that is open for acceptance or the grant,
conveyance or implication of any license under any copyrights, patents or
other industrial or intellectual property rights.
Document status[1][2] Product status[3] Definition
Objective [short] data sheet Development This document contains data from the objective specification for product development.
Preliminary [short] data sheet Qualification This document contains data from the preliminary specification.
Product [short] data sheet Production This document contains the product specification.
139037 All information provided in this document is subject to legal disclaimers. © NXP B.V. 2013. All rights reserved.
Product data sheet
COMPANY PUBLIC
Rev. 3.8 — 11 November 2013
139038 45 of 48
NXP Semiconductors SL3ICS1002/1202
UCODE G2XM and G2XL
Export control — This document as well as the item(s) described herein
may be subject to export control regulations. Export might require a prior
authorization from competent authorities.
Quick reference data — The Quick reference data is an extract of the
product data given in the Limiting values and Characteristics sections of this
document, and as such is not complete, exhaustive or legally binding.
Non-automotive qualified products — Unless this data sheet expressly
states that this specific NXP Semiconductors product is automotive qualified,
the product is not suitable for automotive use. It is neither qualified nor tested
in accordance with automotive testing or application requirements. NXP
Semiconductors accepts no liability for inclusion and/or use of
non-automotive qualified products in automotive equipment or applications.
In the event that customer uses the product for design-in and use in
automotive applications to automotive specifications and standards, customer
(a) shall use the product without NXP Semiconductors’ warranty of the
product for such automotive applications, use and specifications, and (b)
whenever customer uses the product for automotive applications beyond
NXP Semiconductors’ specifications such use shall be solely at customer’s
own risk, and (c) customer fully indemnifies NXP Semiconductors for any
liability, damages or failed product claims resulting from customer design and
use of the product for automotive applications beyond NXP Semiconductors’
standard warranty and NXP Semiconductors’ product specifications.
Translations — A non-English (translated) version of a document is for
reference only. The English version shall prevail in case of any discrepancy
between the translated and English versions.
19.4 Trademarks
Notice: All referenced brands, product names, service names and trademarks
are the property of their respective owners.
UCODE — is a trademark of NXP B.V.
20. Contact information
For more information, please visit: http://www.nxp.com
For sales office addresses, please send an email to: salesaddresses@nxp.com
139037 All information provided in this document is subject to legal disclaimers. © NXP B.V. 2013. All rights reserved.
Product data sheet
COMPANY PUBLIC
Rev. 3.8 — 11 November 2013
139038 46 of 48
NXP Semiconductors SL3ICS1002/1202
UCODE G2XM and G2XL
21. Tables
Table 1. Ordering information G2XM . . . . . . . . . . . . . . . .3
Table 2. Ordering information G2XL. . . . . . . . . . . . . . . . .3
Table 3. Pin description of SOT1122 . . . . . . . . . . . . . . . .7
Table 4. SOT1122 Marking. . . . . . . . . . . . . . . . . . . . . . . .7
Table 5. Limiting values[1][2] . . . . . . . . . . . . . . . . . . . . . .10
Table 6. Wafer characteristics . . . . . . . . . . . . . . . . . . . . 11
Table 7. Package interface characteristics. . . . . . . . . . . 11
Table 8. Symbol description . . . . . . . . . . . . . . . . . . . . . .14
Table 9. Operating distances for UCODE G2X based
tags and labels in released frequency bands . .14
Table 10. G2X memory sections . . . . . . . . . . . . . . . . . . .21
Table 11. Memory map. . . . . . . . . . . . . . . . . . . . . . . . . . .22
Table 12. ReadProtect command. . . . . . . . . . . . . . . . . . .28
Table 13. G2X reply to a successful ReadProtect
procedure . . . . . . . . . . . . . . . . . . . . . . . . . . . . .29
Table 14. ReadProtect command-response table . . . . . .29
Table 15. Reset ReadProtect command . . . . . . . . . . . . .31
Table 16. G2X reply to a successful Reset ReadProtect
command . . . . . . . . . . . . . . . . . . . . . . . . . . . . .31
Table 17. Reset ReadProtect command-response table 31
Table 18. ChangeEAS command . . . . . . . . . . . . . . . . . . 32
Table 19. G2X reply to a successful ChangeEAS
command . . . . . . . . . . . . . . . . . . . . . . . . . . . . . 33
Table 20. ChangeEAS command-response table . . . . . . 33
Table 21. EAS_Alarm command . . . . . . . . . . . . . . . . . . . 34
Table 22. G2X reply to a successful EAS_Alarm
command . . . . . . . . . . . . . . . . . . . . . . . . . . . . . 34
Table 23. Eas_Alarm command-response table. . . . . . . 35
Table 24. Calibrate command . . . . . . . . . . . . . . . . . . . . . 36
Table 25. G2X reply to a successful Calibrate command 36
Table 26. Calibrate command-response table . . . . . . . . . 36
Table 27. Practical example of CRC calculation for a
'Req_RN' command by the reader . . . . . . . . . 37
Table 28. Practical example of CRC calculation for a
'Req_RN' command by the reader. . . . . . . . . . 38
Table 29. Abbreviations . . . . . . . . . . . . . . . . . . . . . . . . . 39
Table 30. Revision history . . . . . . . . . . . . . . . . . . . . . . . . 42
22. Figures
Fig 1. Block diagram of G2X IC . . . . . . . . . . . . . . . . . . . .4
Fig 2. Wafer layout and pinning information . . . . . . . . . .5
Fig 3. Package outline SOT1122 . . . . . . . . . . . . . . . . . . .6
Fig 4. G2X TID memory structure . . . . . . . . . . . . . . . . .21
139037 All information provided in this document is subject to legal disclaimers. © NXP B.V. 2013. All rights reserved.
Product data sheet
COMPANY PUBLIC
Rev. 3.8 — 11 November 2013
139038 47 of 48
continued >>
NXP Semiconductors SL3ICS1002/1202
UCODE G2XM and G2XL
23. Contents
1 General description . . . . . . . . . . . . . . . . . . . . . . 1
2 Features and benefits . . . . . . . . . . . . . . . . . . . . 2
2.1 Key features . . . . . . . . . . . . . . . . . . . . . . . . . . . 2
2.2 Key benefits . . . . . . . . . . . . . . . . . . . . . . . . . . . 2
2.3 Custom commands. . . . . . . . . . . . . . . . . . . . . . 2
3 Applications . . . . . . . . . . . . . . . . . . . . . . . . . . . . 3
4 Ordering information. . . . . . . . . . . . . . . . . . . . . 3
5 Block diagram . . . . . . . . . . . . . . . . . . . . . . . . . . 4
6 Wafer layout and pinning information . . . . . . . 5
6.1 Wafer layout . . . . . . . . . . . . . . . . . . . . . . . . . . . 5
7 Package outline . . . . . . . . . . . . . . . . . . . . . . . . . 6
8 Mechanical specification . . . . . . . . . . . . . . . . . 8
8.1 Wafer specification . . . . . . . . . . . . . . . . . . . . . . 8
8.1.1 Wafer . . . . . . . . . . . . . . . . . . . . . . . . . . . . . . . . 8
8.1.2 Wafer backside . . . . . . . . . . . . . . . . . . . . . . . . . 8
8.1.3 Chip dimensions . . . . . . . . . . . . . . . . . . . . . . . . 8
8.1.4 Passivation on front . . . . . . . . . . . . . . . . . . . . . 8
8.1.5 Au bump . . . . . . . . . . . . . . . . . . . . . . . . . . . . . . 9
8.1.6 Fail die identification . . . . . . . . . . . . . . . . . . . . 9
8.1.7 Map file distribution. . . . . . . . . . . . . . . . . . . . . . 9
9 Limiting values. . . . . . . . . . . . . . . . . . . . . . . . . 10
10 Characteristics. . . . . . . . . . . . . . . . . . . . . . . . . 11
10.1 Wafer characteristics . . . . . . . . . . . . . . . . . . . 11
10.2 Package characteristics . . . . . . . . . . . . . . . . . 11
11 Packing information . . . . . . . . . . . . . . . . . . . . 12
11.1 Wafer . . . . . . . . . . . . . . . . . . . . . . . . . . . . . . . 12
11.2 SOT1122 . . . . . . . . . . . . . . . . . . . . . . . . . . . . 12
12 Functional description . . . . . . . . . . . . . . . . . . 13
12.1 Power transfer . . . . . . . . . . . . . . . . . . . . . . . . 13
12.2 Data transfer . . . . . . . . . . . . . . . . . . . . . . . . . . 13
12.2.1 Reader to G2X Link . . . . . . . . . . . . . . . . . . . . 13
12.2.2 G2X to reader Link . . . . . . . . . . . . . . . . . . . . . 13
12.3 Operating distances . . . . . . . . . . . . . . . . . . . . 14
12.4 Air interface standards . . . . . . . . . . . . . . . . . . 15
13 Physical layer and signaling. . . . . . . . . . . . . . 16
13.1 Reader to G2X communication . . . . . . . . . . . 16
13.1.1 Physical layer . . . . . . . . . . . . . . . . . . . . . . . . . 16
13.1.2 Modulation . . . . . . . . . . . . . . . . . . . . . . . . . . . 16
13.1.3 Data encoding. . . . . . . . . . . . . . . . . . . . . . . . . 16
13.1.4 Data rates . . . . . . . . . . . . . . . . . . . . . . . . . . . . 16
13.1.5 RF envelope for R=>T . . . . . . . . . . . . . . . . . . 16
13.1.6 Interrogator power-up/down waveform. . . . . . 16
13.1.7 Preamble and frame-sync . . . . . . . . . . . . . . . 16
13.2 G2X to reader communication . . . . . . . . . . . . 17
13.2.1 Modulation . . . . . . . . . . . . . . . . . . . . . . . . . . . 17
13.2.2 Data encoding . . . . . . . . . . . . . . . . . . . . . . . . 17
13.2.2.1 FM0 baseband . . . . . . . . . . . . . . . . . . . . . . . . 17
13.2.2.2 FM0 Preamble . . . . . . . . . . . . . . . . . . . . . . . . 17
13.2.2.3 Miller-modulated sub carrier . . . . . . . . . . . . . 17
13.2.2.4 Miller sub carrier preamble . . . . . . . . . . . . . . 17
13.2.3 Data rates . . . . . . . . . . . . . . . . . . . . . . . . . . . 17
13.3 Link timing . . . . . . . . . . . . . . . . . . . . . . . . . . . 18
13.3.1 Regeneration time . . . . . . . . . . . . . . . . . . . . . 18
13.3.2 Start-up time. . . . . . . . . . . . . . . . . . . . . . . . . . 18
13.3.3 Persistence time . . . . . . . . . . . . . . . . . . . . . . 18
13.4 Bit and byte ordering . . . . . . . . . . . . . . . . . . . 18
13.5 Data integrity . . . . . . . . . . . . . . . . . . . . . . . . . 19
13.6 CRC . . . . . . . . . . . . . . . . . . . . . . . . . . . . . . . . 19
14 TAG selection, inventory and access . . . . . . 20
14.1 G2X Memory . . . . . . . . . . . . . . . . . . . . . . . . . 21
14.1.1 Memory map . . . . . . . . . . . . . . . . . . . . . . . . . 22
14.1.1.1 User memory (only G2XM) . . . . . . . . . . . . . . 23
14.1.1.2 Special behavior of user memory address 1Fh 23
14.1.1.3 Supported EPC types . . . . . . . . . . . . . . . . . . 23
14.2 Sessions, selected and inventoried flags. . . . 24
14.2.1 G2X States and slot counter . . . . . . . . . . . . . 24
14.2.2 G2X State Diagram . . . . . . . . . . . . . . . . . . . . 24
14.3 Managing tag populations . . . . . . . . . . . . . . . 24
14.4 Selecting tag populations. . . . . . . . . . . . . . . . 24
14.5 Inventorying tag populations . . . . . . . . . . . . . 24
14.6 Accessing individual tags. . . . . . . . . . . . . . . . 24
14.7 Interrogator commands and tag replies . . . . . 24
14.7.1 Commands. . . . . . . . . . . . . . . . . . . . . . . . . . . 24
14.7.2 State transition tables. . . . . . . . . . . . . . . . . . . 25
14.7.3 Command response tables . . . . . . . . . . . . . . 25
14.7.4 Example data-flow exchange. . . . . . . . . . . . . 25
14.8 Mandatory Select Commands . . . . . . . . . . . . 25
14.8.1 Select . . . . . . . . . . . . . . . . . . . . . . . . . . . . . . . 26
14.9 Mandatory Inventory Commands. . . . . . . . . . 26
14.9.1 Query . . . . . . . . . . . . . . . . . . . . . . . . . . . . . . . 26
14.9.2 QueryAdjust . . . . . . . . . . . . . . . . . . . . . . . . . . 26
14.9.3 QueryRep. . . . . . . . . . . . . . . . . . . . . . . . . . . . 26
14.9.4 ACK . . . . . . . . . . . . . . . . . . . . . . . . . . . . . . . . 26
14.9.5 NAK . . . . . . . . . . . . . . . . . . . . . . . . . . . . . . . . 26
14.10 Mandatory Access Commands . . . . . . . . . . . 27
14.10.1 REQ_RN . . . . . . . . . . . . . . . . . . . . . . . . . . . . 27
14.10.2 READ. . . . . . . . . . . . . . . . . . . . . . . . . . . . . . . 27
14.10.3 WRITE . . . . . . . . . . . . . . . . . . . . . . . . . . . . . . 27
14.10.4 KILL . . . . . . . . . . . . . . . . . . . . . . . . . . . . . . . . 27
14.10.5 LOCK . . . . . . . . . . . . . . . . . . . . . . . . . . . . . . . 27
14.11 Optional Access Command . . . . . . . . . . . . . . 27
14.11.1 Access . . . . . . . . . . . . . . . . . . . . . . . . . . . . . . 27
14.12 Custom Commands . . . . . . . . . . . . . . . . . . . . 28
NXP Semiconductors SL3ICS1002/1202
UCODE G2XM and G2XL
© NXP B.V. 2013. All rights reserved.
For more information, please visit: http://www.nxp.com
For sales office addresses, please send an email to: salesaddresses@nxp.com
Date of release: 11 November 2013
139038
Please be aware that important notices concerning this document and the product(s)
described herein, have been included in section ‘Legal information’.
14.12.1 ReadProtect . . . . . . . . . . . . . . . . . . . . . . . . . . 28
14.12.2 Reset ReadProtect . . . . . . . . . . . . . . . . . . . . . 30
14.12.3 ChangeEAS . . . . . . . . . . . . . . . . . . . . . . . . . . 32
14.12.4 EAS_Alarm . . . . . . . . . . . . . . . . . . . . . . . . . . 34
14.12.5 Calibrate . . . . . . . . . . . . . . . . . . . . . . . . . . . . 36
15 Support information . . . . . . . . . . . . . . . . . . . . 37
15.1 CRC Calculation EXAMPLE . . . . . . . . . . . . . . 37
16 Abbreviations. . . . . . . . . . . . . . . . . . . . . . . . . . 39
17 References . . . . . . . . . . . . . . . . . . . . . . . . . . . . 40
18 Revision history. . . . . . . . . . . . . . . . . . . . . . . . 42
19 Legal information. . . . . . . . . . . . . . . . . . . . . . . 44
19.1 Data sheet status . . . . . . . . . . . . . . . . . . . . . . 44
19.2 Definitions. . . . . . . . . . . . . . . . . . . . . . . . . . . . 44
19.3 Disclaimers . . . . . . . . . . . . . . . . . . . . . . . . . . . 44
19.4 Trademarks. . . . . . . . . . . . . . . . . . . . . . . . . . . 45
20 Contact information. . . . . . . . . . . . . . . . . . . . . 45
21 Tables . . . . . . . . . . . . . . . . . . . . . . . . . . . . . . . . 46
22 Figures . . . . . . . . . . . . . . . . . . . . . . . . . . . . . . . 46
23 Contents . . . . . . . . . . . . . . . . . . . . . . . . . . . . . . 47
1. General description
The LPC11U3x are an ARM Cortex-M0 based, low-cost 32-bit MCU family, designed for
8/16-bit microcontroller applications, offering performance, low power, simple instruction
set and memory addressing together with reduced code size compared to existing 8/16-bit
architectures.
The LPC11U3x operate at CPU frequencies of up to 50 MHz.
Equipped with a highly flexible and configurable full-speed USB 2.0 device controller, the
LPC11U3x brings unparalleled design flexibility and seamless integration to today’s
demanding connectivity solutions.
The peripheral complement of the LPC11U3x includes up to 128 kB of flash memory, up
to 12 kB of SRAM data memory and 4 kB EEPROM, one Fast-mode Plus I2C-bus
interface, one RS-485/EIA-485 USART with support for synchronous mode and smart
card interface, two SSP interfaces, four general purpose counter/timers, a 10-bit ADC,
and up to 54 general purpose I/O pins.
The I/O Handler is a software library-supported hardware engine that can be used to add
performance, connectivity and flexibility to system designs. It is available on the
LPC11U37HFBD64/401. The I/O Handler can emulate serial interfaces such as UART,
I2C, and I2S with no or very low additional CPU load and can off-load the CPU by
performing processing-intensive functions like DMA transfers in hardware. Software
libraries for multiple I/O Handler applications are available on http://www.LPCware.com.
For additional documentation related to the LPC11U3x parts, see Section 15
“References”.
2. Features and benefits
System:
ARM Cortex-M0 processor, running at frequencies of up to 50 MHz.
ARM Cortex-M0 built-in Nested Vectored Interrupt Controller (NVIC).
Non-Maskable Interrupt (NMI) input selectable from several input sources.
System tick timer.
Memory:
Up to 128 kB on-chip flash program memory with sector (4 kB) and page erase
(256 byte) access.
4 kB on-chip EEPROM data memory; byte erasable and byte programmable;
on-chip API support.
Up to 12 kB SRAM data memory.
LPC11U3x
32-bit ARM Cortex-M0 microcontroller; up to 128 kB flash; up
to 12 kB SRAM and 4 kB EEPROM; USB device; USART
Rev. 2.2 — 11 March 2014 Product data sheet
LPC11U3X All information provided in this document is subject to legal disclaimers. © NXP Semiconductors N.V. 2014. All rights reserved.
Product data sheet Rev. 2.2 — 11 March 2014 2 of 77
NXP Semiconductors LPC11U3x
32-bit ARM Cortex-M0 microcontroller
16 kB boot ROM.
In-System Programming (ISP) and In-Application Programming (IAP) via on-chip
bootloader software.
ROM-based USB drivers. Flash updates via USB supported.
ROM-based 32-bit integer division routines.
Debug options:
Standard JTAG (Joint Test Action Group) test interface for BSDL (Boundary Scan
Description Language).
Serial Wire Debug.
Digital peripherals:
Up to 54 General Purpose I/O (GPIO) pins with configurable pull-up/pull-down
resistors, repeater mode, and open-drain mode.
Up to 8 GPIO pins can be selected as edge and level sensitive interrupt sources.
Two GPIO grouped interrupt modules enable an interrupt based on a
programmable pattern of input states of a group of GPIO pins.
High-current source output driver (20 mA) on one pin.
High-current sink driver (20 mA) on true open-drain pins.
Four general purpose counter/timers with a total of up to 8 capture inputs and 13
match outputs.
Programmable Windowed WatchDog Timer (WWDT) with a dedicated, internal
low-power WatchDog Oscillator (WDO).
Analog peripherals:
10-bit ADC with input multiplexing among eight pins.
Serial interfaces:
USB 2.0 full-speed device controller.
USART with fractional baud rate generation, internal FIFO, a full modem control
handshake interface, and support for RS-485/9-bit mode and synchronous mode.
USART supports an asynchronous smart card interface (ISO 7816-3).
Two SSP controllers with FIFO and multi-protocol capabilities.
I2C-bus interface supporting the full I2C-bus specification and Fast-mode Plus with
a data rate of up to 1 Mbit/s with multiple address recognition and monitor mode.
I/O Handler for hardware emulation of serial interfaces and DMA; supported through
software libraries. (LPC11U37HFBD64/401 only.)
Clock generation:
Crystal Oscillator with an operating range of 1 MHz to 25 MHz (system oscillator).
12 MHz high-frequency Internal RC oscillator (IRC) that can optionally be used as
a system clock.
Internal low-power, low-frequency WatchDog Oscillator (WDO) with programmable
frequency output.
PLL allows CPU operation up to the maximum CPU rate with the system oscillator
or the IRC as clock sources.
A second, dedicated PLL is provided for USB.
Clock output function with divider that can reflect the crystal oscillator, the main
clock, the IRC, or the watchdog oscillator.
Power control:
LPC11U3X All information provided in this document is subject to legal disclaimers. © NXP Semiconductors N.V. 2014. All rights reserved.
Product data sheet Rev. 2.2 — 11 March 2014 3 of 77
NXP Semiconductors LPC11U3x
32-bit ARM Cortex-M0 microcontroller
Integrated PMU (Power Management Unit) to minimize power consumption during
Sleep, Deep-sleep, Power-down, and Deep power-down modes.
Power profiles residing in boot ROM provide optimized performance and minimized
power consumption for any given application through one simple function call.
Four reduced power modes: Sleep, Deep-sleep, Power-down, and Deep
power-down.
Processor wake-up from Deep-sleep and Power-down modes via reset, selectable
GPIO pins, watchdog interrupt, or USB port activity.
Processor wake-up from Deep power-down mode using one special function pin.
Power-On Reset (POR).
Brownout detect with up to four separate thresholds for interrupt and forced reset.
Unique device serial number for identification.
Single 3.3 V power supply (1.8 V to 3.6 V).
Temperature range 40 C to +85 C.
Available as LQFP64, LQFP48, TFBGA48, and HVQFN33 packages.
3. Applications
4. Ordering information
Consumer peripherals Handheld scanners
Medical USB audio devices
Industrial control
Table 1. Ordering information
Type number Package
Name Description Version
LPC11U34FHN33/311 HVQFN33 plastic thermal enhanced very thin quad flat package; no leads; 33
terminals; body 7 7 0.85 mm
n/a
LPC11U34FBD48/311 LQFP48 plastic low profile quad flat package; 48 leads; body 7 7 1.4 mm SOT313-2
LPC11U34FHN33/421 HVQFN33 plastic thermal enhanced very thin quad flat package; no leads; 33
terminals; body 7 7 0.85 mm
n/a
LPC11U34FBD48/421 LQFP48 plastic low profile quad flat package; 48 leads; body 7 7 1.4 mm SOT313-2
LPC11U35FHN33/401 HVQFN33 plastic thermal enhanced very thin quad flat package; no leads; 33
terminals; body 7 7 0.85 mm
n/a
LPC11U35FBD48/401 LQFP48 plastic low profile quad flat package; 48 leads; body 7 7 1.4 mm SOT313-2
LPC11U35FBD64/401 LQFP64 plastic low profile quad flat package; 64 leads; body 10 10 1.4 mm SOT314-2
LPC11U35FHI33/501 HVQFN33 plastic thermal enhanced very thin quad flat package; no leads; 33
terminals; body 5 5 0.85 mm
n/a
LPC11U35FET48/501 TFBGA48 plastic thin fine-pitch ball grid array package; 48 balls; body 4.5 4.5
0.7 mm
SOT1155-2
LPC11U36FBD48/401 LQFP48 plastic low profile quad flat package; 48 leads; body 7 7 1.4 mm SOT313-2
LPC11U36FBD64/401 LQFP64 plastic low profile quad flat package; 64 leads; body 10 10 1.4 mm SOT314-2
LPC11U3X All information provided in this document is subject to legal disclaimers. © NXP Semiconductors N.V. 2014. All rights reserved.
Product data sheet Rev. 2.2 — 11 March 2014 4 of 77
NXP Semiconductors LPC11U3x
32-bit ARM Cortex-M0 microcontroller
4.1 Ordering options
[1] For general-purpose use.
[2] For I/O Handler use only.
LPC11U37FBD48/401 LQFP48 plastic low profile quad flat package; 48 leads; body 7 7 1.4 mm SOT313-2
LPC11U37HFBD64/401 LQFP64 plastic low profile quad flat package; 64 leads; body 10 10 1.4 mm SOT314-2
LPC11U37FBD64/501 LQFP64 plastic low profile quad flat package; 64 leads; body 10 10 1.4 mm SOT314-2
Table 1. Ordering information …continued
Type number Package
Name Description Version
Table 2. Ordering options
Type number
Flash in kB
EEPROM in kB
SRAM0 in kB
USB SRAM in kB
SRAM1 in kB
Total
SRAM in kB[1]
I/O Handler
USART
I2C-bus FM+
SSP
USB device
ADC channels
GPIO pins
LPC11U34FHN33/311 40 4 8 - - 8 no 1 1 2 1 8 26
LPC11U34FBD48/311 40 4 8 - - 8 no 1 1 2 1 8 40
LPC11U34FHN33/421 48 4 8 2 - 10 no 1 1 2 1 8 26
LPC11U34FBD48/421 48 4 8 2 - 10 no 1 1 2 1 8 40
LPC11U35FHN33/401 64 4 8 2 - 10 no 1 1 2 1 8 26
LPC11U35FBD48/401 64 4 8 2 - 10 no 1 1 2 1 8 40
LPC11U35FBD64/401 64 4 8 2 - 10 no 1 1 2 1 8 54
LPC11U35FHI33/501 64 4 8 2 2[1] 12 no 1 1 2 1 8 26
LPC11U35FET48/501 64 4 8 2 2[1] 12 no 1 1 2 1 8 40
LPC11U36FBD48/401 96 4 8 2 - 10 no 1 1 2 1 8 40
LPC11U36FBD64/401 96 4 8 2 - 10 no 1 1 2 1 8 54
LPC11U37FBD48/401 128 4 8 2 - 10 no 1 1 2 1 8 40
LPC11U37HFBD64/401 128 4 8 2 2[2] 10 yes 1 1 2 1 8 54
LPC11U37FBD64/501 128 4 8 2 2[1] 12 no 1 1 2 1 8 54
LPC11U3X All information provided in this document is subject to legal disclaimers. © NXP Semiconductors N.V. 2014. All rights reserved.
Product data sheet Rev. 2.2 — 11 March 2014 5 of 77
NXP Semiconductors LPC11U3x
32-bit ARM Cortex-M0 microcontroller
5. Block diagram
(1) Not available on HVQFN33 packages.
(2) CT16B0_CAP1, CT16B1_CAP1 available on LQFP64 packages only; CT32B0_CAP1 available on TFBGA48, LQFP48, and
LQFP64 packages only; CT32B1_CAP1 available in TFBGA48/LQFP64 packages only.
(3) LPC11U37HFBD64/401 only.
Fig 1. Block diagram
SRAM
8/10/12 kB
ARM
CORTEX-M0
TEST/DEBUG
INTERFACE
FLASH
40/48/64/96/128 kB
HIGH-SPEED
GPIO
AHB TO APB
BRIDGE
CLOCK
GENERATION,
POWER CONTROL,
SYSTEM
FUNCTIONS
RESET
SWD, JTAG
LPC11U3x
slave
slave
master
slave slave
ROM
16 kB
slave
AHB-LITE BUS
GPIO ports 0/1
I/O
IOH_[20:0] HANDLER(3)
CLKOUT
IRC, WDO
SYSTEM OSCILLATOR
POR
PLL0 USB PLL
BOD
10-bit ADC
USART/
SMARTCARD INTERFACE AD[7:0]
RXD
TXD
CTS, RTS, DTR
SCLK
GPIO INTERRUPTS
32-bit COUNTER/TIMER 0
CT32B0_MAT[3:0]
CT32B0_CAP[1:0](2)
32-bit COUNTER/TIMER 1
CT32B1_MAT[3:0]
CT32B1_CAP[1:0](2)
DCD, DSR(1), RI(1)
16-bit COUNTER/TIMER 1
WINDOWED WATCHDOG
TIMER
GPIO GROUP0 INTERRUPTS
CT16B1_MAT[1:0]
16-bit COUNTER/TIMER 0
CT16B0_MAT[2:0]
CT16B0_CAP[1:0](2)
CT16B1_CAP[1:0](2)
GPIO pins
GPIO pins
GPIO pins GPIO GROUP1 INTERRUPTS
system bus
SSP0
SCK0, SSEL0,
MISO0, MOSI0
SSP1
SCK1, SSEL1,
MISO1, MOSI1
I2C-BUS
IOCON
SYSTEM CONTROL
PMU
SCL, SDA
XTALIN XTALOUT
USB DEVICE
CONTROLLER
USB_DP
USB_DM
USB_VBUS
USB_FTOGGLE,
USB_CONNECT
002aag345
master
slave
EEPROM
4 kB
LPC11U3X All information provided in this document is subject to legal disclaimers. © NXP Semiconductors N.V. 2014. All rights reserved.
Product data sheet Rev. 2.2 — 11 March 2014 6 of 77
NXP Semiconductors LPC11U3x
32-bit ARM Cortex-M0 microcontroller
6. Pinning information
6.1 Pinning
For parts LPC11U34FHN33/311, LPC11U34FHN33/421, LPC11U35FHN33/401, LPC11U35FHI33/501
Fig 2. Pin configuration (HVQFN33)
002aag809
Transparent top view
PIO0_8/MISO0/CT16B0_MAT0
PIO0_20/CT16B1_CAP0
PIO0_2/SSEL0/CT16B0_CAP0
PIO0_9/MOSI0/CT16B0_MAT1
VDD SWCLK/PIO0_10/SCK0/CT16B0_MAT2
XTALOUT PIO0_22/AD6/CT16B1_MAT1/MISO1
XTALIN TDI/PIO0_11/AD0/CT32B0_MAT3
PIO0_1/CLKOUT/CT32B0_MAT2/USB_FTOGGLE TMS/PIO0_12/AD1/CT32B1_CAP0
RESET/PIO0_0 TDO/PIO0_13/AD2/CT32B1_MAT0
PIO1_19/DTR/SSEL1 TRST/PIO0_14/AD3/CT32B1_MAT1
PIO0_3/USB_VBUS
PIO0_4/SCL
PIO0_5/SDA
PIO0_21/CT16B1_MAT0/MOSI1
USB_DM
USB_DP
PIO0_6/USB_CONNECT/SCK0
PIO0_7/CTS
PIO0_19/TXD/CT32B0_MAT1
PIO0_18/RXD/CT32B0_MAT0
PIO0_17/RTS/CT32B0_CAP0/SCLK
VDD
PIO1_15/DCD/CT16B0_MAT2/SCK1
PIO0_23/AD7
PIO0_16/AD5/CT32B1_MAT3/WAKEUP
SWDIO/PIO0_15/AD4/CT32B1_MAT2
8 17
7 18
6 19
5 20
4 21
3 22
2 23
1 24
9
10
11
12
13
14
15
16
32
31
30
29
28
27
26
25
terminal 1
index area
33 VSS
LPC11U3X All information provided in this document is subject to legal disclaimers. © NXP Semiconductors N.V. 2014. All rights reserved.
Product data sheet Rev. 2.2 — 11 March 2014 7 of 77
NXP Semiconductors LPC11U3x
32-bit ARM Cortex-M0 microcontroller
Fig 3. Pin configuration (TFBGA48)
002aag810
LPC11U35FET48/501
Transparent top view
H
G
F
D
B
E
C
A
1 2 3 4 5 6 7 8
ball A1
index area
LPC11U3X All information provided in this document is subject to legal disclaimers. © NXP Semiconductors N.V. 2014. All rights reserved.
Product data sheet Rev. 2.2 — 11 March 2014 8 of 77
NXP Semiconductors LPC11U3x
32-bit ARM Cortex-M0 microcontroller
Fig 4. Pin configuration (LQFP48)
LPC11U34FBD48/311
LPC11U34FBD48/421
LPC11U35FBD48/401
LPC11U36FBD48/401
LPC11U37FBD48/401
PIO1_25/CT32B0_MAT1 PIO1_13/DTR/CT16B0_MAT0/TXD
PIO1_19/DTR/SSEL1 TRST/PIO0_14/AD3/CT32B1_MAT1
RESET/PIO0_0 TDO/PIO0_13/AD2/CT32B1_MAT0
PIO0_1/CLKOUT/CT32B0_MAT2/USB_FTOGGLE TMS/PIO0_12/AD1/CT32B1_CAP0
VSS TDI/PIO0_11/AD0/CT32B0_MAT3
XTALIN PIO1_29/SCK0/CT32B0_CAP1
XTALOUT PIO0_22/AD6/CT16B1_MAT1/MISO1
VDD SWCLK/PIO0_10/SCK0/CT16B0_MAT2
PIO0_20/CT16B1_CAP0 PIO0_9/MOSI0/CT16B0_MAT1
PIO0_2/SSEL0/CT16B0_CAP0 PIO0_8/MISO0/CT16B0_MAT0
PIO1_26/CT32B0_MAT2/RXD PIO1_21/DCD/MISO1
PIO1_27/CT32B0_MAT3/TXD PIO1_31
PIO1_20/DSR/SCK1 PIO1_16/RI/CT16B0_CAP0
PIO0_3/USB_VBUS PIO0_19/TXD/CT32B0_MAT1
PIO0_4/SCL PIO0_18/RXD/CT32B0_MAT0
PIO0_5/SDA PIO0_17/RTS/CT32B0_CAP0/SCLK
PIO0_21/CT16B1_MAT0/MOSI1 VDD
PIO1_23/CT16B1_MAT1/SSEL1 PIO1_15/DCD/CT16B0_MAT2/SCK1
USB_DM PIO0_23/AD7
USB_DP VSS
PIO1_24/CT32B0_MAT0 PIO0_16/AD5/CT32B1_MAT3/WAKEUP
PIO0_6/USB_CONNECT/SCK0 SWDIO/PIO0_15/AD4/CT32B1_MAT2
PIO0_7/CTS
PIO1_28/CT32B0_CAP0/SCLK
PIO1_22/RI/MOSI1
PIO1_14/DSR/CT16B0_MAT1/RXD
002aag811
1
2
3
4
5
6
7
8
9
10
11
12
36
35
34
33
32
31
30
29
28
27
26
25
13
14
15
16
17
18
19
20
21
22
23
48
47
46
45
44
43
42
41
40
39
38
24 37
LPC11U3X All information provided in this document is subject to legal disclaimers. © NXP Semiconductors N.V. 2014. All rights reserved.
Product data sheet Rev. 2.2 — 11 March 2014 9 of 77
NXP Semiconductors LPC11U3x
32-bit ARM Cortex-M0 microcontroller
See Table 3 for the full pin name.
Fig 5. Pin configuration (LQFP64)
LPC11U35FBD64/401
LPC11U36FBD64/401
LPC11U37HFBD64/401
LPC11U37FBD64/501
PIO1_0 VDD
PIO1_25 PIO1_13
PIO1_19 TRST/PIO0_14
RESET/PIO0_0 TDO/PIO0_13
PIO0_1 TMS/PIO0_12
PIO1_7 PIO1_11
VSS TDI/PIO0_11
XTALIN PIO1_29
XTALOUT PIO0_22
VDD PIO1_8
PIO0_20 SWCLK/PIO0_10
PIO1_10 PIO0_9
PIO0_2 PIO0_8
PIO1_26 PIO1_21
PIO1_27 PIO1_2
PIO1_4 VDD
PIO1_1 PIO1_6
PIO1_20 PIO1_16
PIO0_3 PIO0_19
PIO0_4 PIO0_18
PIO0_5 PIO0_17
PIO0_21 PIO1_12
PIO1_17 VDD
PIO1_23 PIO1_15
USB_DM PIO0_23
USB_DP PIO1_9
PIO1_24 VSS
PIO1_18 PIO0_16
PIO0_6 SWDIO/PIO0_15
PIO0_7 PIO1_22
PIO1_28 PIO1_3
PIO1_5 PIO1_14
002aag812
1
2
3
4
5
6
7
8
9
10
11
12
13
14
15
16
48
47
46
45
44
43
42
41
40
39
38
37
36
35
34
33
17
18
19
20
21
22
23
24
25
26
27
28
29
30
31
32
64
63
62
61
60
59
58
57
56
55
54
53
52
51
50
49
LPC11U3X All information provided in this document is subject to legal disclaimers. © NXP Semiconductors N.V. 2014. All rights reserved.
Product data sheet Rev. 2.2 — 11 March 2014 10 of 77
NXP Semiconductors LPC11U3x
32-bit ARM Cortex-M0 microcontroller
6.2 Pin description
Table 3 shows all pins and their assigned digital or analog functions in order of the GPIO
port number. The default function after reset is listed first. All port pins have internal
pull-up resistors enabled after reset except for the true open-drain pins PIO0_4 and
PIO0_5.
Every port pin has a corresponding IOCON register for programming the digital or analog
function, the pull-up/pull-down configuration, the repeater, and the open-drain modes.
The USART, counter/timer, and SSP functions are available on more than one port pin.
Table 3. Pin description
Symbol
Pin HVQFN33
Pin TFBGA48
Pin LQFP48
Pin LQFP64
Reset
state
[1]
Type Description
RESET/PIO0_0 2 C1 3 4 [2] I; PU I RESET — External reset input with 20 ns glitch filter.
A LOW-going pulse as short as 50 ns on this pin
resets the device, causing I/O ports and peripherals to
take on their default states and processor execution
to begin at address 0. This pin also serves as the
debug select input. LOW level selects the JTAG
boundary scan. HIGH level selects the ARM SWD
debug mode.
In deep power-down mode, this pin must be pulled
HIGH externally. The RESET pin can be left
unconnected or be used as a GPIO pin if an external
RESET function is not needed and Deep power-down
mode is not used.
- I/O PIO0_0 — General purpose digital input/output pin.
PIO0_1/CLKOUT/
CT32B0_MAT2/
USB_FTOGGLE
3 C2 4 5 [3] I; PU I/O PIO0_1 — General purpose digital input/output pin. A
LOW level on this pin during reset starts the ISP
command handler or the USB device enumeration.
- O CLKOUT — Clockout pin.
- O CT32B0_MAT2 — Match output 2 for 32-bit timer 0.
- O USB_FTOGGLE — USB 1 ms Start-of-Frame signal.
PIO0_2/SSEL0/
CT16B0_CAP0/IOH_0
8 F1 10 13 [3] I; PU I/O PIO0_2 — General purpose digital input/output pin.
- I/O SSEL0 — Slave select for SSP0.
- I CT16B0_CAP0 — Capture input 0 for 16-bit timer 0.
- I/O IOH_0 — I/O Handler input/output 0.
LPC11U37HFBD64/401 only.
PIO0_3/USB_VBUS/
IOH_1
9 H2 14 19 [3] I; PU I/O PIO0_3 — General purpose digital input/output pin. A
LOW level on this pin during reset starts the ISP
command handler. A HIGH level during reset starts
the USB device enumeration.
- I USB_VBUS — Monitors the presence of USB bus
power.
- I/O IOH_1 — I/O Handler input/output 1.
LPC11U37HFBD64/401 only.
LPC11U3X All information provided in this document is subject to legal disclaimers. © NXP Semiconductors N.V. 2014. All rights reserved.
Product data sheet Rev. 2.2 — 11 March 2014 11 of 77
NXP Semiconductors LPC11U3x
32-bit ARM Cortex-M0 microcontroller
PIO0_4/SCL/IOH_2 10 G3 15 20 [4] I; IA I/O PIO0_4 — General purpose digital input/output pin
(open-drain).
- I/O SCL — I2C-bus clock input/output (open-drain).
High-current sink only if I2C Fast-mode Plus is
selected in the I/O configuration register.
- I/O IOH_2 — I/O Handler input/output 2.
LPC11U37HFBD64/401 only.
PIO0_5/SDA/IOH_3 11 H3 16 21 [4] I; IA I/O PIO0_5 — General purpose digital input/output pin
(open-drain).
- I/O SDA — I2C-bus data input/output (open-drain).
High-current sink only if I2C Fast-mode Plus is
selected in the I/O configuration register.
- I/O IOH_3 — I/O Handler input/output 3.
LPC11U37HFBD64/401 only.
PIO0_6/USB_CONNECT/
SCK0/IOH_4
15 H6 22 29 [3] I; PU I/O PIO0_6 — General purpose digital input/output pin.
- O USB_CONNECT — Signal used to switch an external
1.5 k resistor under software control. Used with the
SoftConnect USB feature.
- I/O SCK0 — Serial clock for SSP0.
- I/O IOH_4 — I/O Handler input/output 4.
LPC11U37HFBD64/401 only.
PIO0_7/CTS/IOH_5 16 G7 23 30 [5] I; PU I/O PIO0_7 — General purpose digital input/output pin
(high-current output driver).
- I CTS — Clear To Send input for USART.
- I/O IOH_5 — I/O Handler input/output 5.
(LPC11U37HFBD64/401 only.)
PIO0_8/MISO0/
CT16B0_MAT0/R/IOH_6
17 F8 27 36 [3] I; PU I/O PIO0_8 — General purpose digital input/output pin.
- I/O MISO0 — Master In Slave Out for SSP0.
- O CT16B0_MAT0 — Match output 0 for 16-bit timer 0.
- - Reserved.
- I/O IOH_6 — I/O Handler input/output 6.
(LPC11U37HFBD64/401 only.)
PIO0_9/MOSI0/
CT16B0_MAT1/R/IOH_7
18 F7 28 37 [3] I; PU I/O PIO0_9 — General purpose digital input/output pin.
- I/O MOSI0 — Master Out Slave In for SSP0.
- O CT16B0_MAT1 — Match output 1 for 16-bit timer 0.
- - Reserved.
- I/O IOH_7 — I/O Handler input/output 7.
(LPC11U37HFBD64/401 only.)
Table 3. Pin description
Symbol
Pin HVQFN33
Pin TFBGA48
Pin LQFP48
Pin LQFP64
Reset
state
[1]
Type Description
LPC11U3X All information provided in this document is subject to legal disclaimers. © NXP Semiconductors N.V. 2014. All rights reserved.
Product data sheet Rev. 2.2 — 11 March 2014 12 of 77
NXP Semiconductors LPC11U3x
32-bit ARM Cortex-M0 microcontroller
SWCLK/PIO0_10/SCK0/
CT16B0_MAT2
19 E7 29 38 [3] I; PU I SWCLK — Serial wire clock and test clock TCK for
JTAG interface.
- I/O PIO0_10 — General purpose digital input/output pin.
- O SCK0 — Serial clock for SSP0.
- O CT16B0_MAT2 — Match output 2 for 16-bit timer 0.
TDI/PIO0_11/AD0/
CT32B0_MAT3
21 D8 32 42 [6] I; PU I TDI — Test Data In for JTAG interface.
- I/O PIO0_11 — General purpose digital input/output pin.
- I AD0 — A/D converter, input 0.
- O CT32B0_MAT3 — Match output 3 for 32-bit timer 0.
TMS/PIO0_12/AD1/
CT32B1_CAP0
22 C7 33 44 [6] I; PU I TMS — Test Mode Select for JTAG interface.
- I/O PIO_12 — General purpose digital input/output pin.
- I AD1 — A/D converter, input 1.
- I CT32B1_CAP0 — Capture input 0 for 32-bit timer 1.
TDO/PIO0_13/AD2/
CT32B1_MAT0
23 C8 34 45 [6] I; PU O TDO — Test Data Out for JTAG interface.
- I/O PIO0_13 — General purpose digital input/output pin.
- I AD2 — A/D converter, input 2.
- O CT32B1_MAT0 — Match output 0 for 32-bit timer 1.
TRST/PIO0_14/AD3/
CT32B1_MAT1
24 B7 35 46 [6] I; PU I TRST — Test Reset for JTAG interface.
- I/O PIO0_14 — General purpose digital input/output pin.
- I AD3 — A/D converter, input 3.
- O CT32B1_MAT1 — Match output 1 for 32-bit timer 1.
SWDIO/PIO0_15/AD4/
CT32B1_MAT2
25 B6 39 52 [6] I; PU I/O SWDIO — Serial wire debug input/output.
- I/O PIO0_15 — General purpose digital input/output pin.
- I AD4 — A/D converter, input 4.
- O CT32B1_MAT2 — Match output 2 for 32-bit timer 1.
PIO0_16/AD5/
CT32B1_MAT3/IOH_8/
WAKEUP
26 A6 40 53 [6] I; PU I/O PIO0_16 — General purpose digital input/output pin.
- I AD5 — A/D converter, input 5.
- O CT32B1_MAT3 — Match output 3 for 32-bit timer 1.
- I/O IOH_8 — I/O Handler input/output 8.
(LPC11U37HFBD64/401 only.)
- I WAKEUP — Deep power-down mode wake-up pin
with 20 ns glitch filter. Pull this pin HIGH externally
before entering Deep power-down mode, then pull
LOW to exit Deep power-down mode. A LOW-going
pulse as short as 50 ns wakes up the part.
Table 3. Pin description
Symbol
Pin HVQFN33
Pin TFBGA48
Pin LQFP48
Pin LQFP64
Reset
state
[1]
Type Description
LPC11U3X All information provided in this document is subject to legal disclaimers. © NXP Semiconductors N.V. 2014. All rights reserved.
Product data sheet Rev. 2.2 — 11 March 2014 13 of 77
NXP Semiconductors LPC11U3x
32-bit ARM Cortex-M0 microcontroller
PIO0_17/RTS/
CT32B0_CAP0/SCLK
30 A3 45 60 [3] I; PU I/O PIO0_17 — General purpose digital input/output pin.
- O RTS — Request To Send output for USART.
- I CT32B0_CAP0 — Capture input 0 for 32-bit timer 0.
- I/O SCLK — Serial clock input/output for USART in
synchronous mode.
PIO0_18/RXD/
CT32B0_MAT0
31 B3 46 61 [3] I; PU I/O PIO0_18 — General purpose digital input/output pin.
- I RXD — Receiver input for USART. Used in UART ISP
mode.
- O CT32B0_MAT0 — Match output 0 for 32-bit timer 0.
PIO0_19/TXD/
CT32B0_MAT1
32 B2 47 62 [3] I; PU I/O PIO0_19 — General purpose digital input/output pin.
- O TXD — Transmitter output for USART. Used in UART
ISP mode.
- O CT32B0_MAT1 — Match output 1 for 32-bit timer 0.
PIO0_20/CT16B1_CAP0 7 F2 9 11 [3] I; PU I/O PIO0_20 — General purpose digital input/output pin.
- I CT16B1_CAP0 — Capture input 0 for 16-bit timer 1.
PIO0_21/CT16B1_MAT0/
MOSI1
12 G4 17 22 [3] I; PU I/O PIO0_21 — General purpose digital input/output pin.
- O CT16B1_MAT0 — Match output 0 for 16-bit timer 1.
- I/O MOSI1 — Master Out Slave In for SSP1.
PIO0_22/AD6/
CT16B1_MAT1/MISO1
20 E8 30 40 [6] I; PU I/O PIO0_22 — General purpose digital input/output pin.
- I AD6 — A/D converter, input 6.
- O CT16B1_MAT1 — Match output 1 for 16-bit timer 1.
- I/O MISO1 — Master In Slave Out for SSP1.
PIO0_23/AD7/IOH_9 27 A5 42 56 [6] I; PU I/O PIO0_23 — General purpose digital input/output pin.
- I AD7 — A/D converter, input 7.
- I/O IOH_9 — I/O Handler input/output 9.
(LPC11U37HFBD64/401 only.)
PIO1_0/CT32B1_MAT0/
IOH_10
- - - 1 [3] I; PU I/O PIO1_0 — General purpose digital input/output pin.
- O CT32B1_MAT0 — Match output 0 for 32-bit timer 1.
- I/O IOH_10 — I/O Handler input/output 10.
(LPC11U37HFBD64/401 only.)
PIO1_1/CT32B1_MAT1/
IOH_11
- - - 17 [3] I; PU I/O PIO1_1 — General purpose digital input/output pin.
- O CT32B1_MAT1 — Match output 1 for 32-bit timer 1.
- I/O IOH_11 — I/O Handler input/output 11.
(LPC11U37HFBD64/401 only.)
PIO1_2/CT32B1_MAT2/
IOH_12
- - - 34 [3] I; PU I/O PIO1_2 — General purpose digital input/output pin.
- O CT32B1_MAT2 — Match output 2 for 32-bit timer 1.
- I/O IOH_12 — I/O Handler input/output 12.
(LPC11U37HFBD64/401 only.)
Table 3. Pin description
Symbol
Pin HVQFN33
Pin TFBGA48
Pin LQFP48
Pin LQFP64
Reset
state
[1]
Type Description
LPC11U3X All information provided in this document is subject to legal disclaimers. © NXP Semiconductors N.V. 2014. All rights reserved.
Product data sheet Rev. 2.2 — 11 March 2014 14 of 77
NXP Semiconductors LPC11U3x
32-bit ARM Cortex-M0 microcontroller
PIO1_3/CT32B1_MAT3/
IOH_13
- - - 50 [3] I; PU I/O PIO1_3 — General purpose digital input/output pin.
- O CT32B1_MAT3 — Match output 3 for 32-bit timer 1.
- I/O IOH_13 — I/O Handler input/output 13.
(LPC11U37HFBD64/401 only.)
PIO1_4/CT32B1_CAP0/
IOH_14
- - - 16 [3] I; PU I/O PIO1_4 — General purpose digital input/output pin.
- I CT32B1_CAP0 — Capture input 0 for 32-bit timer 1.
- I/O IOH_14 — I/O Handler input/output 14.
(LPC11U37HFBD64/401 only.)
PIO1_5/CT32B1_CAP1
/IOH_15
- H8 - 32 [3] I; PU I/O PIO1_5 — General purpose digital input/output pin.
- I CT32B1_CAP1 — Capture input 1 for 32-bit timer 1.
- I/O IOH_15 — I/O Handler input/output 15.
(LPC11U37HFBD64/401 only.)
PIO1_6/IOH_16 - - - 64 [3] I; PU I/O PIO1_6 — General purpose digital input/output pin.
- I/O IOH_16 — I/O Handler input/output 16.
(LPC11U37HFBD64/401 only.)
PIO1_7/IOH_17 - - - 6 [3] I; PU I/O PIO1_7 — General purpose digital input/output pin.
- I/O IOH_17 — I/O Handler input/output 17.
(LPC11U37HFBD64/401 only.)
PIO1_8/IOH_18 - - - 39 [3] I; PU I/O PIO1_8 — General purpose digital input/output pin.
- I/O IOH_18 — I/O Handler input/output 18.
(LPC11U37HFBD64/401 only.)
PIO1_9 - - - 55 [3] I; PU I/O PIO1_9 — General purpose digital input/output pin.
PIO1_10 - - - 12 [3] I; PU I/O PIO1_10 — General purpose digital input/output pin.
PIO1_11 - - - 43 [3] I; PU I/O PIO1_11 — General purpose digital input/output pin.
PIO1_12 - - - 59 [3] I; PU I/O PIO1_12 — General purpose digital input/output pin.
PIO1_13/DTR/
CT16B0_MAT0/TXD
- B8 36 47 [3] I; PU I/O PIO1_13 — General purpose digital input/output pin.
- O DTR — Data Terminal Ready output for USART.
- O CT16B0_MAT0 — Match output 0 for 16-bit timer 0.
- O TXD — Transmitter output for USART.
PIO1_14/DSR/
CT16B0_MAT1/RXD
- A8 37 49 [3] I; PU I/O PIO1_14 — General purpose digital input/output pin.
- I DSR — Data Set Ready input for USART.
- O CT16B0_MAT1 — Match output 1 for 16-bit timer 0.
- I RXD — Receiver input for USART.
PIO1_15/DCD/
CT16B0_MAT2/SCK1
28 A4 43 57 [3] I; PU I/O PIO1_15 — General purpose digital input/output pin.
I DCD — Data Carrier Detect input for USART.
- O CT16B0_MAT2 — Match output 2 for 16-bit timer 0.
- I/O SCK1 — Serial clock for SSP1.
Table 3. Pin description
Symbol
Pin HVQFN33
Pin TFBGA48
Pin LQFP48
Pin LQFP64
Reset
state
[1]
Type Description
LPC11U3X All information provided in this document is subject to legal disclaimers. © NXP Semiconductors N.V. 2014. All rights reserved.
Product data sheet Rev. 2.2 — 11 March 2014 15 of 77
NXP Semiconductors LPC11U3x
32-bit ARM Cortex-M0 microcontroller
PIO1_16/RI/
CT16B0_CAP0
- A2 48 63 [3] I; PU I/O PIO1_16 — General purpose digital input/output pin.
- I RI — Ring Indicator input for USART.
- I CT16B0_CAP0 — Capture input 0 for 16-bit timer 0.
PIO1_17/CT16B0_CAP1/
RXD
- - - 23 [3] I; PU I/O PIO1_17 — General purpose digital input/output pin.
- I CT16B0_CAP1 — Capture input 1 for 16-bit timer 0.
- I RXD — Receiver input for USART.
PIO1_18/CT16B1_CAP1/
TXD
- - - 28 [3] I; PU I/O PIO1_18 — General purpose digital input/output pin.
- I CT16B1_CAP1 — Capture input 1 for 16-bit timer 1.
- O TXD — Transmitter output for USART.
PIO1_19/DTR/SSEL1 1 B1 2 3 [3] I; PU I/O PIO1_19 — General purpose digital input/output pin.
- O DTR — Data Terminal Ready output for USART.
- I/O SSEL1 — Slave select for SSP1.
PIO1_20/DSR/SCK1 - H1 13 18 [3] I; PU I/O PIO1_20 — General purpose digital input/output pin.
- I DSR — Data Set Ready input for USART.
- I/O SCK1 — Serial clock for SSP1.
PIO1_21/DCD/MISO1 - G8 26 35 [3] I; PU I/O PIO1_21 — General purpose digital input/output pin.
- I DCD — Data Carrier Detect input for USART.
- I/O MISO1 — Master In Slave Out for SSP1.
PIO1_22/RI/MOSI1 - A7 38 51 [3] I; PU I/O PIO1_22 — General purpose digital input/output pin.
- I RI — Ring Indicator input for USART.
- I/O MOSI1 — Master Out Slave In for SSP1.
PIO1_23/CT16B1_MAT1/
SSEL1
- H4 18 24 [3] I; PU I/O PIO1_23 — General purpose digital input/output pin.
- O CT16B1_MAT1 — Match output 1 for 16-bit timer 1.
- I/O SSEL1 — Slave select for SSP1.
PIO1_24/CT32B0_MAT0 - G6 21 27 [3] I; PU I/O PIO1_24 — General purpose digital input/output pin.
- O CT32B0_MAT0 — Match output 0 for 32-bit timer 0.
PIO1_25/CT32B0_MAT1 - A1 1 2 [3] I; PU I/O PIO1_25 — General purpose digital input/output pin.
- O CT32B0_MAT1 — Match output 1 for 32-bit timer 0.
PIO1_26/CT32B0_MAT2/
RXD/IOH_19
- G2 11 14 [3] I; PU I/O PIO1_26 — General purpose digital input/output pin.
- O CT32B0_MAT2 — Match output 2 for 32-bit timer 0.
- I RXD — Receiver input for USART.
- I/O IOH_19 — I/O Handler input/output 19.
(LPC11U37HFBD64/401 only.)
PIO1_27/CT32B0_MAT3/
TXD/IOH_20
- G1 12 15 [3] I; PU I/O PIO1_27 — General purpose digital input/output pin.
- O CT32B0_MAT3 — Match output 3 for 32-bit timer 0.
- O TXD — Transmitter output for USART.
- I/O IOH_20 — I/O Handler input/output 20.
(LPC11U37HFBD64/401 only.)
Table 3. Pin description
Symbol
Pin HVQFN33
Pin TFBGA48
Pin LQFP48
Pin LQFP64
Reset
state
[1]
Type Description
LPC11U3X All information provided in this document is subject to legal disclaimers. © NXP Semiconductors N.V. 2014. All rights reserved.
Product data sheet Rev. 2.2 — 11 March 2014 16 of 77
NXP Semiconductors LPC11U3x
32-bit ARM Cortex-M0 microcontroller
[1] Pin state at reset for default function: I = Input; O = Output; PU = internal pull-up enabled; IA = inactive, no pull-up/down enabled;
F = floating; If the pins are not used, tie floating pins to ground or power to minimize power consumption.
[2] 5 V tolerant pad. RESET functionality is not available in Deep power-down mode. Use the WAKEUP pin to reset the chip and wake up
from Deep power-down mode. An external pull-up resistor is required on this pin for the Deep power-down mode. See Figure 32 for the
reset pad configuration.
[3] 5 V tolerant pad providing digital I/O functions with configurable pull-up/pull-down resistors and configurable hysteresis (see Figure 31).
[4] I2C-bus pin compliant with the I2C-bus specification for I2C standard mode, I2C Fast-mode, and I2C Fast-mode Plus. The pin requires an
external pull-up to provide output functionality. When power is switched off, this pin is floating and does not disturb the I2C lines.
Open-drain configuration applies to all functions on this pin.
[5] 5 V tolerant pad providing digital I/O functions with configurable pull-up/pull-down resistors and configurable hysteresis (see Figure 31);
includes high-current output driver.
[6] 5 V tolerant pad providing digital I/O functions with configurable pull-up/pull-down resistors, configurable hysteresis, and analog input.
When configured as a ADC input, digital section of the pad is disabled and the pin is not 5 V tolerant (see Figure 31); includes digital
input glitch filter.
[7] Pad provides USB functions. It is designed in accordance with the USB specification, revision 2.0 (Full-speed and Low-speed mode
only). This pad is not 5 V tolerant.
[8] When the system oscillator is not used, connect XTALIN and XTALOUT as follows: XTALIN can be left floating or can be grounded
(grounding is preferred to reduce susceptibility to noise). Leave XTALOUT floating.
PIO1_28/CT32B0_CAP0/
SCLK
- H7 24 31 [3] I; PU I/O PIO1_28 — General purpose digital input/output pin.
- I CT32B0_CAP0 — Capture input 0 for 32-bit timer 0.
- I/O SCLK — Serial clock input/output for USART in
synchronous mode.
PIO1_29/SCK0/
CT32B0_CAP1
- D7 31 41 [3] I; PU I/O PIO1_29 — General purpose digital input/output pin.
- I/O SCK0 — Serial clock for SSP0.
- I CT32B0_CAP1 — Capture input 1 for 32-bit timer 0.
PIO1_31 - - 25 - [3] I; PU I/O PIO1_31 — General purpose digital input/output pin.
USB_DM 13 G5 19 25 [7] F - USB_DM — USB bidirectional D line.
USB_DP 14 H5 20 26 [7] F - USB_DP — USB bidirectional D+ line.
XTALIN 4 D1 6 8 [8] - - Input to the oscillator circuit and internal clock
generator circuits. Input voltage must not exceed
1.8 V.
XTALOUT 5 E1 7 9 [8] - - Output from the oscillator amplifier.
VDD 6;
29
B4;
E2
8;
44
10;
33;
48;
58
- - Supply voltage to the internal regulator, the external
rail, and the ADC. Also used as the ADC reference
voltage.
VSS 33 B5;
D2
5;
41
7;
54
- - Ground.
Table 3. Pin description
Symbol
Pin HVQFN33
Pin TFBGA48
Pin LQFP48
Pin LQFP64
Reset
state
[1]
Type Description
LPC11U3X All information provided in this document is subject to legal disclaimers. © NXP Semiconductors N.V. 2014. All rights reserved.
Product data sheet Rev. 2.2 — 11 March 2014 17 of 77
NXP Semiconductors LPC11U3x
32-bit ARM Cortex-M0 microcontroller
7. Functional description
7.1 On-chip flash programming memory
The LPC11U3x contain up to 128 kB on-chip flash program memory. The flash can be
programmed using In-System Programming (ISP) or In-Application Programming (IAP)
via the on-chip boot loader software.
The flash memory is divided into 4 kB sectors with each sector consisting of 16 pages.
Individual pages can be erased using the IAP erase page command.
7.2 EEPROM
The LPC11U3x contain 4 kB of on-chip byte-erasable and byte-programmable EEPROM
data memory. The EEPROM can be programmed using In-Application Programming (IAP)
via the on-chip boot loader software.
7.3 SRAM
The LPC11U3x contain a total of 8 kB, 10 kB, or 12 kB on-chip static RAM memory.
On the LPC11U37HFBD64/401, the 2 kB SRAM1 region at location 0x2000 0000 to
0x2000 07FFF is used for the I/O Handler software library. Do not use this memory
location for data or other user code.
7.4 On-chip ROM
The on-chip ROM contains the boot loader and the following Application Programming
Interfaces (APIs):
• In-System Programming (ISP) and In-Application Programming (IAP) support for flash
including IAP erase page command.
• IAP support for EEPROM
• USB API
• Power profiles for configuring power consumption and PLL settings
• 32-bit integer division routines
7.5 Memory map
The LPC11U3x incorporates several distinct memory regions, shown in the following
figures. Figure 6 shows the overall map of the entire address space from the user
program viewpoint following reset. The interrupt vector area supports address remapping.
The AHB (Advanced High-performance Bus) peripheral area is 2 MB in size and is divided
to allow for up to 128 peripherals. The APB (Advanced Peripheral Bus) peripheral area is
512 kB in size and is divided to allow for up to 32 peripherals. Each peripheral of either
type is allocated 16 kB of space. This addressing scheme allows simplifying the address
decoding for each peripheral.
LPC11U3X All information provided in this document is subject to legal disclaimers. © NXP Semiconductors N.V. 2014. All rights reserved.
Product data sheet Rev. 2.2 — 11 March 2014 18 of 77
NXP Semiconductors LPC11U3x
32-bit ARM Cortex-M0 microcontroller
7.6 Nested Vectored Interrupt Controller (NVIC)
The Nested Vectored Interrupt Controller (NVIC) is part of the Cortex-M0. The tight
coupling to the CPU allows for low interrupt latency and efficient processing of late arriving
interrupts.
7.6.1 Features
• Controls system exceptions and peripheral interrupts.
• In the LPC11U3x, the NVIC supports 24 vectored interrupts.
Fig 6. LPC11U3x memory map
APB peripherals
0x4000 4000
0x4000 8000
0x4000 C000
0x4001 0000
0x4001 8000
0x4002 0000
0x4002 8000
0x4003 8000
0x4003 C000
0x4004 0000
0x4004 4000
0x4004 8000
0x4004 C000
0x4004 C000
0x4005 8000
0x4005 C000
0x4006 0000
0x4006 4000
0x4008 0000
0x4002 4000
0x4001 C000
0x4001 4000
0x4000 0000
WWDT
32-bit counter/timer 0
32-bit counter/timer 1
ADC
USART/SMART CARD
PMU
I2C-bus
20 - 21 reserved
10 - 13 reserved
reserved
reserved
25 - 31 reserved
0
1
2
3
4
5
6
7
8
9
16
15
14
17
18
reserved
reserved
0 GB 0x0000 0000
0.5 GB
4 GB
1 GB
0x1000 0000
0x1FFF 0000
0x1FFF 4000
0x2000 0000
0x5000 0000
0x5000 4000
0xFFFF FFFF
reserved
reserved
reserved
2 kB USB RAM (LPC11U34/421
LPC11U35/401/501
LPC11U36/401/501
LPC11U37/401/501,
LPC11U37H/401)
reserved
0x4000 0000
0x4008 0000
0x4008 4000
APB peripherals
USB
GPIO
0x2000 4000
0x2000 4800
0x1000 2000
8 kB SRAM0 (LPC11U3x)
LPC11U3x
0x0000 A000
40 kB on-chip flash (LPC11U34/311)
0x0000 C000
48 kB on-chip flash (LPC11U34/421)
0x0001 0000
64 kB on-chip flash (LPC11U35)
0x0001 8000
96 kB on-chip flash (LPC11U36)
0x0002 0000
128 kB on-chip flash (LPC11U37/7H)
16 kB boot ROM
0x0000 0000
0x0000 00C0
active interrupt vectors
002aag813
reserved
reserved
SSP0
SSP1
16-bit counter/timer 1
16-bit counter/timer 0
IOCON
system control
19 GPIO interrupts
22
23 GPIO GROUP0 INT
24 GPIO GROUP1 INT
flash/EEPROM controller
0xE000 0000
0xE010 0000
private peripheral bus
2 kB SRAM1 (LPC11U35/501
LPC11U37/501)
I/O Handler code area
for LPC11U37HFBD64/401
0x2000 0800
LPC11U3X All information provided in this document is subject to legal disclaimers. © NXP Semiconductors N.V. 2014. All rights reserved.
Product data sheet Rev. 2.2 — 11 March 2014 19 of 77
NXP Semiconductors LPC11U3x
32-bit ARM Cortex-M0 microcontroller
• Four programmable interrupt priority levels, with hardware priority level masking.
• Software interrupt generation.
7.6.2 Interrupt sources
Each peripheral device has one interrupt line connected to the NVIC but can have several
interrupt flags. Individual interrupt flags can also represent more than one interrupt
source.
7.7 IOCON block
The IOCON block allows selected pins of the microcontroller to have more than one
function. Configuration registers control the multiplexers to allow connection between the
pin and the on-chip peripherals.
Connect peripherals to the appropriate pins before activating the peripheral and before
enabling any related interrupt. Activity of any enabled peripheral function that is not
mapped to a related pin is treated as undefined.
7.7.1 Features
• Programmable pull-up, pull-down, or repeater mode.
• All GPIO pins (except PIO0_4 and PIO0_5) are pulled up to 3.3 V (VDD = 3.3 V) if their
pull-up resistor is enabled.
• Programmable pseudo open-drain mode.
• Programmable 10 ns glitch filter on pins PIO0_22, PIO0_23, and PIO0_11 to
PIO0_16. The glitch filter is turned on by default.
• Programmable hysteresis.
• Programmable input inverter.
7.8 General-Purpose Input/Output GPIO
The GPIO registers control device pin functions that are not connected to a specific
peripheral function. Pins can be dynamically configured as inputs or outputs. Multiple
outputs can be set or cleared in one write operation.
LPC11U3x use accelerated GPIO functions:
• GPIO registers are a dedicated AHB peripheral so that the fastest possible I/O timing
can be achieved.
• Entire port value can be written in one instruction.
Any GPIO pin providing a digital function can be programmed to generate an interrupt on
a level, a rising or falling edge, or both.
The GPIO block consists of three parts:
1. The GPIO ports.
2. The GPIO pin interrupt block to control eight GPIO pins selected as pin interrupts.
3. Two GPIO group interrupt blocks to control two combined interrupts from all GPIO
pins.
LPC11U3X All information provided in this document is subject to legal disclaimers. © NXP Semiconductors N.V. 2014. All rights reserved.
Product data sheet Rev. 2.2 — 11 March 2014 20 of 77
NXP Semiconductors LPC11U3x
32-bit ARM Cortex-M0 microcontroller
7.8.1 Features
• GPIO pins can be configured as input or output by software.
• All GPIO pins default to inputs with interrupt disabled at reset.
• Pin registers allow pins to be sensed and set individually.
• Up to eight GPIO pins can be selected from all GPIO pins to create an edge- or
level-sensitive GPIO interrupt request.
• Any pin or pins in each port can trigger a port interrupt.
7.9 USB interface
The Universal Serial Bus (USB) is a 4-wire bus that supports communication between a
host and one or more (up to 127) peripherals. The host controller allocates the USB
bandwidth to attached devices through a token-based protocol. The bus supports
hot-plugging and dynamic configuration of the devices. The host controller initiates all
transactions.
The LPC11U3x USB interface consists of a full-speed device controller with on-chip PHY
(PHYsical layer) for device functions.
Remark: Configure the LPC11U3x in default power mode with the power profiles before
using the USB (see Section 7.18.5.1). Do not use the USB with the part in performance,
efficiency, or low-power mode.
7.9.1 Full-speed USB device controller
The device controller enables 12 Mbit/s data exchange with a USB Host controller. It
consists of a register interface, serial interface engine, and endpoint buffer memory. The
serial interface engine decodes the USB data stream and writes data to the appropriate
endpoint buffer. The status of a completed USB transfer or error condition is indicated via
status registers. If enabled, an interrupt is generated.
7.9.1.1 Features
• Dedicated USB PLL available.
• Fully compliant with USB 2.0 specification (full speed).
• Supports 10 physical (5 logical) endpoints including one control endpoint.
• Single and double buffering supported.
• Each non-control endpoint supports bulk, interrupt, or isochronous endpoint types.
• Supports wake-up from Deep-sleep mode and Power-down mode on USB activity
and remote wake-up.
• Supports SoftConnect.
7.10 I/O Handler (LPC11U37HFBD64/401 only)
The I/O Handler is a software library-supported hardware engine for emulating serial
interfaces and off-loading the CPU for processing-intensive functions. The I/O Handler
can emulate, among others, DMA and serial interfaces such as UART, I2C, or I2S with no
or very low additional CPU load. The software libraries are available with supporting
LPC11U3X All information provided in this document is subject to legal disclaimers. © NXP Semiconductors N.V. 2014. All rights reserved.
Product data sheet Rev. 2.2 — 11 March 2014 21 of 77
NXP Semiconductors LPC11U3x
32-bit ARM Cortex-M0 microcontroller
application notes from NXP (see http://www.LPCware.com.) LPCXpresso, Keil, and IAR
IDEs are supported. I/O Handler library code must be executed from the memory area
0x2000 0000 to 0x2000 07FF. This memory is not available for other use.
For application examples, see Section 11.8 “I/O Handler software library applications”.
Each I/O Handler library uses a specific subset of I/O Handler pins and in some cases
other pins and peripherals such as the counter/timers.
7.11 USART
The LPC11U3x contains one USART.
The USART includes full modem control, support for synchronous mode, and a smart
card interface. The RS-485/9-bit mode allows both software address detection and
automatic address detection using 9-bit mode.
The USART uses a fractional baud rate generator. Standard baud rates such as
115200 Bd can be achieved with any crystal frequency above 2 MHz.
7.11.1 Features
• Maximum USART data bit rate of 3.125 Mbit/s.
• 16 byte receive and transmit FIFOs.
• Register locations conform to 16C550 industry standard.
• Receiver FIFO trigger points at 1 B, 4 B, 8 B, and 14 B.
• Built-in fractional baud rate generator covering wide range of baud rates without a
need for external crystals of particular values.
• Fractional divider for baud rate control, auto baud capabilities and FIFO control
mechanism that enables software flow control implementation.
• Support for RS-485/9-bit mode.
• Support for modem control.
• Support for synchronous mode.
• Includes smart card interface.
7.12 SSP serial I/O controller
The SSP controllers operate on a SSP, 4-wire SSI, or Microwire bus. The controller can
interact with multiple masters and slaves on the bus. Only a single master and a single
slave can communicate on the bus during a given data transfer. The SSP supports full
duplex transfers, with frames of 4 bit to 16 bit of data flowing from the master to the slave
and from the slave to the master. In practice, often only one of these data flows carries
meaningful data.
7.12.1 Features
• Maximum SSP speed of 25 Mbit/s (master) or 4.17 Mbit/s (slave) (in SSP mode)
• Compatible with Motorola SPI (Serial Peripheral Interface), 4-wire Texas Instruments
SSI (Serial Synchronous Interface), and National Semiconductor Microwire buses
• Synchronous serial communication
• Master or slave operation
LPC11U3X All information provided in this document is subject to legal disclaimers. © NXP Semiconductors N.V. 2014. All rights reserved.
Product data sheet Rev. 2.2 — 11 March 2014 22 of 77
NXP Semiconductors LPC11U3x
32-bit ARM Cortex-M0 microcontroller
• 8-frame FIFOs for both transmit and receive
• 4-bit to 16-bit frame
7.13 I2C-bus serial I/O controller
The LPC11U3x contain one I2C-bus controller.
The I2C-bus is bidirectional for inter-IC control using only two wires: a Serial CLock line
(SCL) and a Serial DAta line (SDA). Each device is recognized by a unique address and
can operate as either a receiver-only device (e.g., an LCD driver) or a transmitter with the
capability to both receive and send information (such as memory). Transmitters and/or
receivers can operate in either master or slave mode, depending on whether the chip has
to initiate a data transfer or is only addressed. The I2C-bus is a multi-master bus, and
more than one bus master connected to the interface can be controlled the bus.
7.13.1 Features
• The I2C-interface is an I2C-bus compliant interface with open-drain pins. The I2C-bus
interface supports Fast-mode Plus with bit rates up to 1 Mbit/s.
• Easy to configure as master, slave, or master/slave.
• Programmable clocks allow versatile rate control.
• Bidirectional data transfer between masters and slaves.
• Multi-master bus (no central master).
• Arbitration between simultaneously transmitting masters without corruption of serial
data on the bus.
• Serial clock synchronization allows devices with different bit rates to communicate via
one serial bus.
• Serial clock synchronization can be used as a handshake mechanism to suspend and
resume serial transfer.
• The I2C-bus can be used for test and diagnostic purposes.
• The I2C-bus controller supports multiple address recognition and a bus monitor mode.
7.14 10-bit ADC
The LPC11U3x contains one ADC. It is a single 10-bit successive approximation ADC with
eight channels.
7.14.1 Features
• 10-bit successive approximation ADC.
• Input multiplexing among 8 pins.
• Power-down mode.
• Measurement range 0 V to VDD.
• 10-bit conversion time 2.44 s (up to 400 kSamples/s).
• Burst conversion mode for single or multiple inputs.
• Optional conversion on transition of input pin or timer match signal.
• Individual result registers for each ADC channel to reduce interrupt overhead.
LPC11U3X All information provided in this document is subject to legal disclaimers. © NXP Semiconductors N.V. 2014. All rights reserved.
Product data sheet Rev. 2.2 — 11 March 2014 23 of 77
NXP Semiconductors LPC11U3x
32-bit ARM Cortex-M0 microcontroller
7.15 General purpose external event counter/timers
The LPC11U3x includes two 32-bit counter/timers and two 16-bit counter/timers. The
counter/timer is designed to count cycles of the system derived clock. It can optionally
generate interrupts or perform other actions at specified timer values, based on four
match registers. Each counter/timer also includes one capture input to trap the timer value
when an input signal transitions, optionally generating an interrupt.
7.15.1 Features
• A 32-bit/16-bit timer/counter with a programmable 32-bit/16-bit prescaler.
• Counter or timer operation.
• Up to two capture channels per timer, that can take a snapshot of the timer value
when an input signal transitions. A capture event can also generate an interrupt.
• Four match registers per timer that allow:
– Continuous operation with optional interrupt generation on match.
– Stop timer on match with optional interrupt generation.
– Reset timer on match with optional interrupt generation.
• Up to four external outputs corresponding to match registers, with the following
capabilities:
– Set LOW on match.
– Set HIGH on match.
– Toggle on match.
– Do nothing on match.
• The timer and prescaler can be configured to be cleared on a designated capture
event. This feature permits easy pulse-width measurement by clearing the timer on
the leading edge of an input pulse and capturing the timer value on the trailing edge.
7.16 System tick timer
The ARM Cortex-M0 includes a system tick timer (SYSTICK) that is intended to generate
a dedicated SYSTICK exception at a fixed time interval (typically 10 ms).
7.17 Windowed WatchDog Timer (WWDT)
The purpose of the WWDT is to prevent an unresponsive system state. If software fails to
update the watchdog within a programmable time window, the watchdog resets the
microcontroller
7.17.1 Features
• Internally resets chip if not periodically reloaded during the programmable time-out
period.
• Optional windowed operation requires reload to occur between a minimum and
maximum time period, both programmable.
• Optional warning interrupt can be generated at a programmable time before watchdog
time-out.
LPC11U3X All information provided in this document is subject to legal disclaimers. © NXP Semiconductors N.V. 2014. All rights reserved.
Product data sheet Rev. 2.2 — 11 March 2014 24 of 77
NXP Semiconductors LPC11U3x
32-bit ARM Cortex-M0 microcontroller
• Software enables the WWDT, but a hardware reset or a watchdog reset/interrupt is
required to disable the WWDT.
• Incorrect feed sequence causes reset or interrupt, if enabled.
• Flag to indicate watchdog reset.
• Programmable 24-bit timer with internal prescaler.
• Selectable time period from (Tcy(WDCLK) 256 4) to (Tcy(WDCLK) 224 4) in
multiples of Tcy(WDCLK) 4.
• The Watchdog Clock (WDCLK) source can be selected from the IRC or the dedicated
watchdog oscillator (WDO). The clock source selection provides a wide range of
potential timing choices of watchdog operation under different power conditions.
7.18 Clocking and power control
7.18.1 Integrated oscillators
The LPC11U3x include three independent oscillators: the system oscillator, the Internal
RC oscillator (IRC), and the watchdog oscillator. Each oscillator can be used for more
than one purpose as required in a particular application.
Following reset, the LPC11U3x operates from the internal RC oscillator until software
switches to a different clock source. The IRC allows the system to operate without any
external crystal and the bootloader code to operate at a known frequency.
See Figure 7 for an overview of the LPC11U3x clock generation.
LPC11U3X All information provided in this document is subject to legal disclaimers. © NXP Semiconductors N.V. 2014. All rights reserved.
Product data sheet Rev. 2.2 — 11 March 2014 25 of 77
NXP Semiconductors LPC11U3x
32-bit ARM Cortex-M0 microcontroller
7.18.1.1 Internal RC oscillator
The IRC can be used as the clock source for the WDT, and/or as the clock that drives the
system PLL and then the CPU. The nominal IRC frequency is 12 MHz.
Upon power-up, any chip reset, or wake-up from Deep power-down mode, the LPC11U3x
use the IRC as the clock source. Software can later switch to one of the other available
clock sources.
Fig 7. LPC11U3x clocking generation block diagram
system oscillator
watchdog oscillator
IRC oscillator
USB PLL
USBPLLCLKSEL
(USB clock select)
SYSTEM CLOCK
DIVIDER
SYSAHBCLKCTRLn
(AHB clock enable)
CPU, system control,
PMU
memories,
peripheral clocks
SSP0 PERIPHERAL
CLOCK DIVIDER SSP0
SSP1 PERIPHERAL
CLOCK DIVIDER SSP1
USART PERIPHERAL
CLOCK DIVIDER UART
WDT
WDCLKSEL
(WDT clock select)
USB 48 MHz CLOCK
DIVIDER USB
USBUEN
(USB clock update enable)
watchdog oscillator
IRC oscillator
system oscillator CLKOUT PIN CLOCK
DIVIDER CLKOUT pin
CLKOUTUEN
(CLKOUT update enable)
002aaf892
system clock
SYSTEM PLL
IRC oscillator
system oscillator
watchdog oscillator
MAINCLKSEL
(main clock select)
SYSPLLCLKSEL
(system PLL clock select)
main clock
IRC oscillator
n
LPC11U3X All information provided in this document is subject to legal disclaimers. © NXP Semiconductors N.V. 2014. All rights reserved.
Product data sheet Rev. 2.2 — 11 March 2014 26 of 77
NXP Semiconductors LPC11U3x
32-bit ARM Cortex-M0 microcontroller
7.18.1.2 System oscillator
The system oscillator can be used as the clock source for the CPU, with or without using
the PLL. On the LPC11U3x, use the system oscillator to provide the clock source to USB.
The system oscillator operates at frequencies of 1 MHz to 25 MHz. This frequency can be
boosted to a higher frequency, up to the maximum CPU operating frequency, by the
system PLL.
7.18.1.3 Watchdog oscillator
The watchdog oscillator can be used as a clock source that directly drives the CPU, the
watchdog timer, or the CLKOUT pin. The watchdog oscillator nominal frequency is
programmable between 9.4 kHz and 2.3 MHz. The frequency spread over processing and
temperature is 40 % (see also Table 13).
7.18.2 System PLL and USB PLL
The LPC11U3x contain a system PLL and a dedicated PLL for generating the 48 MHz
USB clock. The system and USB PLLs are identical.
The PLL accepts an input clock frequency in the range of 10 MHz to 25 MHz. The input
frequency is multiplied up to a high frequency with a Current Controlled Oscillator (CCO).
The multiplier can be an integer value from 1 to 32. The CCO operates in the range of
156 MHz to 320 MHz. To support this frequency range, an additional divider keeps the
CCO within its frequency range while the PLL is providing the desired output frequency.
The output divider can be set to divide by 2, 4, 8, or 16 to produce the output clock. The
PLL output frequency must be lower than 100 MHz. Since the minimum output divider
value is 2, it is insured that the PLL output has a 50 % duty cycle. The PLL is turned off
and bypassed following a chip reset. Software can enable the PLL later. The program
must configure and activate the PLL, wait for the PLL to lock, and then connect to the PLL
as a clock source. The PLL settling time is 100 s.
7.18.3 Clock output
The LPC11U3x feature a clock output function that routes the IRC oscillator, the system
oscillator, the watchdog oscillator, or the main clock to an output pin.
7.18.4 Wake-up process
The LPC11U3x begin operation by using the 12 MHz IRC oscillator as the clock source at
power-up and when awakened from Deep power-down mode . This mechanism allows
chip operation to resume quickly. If the application uses the main oscillator or the PLL,
software must enable these components and wait for them to stabilize. Only then can the
system use the PLL and main oscillator as a clock source.
7.18.5 Power control
The LPC11U3x support various power control features. There are four special modes of
processor power reduction: Sleep mode, Deep-sleep mode, Power-down mode, and
Deep power-down mode. The CPU clock rate can also be controlled as needed by
changing clock sources, reconfiguring PLL values, and/or altering the CPU clock divider
value. This power control mechanism allows a trade-off of power versus processing speed
based on application requirements. In addition, a register is provided for shutting down the
clocks to individual on-chip peripherals. This register allows fine-tuning of power
LPC11U3X All information provided in this document is subject to legal disclaimers. © NXP Semiconductors N.V. 2014. All rights reserved.
Product data sheet Rev. 2.2 — 11 March 2014 27 of 77
NXP Semiconductors LPC11U3x
32-bit ARM Cortex-M0 microcontroller
consumption by eliminating all dynamic power use in any peripherals that are not required
for the application. Selected peripherals have their own clock divider which provides even
better power control.
7.18.5.1 Power profiles
The power consumption in Active and Sleep modes can be optimized for the application
through simple calls to the power profile. The power configuration routine configures the
LPC11U3x for one of the following power modes:
• Default mode corresponding to power configuration after reset.
• CPU performance mode corresponding to optimized processing capability.
• Efficiency mode corresponding to optimized balance of current consumption and CPU
performance.
• Low-current mode corresponding to lowest power consumption.
In addition, the power profile includes routines to select the optimal PLL settings for a
given system clock and PLL input clock.
Remark: When using the USB, configure the LPC11U3x in Default mode.
7.18.5.2 Sleep mode
When Sleep mode is entered, the clock to the core is stopped. Resumption from the Sleep
mode does not need any special sequence but re-enabling the clock to the ARM core.
In Sleep mode, execution of instructions is suspended until either a reset or interrupt
occurs. Peripheral functions continue operation during Sleep mode and can generate
interrupts to cause the processor to resume execution. Sleep mode eliminates dynamic
power used by the processor itself, by memory systems and related controllers, and by
internal buses.
7.18.5.3 Deep-sleep mode
In Deep-sleep mode, the LPC11U3x is in Sleep-mode and all peripheral clocks and all
clock sources are off except for the IRC. The IRC output is disabled unless the IRC is
selected as input to the watchdog timer. In addition all analog blocks are shut down and
the flash is in stand-by mode. In Deep-sleep mode, the application can keep the watchdog
oscillator and the BOD circuit running for self-timed wake-up and BOD protection.
The LPC11U3x can wake up from Deep-sleep mode via reset, selected GPIO pins, a
watchdog timer interrupt, or an interrupt generating USB port activity.
Deep-sleep mode saves power and allows for short wake-up times.
7.18.5.4 Power-down mode
In Power-down mode, the LPC11U3x is in Sleep-mode and all peripheral clocks and all
clock sources are off except for watchdog oscillator if selected. In addition all analog
blocks and the flash are shut down. In Power-down mode, the application can keep the
BOD circuit running for BOD protection.
The LPC11U3x can wake up from Power-down mode via reset, selected GPIO pins, a
watchdog timer interrupt, or an interrupt generating USB port activity.
LPC11U3X All information provided in this document is subject to legal disclaimers. © NXP Semiconductors N.V. 2014. All rights reserved.
Product data sheet Rev. 2.2 — 11 March 2014 28 of 77
NXP Semiconductors LPC11U3x
32-bit ARM Cortex-M0 microcontroller
Power-down mode reduces power consumption compared to Deep-sleep mode at the
expense of longer wake-up times.
7.18.5.5 Deep power-down mode
In Deep power-down mode, power is shut off to the entire chip except for the WAKEUP
pin. The LPC11U3x can wake up from Deep power-down mode via the WAKEUP pin.
The LPC11U3x can be prevented from entering Deep power-down mode by setting a lock
bit in the PMU block. Locking out Deep power-down mode enables the application to keep
the watchdog timer or the BOD running at all times.
When entering Deep power-down mode, an external pull-up resistor is required on the
WAKEUP pin to hold it HIGH. Pull the RESET pin HIGH to prevent it from floating while in
Deep power-down mode.
7.18.6 System control
7.18.6.1 Reset
Reset has four sources on the LPC11U3x: the RESET pin, the Watchdog reset, power-on
reset (POR), and the BrownOut Detection (BOD) circuit. The RESET pin is a Schmitt
trigger input pin. Assertion of chip reset by any source, once the operating voltage attains
a usable level, starts the IRC and initializes the flash controller.
A LOW-going pulse as short as 50 ns resets the part.
When the internal Reset is removed, the processor begins executing at address 0, which
is initially the Reset vector mapped from the boot block. At that point, all of the processor
and peripheral registers have been initialized to predetermined values.
In Deep power-down mode, an external pull-up resistor is required on the RESET pin.
7.18.6.2 Brownout detection
The LPC11U3x includes up to four levels for monitoring the voltage on the VDD pin. If this
voltage falls below one of the selected levels, the BOD asserts an interrupt signal to the
NVIC. This signal can be enabled for interrupt in the Interrupt Enable Register in the NVIC
to cause a CPU interrupt. Alternatively, software can monitor the signal by reading a
dedicated status register. Four threshold levels can be selected to cause a forced reset of
the chip.
7.18.6.3 Code security (Code Read Protection - CRP)
CRP provides different levels of security in the system so that access to the on-chip flash
and use of the Serial Wire Debugger (SWD) and In-System Programming (ISP) can be
restricted. Programming a specific pattern into a dedicated flash location invokes CRP.
IAP commands are not affected by the CRP.
In addition, ISP entry via the PIO0_1 pin can be disabled without enabling CRP. For
details, see the LPC11Uxx user manual.
There are three levels of Code Read Protection:
LPC11U3X All information provided in this document is subject to legal disclaimers. © NXP Semiconductors N.V. 2014. All rights reserved.
Product data sheet Rev. 2.2 — 11 March 2014 29 of 77
NXP Semiconductors LPC11U3x
32-bit ARM Cortex-M0 microcontroller
1. CRP1 disables access to the chip via the SWD and allows partial flash update
(excluding flash sector 0) using a limited set of the ISP commands. This mode is
useful when CRP is required and flash field updates are needed but all sectors cannot
be erased.
2. CRP2 disables access to the chip via the SWD and only allows full flash erase and
update using a reduced set of the ISP commands.
3. Running an application with level CRP3 selected, fully disables any access to the chip
via the SWD pins and the ISP. This mode effectively disables ISP override using
PIO0_1 pin as well. If necessary, the application must provide a flash update
mechanism using IAP calls or using a call to the reinvoke ISP command to enable
flash update via the USART.
In addition to the three CRP levels, sampling of pin PIO0_1 for valid user code can be
disabled. For details, see the LPC11Uxx user manual.
7.18.6.4 APB interface
The APB peripherals are located on one APB bus.
7.18.6.5 AHBLite
The AHBLite connects the CPU bus of the ARM Cortex-M0 to the flash memory, the main
static RAM, and the ROM.
7.18.6.6 External interrupt inputs
All GPIO pins can be level or edge sensitive interrupt inputs.
CAUTION
If level three Code Read Protection (CRP3) is selected, no future factory testing can be
performed on the device.
LPC11U3X All information provided in this document is subject to legal disclaimers. © NXP Semiconductors N.V. 2014. All rights reserved.
Product data sheet Rev. 2.2 — 11 March 2014 30 of 77
NXP Semiconductors LPC11U3x
32-bit ARM Cortex-M0 microcontroller
7.19 Emulation and debugging
Debug functions are integrated into the ARM Cortex-M0. Serial wire debug functions are
supported in addition to a standard JTAG boundary scan. The ARM Cortex-M0 is
configured to support up to four breakpoints and two watch points.
The RESET pin selects between the JTAG boundary scan (RESET = LOW) and the ARM
SWD debug (RESET = HIGH). The ARM SWD debug port is disabled while the
LPC11U3x is in reset.
To perform boundary scan testing, follow these steps:
1. Erase any user code residing in flash.
2. Power up the part with the RESET pin pulled HIGH externally.
3. Wait for at least 250 s.
4. Pull the RESET pin LOW externally.
5. Perform boundary scan operations.
6. Once the boundary scan operations are completed, assert the TRST pin to enable the
SWD debug mode, and release the RESET pin (pull HIGH).
Remark: The JTAG interface cannot be used for debug purposes.
LPC11U3X All information provided in this document is subject to legal disclaimers. © NXP Semiconductors N.V. 2014. All rights reserved.
Product data sheet Rev. 2.2 — 11 March 2014 31 of 77
NXP Semiconductors LPC11U3x
32-bit ARM Cortex-M0 microcontroller
8. Limiting values
[1] The following applies to the limiting values:
a) This product includes circuitry specifically designed for the protection of its internal devices from the damaging effects of excessive
static charge. Nonetheless, it is suggested that conventional precautions be taken to avoid applying greater than the rated
maximum.
b) Parameters are valid over operating temperature range unless otherwise specified. All voltages are with respect to VSS unless
otherwise noted.
c) The limiting values are stress ratings only. Operating the part at these values is not recommended, and proper operation is not
guaranteed. The conditions for functional operation are specified in Table 5.
[2] Maximum/minimum voltage above the maximum operating voltage (see Table 5) and below ground that can be applied for a short time
(< 10 ms) to a device without leading to irrecoverable failure. Failure includes the loss of reliability and shorter lifetime of the device.
[3] See Table 6 for maximum operating voltage.
[4] VDD present or not present. Compliant with the I2C-bus standard. 5.5 V can be applied to this pin when VDD is powered down.
[5] Including voltage on outputs in 3-state mode.
[6] The maximum non-operating storage temperature is different than the temperature for required shelf life which should be determined
based on required shelf lifetime. Please refer to the JEDEC spec (J-STD-033B.1) for further details.
[7] Human body model: equivalent to discharging a 100 pF capacitor through a 1.5 k series resistor.
Table 4. Limiting values
In accordance with the Absolute Maximum Rating System (IEC 60134).[1]
Symbol Parameter Conditions Min Max Unit
VDD supply voltage (core and
external rail)
[2] 0.5 +4.6 V
VI input voltage 5 V tolerant digital I/O pins;
VDD 1.8 V
[5][2] 0.5 +5.5 V
VDD = 0 V 0.5 +3.6 V
5 V tolerant open-drain pins
PIO0_4 and PIO0_5
[2][4] 0.5 +5.5
VIA analog input voltage pin configured as analog input [2]
[3]
0.5 4.6 V
IDD supply current per supply pin - 100 mA
ISS ground current per ground pin - 100 mA
Ilatch I/O latch-up current (0.5VDD) < VI < (1.5VDD);
Tj < 125 C
- 100 mA
Tstg storage temperature non-operating [6] 65 +150 C
Tj(max) maximum junction
temperature
- 150 C
Ptot(pack) total power dissipation (per
package)
based on package heat
transfer, not device power
consumption
- 1.5 W
VESD electrostatic discharge
voltage
human body model; all pins [7]- +6500 V
LPC11U3X All information provided in this document is subject to legal disclaimers. © NXP Semiconductors N.V. 2014. All rights reserved.
Product data sheet Rev. 2.2 — 11 March 2014 32 of 77
NXP Semiconductors LPC11U3x
32-bit ARM Cortex-M0 microcontroller
9. Static characteristics
Table 5. Static characteristics
Tamb = 40 C to +85 C, unless otherwise specified.
Symbol Parameter Conditions Min Typ[1] Max Unit
VDD supply voltage (core
and external rail)
[2] 1.8 3.3 3.6 V
IDD supply current Active mode; VDD = 3.3 V;
Tamb = 25 C; code
while(1){}
executed from flash;
system clock = 12 MHz [3][4][5]
[6][7][8]
- 2 - mA
system clock = 50 MHz [4][5][6]
[7][8][9]
- 7 - mA
Sleep mode;
VDD = 3.3 V; Tamb = 25 C;
system clock = 12 MHz
[3][4][5]
[6][7][8]
- 1 - mA
Deep-sleep mode; VDD = 3.3 V;
Tamb = 25 C
[4][7]- 300 - A
Power-down mode; VDD = 3.3 V;
Tamb = 25 C
- 2 - A
Deep power-down mode;
VDD = 3.3 V; Tamb = 25 C
[10]- 220 - nA
Standard port pins, RESET
IIL LOW-level input current VI = 0 V; on-chip pull-up resistor
disabled
- 0.5 10 nA
IIH HIGH-level input
current
VI = VDD; on-chip pull-down resistor
disabled
- 0.5 10 nA
IOZ OFF-state output
current
VO = 0 V; VO = VDD; on-chip
pull-up/down resistors disabled
- 0.5 10 nA
VI input voltage pin configured to provide a digital
function; VDD 1.8 V
[11]
[12]
0 - 5.0 V
VDD = 0 V 0 - 3.6 V
VO output voltage output active 0 - VDD V
VIH HIGH-level input
voltage
0.7VDD - - V
VIL LOW-level input voltage - - 0.3VDD V
Vhys hysteresis voltage - 0.4 - V
VOH HIGH-level output
voltage
2.0 V VDD 3.6 V; IOH = 4 mA VDD 0.4- - V
1.8 V VDD < 2.0 V; IOH = 3 mA VDD 0.4- - V
VOL LOW-level output
voltage
2.0 V VDD 3.6 V; IOL = 4 mA - - 0.4 V
1.8 V VDD < 2.0 V; IOL = 3 mA - - 0.4 V
IOH HIGH-level output
current
VOH = VDD 0.4 V;
2.0 V VDD 3.6 V
4 - - mA
1.8 V VDD < 2.0 V 3 - - mA
LPC11U3X All information provided in this document is subject to legal disclaimers. © NXP Semiconductors N.V. 2014. All rights reserved.
Product data sheet Rev. 2.2 — 11 March 2014 33 of 77
NXP Semiconductors LPC11U3x
32-bit ARM Cortex-M0 microcontroller
IOL LOW-level output
current
VOL = 0.4 V
2.0 V VDD 3.6 V
4 - - mA
1.8 V VDD < 2.0 V 3 - - mA
IOHS HIGH-level short-circuit
output current
VOH = 0 V [13]- - 45 mA
IOLS LOW-level short-circuit
output current
VOL = VDD [13]- - 50 mA
Ipd pull-down current VI = 5 V 10 50 150 A
Ipu pull-up current VI = 0 V;
2.0 V VDD 3.6 V
15 50 85 A
1.8 V VDD < 2.0 V 10 50 85 A
VDD < VI < 5 V 0 0 0 A
High-drive output pin (PIO0_7)
IIL LOW-level input current VI = 0 V; on-chip pull-up resistor
disabled
- 0.5 10 nA
IIH HIGH-level input
current
VI = VDD; on-chip pull-down resistor
disabled
- 0.5 10 nA
IOZ OFF-state output
current
VO = 0 V; VO = VDD; on-chip
pull-up/down resistors disabled
- 0.5 10 nA
VI input voltage pin configured to provide a digital
function; VDD 1.8 V
[11]
[12]
0 - 5.0 V
VDD = 0 V 0 - 3.6 V
VO output voltage output active 0 - VDD V
VIH HIGH-level input
voltage
0.7VDD - - V
VIL LOW-level input voltage - - 0.3VDD V
Vhys hysteresis voltage 0.4 - - V
VOH HIGH-level output
voltage
2.5 V VDD 3.6 V; IOH = 20 mA VDD 0.4- - V
1.8 V VDD < 2.5 V; IOH = 12 mA VDD 0.4- - V
VOL LOW-level output
voltage
2.0 V VDD 3.6 V; IOL = 4 mA - - 0.4 V
1.8 V VDD < 2.0 V; IOL = 3 mA - - 0.4 V
IOH HIGH-level output
current
VOH = VDD 0.4 V;
2.5 V VDD 3.6 V
20 - - mA
1.8 V VDD < 2.5 V 12 - - mA
IOL LOW-level output
current
VOL = 0.4 V
2.0 V VDD 3.6 V
4 - - mA
1.8 V VDD < 2.0 V 3 - - mA
IOLS LOW-level short-circuit
output current
VOL = VDD [13]- - 50 mA
Ipd pull-down current VI = 5 V 10 50 150 A
Table 5. Static characteristics …continued
Tamb = 40 C to +85 C, unless otherwise specified.
Symbol Parameter Conditions Min Typ[1] Max Unit
LPC11U3X All information provided in this document is subject to legal disclaimers. © NXP Semiconductors N.V. 2014. All rights reserved.
Product data sheet Rev. 2.2 — 11 March 2014 34 of 77
NXP Semiconductors LPC11U3x
32-bit ARM Cortex-M0 microcontroller
Ipu pull-up current VI = 0 V
2.0 V VDD 3.6 V
15 50 85 A
1.8 V VDD < 2.0 V 10 50 85 A
VDD < VI < 5 V 0 0 0 A
I2C-bus pins (PIO0_4 and PIO0_5)
VIH HIGH-level input
voltage
0.7VDD - - V
VIL LOW-level input voltage - - 0.3VDD V
Vhys hysteresis voltage - 0.05VDD - V
IOL LOW-level output
current
VOL = 0.4 V; I2C-bus pins configured
as standard mode pins
2.0 V VDD 3.6 V
3.5 - - mA
1.8 V VDD < 2.0 V 3 - -
IOL LOW-level output
current
VOL = 0.4 V; I2C-bus pins configured
as Fast-mode Plus pins
2.0 V VDD 3.6 V
20 - - mA
1.8 V VDD < 2.0 V 16 - -
ILI input leakage current VI = VDD [14]- 2 4 A
VI = 5 V - 10 22 A
Oscillator pins
Vi(xtal) crystal input voltage 0.5 1.8 1.95 V
Vo(xtal) crystal output voltage 0.5 1.8 1.95 V
USB pins
IOZ OFF-state output
current
0 V < VI < 3.3 V [2]- - 10 A
VBUS bus supply voltage [2]- - 5.25 V
VDI differential input
sensitivity voltage
(D+) (D) [2] 0.2 - - V
VCM differential common
mode voltage range
includes VDI range [2] 0.8 - 2.5 V
Vth(rs)se single-ended receiver
switching threshold
voltage
[2] 0.8 - 2.0 V
VOL LOW-level output
voltage
for low-/full-speed;
RL of 1.5 k to 3.6 V
[2]- - 0.18 V
VOH HIGH-level output
voltage
driven; for low-/full-speed;
RL of 15 k to GND
[2] 2.8 - 3.5 V
Ctrans transceiver capacitance pin to GND [2]- - 20 pF
ZDRV driver output
impedance for driver
which is not high-speed
capable
with 33 series resistor; steady state
drive
[15][2] 36 - 44.1
Table 5. Static characteristics …continued
Tamb = 40 C to +85 C, unless otherwise specified.
Symbol Parameter Conditions Min Typ[1] Max Unit
LPC11U3X All information provided in this document is subject to legal disclaimers. © NXP Semiconductors N.V. 2014. All rights reserved.
Product data sheet Rev. 2.2 — 11 March 2014 35 of 77
NXP Semiconductors LPC11U3x
32-bit ARM Cortex-M0 microcontroller
[1] Typical ratings are not guaranteed. The values listed are at room temperature (25 C), nominal supply voltages.
[2] For USB operation 3.0 V VDD 3.6 V. Guaranteed by design.
[3] IRC enabled; system oscillator disabled; system PLL disabled.
[4] IDD measurements were performed with all pins configured as GPIO outputs driven LOW and pull-up resistors disabled.
[5] BOD disabled.
[6] All peripherals disabled in the AHBCLKCTRL register. Peripheral clocks to USART, SSP0/1 disabled in the SYSCON block.
[7] USB_DP and USB_DM pulled LOW externally.
[8] Low-current mode PWR_LOW_CURRENT selected when running the set_power routine in the power profiles.
[9] IRC disabled; system oscillator enabled; system PLL enabled.
[10] WAKEUP pin pulled HIGH externally. An external pull-up resistor is required on the RESET pin for the Deep power-down mode.
[11] Including voltage on outputs in 3-state mode.
[12] 3-state outputs go into 3-state mode in Deep power-down mode.
[13] Allowed as long as the current limit does not exceed the maximum current allowed by the device.
[14] To VSS.
[15] Includes external resistors of 33 1 % on USB_DP and USB_DM.
Pin capacitance
Cio input/output
capacitance
pins configured for analog function - - 7.1 pF
I2C-bus pins (PIO0_4 and PIO0_5) - - 2.5 pF
pins configured as GPIO - - 2.8 pF
Table 5. Static characteristics …continued
Tamb = 40 C to +85 C, unless otherwise specified.
Symbol Parameter Conditions Min Typ[1] Max Unit
LPC11U3X All information provided in this document is subject to legal disclaimers. © NXP Semiconductors N.V. 2014. All rights reserved.
Product data sheet Rev. 2.2 — 11 March 2014 36 of 77
NXP Semiconductors LPC11U3x
32-bit ARM Cortex-M0 microcontroller
[1] The ADC is monotonic, there are no missing codes.
[2] The differential linearity error (ED) is the difference between the actual step width and the ideal step width. See Figure 8.
[3] The integral non-linearity (EL(adj)) is the peak difference between the center of the steps of the actual and the ideal transfer curve after
appropriate adjustment of gain and offset errors. See Figure 8.
[4] The offset error (EO) is the absolute difference between the straight line which fits the actual curve and the straight line which fits the
ideal curve. See Figure 8.
[5] The gain error (EG) is the relative difference in percent between the straight line fitting the actual transfer curve after removing offset
error, and the straight line which fits the ideal transfer curve. See Figure 8.
[6] The absolute error (ET) is the maximum difference between the center of the steps of the actual transfer curve of the non-calibrated
ADC and the ideal transfer curve. See Figure 8.
[7] Tamb = 25 C; maximum sampling frequency fs = 400 kSamples/s and analog input capacitance Cia = 1 pF.
[8] Input resistance Ri depends on the sampling frequency fs: Ri = 1 / (fs Cia).
Table 6. ADC static characteristics
Tamb = 40 C to +85 C unless otherwise specified; ADC frequency 4.5 MHz, VDD = 2.5 V to 3.6 V.
Symbol Parameter Conditions Min Typ Max Unit
VIA analog input voltage 0 - VDD V
Cia analog input capacitance - - 1 pF
ED differential linearity error [1][2]- - 1 LSB
EL(adj) integral non-linearity [3]- - 1.5 LSB
EO offset error [4]- - 3.5 LSB
EG gain error [5]- - 0.6 %
ET absolute error [6]- - 4 LSB
Rvsi voltage source interface
resistance
- - 40 k
Ri input resistance [7][8]- - 2.5 M
LPC11U3X All information provided in this document is subject to legal disclaimers. © NXP Semiconductors N.V. 2014. All rights reserved.
Product data sheet Rev. 2.2 — 11 March 2014 37 of 77
NXP Semiconductors LPC11U3x
32-bit ARM Cortex-M0 microcontroller
(1) Example of an actual transfer curve.
(2) The ideal transfer curve.
(3) Differential linearity error (ED).
(4) Integral non-linearity (EL(adj)).
(5) Center of a step of the actual transfer curve.
Fig 8. ADC characteristics
002aaf426
1023
1022
1021
1020
1019
(2)
(1)
1 2 3 4 5 6 7 1018 1019 1020 1021 1022 1023 1024
7
6
5
4
3
2
1
0
1018
(5)
(4)
(3)
1 LSB
(ideal)
code
out
VDD − VSS
1024
offset
error
EO
gain
error
EG
offset error
EO
VIA (LSBideal)
1 LSB =
LPC11U3X All information provided in this document is subject to legal disclaimers. © NXP Semiconductors N.V. 2014. All rights reserved.
Product data sheet Rev. 2.2 — 11 March 2014 38 of 77
NXP Semiconductors LPC11U3x
32-bit ARM Cortex-M0 microcontroller
9.1 BOD static characteristics
[1] Interrupt levels are selected by writing the level value to the BOD control register BODCTRL, see the
LPC11Uxx user manual.
9.2 Power consumption
Power measurements in Active, Sleep, and Deep-sleep modes were performed under the
following conditions (see the LPC11Uxx user manual):
• Configure all pins as GPIO with pull-up resistor disabled in the IOCON block.
• Configure GPIO pins as outputs using the GPIOnDIR registers.
• Write 0 to all GPIOnDATA registers to drive the outputs LOW.
Table 7. BOD static characteristics[1]
Tamb = 25 C.
Symbol Parameter Conditions Min Typ Max Unit
Vth threshold voltage interrupt level 1
assertion - 2.22 - V
de-assertion - 2.35 - V
interrupt level 2
assertion - 2.52 - V
de-assertion - 2.66 - V
interrupt level 3
assertion - 2.80 - V
de-assertion - 2.90 - V
reset level 0
assertion - 1.46 - V
de-assertion - 1.63 - V
reset level 1
assertion - 2.06 - V
de-assertion - 2.15 - V
reset level 2
assertion - 2.35 - V
de-assertion - 2.43 - V
reset level 3
assertion - 2.63 - V
de-assertion - 2.71 - V
LPC11U3X All information provided in this document is subject to legal disclaimers. © NXP Semiconductors N.V. 2014. All rights reserved.
Product data sheet Rev. 2.2 — 11 March 2014 39 of 77
NXP Semiconductors LPC11U3x
32-bit ARM Cortex-M0 microcontroller
Conditions: Tamb = 25 C; Active mode entered executing code while(1){} from flash;
internal pull-up resistors disabled; BOD disabled; all peripherals disabled in the
SYSAHBCLKCTRL register; all peripheral clocks disabled; low-current mode; USB_DP and
USB_DM pulled LOW externally.
(1) System oscillator and system PLL disabled; IRC enabled.
(2) System oscillator and system PLL enabled; IRC disabled.
Fig 9. Typical supply current versus regulator supply voltage VDD in active mode
Conditions: VDD = 3.3 V; Active mode entered executing code while(1){} from flash; internal
pull-up resistors disabled; BOD disabled; all peripherals disabled in the SYSAHBCLKCTRL
register; all peripheral clocks disabled; low-current mode; USB_DP and USB_DM pulled LOW
externally.
(1) System oscillator and system PLL disabled; IRC enabled.
(2) System oscillator and system PLL enabled; IRC disabled.
Fig 10. Typical supply current versus temperature in Active mode
VDD (V)
1.8 2.4 3.0 3.6
002aag749
3
6
9
IDD
(mA)
0
12 MHz(1)
24 MHz(2)
36 MHz(2)
48 MHz(2)
temperature (°C)
-40 -15 10 35 60 85
002aag750
3
6
9
IDD
(mA)
0
12 MHz(1)
24 MHz(2)
36 MHz(2)
48 MHz(2)
LPC11U3X All information provided in this document is subject to legal disclaimers. © NXP Semiconductors N.V. 2014. All rights reserved.
Product data sheet Rev. 2.2 — 11 March 2014 40 of 77
NXP Semiconductors LPC11U3x
32-bit ARM Cortex-M0 microcontroller
Conditions: VDD = 3.3 V; Sleep mode entered from flash; internal pull-up resistors disabled; BOD
disabled; all peripherals disabled in the SYSAHBCLKCTRL register; all peripheral clocks disabled;
low-current mode; USB_DP and USB_DM pulled LOW externally.
(1) System oscillator and system PLL disabled; IRC enabled.
(2) System oscillator and system PLL enabled; IRC disabled.
Fig 11. Typical supply current versus temperature in Sleep mode
Conditions: BOD disabled; all oscillators and analog blocks turned off in the PDSLEEPCFG
register; USB_DP and USB_DM pulled LOW externally.
Fig 12. Typical supply current versus temperature in Deep-sleep mode
002aag751
temperature (°C)
-40 -15 10 35 60 85
1
3
2
4
IDD
(mA)
0
12 MHz(1)
36 MHz(2)
48 MHz(2)
24 MHz(2)
002aag745
temperature (°C)
-40 -15 10 35 60 85
355
375
365
385
IDD
(μA)
345
VDD = 3.6 V
VDD = 3.3 V
VDD = 2.0 V
VDD = 1.8 V
LPC11U3X All information provided in this document is subject to legal disclaimers. © NXP Semiconductors N.V. 2014. All rights reserved.
Product data sheet Rev. 2.2 — 11 March 2014 41 of 77
NXP Semiconductors LPC11U3x
32-bit ARM Cortex-M0 microcontroller
9.3 Peripheral power consumption
The supply current per peripheral is measured as the difference in supply current between
the peripheral block enabled and the peripheral block disabled in the SYSAHBCLKCFG
and PDRUNCFG (for analog blocks) registers. All other blocks are disabled in both
registers and no code is executed. Measured on a typical sample at Tamb = 25 C. Unless
noted otherwise, the system oscillator and PLL are running in both measurements.
The supply currents are shown for system clock frequencies of 12 MHz and 48 MHz.
Conditions: BOD disabled; all oscillators and analog blocks turned off in the PDSLEEPCFG
register; USB_DP and USB_DM pulled LOW externally.
Fig 13. Typical supply current versus temperature in Power-down mode
Fig 14. Typical supply current versus temperature in Deep power-down mode
002aag746
temperature (°C)
-40 -15 10 35 60 85
5
15
10
20
IDD
(μA)
0
VDD = 3.6 V, 3.3 V
VDD = 2.0 V
VDD = 1.8 V
002aag747
temperature (°C)
-40 -15 10 35 60 85
0.2
0.6
0.4
0.8
IDD
(μA)
0
VDD = 3.6 V
VDD = 3.3 V
VDD = 2.0 V
VDD = 1.8 V
LPC11U3X All information provided in this document is subject to legal disclaimers. © NXP Semiconductors N.V. 2014. All rights reserved.
Product data sheet Rev. 2.2 — 11 March 2014 42 of 77
NXP Semiconductors LPC11U3x
32-bit ARM Cortex-M0 microcontroller
Table 8. Power consumption for individual analog and digital blocks
Peripheral Typical supply current in
mA
Notes
n/a 12 MHz 48 MHz
IRC 0.27 - - System oscillator running; PLL off; independent
of main clock frequency.
System oscillator
at 12 MHz
0.22 - - IRC running; PLL off; independent of main clock
frequency.
Watchdog
oscillator at
500 kHz/2
0.004 - - System oscillator running; PLL off; independent
of main clock frequency.
BOD 0.051 - - Independent of main clock frequency.
Main PLL - 0.21 - -
ADC - 0.08 0.29 -
CLKOUT - 0.12 0.47 Main clock divided by 4 in the CLKOUTDIV
register.
CT16B0 - 0.02 0.06 -
CT16B1 - 0.02 0.06 -
CT32B0 - 0.02 0.07 -
CT32B1 - 0.02 0.06 -
GPIO - 0.23 0.88 GPIO pins configured as outputs and set to
LOW. Direction and pin state are maintained if
the GPIO is disabled in the SYSAHBCLKCFG
register.
IOCONFIG - 0.03 0.10 -
I2C - 0.04 0.13 -
ROM - 0.04 0.15 -
SPI0 - 0.12 0.45 -
SPI1 - 0.12 0.45 -
UART - 0.22 0.82 -
WWDT - 0.02 0.06 Main clock selected as clock source for the
WDT.
USB - - 1.2 -
LPC11U3X All information provided in this document is subject to legal disclaimers. © NXP Semiconductors N.V. 2014. All rights reserved.
Product data sheet Rev. 2.2 — 11 March 2014 43 of 77
NXP Semiconductors LPC11U3x
32-bit ARM Cortex-M0 microcontroller
9.4 Electrical pin characteristics
Conditions: VDD = 3.3 V; on pin PIO0_7.
Fig 15. High-drive output: Typical HIGH-level output voltage VOH versus HIGH-level
output current IOH.
Conditions: VDD = 3.3 V; on pins PIO0_4 and PIO0_5.
Fig 16. I2C-bus pins (high current sink): Typical LOW-level output current IOL versus
LOW-level output voltage VOL
IOH (mA)
0 10 20 30 40 50 60
002aae990
2.8
2.4
3.2
3.6
VOH
(V)
2
T = 85 °C
25 °C
−40 °C
VOL (V)
0 0.2 0.4 0.6
002aaf019
20
40
60
IOL
(mA)
0
T = 85 °C
25 °C
−40 °C
LPC11U3X All information provided in this document is subject to legal disclaimers. © NXP Semiconductors N.V. 2014. All rights reserved.
Product data sheet Rev. 2.2 — 11 March 2014 44 of 77
NXP Semiconductors LPC11U3x
32-bit ARM Cortex-M0 microcontroller
Conditions: VDD = 3.3 V; standard port pins and PIO0_7.
Fig 17. Typical LOW-level output current IOL versus LOW-level output voltage VOL
Conditions: VDD = 3.3 V; standard port pins.
Fig 18. Typical HIGH-level output voltage VOH versus HIGH-level output source current
IOH
VOL (V)
0 0.2 0.4 0.6
002aae991
5
10
15
IOL
(mA)
0
T = 85 °C
25 °C
−40 °C
IOH (mA)
0 8 16 24
002aae992
2.8
2.4
3.2
3.6
VOH
(V)
2
T = 85 °C
25 °C
−40 °C
LPC11U3X All information provided in this document is subject to legal disclaimers. © NXP Semiconductors N.V. 2014. All rights reserved.
Product data sheet Rev. 2.2 — 11 March 2014 45 of 77
NXP Semiconductors LPC11U3x
32-bit ARM Cortex-M0 microcontroller
Conditions: VDD = 3.3 V; standard port pins.
Fig 19. Typical pull-up current Ipu versus input voltage VI
Conditions: VDD = 3.3 V; standard port pins.
Fig 20. Typical pull-down current Ipd versus input voltage VI
VI (V)
0 1 2 3 4 5
002aae988
−30
−50
−10
10
Ipu
(μA)
−70
T = 85 °C
25 °C
−40 °C
VI (V)
0 1 2 3 4 5
002aae989
40
20
60
80
Ipd
(μA)
0
T = 85 °C
25 °C
−40 °C
LPC11U3X All information provided in this document is subject to legal disclaimers. © NXP Semiconductors N.V. 2014. All rights reserved.
Product data sheet Rev. 2.2 — 11 March 2014 46 of 77
NXP Semiconductors LPC11U3x
32-bit ARM Cortex-M0 microcontroller
10. Dynamic characteristics
10.1 Flash memory
[1] Number of program/erase cycles.
[2] Programming times are given for writing 256 bytes from RAM to the flash. Data must be written to the flash
in blocks of 256 bytes.
10.2 External clock
[1] Parameters are valid over operating temperature range unless otherwise specified.
[2] Typical ratings are not guaranteed. The values listed are at room temperature (25 C), nominal supply
voltages.
Table 9. Flash characteristics
Tamb = 40 C to +85 C, unless otherwise specified.
Symbol Parameter Conditions Min Typ Max Unit
Nendu endurance [1] 10000 100000 - cycles
tret retention time powered 10 - - years
unpowered 20 - - years
ter erase time sector or multiple
consecutive sectors
95 100 105 ms
tprog programming time [2] 0.95 1 1.05 ms
Table 10. EEPROM characteristics
Tamb = 40 C to +85C; VDD = 2.7 V to 3.6 V. Based on JEDEC NVM qualification. Failure rate <
10 ppm for parts as specified below.
Symbol Parameter Conditions Min Typ Max Unit
Nendu endurance 100000 1000000 - cycles
tret retention time powered 100 200 - years
unpowered 150 300 - years
tprog programming
time
64 bytes - 2.9 - ms
Table 11. Dynamic characteristic: external clock
Tamb = 40 C to +85 C; VDD over specified ranges.[1]
Symbol Parameter Conditions Min Typ[2] Max Unit
fosc oscillator frequency 1 - 25 MHz
Tcy(clk) clock cycle time 40 - 1000 ns
tCHCX clock HIGH time Tcy(clk) 0.4 - - ns
tCLCX clock LOW time Tcy(clk) 0.4 - - ns
tCLCH clock rise time - - 5 ns
tCHCL clock fall time - - 5 ns
LPC11U3X All information provided in this document is subject to legal disclaimers. © NXP Semiconductors N.V. 2014. All rights reserved.
Product data sheet Rev. 2.2 — 11 March 2014 47 of 77
NXP Semiconductors LPC11U3x
32-bit ARM Cortex-M0 microcontroller
10.3 Internal oscillators
[1] Parameters are valid over operating temperature range unless otherwise specified.
[2] Typical ratings are not guaranteed. The values listed are at room temperature (25 C), nominal supply
voltages.
[1] Typical ratings are not guaranteed. The values listed are at nominal supply voltages.
Fig 21. External clock timing (with an amplitude of at least Vi(RMS) = 200 mV)
tCHCL tCLCX
tCHCX
Tcy(clk)
tCLCH
002aaa907
Table 12. Dynamic characteristics: IRC
Tamb = 40 C to +85 C; 2.7 V VDD 3.6 V[1].
Symbol Parameter Conditions Min Typ[2] Max Unit
fosc(RC) internal RC oscillator
frequency
- 11.88 12 12.12 MHz
Conditions: Frequency values are typical values. 12 MHz 1 % accuracy is guaranteed for
2.7 V VDD 3.6 V and Tamb = 40 C to +85 C. Variations between parts may cause the IRC to
fall outside the 12 MHz 1 % accuracy specification for voltages below 2.7 V.
Fig 22. Internal RC oscillator frequency versus temperature
Table 13. Dynamic characteristics: Watchdog oscillator
Symbol Parameter Conditions Min Typ[1] Max Unit
fosc(int) internal oscillator
frequency
DIVSEL = 0x1F, FREQSEL = 0x1
in the WDTOSCCTRL register;
[2][3]- 9.4 - kHz
DIVSEL = 0x00, FREQSEL = 0xF
in the WDTOSCCTRL register
[2][3] - 2300 - kHz
002aaf403
11.95
12.05
12.15
f
(MHz)
11.85
temperature (°C)
−40 −15 10 35 60 85
VDD = 3.6 V
3.3 V
3.0 V
2.7 V
2.4 V
2.0 V
LPC11U3X All information provided in this document is subject to legal disclaimers. © NXP Semiconductors N.V. 2014. All rights reserved.
Product data sheet Rev. 2.2 — 11 March 2014 48 of 77
NXP Semiconductors LPC11U3x
32-bit ARM Cortex-M0 microcontroller
[2] The typical frequency spread over processing and temperature (Tamb = 40 C to +85 C) is 40 %.
[3] See the LPC11Uxx user manual.
10.4 I/O pins
[1] Applies to standard port pins and RESET pin.
10.5 I2C-bus
[1] See the I2C-bus specification UM10204 for details.
[2] Parameters are valid over operating temperature range unless otherwise specified.
[3] tHD;DAT is the data hold time that is measured from the falling edge of SCL; applies to data in transmission and the acknowledge.
[4] A device must internally provide a hold time of at least 300 ns for the SDA signal (with respect to the VIH(min) of the SCL signal) to
bridge the undefined region of the falling edge of SCL.
[5] Cb = total capacitance of one bus line in pF.
[6] The maximum tf for the SDA and SCL bus lines is specified at 300 ns. The maximum fall time for the SDA output stage tf is specified at
250 ns. This allows series protection resistors to be connected in between the SDA and the SCL pins and the SDA/SCL bus lines
without exceeding the maximum specified tf.
Table 14. Dynamic characteristics: I/O pins[1]
Tamb = 40 C to +85 C; 3.0 V VDD 3.6 V.
Symbol Parameter Conditions Min Typ Max Unit
tr rise time pin configured as output 3.0 - 5.0 ns
tf fall time pin configured as output 2.5 - 5.0 ns
Table 15. Dynamic characteristic: I2C-bus pins[1]
Tamb = 40 C to +85 C.[2]
Symbol Parameter Conditions Min Max Unit
fSCL SCL clock
frequency
Standard-mode 0 100 kHz
Fast-mode 0 400 kHz
Fast-mode Plus 0 1 MHz
tf fall time [4][5][6][7] of both SDA and SCL
signals
Standard-mode
- 300 ns
Fast-mode 20 + 0.1 Cb 300 ns
Fast-mode Plus - 120 ns
tLOW LOW period of the
SCL clock
Standard-mode 4.7 - s
Fast-mode 1.3 - s
Fast-mode Plus 0.5 - s
tHIGH HIGH period of the
SCL clock
Standard-mode 4.0 - s
Fast-mode 0.6 - s
Fast-mode Plus 0.26 - s
tHD;DAT data hold time [3][4][8] Standard-mode 0 - s
Fast-mode 0 - s
Fast-mode Plus 0 - s
tSU;DAT data set-up time [9][10] Standard-mode 250 - ns
Fast-mode 100 - ns
Fast-mode Plus 50 - ns
LPC11U3X All information provided in this document is subject to legal disclaimers. © NXP Semiconductors N.V. 2014. All rights reserved.
Product data sheet Rev. 2.2 — 11 March 2014 49 of 77
NXP Semiconductors LPC11U3x
32-bit ARM Cortex-M0 microcontroller
[7] In Fast-mode Plus, fall time is specified the same for both output stage and bus timing. If series resistors are used, designers should
allow for this when considering bus timing.
[8] The maximum tHD;DAT could be 3.45 s and 0.9 s for Standard-mode and Fast-mode but must be less than the maximum of tVD;DAT or
tVD;ACK by a transition time (see UM10204). This maximum must only be met if the device does not stretch the LOW period (tLOW) of the
SCL signal. If the clock stretches the SCL, the data must be valid by the set-up time before it releases the clock.
[9] tSU;DAT is the data set-up time that is measured with respect to the rising edge of SCL; applies to data in transmission and the
acknowledge.
[10] A Fast-mode I2C-bus device can be used in a Standard-mode I2C-bus system but the requirement tSU;DAT = 250 ns must then be met.
This will automatically be the case if the device does not stretch the LOW period of the SCL signal. If such a device does stretch the
LOW period of the SCL signal, it must output the next data bit to the SDA line tr(max) + tSU;DAT = 1000 + 250 = 1250 ns (according to the
Standard-mode I2C-bus specification) before the SCL line is released. Also the acknowledge timing must meet this set-up time.
Fig 23. I2C-bus pins clock timing
002aaf425
tf
70 %
SDA 30 %
tf
70 %
30 %
S
70 %
30 %
70 %
30 %
tHD;DAT
SCL
1 / fSCL
70 %
30 %
70 %
30 %
tVD;DAT
tHIGH
tLOW
tSU;DAT
LPC11U3X All information provided in this document is subject to legal disclaimers. © NXP Semiconductors N.V. 2014. All rights reserved.
Product data sheet Rev. 2.2 — 11 March 2014 50 of 77
NXP Semiconductors LPC11U3x
32-bit ARM Cortex-M0 microcontroller
10.6 SSP interface
[1] Tcy(clk) = (SSPCLKDIV (1 + SCR) CPSDVSR) / fmain. The clock cycle time derived from the SPI bit rate Tcy(clk) is a function of the
main clock frequency fmain, the SPI peripheral clock divider (SSPCLKDIV), the SPI SCR parameter (specified in the SSP0CR0 register),
and the SPI CPSDVSR parameter (specified in the SPI clock prescale register).
[2] Tamb = 40 C to 85 C.
[3] Tcy(clk) = 12 Tcy(PCLK).
[4] Tamb = 25 C; for normal voltage supply range: VDD = 3.3 V.
Table 16. Dynamic characteristics of SPI pins in SPI mode
Symbol Parameter Conditions Min Typ Max Unit
SPI master (in SPI mode)
Tcy(clk) clock cycle time full-duplex mode [1] 50 - - ns
when only transmitting [1] 40 ns
tDS data set-up time in SPI mode
2.4 V VDD 3.6 V
[2] 15 - - ns
2.0 V VDD < 2.4 V [2] 20 ns
1.8 V VDD < 2.0 V [2] 24 - - ns
tDH data hold time in SPI mode [2] 0 - - ns
tv(Q) data output valid time in SPI mode [2] - - 10 ns
th(Q) data output hold time in SPI mode [2] 0 - - ns
SPI slave (in SPI mode)
Tcy(PCLK) PCLK cycle time 20 - - ns
tDS data set-up time in SPI mode [3][4] 0 - - ns
tDH data hold time in SPI mode [3][4] 3 Tcy(PCLK) + 4 - - ns
tv(Q) data output valid time in SPI mode [3][4] - - 3 Tcy(PCLK) + 11 ns
th(Q) data output hold time in SPI mode [3][4] - - 2 Tcy(PCLK) + 5 ns
LPC11U3X All information provided in this document is subject to legal disclaimers. © NXP Semiconductors N.V. 2014. All rights reserved.
Product data sheet Rev. 2.2 — 11 March 2014 51 of 77
NXP Semiconductors LPC11U3x
32-bit ARM Cortex-M0 microcontroller
Fig 24. SSP master timing in SPI mode
SCK (CPOL = 0)
MOSI
MISO
Tcy(clk)
tDS tDH
tv(Q)
DATA VALID DATA VALID
th(Q)
SCK (CPOL = 1)
DATA VALID DATA VALID
MOSI
MISO
tDS tDH
DATA VALID DATA VALID
th(Q)
DATA VALID DATA VALID
tv(Q)
CPHA = 1
CPHA = 0
002aae829
LPC11U3X All information provided in this document is subject to legal disclaimers. © NXP Semiconductors N.V. 2014. All rights reserved.
Product data sheet Rev. 2.2 — 11 March 2014 52 of 77
NXP Semiconductors LPC11U3x
32-bit ARM Cortex-M0 microcontroller
Fig 25. SSP slave timing in SPI mode
SCK (CPOL = 0)
MOSI
MISO
Tcy(clk)
tDS tDH
tv(Q)
DATA VALID DATA VALID
th(Q)
SCK (CPOL = 1)
DATA VALID DATA VALID
MOSI
MISO
tDS tDH
tv(Q)
DATA VALID DATA VALID
th(Q)
DATA VALID DATA VALID
CPHA = 1
CPHA = 0
002aae830
LPC11U3X All information provided in this document is subject to legal disclaimers. © NXP Semiconductors N.V. 2014. All rights reserved.
Product data sheet Rev. 2.2 — 11 March 2014 53 of 77
NXP Semiconductors LPC11U3x
32-bit ARM Cortex-M0 microcontroller
10.7 USB interface
[1] Characterized but not implemented as production test. Guaranteed by design.
Table 17. Dynamic characteristics: USB pins (full-speed)
CL = 50 pF; Rpu = 1.5 k on D+ to VDD; 3.0 V VDD 3.6 V.
Symbol Parameter Conditions Min Typ Max Unit
tr rise time 10 % to 90 % 8.5 - 13.8 ns
tf fall time 10 % to 90 % 7.7 - 13.7 ns
tFRFM differential rise and fall time
matching
tr / tf - - 109 %
VCRS output signal crossover voltage 1.3 - 2.0 V
tFEOPT source SE0 interval of EOP see Figure 26 160 - 175 ns
tFDEOP source jitter for differential transition
to SE0 transition
see Figure 26 2 - +5 ns
tJR1 receiver jitter to next transition 18.5 - +18.5 ns
tJR2 receiver jitter for paired transitions 10 % to 90 % 9 - +9 ns
tEOPR EOP width at receiver must accept as
EOP; see
Figure 26
[1] 82 - - ns
Fig 26. Differential data-to-EOP transition skew and EOP width
aaa-009330
TPERIOD
differential
data lines
crossover point
source EOP width: tFEOPT
receiver EOP width: tEOPR
crossover point
extended
differential data to
SE0/EOP skew
n TPERIOD + tFDEOP
LPC11U3X All information provided in this document is subject to legal disclaimers. © NXP Semiconductors N.V. 2014. All rights reserved.
Product data sheet Rev. 2.2 — 11 March 2014 54 of 77
NXP Semiconductors LPC11U3x
32-bit ARM Cortex-M0 microcontroller
11. Application information
11.1 Suggested USB interface solutions
The USB device can be connected to the USB as self-powered device (see Figure 27) or
bus-powered device (see Figure 28).
On the LPC11U3x, the PIO0_3/USB_VBUS pin is 5 V tolerant only when VDD is applied
and at operating voltage level. Therefore, if the USB_VBUS function is connected to the
USB connector and the device is self-powered, the USB_VBUS pin must be protected for
situations when VDD = 0 V.
If VDD is always greater than 0 V while VBUS = 5 V, the USB_VBUS pin can be connected
directly to the VBUS pin on the USB connector.
For systems where VDD can be 0 V and VBUS is directly applied to the VBUS pin,
precautions must be taken to reduce the voltage to below 3.6 V, which is the maximum
allowable voltage on the USB_VBUS pin in this case.
One method is to use a voltage divider to connect the USB_VBUS pin to the VBUS on the
USB connector. The voltage divider ratio should be such that the USB_VBUS pin will be
greater than 0.7VDD to indicate a logic HIGH while below the 3.6 V allowable maximum
voltage.
For the following operating conditions
VBUSmax = 5.25 V
VDD = 3.6 V,
the voltage divider should provide a reduction of 3.6 V/5.25 V or ~0.686 V.
Fig 27. USB interface on a self-powered device where USB_VBUS = 5 V
LPC1xxx
USB-B
connector
USB_DP
USB_CONNECT
soft-connect switch
USB_DM
USB_VBUS
VSS
VDD
R1
1.5 kΩ
RS = 33 Ω
aaa-010178
RS = 33 Ω
R2
R3
LPC11U3X All information provided in this document is subject to legal disclaimers. © NXP Semiconductors N.V. 2014. All rights reserved.
Product data sheet Rev. 2.2 — 11 March 2014 55 of 77
NXP Semiconductors LPC11U3x
32-bit ARM Cortex-M0 microcontroller
For a bus-powered device, the VBUS signal does not need to be connected to the
USB_VBUS pin (see Figure 28). The USB_CONNECT function can additionally be
connected as shown in Figure 27 to prevent the USB from timing out when there is a
significant delay between power-up and handling USB traffic.
Remark: When a bus-powered circuit as shown in Figure 28 is used, configure the
PIO0_3/USB_VBUS pin for GPIO (PIO0_3) in the IOCON block to ensure that the
USB_CONNECT signal can still be controlled by software. For details on the soft-connect
feature, see the LPC11U3x user manual (Ref. 1).
Remark: When a self-powered circuit is used without connecting VBUS, configure the
PIO0_3/USB_VBUS pin for GPIO (PIO0_3) and provide software that can detect the host
presence through some other mechanism before enabling USB_CONNECT and the
soft-connect feature. Enabling the soft-connect without host presence will lead to USB
compliance failure.
Fig 28. USB interface on a bus-powered device
LPC1xxx
VDD
R1
1.5 kΩ
aaa-010179
USB-B
connector
USB_DP
USB_DM
VSS
RS = 33 Ω
RS = 33 Ω
REGULATOR
VBUS
LPC11U3X All information provided in this document is subject to legal disclaimers. © NXP Semiconductors N.V. 2014. All rights reserved.
Product data sheet Rev. 2.2 — 11 March 2014 56 of 77
NXP Semiconductors LPC11U3x
32-bit ARM Cortex-M0 microcontroller
11.2 XTAL input
The input voltage to the on-chip oscillators is limited to 1.8 V. If the oscillator is driven by a
clock in slave mode, it is recommended that the input be coupled through a capacitor with
Ci = 100 pF. To limit the input voltage to the specified range, choose an additional
capacitor to ground Cg which attenuates the input voltage by a factor Ci/(Ci + Cg). In slave
mode, a minimum of 200 mV (RMS) is needed.
In slave mode, couple the input clock signal with a capacitor of 100 pF (Figure 29), with an
amplitude between 200 mV (RMS) and 1000 mV (RMS). This signal corresponds to a
square wave signal with a signal swing of between 280 mV and 1.4 V. The XTALOUT pin
in this configuration can be left unconnected.
External components and models used in oscillation mode are shown in Figure 30 and in
Table 18 and Table 19. Since the feedback resistance is integrated on chip, only a crystal
and the capacitances CX1 and CX2 need to be connected externally in case of
fundamental mode oscillation (L, CL and RS represent the fundamental frequency).
Capacitance CP in Figure 30 represents the parallel package capacitance and must not be
larger than 7 pF. Parameters FOSC, CL, RS and CP are supplied by the crystal
manufacturer.
Fig 29. Slave mode operation of the on-chip oscillator
Fig 30. Oscillator modes and models: oscillation mode of operation and external crystal
model used for CX1/CX2 evaluation
LPC1xxx
XTALIN
Ci
100 pF
Cg
002aae788
002aaf424
LPC1xxx
XTALIN XTALOUT
CX1 CX2
XTAL
= CL CP
RS
L
LPC11U3X All information provided in this document is subject to legal disclaimers. © NXP Semiconductors N.V. 2014. All rights reserved.
Product data sheet Rev. 2.2 — 11 March 2014 57 of 77
NXP Semiconductors LPC11U3x
32-bit ARM Cortex-M0 microcontroller
11.3 XTAL Printed-Circuit Board (PCB) layout guidelines
Follow these guidelines for PCB layout:
• Connect the crystal on the PCB as close as possible to the oscillator input and output
pins of the chip.
• Take care that the load capacitors Cx1, Cx2, and Cx3 in case of third overtone crystal
use have a common ground plane.
• Connect the external components to the ground plain.
• To keep parasitics and the noise coupled in via the PCB as small as possible, keep
loops as small as possible.
• Choose smaller values of Cx1 and Cx2 if parasitics of the PCB layout increase.
Table 18. Recommended values for CX1/CX2 in oscillation mode (crystal and external
components parameters) low frequency mode
Fundamental oscillation
frequency FOSC
Crystal load
capacitance CL
Maximum crystal
series resistance RS
External load
capacitors CX1, CX2
1 MHz to 5 MHz 10 pF < 300 18 pF, 18 pF
20 pF < 300 39 pF, 39 pF
30 pF < 300 57 pF, 57 pF
5 MHz to 10 MHz 10 pF < 300 18 pF, 18 pF
20 pF < 200 39 pF, 39 pF
30 pF < 100 57 pF, 57 pF
10 MHz to 15 MHz 10 pF < 160 18 pF, 18 pF
20 pF < 60 39 pF, 39 pF
15 MHz to 20 MHz 10 pF < 80 18 pF, 18 pF
Table 19. Recommended values for CX1/CX2 in oscillation mode (crystal and external
components parameters) high frequency mode
Fundamental oscillation
frequency FOSC
Crystal load
capacitance CL
Maximum crystal
series resistance RS
External load
capacitors CX1, CX2
15 MHz to 20 MHz 10 pF < 180 18 pF, 18 pF
20 pF < 100 39 pF, 39 pF
20 MHz to 25 MHz 10 pF < 160 18 pF, 18 pF
20 pF < 80 39 pF, 39 pF
LPC11U3X All information provided in this document is subject to legal disclaimers. © NXP Semiconductors N.V. 2014. All rights reserved.
Product data sheet Rev. 2.2 — 11 March 2014 58 of 77
NXP Semiconductors LPC11U3x
32-bit ARM Cortex-M0 microcontroller
11.4 Standard I/O pad configuration
Figure 31 shows the possible pin modes for standard I/O pins with analog input function:
• Digital output driver
• Digital input: Pull-up enabled/disabled
• Digital input: Pull-down enabled/disabled
• Digital input: Repeater mode enabled/disabled
• Analog input
Fig 31. Standard I/O pad configuration
PIN
VDD VDD
ESD
VSS
ESD
strong
pull-up
strong
pull-down
VDD
weak
pull-up
weak
pull-down
open-drain enable
output enable
repeater mode
enable
pull-up enable
pull-down enable
select data
inverter
data output
data input
select glitch
filter
analog input
select analog input
002aaf695
pin configured
as digital output
driver
pin configured
as digital input
pin configured
as analog input
10 ns RC
GLITCH FILTER
LPC11U3X All information provided in this document is subject to legal disclaimers. © NXP Semiconductors N.V. 2014. All rights reserved.
Product data sheet Rev. 2.2 — 11 March 2014 59 of 77
NXP Semiconductors LPC11U3x
32-bit ARM Cortex-M0 microcontroller
11.5 Reset pad configuration
11.6 ADC effective input impedance
A simplified diagram of the ADC input channels can be used to determine the effective
input impedance seen from an external voltage source. See Figure 33.
The effective input impedance, Rin, seen by the external voltage source, VEXT, is the
parallel impedance of ((1/fs x Cia) + Rmux + Rsw) and (1/fs x Cio), and can be calculated
using Equation 1 with
fs = sampling frequency
Cia = ADC analog input capacitance
Rmux = analog mux resistance
Rsw = switch resistance
Cio = pin capacitance
(1)
Fig 32. Reset pad configuration
VSS
reset
002aaf274
VDD
VDD
VDD
Rpu ESD
ESD
20 ns RC
GLITCH FILTER
PIN
Fig 33. ADC input channel
Cia
Rs
VSS
VEXT
002aah615
ADC
COMPARATOR
ADC Block
Rin
Cio
Rmux Rsw
Source
<2 kΩ <1.3 kΩ
Rin
1
fs Cia ----------------- + Rmux + Rsw
1
fs Cio -----------------
=
LPC11U3X All information provided in this document is subject to legal disclaimers. © NXP Semiconductors N.V. 2014. All rights reserved.
Product data sheet Rev. 2.2 — 11 March 2014 60 of 77
NXP Semiconductors LPC11U3x
32-bit ARM Cortex-M0 microcontroller
Under nominal operating condition VDD = 3.3 V and with the maximum sampling
frequency fs = 400 kHz, the parameters assume the following values:
Cia = 1 pF (max)
Rmux = 2 kΩ (max)
Rsw = 1.3 kΩ (max)
Cio = 7.1 pF (max)
The effective input impedance with these parameters is Rin = 308 kΩ.
11.7 ADC usage notes
The following guidelines show how to increase the performance of the ADC in a noisy
environment beyond the ADC specifications listed in Table 6:
• The ADC input trace must be short and as close as possible to the LPC11U3x chip.
• Shield The ADC input traces from fast switching digital signals and noisy power
supply lines.
• The ADC and the digital core share the same power supply. Therefore, filter the power
supply line adequately.
• To improve the ADC performance in a noisy environment, put the device in Sleep
mode during the ADC conversion.
11.8 I/O Handler software library applications
The following sections provide application examples for the I/O Handler software library.
All library examples make use of the I/O Handler hardware to extend the functionality of
the part through software library calls. The libraries are available on
http://www.LPCware.com.
11.8.1 I/O Handler I2S
The I/O Handler software library provides functions to emulate an I2S master transmit
interface using the I/O Handler hardware block.
The emulated I2S interface loops over a 1 kB buffer, transmitting the datawords according
to the I2S protocol. Interrupts are generated every time when the first 512 bytes have been
transmitted and when the last 512 bytes have been transmitted. This allows the ARM core
to load the free portion of the buffer with new data, thereby enabling streaming audio.
Two channels with 16-bit per channel are supported. The code size of the software library
is 1 kB and code must be executed from the SRAM1 memory area reserved for the I/O
Handler code.
11.8.2 I/O Handler UART
The I/O Handler UART library emulates one additional full-duplex UART. The emulated
UART can be configured for 7 or 8 data bits, no parity, and 1 or 2 stop bits. The baud rate
is configurable up to 115200 baud. The RXD signal is available on three I/O Handler pins
(IOH_6, IOH_16, IOH_20), while TXD and CTS are available on all 21 I/O Handler pins.
The code size of the software library is about 1.2 kB and code must be executed from the
SRAM1 memory area reserved for the I/O Handler code.
LPC11U3X All information provided in this document is subject to legal disclaimers. © NXP Semiconductors N.V. 2014. All rights reserved.
Product data sheet Rev. 2.2 — 11 March 2014 61 of 77
NXP Semiconductors LPC11U3x
32-bit ARM Cortex-M0 microcontroller
11.8.3 I/O Handler I2C
The I/O Handler I2C library allows to have an additional I2C-bus master. I2C read, I2C write
and combined I2C read/write are supported. Data is automatically read from and written to
user-defined buffers.
The I/O Handler I2C library combined with the on-chip I2C module allows to have two
distinct I2C buses, allowing to separate low-speed from high-speed devices or bridging
two I2C buses.
11.8.4 I/O Handler DMA
The I/O Handler DMA library offers DMA-like functionality. Four types of transfer are
supported: memory to memory, memory to peripheral, peripheral to memory and
peripheral to peripheral. Supported peripherals are USART, SSP0/1, ADC and GPIO.
DMA transfers can be triggered by the source/target peripheral, software, counter/timer
module CT16B1, or I/O Handler pin PIO1_6/IOH_16.
LPC11U3X All information provided in this document is subject to legal disclaimers. © NXP Semiconductors N.V. 2014. All rights reserved.
Product data sheet Rev. 2.2 — 11 March 2014 62 of 77
NXP Semiconductors LPC11U3x
32-bit ARM Cortex-M0 microcontroller
12. Package outline
Fig 34. Package outline HVQFN33 (5 x 5 x 0.85 mm)
Outline References
version
European
projection Issue date
IEC JEDEC JEITA
MO-220
hvqfn33f_po
11-10-11
11-10-17
Unit(1)
mm
max
nom
min
0.85
0.05
0.00
0.2
5.1
4.9
3.75
3.45
5.1
4.9
3.75
3.45
0.5 3.5
A1
Dimensions (mm are the original dimensions)
Note
1. Plastic or metal protrusions of 0.075 mm maximum per side are not included.
HVQFN33: plastic thermal enhanced very thin quad flat package; no leads;
32 terminals; body 5 x 5 x 0.85 mm
b c
0.30
0.18
A(1) D(1) Dh E(1) Eh e e1 e2 L
3.5
v w
0.1 0.1
y
0.05
0.5
0.3
y1
0.05
0 2.5 5 mm
scale
1/2 e
v C A B
w C
terminal 1
index area
A
A1
c
detail X
y1 C y e
L
Eh
Dh
e
e1
b
9 16
32 25
24
8 17
1
X
D
E
C
B A
e2
terminal 1
index area
1/2 e
LPC11U3X All information provided in this document is subject to legal disclaimers. © NXP Semiconductors N.V. 2014. All rights reserved.
Product data sheet Rev. 2.2 — 11 March 2014 63 of 77
NXP Semiconductors LPC11U3x
32-bit ARM Cortex-M0 microcontroller
Fig 35. Package outline HVQFN33 (7 x 7 x 0.85 mm)
Outline References
version
European
projection
Issue date
IEC JEDEC JEITA
- - -
hvqfn33_po
09-03-17
09-03-23
Unit
mm
max
nom
min
1.00
0.85
0.80
0.05
0.02
0.00
0.2
7.1
7.0
6.9
4.85
4.70
4.55
7.1
7.0
6.9
0.65 4.55
0.75
0.60
0.45
0.1
A(1)
Dimensions
Note
1. Plastic or metal protrusions of 0.075 mm maximum per side are not included.
HVQFN33: plastic thermal enhanced very thin quad flat package; no leads;
33 terminals; body 7 x 7 x 0.85 mm
A1 b
0.35
0.28
0.23
c D(1) Dh E(1) Eh
4.85
4.70
4.55
e e1 e2
4.55
L v
0.1
w
0.05
y
0.08
y1
0 2.5 5 mm
scale
terminal 1
index area
D B A
E
C
y1 C y
X
detail X
A1
A
c
b
e2
e1
e
e
v C A B
w C
terminal 1
index area Dh
Eh
L
9 16
32
33
25
17
24
8
1
LPC11U3X All information provided in this document is subject to legal disclaimers. © NXP Semiconductors N.V. 2014. All rights reserved.
Product data sheet Rev. 2.2 — 11 March 2014 64 of 77
NXP Semiconductors LPC11U3x
32-bit ARM Cortex-M0 microcontroller
Fig 36. Package outline TFBGA48 (SOT1155-2)
Outline References
version
European
projection Issue date
IEC JEDEC JEITA
SOT1155-2 - - -
sot1155-2_po
13-06-17
13-06-19
Unit
mm
max
nom
min
1.10
0.95
0.85
0.30
0.25
0.20
0.35
0.30
0.25
4.6
4.5
4.4
4.6
4.5
4.4
0.5 3.5 0.15 0.08
A
Dimensions
TFBGA48: plastic thin fine-pitch ball grid array package; 48 balls; body 4.5 x 4.5 x 0.7 mm SOT1155-2
A1 A2
0.80
0.70
0.65
b D E e e1
3.5
e2 v w
0.05
y y1
0.1
0 5 mm
scale
ball A1
index area
D B A
E
A
B
C
D
E
F
H
G
1 2 3 4 5 6 7 8
b
e2
e1
e
e 1/2 e
1/2 e
ball A1
index area
solder mask open area
not for solder ball
C
y1 C y
X
detail X
A
A2
A1
Ø v C A B
Ø w C
LPC11U3X All information provided in this document is subject to legal disclaimers. © NXP Semiconductors N.V. 2014. All rights reserved.
Product data sheet Rev. 2.2 — 11 March 2014 65 of 77
NXP Semiconductors LPC11U3x
32-bit ARM Cortex-M0 microcontroller
Fig 37. Package outline LQFP48 (SOT313-2)
UNIT
A
max. A1 A2 A3 bp c E(1) e HE L Lp v w y Z θ
OUTLINE REFERENCES
VERSION
EUROPEAN
PROJECTION ISSUE DATE
IEC JEDEC JEITA
mm 1.6 0.20
0.05
1.45
1.35
0.25
0.27
0.17
0.18
0.12
7.1
6.9 0.5
9.15
8.85
0.95
0.55
7
0
o
1 0.2 0.12 0.1 o
DIMENSIONS (mm are the original dimensions)
Note
1. Plastic or metal protrusions of 0.25 mm maximum per side are not included.
0.75
0.45
SOT313-2 136E05 MS-026
00-01-19
03-02-25
D(1) (1) (1)
7.1
6.9
HD
9.15
8.85
Z E
0.95
0.55
D
bp
e
E
B
12
HD
bp
HE
v M B
D
ZD
A
ZE
e
v M A
1
48
37
36 25
24
13
θ
A1
A
Lp
detail X
L
(A 3 )
A2
X
y
c
w M
w M
0 2.5 5 mm
scale
pin 1 index
LQFP48: plastic low profile quad flat package; 48 leads; body 7 x 7 x 1.4 mm SOT313-2
LPC11U3X All information provided in this document is subject to legal disclaimers. © NXP Semiconductors N.V. 2014. All rights reserved.
Product data sheet Rev. 2.2 — 11 March 2014 66 of 77
NXP Semiconductors LPC11U3x
32-bit ARM Cortex-M0 microcontroller
Fig 38. Package outline LQFP64 (SOT314-2)
UNIT
A
max. A1 A2 A3 bp c E(1) e HE L Lp v w y Z θ
OUTLINE REFERENCES
VERSION
EUROPEAN
PROJECTION ISSUE DATE
IEC JEDEC JEITA
mm 1.6 0.20
0.05
1.45
1.35
0.25
0.27
0.17
0.18
0.12
10.1
9.9
0.5
12.15
11.85
1.45
1.05
7
0
o
1 0.2 0.12 0.1 o
DIMENSIONS (mm are the original dimensions)
Note
1. Plastic or metal protrusions of 0.25 mm maximum per side are not included.
0.75
0.45
SOT314-2 136E10 MS-026
00-01-19
03-02-25
D(1) (1) (1)
10.1
9.9
HD
12.15
11.85
Z E
1.45
1.05
D
bp
e
θ
E
A1
A
Lp
detail X
L
(A 3 )
B
16
c
HD
bp
HE A2
v M B
D
ZD
A
ZE
e
v M A
X
1
64
49
48 33
32
17
y
pin 1 index
w M
w M
0 2.5 5 mm
scale
LQFP64: plastic low profile quad flat package; 64 leads; body 10 x 10 x 1.4 mm SOT314-2
LPC11U3X All information provided in this document is subject to legal disclaimers. © NXP Semiconductors N.V. 2014. All rights reserved.
Product data sheet Rev. 2.2 — 11 March 2014 67 of 77
NXP Semiconductors LPC11U3x
32-bit ARM Cortex-M0 microcontroller
13. Soldering
Fig 39. Reflow soldering for the HVQFN33 (5x5) package
Footprint information for reflow soldering of HVQFN33 package
occupied area
solder paste
solder land
Dimensions in mm
P
0.5
Issue date 002aag766 11-11-15
11-11-20
Ax Ay Bx C D
5.95 5.95 4.25 0.85
By
4.25 0.27
Gx
5.25
Gy
5.25
Hy
6.2
Hx
6.2
SLx SLy nSPx nSPy
3.75 3.75 3 3
0.30
0.60
detail X
C
SLy
D
SLx
Bx
Ay
P
nSPy
nSPx
see detail X
Gx
Hx
Hy Gy By
Ax
LPC11U3X All information provided in this document is subject to legal disclaimers. © NXP Semiconductors N.V. 2014. All rights reserved.
Product data sheet Rev. 2.2 — 11 March 2014 68 of 77
NXP Semiconductors LPC11U3x
32-bit ARM Cortex-M0 microcontroller
Fig 40. Reflow soldering for the HVQFN33 (7x7) package
Footprint information for reflow soldering of HVQFN33 package
occupied area 001aao134
solder land
solder resist
solder land plus solder paste
solder paste deposit
Dimensions in mm
Remark:
Stencil thickness: 0.125 mm
e = 0.65
evia = 4.25
OwDtot = 5.10 OA
PID = 7.25 PA+OA
OID = 8.20 OA
0.20 SR
chamfer (4×)
0.45 DM
evia = 1.05
W = 0.30 CU
evia = 4.25
evia = 2.40
LbE = 5.80 CU
LbD = 5.80 CU
PIE = 7.25 PA+OA
LaE = 7.95 CU
LaD = 7.95 CU
OIE = 8.20 OA
OwEtot = 5.10 OA
EHS = 4.85 CU
DHS = 4.85 CU
4.55 SR
4.55 SR
B-side
(A-side fully covered)
number of vias: 20
Solder resist
covered via
0.30 PH
0.60 SR cover
0.60 CU
SEhtot = 2.70 SP
SDhtot = 2.70 SP
GapE = 0.70 SP
SPE = 1.00 SP
SPD = 1.00 SP 0.45 DM
GapD = 0.70 SP
LPC11U3X All information provided in this document is subject to legal disclaimers. © NXP Semiconductors N.V. 2014. All rights reserved.
Product data sheet Rev. 2.2 — 11 March 2014 69 of 77
NXP Semiconductors LPC11U3x
32-bit ARM Cortex-M0 microcontroller
Fig 41. Reflow soldering for the TFBGA48 package
DIMENSIONS in mm
P SL SP SR Hx Hy
Hx
Hy
SOT1155-2
solder land plus solder paste
occupied area
Footprint information for reflow soldering of TFBGA48 package
solder land
solder paste deposit
solder resist
P
P
SL
SP
SR
detail X
see detail X
0.50 0.225 0.275 0.325 4.75 4.75 sot1155-2_fr
LPC11U3X All information provided in this document is subject to legal disclaimers. © NXP Semiconductors N.V. 2014. All rights reserved.
Product data sheet Rev. 2.2 — 11 March 2014 70 of 77
NXP Semiconductors LPC11U3x
32-bit ARM Cortex-M0 microcontroller
Fig 42. Reflow soldering for the LQFP48 package
SOT313-2
DIMENSIONS in mm
occupied area
Footprint information for reflow soldering of LQFP48 package
Ax
Bx
Gx
Hy Gy
Hx
By Ay
P1
D2 (8×) D1
(0.125)
Ax Ay Bx By D1 D2 Gx Gy Hx Hy
10.350
P2
0.560 10.350 7.350 7.350
P1
0.500 0.280
C
1.500 0.500 7.500 7.500 10.650 10.650
sot313-2_fr
solder land
C
Generic footprint pattern
Refer to the package outline drawing for actual layout
P2
LPC11U3X All information provided in this document is subject to legal disclaimers. © NXP Semiconductors N.V. 2014. All rights reserved.
Product data sheet Rev. 2.2 — 11 March 2014 71 of 77
NXP Semiconductors LPC11U3x
32-bit ARM Cortex-M0 microcontroller
Fig 43. Reflow soldering for the LQFP64 package
SOT314-2
DIMENSIONS in mm
occupied area
Footprint information for reflow soldering of LQFP64 package
Ax
Bx
Gx
Hy Gy
Hx
By Ay
P2 P1
D2 (8×) D1
(0.125)
Ax Ay Bx By D1 D2 Gx Gy Hx Hy
13.300 13.300 10.300 10.300
P1
0.500
P2
0.560 0.280
C
1.500 0.400 10.500 10.500 13.550 13.550
sot314-2_fr
solder land
C
Generic footprint pattern
Refer to the package outline drawing for actual layout
LPC11U3X All information provided in this document is subject to legal disclaimers. © NXP Semiconductors N.V. 2014. All rights reserved.
Product data sheet Rev. 2.2 — 11 March 2014 72 of 77
NXP Semiconductors LPC11U3x
32-bit ARM Cortex-M0 microcontroller
14. Abbreviations
15. References
[1] LPC11U3x User manual UM10462:
http://www.nxp.com/documents/user_manual/UM10462.pdf
[2] LPC11U3x Errata sheet:
http://www.nxp.com/documents/errata_sheet/ES_LPC11U3X.pdf
Table 20. Abbreviations
Acronym Description
A/D Analog-to-Digital
ADC Analog-to-Digital Converter
AHB Advanced High-performance Bus
APB Advanced Peripheral Bus
BOD BrownOut Detection
GPIO General Purpose Input/Output
JTAG Joint Test Action Group
PLL Phase-Locked Loop
RC Resistor-Capacitor
SPI Serial Peripheral Interface
SSI Serial Synchronous Interface
SSP Synchronous Serial Port
TAP Test Access Port
USART Universal Synchronous Asynchronous Receiver/Transmitter
LPC11U3X All information provided in this document is subject to legal disclaimers. © NXP Semiconductors N.V. 2014. All rights reserved.
Product data sheet Rev. 2.2 — 11 March 2014 73 of 77
NXP Semiconductors LPC11U3x
32-bit ARM Cortex-M0 microcontroller
16. Revision history
Table 21. Revision history
Document ID Release date Data sheet status Change notice Supersedes
LPC11U3X v.2.2 20140311 Product data sheet - LPC11U3X v.2.1
Modifications: • Use of USB_CONNECT signal explained in Section 11.1 “Suggested USB interface
solutions”.
• Open-drain I2C-bus and RESET pin descriptions clarified. See Table 3.
LPC11U3X v.2.1 20131230 Product data sheet - LPC11U3X v.2
Modifications: Add reserved function to pins PIO0_8/MISO0/CT16B0_MAT0/R/IOH_6 and
PIO0_9/MOSI0/CT16B0_MAT1/R/IOH_7.
LPC11U3X v.2 20131125 Product data sheet - LPC11U3X v.1.1
Modifications: • Part LPC11U37HFBD64/401 with I/O handler added.
• Additional I/O Handler pin functions added in Table 3.
• Typical range of watchdog oscillator frequency changed to 9.4 kHz to
2.3 MHz.See Table 13.
• Section 11.8 “I/O Handler software library applications” added.
• Updated Section 11.1 “Suggested USB interface solutions” for clarity.
• Condition VDD = 0 V added to Parameter VI in Table 5 for clarity.
LPC11U3X v.1.1 20130924 Product data sheet - LPC11U3X v.1
Modifications: • Removed the footnote “The peak current is limited to 25 times the
corresponding maximum current.” in Table 4.
• Table 3: Added “5 V tolerant pad” to RESET/PIO0_0 table note.
• Table 7: Removed BOD interrupt level 0.
• Programmable glitch filter is enabled by default. See Section 7.7.1.
• Added Section 11.6 “ADC effective input impedance”.
• Table 5 “Static characteristics” added Pin capacitance section.
• Updated Section 11.1 “Suggested USB interface solutions”.
• Table 4 “Limiting values”:
– Updated VDD min and max.
– Updated VI conditions.
• Table 10 “EEPROM characteristics”:
– Removed fclk and ter; the user does not have control over these
parameters.
– Changed the tprog from 1.1 ms to 2.9 ms; the EEPROM IAP always
does an erase and program, thus the total program time is ter + tprog.
• Changed title of Figure 29 from “USB interface on a self-powered
device” to “USB interface with soft-connect”.
• Section 10.7 “USB interface” added. Parameter tEOPR1 and tEOPR2
renamed to tEOPR.
LPC11U3X v.1 20120420 Product data sheet - -
LPC11U3X All information provided in this document is subject to legal disclaimers. © NXP Semiconductors N.V. 2014. All rights reserved.
Product data sheet Rev. 2.2 — 11 March 2014 74 of 77
NXP Semiconductors LPC11U3x
32-bit ARM Cortex-M0 microcontroller
17. Legal information
17.1 Data sheet status
[1] Please consult the most recently issued document before initiating or completing a design.
[2] The term ‘short data sheet’ is explained in section “Definitions”.
[3] The product status of device(s) described in this document may have changed since this document was published and may differ in case of multiple devices. The latest product status
information is available on the Internet at URL http://www.nxp.com.
17.2 Definitions
Draft — The document is a draft version only. The content is still under
internal review and subject to formal approval, which may result in
modifications or additions. NXP Semiconductors does not give any
representations or warranties as to the accuracy or completeness of
information included herein and shall have no liability for the consequences of
use of such information.
Short data sheet — A short data sheet is an extract from a full data sheet
with the same product type number(s) and title. A short data sheet is intended
for quick reference only and should not be relied upon to contain detailed and
full information. For detailed and full information see the relevant full data
sheet, which is available on request via the local NXP Semiconductors sales
office. In case of any inconsistency or conflict with the short data sheet, the
full data sheet shall prevail.
Product specification — The information and data provided in a Product
data sheet shall define the specification of the product as agreed between
NXP Semiconductors and its customer, unless NXP Semiconductors and
customer have explicitly agreed otherwise in writing. In no event however,
shall an agreement be valid in which the NXP Semiconductors product is
deemed to offer functions and qualities beyond those described in the
Product data sheet.
17.3 Disclaimers
Limited warranty and liability — Information in this document is believed to
be accurate and reliable. However, NXP Semiconductors does not give any
representations or warranties, expressed or implied, as to the accuracy or
completeness of such information and shall have no liability for the
consequences of use of such information. NXP Semiconductors takes no
responsibility for the content in this document if provided by an information
source outside of NXP Semiconductors.
In no event shall NXP Semiconductors be liable for any indirect, incidental,
punitive, special or consequential damages (including - without limitation - lost
profits, lost savings, business interruption, costs related to the removal or
replacement of any products or rework charges) whether or not such
damages are based on tort (including negligence), warranty, breach of
contract or any other legal theory.
Notwithstanding any damages that customer might incur for any reason
whatsoever, NXP Semiconductors’ aggregate and cumulative liability towards
customer for the products described herein shall be limited in accordance
with the Terms and conditions of commercial sale of NXP Semiconductors.
Right to make changes — NXP Semiconductors reserves the right to make
changes to information published in this document, including without
limitation specifications and product descriptions, at any time and without
notice. This document supersedes and replaces all information supplied prior
to the publication hereof.
Suitability for use — NXP Semiconductors products are not designed,
authorized or warranted to be suitable for use in life support, life-critical or
safety-critical systems or equipment, nor in applications where failure or
malfunction of an NXP Semiconductors product can reasonably be expected
to result in personal injury, death or severe property or environmental
damage. NXP Semiconductors and its suppliers accept no liability for
inclusion and/or use of NXP Semiconductors products in such equipment or
applications and therefore such inclusion and/or use is at the customer’s own
risk.
Applications — Applications that are described herein for any of these
products are for illustrative purposes only. NXP Semiconductors makes no
representation or warranty that such applications will be suitable for the
specified use without further testing or modification.
Customers are responsible for the design and operation of their applications
and products using NXP Semiconductors products, and NXP Semiconductors
accepts no liability for any assistance with applications or customer product
design. It is customer’s sole responsibility to determine whether the NXP
Semiconductors product is suitable and fit for the customer’s applications and
products planned, as well as for the planned application and use of
customer’s third party customer(s). Customers should provide appropriate
design and operating safeguards to minimize the risks associated with their
applications and products.
NXP Semiconductors does not accept any liability related to any default,
damage, costs or problem which is based on any weakness or default in the
customer’s applications or products, or the application or use by customer’s
third party customer(s). Customer is responsible for doing all necessary
testing for the customer’s applications and products using NXP
Semiconductors products in order to avoid a default of the applications and
the products or of the application or use by customer’s third party
customer(s). NXP does not accept any liability in this respect.
Limiting values — Stress above one or more limiting values (as defined in
the Absolute Maximum Ratings System of IEC 60134) will cause permanent
damage to the device. Limiting values are stress ratings only and (proper)
operation of the device at these or any other conditions above those given in
the Recommended operating conditions section (if present) or the
Characteristics sections of this document is not warranted. Constant or
repeated exposure to limiting values will permanently and irreversibly affect
the quality and reliability of the device.
Terms and conditions of commercial sale — NXP Semiconductors
products are sold subject to the general terms and conditions of commercial
sale, as published at http://www.nxp.com/profile/terms, unless otherwise
agreed in a valid written individual agreement. In case an individual
agreement is concluded only the terms and conditions of the respective
agreement shall apply. NXP Semiconductors hereby expressly objects to
applying the customer’s general terms and conditions with regard to the
purchase of NXP Semiconductors products by customer.
No offer to sell or license — Nothing in this document may be interpreted or
construed as an offer to sell products that is open for acceptance or the grant,
conveyance or implication of any license under any copyrights, patents or
other industrial or intellectual property rights.
Document status[1][2] Product status[3] Definition
Objective [short] data sheet Development This document contains data from the objective specification for product development.
Preliminary [short] data sheet Qualification This document contains data from the preliminary specification.
Product [short] data sheet Production This document contains the product specification.
LPC11U3X All information provided in this document is subject to legal disclaimers. © NXP Semiconductors N.V. 2014. All rights reserved.
Product data sheet Rev. 2.2 — 11 March 2014 75 of 77
NXP Semiconductors LPC11U3x
32-bit ARM Cortex-M0 microcontroller
Export control — This document as well as the item(s) described herein
may be subject to export control regulations. Export might require a prior
authorization from competent authorities.
Non-automotive qualified products — Unless this data sheet expressly
states that this specific NXP Semiconductors product is automotive qualified,
the product is not suitable for automotive use. It is neither qualified nor tested
in accordance with automotive testing or application requirements. NXP
Semiconductors accepts no liability for inclusion and/or use of
non-automotive qualified products in automotive equipment or applications.
In the event that customer uses the product for design-in and use in
automotive applications to automotive specifications and standards, customer
(a) shall use the product without NXP Semiconductors’ warranty of the
product for such automotive applications, use and specifications, and (b)
whenever customer uses the product for automotive applications beyond
NXP Semiconductors’ specifications such use shall be solely at customer’s
own risk, and (c) customer fully indemnifies NXP Semiconductors for any
liability, damages or failed product claims resulting from customer design and
use of the product for automotive applications beyond NXP Semiconductors’
standard warranty and NXP Semiconductors’ product specifications.
17.4 Trademarks
Notice: All referenced brands, product names, service names and trademarks
are the property of their respective owners.
I2C-bus — logo is a trademark of NXP Semiconductors N.V.
18. Contact information
For more information, please visit: http://www.nxp.com
For sales office addresses, please send an email to: salesaddresses@nxp.com
LPC11U3X All information provided in this document is subject to legal disclaimers. © NXP Semiconductors N.V. 2014. All rights reserved.
Product data sheet Rev. 2.2 — 11 March 2014 76 of 77
continued >>
NXP Semiconductors LPC11U3x
32-bit ARM Cortex-M0 microcontroller
19. Contents
1 General description . . . . . . . . . . . . . . . . . . . . . . 1
2 Features and benefits . . . . . . . . . . . . . . . . . . . . 1
3 Applications . . . . . . . . . . . . . . . . . . . . . . . . . . . . 3
4 Ordering information. . . . . . . . . . . . . . . . . . . . . 3
4.1 Ordering options . . . . . . . . . . . . . . . . . . . . . . . . 4
5 Block diagram . . . . . . . . . . . . . . . . . . . . . . . . . . 5
6 Pinning information. . . . . . . . . . . . . . . . . . . . . . 6
6.1 Pinning . . . . . . . . . . . . . . . . . . . . . . . . . . . . . . . 6
6.2 Pin description . . . . . . . . . . . . . . . . . . . . . . . . 10
7 Functional description . . . . . . . . . . . . . . . . . . 17
7.1 On-chip flash programming memory . . . . . . . 17
7.2 EEPROM . . . . . . . . . . . . . . . . . . . . . . . . . . . . 17
7.3 SRAM . . . . . . . . . . . . . . . . . . . . . . . . . . . . . . . 17
7.4 On-chip ROM . . . . . . . . . . . . . . . . . . . . . . . . . 17
7.5 Memory map. . . . . . . . . . . . . . . . . . . . . . . . . . 17
7.6 Nested Vectored Interrupt Controller
(NVIC). . . . . . . . . . . . . . . . . . . . . . . . . . . . . . . 18
7.6.1 Features . . . . . . . . . . . . . . . . . . . . . . . . . . . . . 18
7.6.2 Interrupt sources. . . . . . . . . . . . . . . . . . . . . . . 19
7.7 IOCON block . . . . . . . . . . . . . . . . . . . . . . . . . 19
7.7.1 Features . . . . . . . . . . . . . . . . . . . . . . . . . . . . . 19
7.8 General-Purpose Input/Output GPIO . . . . . . . 19
7.8.1 Features . . . . . . . . . . . . . . . . . . . . . . . . . . . . . 20
7.9 USB interface . . . . . . . . . . . . . . . . . . . . . . . . 20
7.9.1 Full-speed USB device controller . . . . . . . . . . 20
7.9.1.1 Features . . . . . . . . . . . . . . . . . . . . . . . . . . . . . 20
7.10 I/O Handler
(LPC11U37HFBD64/401 only) . . . . . . . . . . . . 20
7.11 USART . . . . . . . . . . . . . . . . . . . . . . . . . . . . . . 21
7.11.1 Features . . . . . . . . . . . . . . . . . . . . . . . . . . . . . 21
7.12 SSP serial I/O controller . . . . . . . . . . . . . . . . . 21
7.12.1 Features . . . . . . . . . . . . . . . . . . . . . . . . . . . . . 21
7.13 I2C-bus serial I/O controller . . . . . . . . . . . . . . 22
7.13.1 Features . . . . . . . . . . . . . . . . . . . . . . . . . . . . . 22
7.14 10-bit ADC . . . . . . . . . . . . . . . . . . . . . . . . . . . 22
7.14.1 Features . . . . . . . . . . . . . . . . . . . . . . . . . . . . . 22
7.15 General purpose external event
counter/timers. . . . . . . . . . . . . . . . . . . . . . . . . 23
7.15.1 Features . . . . . . . . . . . . . . . . . . . . . . . . . . . . . 23
7.16 System tick timer . . . . . . . . . . . . . . . . . . . . . . 23
7.17 Windowed WatchDog Timer
(WWDT) . . . . . . . . . . . . . . . . . . . . . . . . . . . . . 23
7.17.1 Features . . . . . . . . . . . . . . . . . . . . . . . . . . . . . 23
7.18 Clocking and power control . . . . . . . . . . . . . . 24
7.18.1 Integrated oscillators . . . . . . . . . . . . . . . . . . . 24
7.18.1.1 Internal RC oscillator . . . . . . . . . . . . . . . . . . . 25
7.18.1.2 System oscillator . . . . . . . . . . . . . . . . . . . . . . 26
7.18.1.3 Watchdog oscillator . . . . . . . . . . . . . . . . . . . . 26
7.18.2 System PLL and USB PLL. . . . . . . . . . . . . . . 26
7.18.3 Clock output . . . . . . . . . . . . . . . . . . . . . . . . . . 26
7.18.4 Wake-up process . . . . . . . . . . . . . . . . . . . . . . 26
7.18.5 Power control . . . . . . . . . . . . . . . . . . . . . . . . . 26
7.18.5.1 Power profiles . . . . . . . . . . . . . . . . . . . . . . . . 27
7.18.5.2 Sleep mode . . . . . . . . . . . . . . . . . . . . . . . . . . 27
7.18.5.3 Deep-sleep mode. . . . . . . . . . . . . . . . . . . . . . 27
7.18.5.4 Power-down mode. . . . . . . . . . . . . . . . . . . . . 27
7.18.5.5 Deep power-down mode . . . . . . . . . . . . . . . . 28
7.18.6 System control . . . . . . . . . . . . . . . . . . . . . . . . 28
7.18.6.1 Reset . . . . . . . . . . . . . . . . . . . . . . . . . . . . . . . 28
7.18.6.2 Brownout detection . . . . . . . . . . . . . . . . . . . . 28
7.18.6.3 Code security
(Code Read Protection - CRP) . . . . . . . . . . . 28
7.18.6.4 APB interface . . . . . . . . . . . . . . . . . . . . . . . . . 29
7.18.6.5 AHBLite . . . . . . . . . . . . . . . . . . . . . . . . . . . . . 29
7.18.6.6 External interrupt inputs . . . . . . . . . . . . . . . . . 29
7.19 Emulation and debugging . . . . . . . . . . . . . . . 30
8 Limiting values . . . . . . . . . . . . . . . . . . . . . . . . 31
9 Static characteristics . . . . . . . . . . . . . . . . . . . 32
9.1 BOD static characteristics . . . . . . . . . . . . . . . 38
9.2 Power consumption . . . . . . . . . . . . . . . . . . . 38
9.3 Peripheral power consumption . . . . . . . . . . . 41
9.4 Electrical pin characteristics. . . . . . . . . . . . . . 43
10 Dynamic characteristics. . . . . . . . . . . . . . . . . 46
10.1 Flash memory . . . . . . . . . . . . . . . . . . . . . . . . 46
10.2 External clock. . . . . . . . . . . . . . . . . . . . . . . . . 46
10.3 Internal oscillators . . . . . . . . . . . . . . . . . . . . . 47
10.4 I/O pins . . . . . . . . . . . . . . . . . . . . . . . . . . . . . 48
10.5 I2C-bus. . . . . . . . . . . . . . . . . . . . . . . . . . . . . . 48
10.6 SSP interface . . . . . . . . . . . . . . . . . . . . . . . . . 50
10.7 USB interface . . . . . . . . . . . . . . . . . . . . . . . . 53
11 Application information . . . . . . . . . . . . . . . . . 54
11.1 Suggested USB interface solutions . . . . . . . . 54
11.2 XTAL input . . . . . . . . . . . . . . . . . . . . . . . . . . . 56
11.3 XTAL Printed-Circuit Board
(PCB) layout guidelines . . . . . . . . . . . . . . . . . 57
11.4 Standard I/O pad configuration . . . . . . . . . . . 58
11.5 Reset pad configuration . . . . . . . . . . . . . . . . . 59
11.6 ADC effective input impedance . . . . . . . . . . . 59
11.7 ADC usage notes. . . . . . . . . . . . . . . . . . . . . . 60
11.8 I/O Handler software library applications . . . . 60
11.8.1 I/O Handler I2S. . . . . . . . . . . . . . . . . . . . . . . . 60
11.8.2 I/O Handler UART . . . . . . . . . . . . . . . . . . . . . 60
11.8.3 I/O Handler I2C. . . . . . . . . . . . . . . . . . . . . . . . 61
11.8.4 I/O Handler DMA . . . . . . . . . . . . . . . . . . . . . . 61
NXP Semiconductors LPC11U3x
32-bit ARM Cortex-M0 microcontroller
© NXP Semiconductors N.V. 2014. All rights reserved.
For more information, please visit: http://www.nxp.com
For sales office addresses, please send an email to: salesaddresses@nxp.com
Date of release: 11 March 2014
Document identifier: LPC11U3X
Please be aware that important notices concerning this document and the product(s)
described herein, have been included in section ‘Legal information’.
12 Package outline . . . . . . . . . . . . . . . . . . . . . . . . 62
13 Soldering . . . . . . . . . . . . . . . . . . . . . . . . . . . . . 67
14 Abbreviations. . . . . . . . . . . . . . . . . . . . . . . . . . 72
15 References . . . . . . . . . . . . . . . . . . . . . . . . . . . . 72
16 Revision history. . . . . . . . . . . . . . . . . . . . . . . . 73
17 Legal information. . . . . . . . . . . . . . . . . . . . . . . 74
17.1 Data sheet status . . . . . . . . . . . . . . . . . . . . . . 74
17.2 Definitions. . . . . . . . . . . . . . . . . . . . . . . . . . . . 74
17.3 Disclaimers . . . . . . . . . . . . . . . . . . . . . . . . . . . 74
17.4 Trademarks. . . . . . . . . . . . . . . . . . . . . . . . . . . 75
18 Contact information. . . . . . . . . . . . . . . . . . . . . 75
19 Contents . . . . . . . . . . . . . . . . . . . . . . . . . . . . . . 76
1. General description
The PCA9545A/45B/45C is a quad bidirectional translating switch controlled via the
I2C-bus. The SCL/SDA upstream pair fans out to four downstream pairs, or channels. Any
individual SCx/SDx channel or combination of channels can be selected, determined by
the contents of the programmable control register. Four interrupt inputs, INT0 to INT3, one
for each of the downstream pairs, are provided. One interrupt output, INT, acts as an AND
of the four interrupt inputs.
An active LOW reset input allows the PCA9545A/45B/45C to recover from a situation
where one of the downstream I2C-buses is stuck in a LOW state. Pulling the RESET pin
LOW resets the I2C-bus state machine and causes all the channels to be deselected as
does the internal power-on reset function.
The pass gates of the switches are constructed such that the VDD pin can be used to limit
the maximum high voltage which is passed by the PCA9545A/45B/45C. This allows the
use of different bus voltages on each pair, so that 1.8 V or 2.5 V or 3.3 V parts can
communicate with 5 V parts without any additional protection. External pull-up resistors
pull the bus up to the desired voltage level for each channel. All I/O pins are 5 V tolerant.
The PCA9545A, PCA9545B and PCA9545C are identical except for the fixed portion of
the slave address.
2. Features and benefits
1-of-4 bidirectional translating switches
I2C-bus interface logic; compatible with SMBus standards
4 active LOW interrupt inputs
Active LOW interrupt output
Active LOW reset input
2 address pins allowing up to 4 devices on the I2C-bus
Alternate address versions A, B and C allow up to a total of 12 devices on the bus for
larger systems or to resolve address conflicts
Channel selection via I2C-bus, in any combination
Power-up with all switch channels deselected
Low Ron switches
Allows voltage level translation between 1.8 V, 2.5 V, 3.3 V and 5 V buses
No glitch on power-up
Supports hot insertion
Low standby current
Operating power supply voltage range of 2.3 V to 5.5 V
PCA9545A/45B/45C
4-channel I2C-bus switch with interrupt logic and reset
Rev. 9 — 5 May 2014 Product data sheet
PCA9545A_45B_45C All information provided in this document is subject to legal disclaimers. © NXP Semiconductors N.V. 2014. All rights reserved.
Product data sheet Rev. 9 — 5 May 2014 2 of 32
NXP Semiconductors PCA9545A/45B/45C
4-channel I2C-bus switch with interrupt logic and reset
5 V tolerant Inputs
0 Hz to 400 kHz clock frequency
ESD protection exceeds 2000 V HBM per JESD22-A114 and 1000 V CDM per
JESD22-C101
Latch-up protection exceeds 100 mA per JESD78
Three packages offered: SO20, TSSOP20, and HVQFN20
3. Ordering information
3.1 Ordering options
Table 1. Ordering information
Type number Topside
marking
Package
Name Description Version
PCA9545ABS 9545A HVQFN20 plastic thermal enhanced very thin quad flat package;
no leads; 20 terminals; body 5 5 0.85 mm
SOT662-1
PCA9545AD PCA9545AD SO20 plastic small outline package; 20 leads;
body width 7.5 mm
SOT163-1
PCA9545APW PA9545A TSSOP20 plastic thin shrink small outline package; 20 leads;
body width 4.4 mm
SOT360-1
PCA9545BPW PA9545B
PCA9545CPW PA9545C
Table 2. Ordering options
Type number Orderable
part number
Package Packing method Minimum
order
quantity
Temperature range
PCA9545ABS PCA9545ABS,118 HVQFN20 Reel 13” Q1/T1
*standard mark SMD
6000 Tamb = 40 C to +85 C
PCA9545AD PCA9545AD,112 SO20 Standard marking
* IC’s tube - DSC bulk pack
1520 Tamb = 40 C to +85 C
PCA9545AD,118 SO20 Reel 13” Q1/T1
*standard mark SMD
2000 Tamb = 40 C to +85 C
PCA9545APW PCA9545APW,112 TSSOP20 Standard marking
* IC’s tube - DSC bulk pack
1875 Tamb = 40 C to +85 C
PCA9545APW,118 TSSOP20 Reel 13” Q1/T1
*standard mark SMD
2500 Tamb = 40 C to +85 C
PCA9545BPW PCA9545BPW,118 TSSOP20 Reel 13” Q1/T1
*standard mark SMD
2500 Tamb = 40 C to +85 C
PCA9545CPW PCA9545CPW,118 TSSOP20 Reel 13” Q1/T1
*standard mark SMD
2500 Tamb = 40 C to +85 C
PCA9545A_45B_45C All information provided in this document is subject to legal disclaimers. © NXP Semiconductors N.V. 2014. All rights reserved.
Product data sheet Rev. 9 — 5 May 2014 3 of 32
NXP Semiconductors PCA9545A/45B/45C
4-channel I2C-bus switch with interrupt logic and reset
4. Block diagram
Fig 1. Block diagram of PCA9545A/45B/45C
SWITCH CONTROL LOGIC
PCA9545A/PCA9545B/PCA9545C
POWER-ON
RESET
002aab168
SC0
SC1
SC2
SC3
SD0
SD1
SD2
SD3
VSS
VDD
RESET
I2C-BUS
CONTROL
INPUT
FILTER
SCL
SDA
A0
A1
INTERRUPT LOGIC
INT0
to
INT3
INT
PCA9545A_45B_45C All information provided in this document is subject to legal disclaimers. © NXP Semiconductors N.V. 2014. All rights reserved.
Product data sheet Rev. 9 — 5 May 2014 4 of 32
NXP Semiconductors PCA9545A/45B/45C
4-channel I2C-bus switch with interrupt logic and reset
5. Pinning information
5.1 Pinning
Fig 2. Pin configuration for SO20 Fig 3. Pin configuration for TSSOP20
Fig 4. Pin configuration for HVQFN20 (transparent top view)
PCA9545AD
A0 VDD
A1 SDA
RESET SCL
INT0 INT
SD0 SC3
SC0 SD3
INT1 INT3
SD1 SC2
SC1 SD2
VSS INT2
002aab165
1
2
3
4
5
6
7
8
9
10
12
11
14
13
16
15
18
17
20
19
VDD
SDA
SCL
INT
SC3
SD3
INT3
SC2
SD2
INT2
A0
A1
RESET
INT0
SD0
SC0
INT1
SD1
SC1
VSS
PCA9545APW
PCA9545BPW
PCA9545CPW
002aab166
1
2
3
4
5
6
7
8
9
10
12
11
14
13
16
15
18
17
20
19
VDD
SDA
SCL
INT
SC3
SD3
INT3
SC2
A0
A1
RESET
INT0
SD0
SC0
INT1
SD1
SC1
VSS
002aab167
PCA9545ABS
Transparent top view
5 11
4 12
3 13
2 14
1 15
6
7
8
9
10
20
19
18
17
16
terminal 1
index area
SD2
INT2
PCA9545A_45B_45C All information provided in this document is subject to legal disclaimers. © NXP Semiconductors N.V. 2014. All rights reserved.
Product data sheet Rev. 9 — 5 May 2014 5 of 32
NXP Semiconductors PCA9545A/45B/45C
4-channel I2C-bus switch with interrupt logic and reset
5.2 Pin description
[1] HVQFN20 package die supply ground is connected to both the VSS pin and the exposed center pad. The
VSS pin must be connected to supply ground for proper device operation. For enhanced thermal, electrical,
and board-level performance, the exposed pad must be soldered to the board using a corresponding
thermal pad on the board, and for proper heat conduction through the board thermal vias must be
incorporated in the PCB in the thermal pad region.
Table 3. Pin description
Symbol Pin Description
SO20, TSSOP20 HVQFN20
A0 1 19 address input 0
A1 2 20 address input 1
RESET 3 1 active LOW reset input
INT0 4 2 active LOW interrupt input 0
SD0 5 3 serial data 0
SC0 6 4 serial clock 0
INT1 7 5 active LOW interrupt input 1
SD1 8 6 serial data 1
SC1 9 7 serial clock 1
VSS 10 8[1] supply ground
INT2 11 9 active LOW interrupt input 2
SD2 12 10 serial data 2
SC2 13 11 serial clock 2
INT3 14 12 active LOW interrupt input 3
SD3 15 13 serial data 3
SC3 16 14 serial clock 3
INT 17 15 active LOW interrupt output
SCL 18 16 serial clock line
SDA 19 17 serial data line
VDD 20 18 supply voltage
PCA9545A_45B_45C All information provided in this document is subject to legal disclaimers. © NXP Semiconductors N.V. 2014. All rights reserved.
Product data sheet Rev. 9 — 5 May 2014 6 of 32
NXP Semiconductors PCA9545A/45B/45C
4-channel I2C-bus switch with interrupt logic and reset
6. Functional description
Refer to Figure 1 “Block diagram of PCA9545A/45B/45C”.
6.1 Device address
Following a START condition, the bus master must output the address of the slave it is
accessing. The address of the PCA9545A is shown in Figure 5. To conserve power, no
internal pull-up resistors are incorporated on the hardware selectable address pins and
they must be pulled HIGH or LOW.
The last bit of the slave address defines the operation to be performed. When set to
logic 1, a read is selected while a logic 0 selects a write operation.
The PCA9545BPW and PCA9545CPW are alternate address versions if needed for larger
systems or to resolve conflicts. The data sheet references the PCA9545A, but the
PCA9545B and PCA9545C function identically except for the slave address.
Fig 5. Slave address PCA9545A
Fig 6. Slave address PCA9545B Fig 7. Slave address PCA9545C
002aab169
1 1 1 0 0 A1 A0 R/W
fixed hardware
selectable
002aab835
1 1 0 1 0 A1 A0 R/W
fixed hardware
selectable
002aab836
1 0 1 1 0 A1 A0 R/W
fixed hardware
selectable
PCA9545A_45B_45C All information provided in this document is subject to legal disclaimers. © NXP Semiconductors N.V. 2014. All rights reserved.
Product data sheet Rev. 9 — 5 May 2014 7 of 32
NXP Semiconductors PCA9545A/45B/45C
4-channel I2C-bus switch with interrupt logic and reset
6.2 Control register
Following the successful acknowledgement of the slave address, the bus master sends a
byte to the PCA9545A/45B/45C, which is stored in the control register. If multiple bytes
are received by the PCA9545A/45B/45C, it saves the last byte received. This register can
be written and read via the I2C-bus.
6.2.1 Control register definition
One or several SCx/SDx downstream pair, or channel, is selected by the contents of the
control register. This register is written after the PCA9545A/45B/45C has been addressed.
The 4 LSBs of the control byte are used to determine which channel is to be selected.
When a channel is selected, the channel will become active after a STOP condition has
been placed on the I2C-bus. This ensures that all SCx/SDx lines are in a HIGH state when
the channel is made active, so that no false conditions are generated at the time of
connection.
Remark: Several channels can be enabled at the same time. Example: B3 = 0, B2 = 1,
B1 = 1, B0 = 0, means that channel 0 and channel 3 are disabled and channel 1 and
channel 2 are enabled. Care should be taken not to exceed the maximum bus capacity.
Fig 8. Control register
002aab170
INT
3
INT
2
INT
1
INT
0 B3 B2 B1 B0
channel selection bits
(read/write)
7 6 5 4 3 2 1 0
interrupt bits
(read only)
channel 0
channel 1
channel 2
channel 3
INT0
INT1
INT2
INT3
Table 4. Control register: write (channel selection); read (channel status)
INT3 INT2 INT1 INT0 B3 B2 B1 B0 Command
X X X X X X X
0 channel 0 disabled
1 channel 0 enabled
X X X X X X
0
X
channel 1 disabled
1 channel 1 enabled
X X X X X
0
X X
channel 2 disabled
1 channel 2 enabled
X X X X
0
X X X
channel 3 disabled
1 channel 3 enabled
0 0 0 0 0 0 0 0 no channel selected;
power-up/reset default state
PCA9545A_45B_45C All information provided in this document is subject to legal disclaimers. © NXP Semiconductors N.V. 2014. All rights reserved.
Product data sheet Rev. 9 — 5 May 2014 8 of 32
NXP Semiconductors PCA9545A/45B/45C
4-channel I2C-bus switch with interrupt logic and reset
6.2.2 Interrupt handling
The PCA9545A/45B/45C provides 4 interrupt inputs, one for each channel, and one
open-drain interrupt output. When an interrupt is generated by any device, it is detected
by the PCA9545A/45B/45C and the interrupt output is driven LOW. The channel does not
need to be active for detection of the interrupt. A bit is also set in the control register.
Bit 4 through bit 7 of the control register corresponds to channel 0 through channel 3 of
the PCA9545A/45B/45C, respectively. Therefore, if an interrupt is generated by any
device connected to channel 1, the state of the interrupt inputs is loaded into the control
register when a read is accomplished. Likewise, an interrupt on any device connected to
channel 0 would cause bit 4 of the control register to be set on the read. The master can
then address the PCA9545A/45B/45C and read the contents of the control register to
determine which channel contains the device generating the interrupt. The master can
then reconfigure the PCA9545A/45B/45C to select this channel, and locate the device
generating the interrupt and clear it.
It should be noted that more than one device can provide an interrupt on a channel, so it is
up to the master to ensure that all devices on a channel are interrogated for an interrupt.
If the interrupt function is not required, the interrupt inputs may be used as
general-purpose inputs.
If unused, interrupt inputs must be connected to VDD through a pull-up resistor.
Remark: Several interrupts can be active at the same time. Example: INT3 = 0, INT2 = 1,
INT1 = 1, INT0 = 0, means that there is no interrupt on channel 0 and channel 3, and
there is interrupt on channel 1 and channel 2.
6.3 RESET input
The RESET input is an active LOW signal which may be used to recover from a bus fault
condition. By asserting this signal LOW for a minimum of tw(rst)L, the PCA9545A/45B/45C
resets its registers and I2C-bus state machine and deselects all channels. The RESET
input must be connected to VDD through a pull-up resistor.
Table 5. Control register: Read — interrupt
INT3 INT2 INT1 INT0 B3 B2 B1 B0 Command
X X X
0
X X X X
no interrupt on channel 0
1 interrupt on channel 0
X X
0
X X X X X
no interrupt on channel 1
1 interrupt on channel 1
X
0
X X X X X X
no interrupt on channel 2
1 interrupt on channel 2
0
X X X X X X X
no interrupt on channel 3
1 interrupt on channel 3
PCA9545A_45B_45C All information provided in this document is subject to legal disclaimers. © NXP Semiconductors N.V. 2014. All rights reserved.
Product data sheet Rev. 9 — 5 May 2014 9 of 32
NXP Semiconductors PCA9545A/45B/45C
4-channel I2C-bus switch with interrupt logic and reset
6.4 Power-on reset
When power is applied to VDD, an internal Power-On Reset (POR) holds the
PCA9545A/45B/45C in a reset condition until VDD has reached VPOR. At this point, the
reset condition is released and the PCA9545A/45B/45C registers and I2C-bus state
machine are initialized to their default states (all zeroes) causing all the channels to be
deselected. Thereafter, VDD must be lowered below 0.2 V for at least 5 s in order to reset
the device.
6.5 Voltage translation
The pass gate transistors of the PCA9545A/45B/45C are constructed such that the VDD
voltage can be used to limit the maximum voltage that is passed from one I2C-bus to
another.
Figure 9 shows the voltage characteristics of the pass gate transistors (note that the graph
was generated using the data specified in Section 11 “Static characteristics” of this data
sheet). In order for the PCA9545A/45B/45C to act as a voltage translator, the Vo(sw)
voltage should be equal to, or lower than the lowest bus voltage. For example, if the main
bus was running at 5 V, and the downstream buses were 3.3 V and 2.7 V, then Vo(sw)
should be equal to or below 2.7 V to clamp the downstream bus voltages effectively.
Looking at Figure 9, we see that Vo(sw)(max) is at 2.7 V when the PCA9545A/45B/45C
supply voltage is 3.5 V or lower, so the PCA9545A/45B/45C supply voltage could be set to
3.3 V. Pull-up resistors can then be used to bring the bus voltages to their appropriate
levels (see Figure 16).
More Information can be found in Application Note AN262: PCA954X family of I2C/SMBus
multiplexers and switches.
(1) maximum
(2) typical
(3) minimum
Fig 9. Pass gate voltage versus supply voltage
VDD (V)
2.0 3.0 4.0 4.5 5.5
002aaa964
3.0
2.0
4.0
5.0
Vo(sw)
(V)
1.0
2.5 3.5 5.0
(1)
(2)
(3)
PCA9545A_45B_45C All information provided in this document is subject to legal disclaimers. © NXP Semiconductors N.V. 2014. All rights reserved.
Product data sheet Rev. 9 — 5 May 2014 10 of 32
NXP Semiconductors PCA9545A/45B/45C
4-channel I2C-bus switch with interrupt logic and reset
7. Characteristics of the I2C-bus
The I2C-bus is for 2-way, 2-line communication between different ICs or modules. The two
lines are a serial data line (SDA) and a serial clock line (SCL). Both lines must be
connected to a positive supply via a pull-up resistor when connected to the output stages
of a device. Data transfer may be initiated only when the bus is not busy.
7.1 Bit transfer
One data bit is transferred during each clock pulse. The data on the SDA line must remain
stable during the HIGH period of the clock pulse, as changes in the data line at this time
are interpreted as control signals (see Figure 10).
7.2 START and STOP conditions
Both data and clock lines remain HIGH when the bus is not busy. A HIGH-to-LOW
transition of the data line, while the clock is HIGH is defined as the START condition (S).
A LOW-to-HIGH transition of the data line while the clock is HIGH is defined as the
STOP condition (P) (see Figure 11).
Fig 10. Bit transfer
Fig 11. Definition of START and STOP conditions
PCA9545A_45B_45C All information provided in this document is subject to legal disclaimers. © NXP Semiconductors N.V. 2014. All rights reserved.
Product data sheet Rev. 9 — 5 May 2014 11 of 32
NXP Semiconductors PCA9545A/45B/45C
4-channel I2C-bus switch with interrupt logic and reset
7.3 System configuration
A device generating a message is a ‘transmitter’, a device receiving is the ‘receiver’. The
device that controls the message is the ‘master’ and the devices which are controlled by
the master are the ‘slaves’ (see Figure 12).
7.4 Acknowledge
The number of data bytes transferred between the START and the STOP conditions from
transmitter to receiver is not limited. Each byte of 8 bits is followed by one acknowledge
bit. The acknowledge bit is a HIGH level put on the bus by the transmitter, whereas the
master generates an extra acknowledge related clock pulse.
A slave receiver which is addressed must generate an acknowledge after the reception of
each byte. Also a master must generate an acknowledge after the reception of each byte
that has been clocked out of the slave transmitter. The device that acknowledges has to
pull down the SDA line during the acknowledge clock pulse, so that the SDA line is stable
LOW during the HIGH period of the acknowledge related clock pulse; set-up and hold
times must be taken into account.
A master receiver must signal an end of data to the transmitter by not generating an
acknowledge on the last byte that has been clocked out of the slave. In this event, the
transmitter must leave the data line HIGH to enable the master to generate a STOP
condition.
Fig 12. System configuration
Fig 13. Acknowledgement on the I2C-bus
PCA9545A_45B_45C All information provided in this document is subject to legal disclaimers. © NXP Semiconductors N.V. 2014. All rights reserved.
Product data sheet Rev. 9 — 5 May 2014 12 of 32
NXP Semiconductors PCA9545A/45B/45C
4-channel I2C-bus switch with interrupt logic and reset
7.5 Bus transactions
Data is transmitted to the PCA9545A/45B/45C control register using the Write mode as
shown in Figure 14.
Data is read from PCA9545A/45B/45C using the Read mode as shown in Figure 15.
Fig 14. Write control register
Fig 15. Read control register
002aab172
S 1 1 1 0 0 A1 A0 0 A X X X X B3 B2 B1 B0 A P
slave address
START condition R/W acknowledge
from slave
acknowledge
from slave
control register
SDA
STOP condition
002aab173
S 1 1 1 0 0 A1 A0 1 A INT3 INT2 INT1 INT0 B3 B2 B1 B0 NA P
slave address
START condition R/W acknowledge
from slave
no acknowledge
from master
control register
SDA
STOP condition
last byte
PCA9545A_45B_45C All information provided in this document is subject to legal disclaimers. © NXP Semiconductors N.V. 2014. All rights reserved.
Product data sheet Rev. 9 — 5 May 2014 13 of 32
NXP Semiconductors PCA9545A/45B/45C
4-channel I2C-bus switch with interrupt logic and reset
8. Application design-in information
(1) If the device generating the interrupt has an open-drain output structure or can be 3-stated, a
pull-up resistor is required.
If the device generating the interrupt has a totem-pole output structure and cannot be 3-stated, a
pull-up resistor is not required.
The interrupt inputs should not be left floating.
Fig 16. Typical application
PCA9545A
SD0
SC0
A1
A0
VSS
SDA
SCL
RESET
VDD = 2.7 V to 5.5 V VDD = 3.3 V
I2C-bus/SMBus master
002aab171
SDA
SCL
channel 0
V = 2.7 V to 5.5 V
INT INT0
see note (1)
SD1
SC1
channel 1
V = 2.7 V to 5.5 V
INT1
see note (1)
SD2
SC2
channel 2
V = 2.7 V to 5.5 V
INT2
see note (1)
SD3
SC3
channel 3
V = 2.7 V to 5.5 V
INT3
see note (1)
PCA9545A_45B_45C All information provided in this document is subject to legal disclaimers. © NXP Semiconductors N.V. 2014. All rights reserved.
Product data sheet Rev. 9 — 5 May 2014 14 of 32
NXP Semiconductors PCA9545A/45B/45C
4-channel I2C-bus switch with interrupt logic and reset
9. Limiting values
[1] The performance capability of a high-performance integrated circuit in conjunction with its thermal
environment can create junction temperatures which are detrimental to reliability. The maximum junction
temperature of this integrated circuit should not exceed 125 C.
10. Thermal characteristics
Table 6. Limiting values
In accordance with the Absolute Maximum Rating System (IEC 60134). Voltages are referenced to
VSS (ground = 0 V).
Symbol Parameter Conditions Min Max Unit
VDD supply voltage 0.5 +7.0 V
VI input voltage 0.5 +7.0 V
II input current - 20 mA
IO output current - 25 mA
IDD supply current - 100 mA
ISS ground supply current - 100 mA
Ptot total power dissipation - 400 mW
Tj(max) maximum junction temperature [1] - 125 C
Tstg storage temperature 60 +150 C
Tamb ambient temperature operating 40 +85 C
Table 7. Thermal characteristics
Symbol Parameter Conditions Typ Unit
Rth(j-a) thermal resistance from junction
to ambient
HVQFN20 package 32 C/W
SO20 package 90 C/W
TSSOP20 package 146 C/W
PCA9545A_45B_45C All information provided in this document is subject to legal disclaimers. © NXP Semiconductors N.V. 2014. All rights reserved.
Product data sheet Rev. 9 — 5 May 2014 15 of 32
NXP Semiconductors PCA9545A/45B/45C
4-channel I2C-bus switch with interrupt logic and reset
11. Static characteristics
[1] For operation between published voltage ranges, refer to the worst-case parameter in both ranges.
[2] VDD must be lowered to 0.2 V for at least 5 s in order to reset part.
Table 8. Static characteristics at VDD = 2.3 V to 3.6 V
VSS = 0 V; Tamb = 40 C to +85 C; unless otherwise specified. See Table 9 on page 16 for VDD = 4.5 V to 5.5 V[1].
Symbol Parameter Conditions Min Typ Max Unit
Supply
VDD supply voltage 2.3 - 3.6 V
IDD supply current Operating mode; VDD = 3.6 V; no load;
VI = VDD or VSS; fSCL = 100 kHz
- 10 30 A
Istb standby current Standby mode; VDD = 3.6 V; no load;
VI = VDD or VSS
- 0.1 1 A
VPOR power-on reset voltage no load; VI = VDD or VSS [2]- 1.6 2.1 V
Input SCL; input/output SDA
VIL LOW-level input voltage 0.5 - +0.3VDD V
VIH HIGH-level input voltage 0.7VDD - 6 V
IOL LOW-level output current VOL = 0.4 V 3 7 - mA
VOL = 0.6 V 6 10 - mA
IL leakage current VI = VDD or VSS 1 - +1 A
Ci input capacitance VI = VSS - 10 13 pF
Select inputs A0, A1, INT0 to INT3, RESET
VIL LOW-level input voltage 0.5 - +0.3VDD V
VIH HIGH-level input voltage 0.7VDD - 6 V
ILI input leakage current pin at VDD or VSS 1 - +1 A
Ci input capacitance VI = VSS - 1.6 3 pF
Pass gate
Ron ON-state resistance VDD = 3.6 V; VO = 0.4 V; IO = 15 mA 5 11 30
VDD = 2.3 V to 2.7 V; VO = 0.4 V;
IO = 10 mA
7 16 55
Vo(sw) switch output voltage Vi(sw) = VDD = 3.3 V; Io(sw) = 100 A - 1.9 - V
Vi(sw) = VDD = 3.0 V to 3.6 V;
Io(sw) = 100 A
1.6 - 2.8 V
Vi(sw) = VDD = 2.5 V; Io(sw) = 100 A - 1.5 - V
Vi(sw) = VDD = 2.3 V to 2.7 V;
Io(sw) = 100 A
1.1 - 2.0 V
IL leakage current VI = VDD or VSS 1 - +1 A
Cio input/output capacitance VI = VSS - 3 5 pF
INT output
IOL LOW-level output current VOL = 0.4 V 3 - - mA
IOH HIGH-level output current - - +10 A
PCA9545A_45B_45C All information provided in this document is subject to legal disclaimers. © NXP Semiconductors N.V. 2014. All rights reserved.
Product data sheet Rev. 9 — 5 May 2014 16 of 32
NXP Semiconductors PCA9545A/45B/45C
4-channel I2C-bus switch with interrupt logic and reset
[1] For operation between published voltage ranges, refer to the worst-case parameter in both ranges.
[2] VDD must be lowered to 0.2 V for at least 5 s in order to reset part.
Table 9. Static characteristics at VDD = 4.5 V to 5.5 V
VSS = 0 V; Tamb = 40 C to +85 C; unless otherwise specified. See Table 8 on page 15 for VDD = 2.3 V to 3.6 V[1].
Symbol Parameter Conditions Min Typ Max Unit
Supply
VDD supply voltage 4.5 - 5.5 V
IDD supply current Operating mode; VDD = 5.5 V;
no load; VI = VDD or VSS;
fSCL = 100 kHz
- 25 100 A
Istb standby current Standby mode; VDD = 5.5 V;
no load; VI = VDD or VSS
- 0.3 1 A
VPOR power-on reset voltage no load; VI = VDD or VSS [2]- 1.7 2.1 V
Input SCL; input/output SDA
VIL LOW-level input voltage 0.5 - +0.3VDD V
VIH HIGH-level input voltage 0.7VDD - 6 V
IOL LOW-level output current VOL = 0.4 V 3 - - mA
VOL = 0.6 V 6 - - mA
IL leakage current VI = VSS 1 - +1 A
Ci input capacitance VI = VSS - 10 13 pF
Select inputs A0, A1, INT0 to INT3, RESET
VIL LOW-level input voltage 0.5 - +0.3VDD V
VIH HIGH-level input voltage 0.7VDD - 6 V
ILI input leakage current VI = VDD or VSS 1 - +1 A
Ci input capacitance VI = VSS - 2 5 pF
Pass gate
Ron ON-state resistance VDD = 4.5 V to 5.5 V; VO = 0.4 V;
IO = 15 mA
4 9 24
Vo(sw) switch output voltage Vi(sw) = VDD = 5.0 V;
Io(sw) = 100 A
- 3.6 - V
Vi(sw) = VDD = 4.5 V to 5.5 V;
Io(sw) = 100 A
2.6 - 4.5 V
IL leakage current VI = VDD or VSS 1 - +1 A
Cio input/output capacitance VI = VSS - 3 5 pF
INT output
IOL LOW-level output current VOL = 0.4 V 3 - - mA
IOH HIGH-level output current - - +10 A
PCA9545A_45B_45C All information provided in this document is subject to legal disclaimers. © NXP Semiconductors N.V. 2014. All rights reserved.
Product data sheet Rev. 9 — 5 May 2014 17 of 32
NXP Semiconductors PCA9545A/45B/45C
4-channel I2C-bus switch with interrupt logic and reset
12. Dynamic characteristics
[1] Pass gate propagation delay is calculated from the 20 typical Ron and the 15 pF load capacitance.
[2] After this period, the first clock pulse is generated.
[3] A device must internally provide a hold time of at least 300 ns for the SDA signal (referred to the VIH(min) of the SCL signal) in order to
bridge the undefined region of the falling edge of SCL.
[4] Cb = total capacitance of one bus line in pF.
[5] Measurements taken with 1 k pull-up resistor and 50 pF load.
Table 10. Dynamic characteristics
Symbol Parameter Conditions Standard-mode
I2C-bus
Fast-mode I2C-bus Unit
Min Max Min Max
tPD propagation delay from SDA to SDx,
or SCL to SCx
- 0.3[1] - 0.3[1] ns
fSCL SCL clock frequency 0 100 0 400 kHz
tBUF bus free time between a STOP and
START condition
4.7 - 1.3 - s
tHD;STA hold time (repeated) START condition [2] 4.0 - 0.6 - s
tLOW LOW period of the SCL clock 4.7 - 1.3 - s
tHIGH HIGH period of the SCL clock 4.0 - 0.6 - s
tSU;STA set-up time for a repeated START
condition
4.7 - 0.6 - s
tSU;STO set-up time for STOP condition 4.0 - 0.6 - s
tHD;DAT data hold time 0[3] 3.45 0[3] 0.9 s
tSU;DAT data set-up time 250 - 100 - ns
tr rise time of both SDA and SCL
signals
- 1000 20 + 0.1Cb[4] 300 ns
tf fall time of both SDA and SCL signals - 300 20 + 0.1Cb[4] 300 ns
Cb capacitive load for each bus line - 400 - 400 pF
tSP pulse width of spikes that must be
suppressed by the input filter
- 50 - 50 ns
tVD;DAT data valid time HIGH-to-LOW [5] - 1 - 1 s
LOW-to-HIGH [5] - 0.6 - 0.6 s
tVD;ACK data valid acknowledge time - 1 - 1 s
INT
tv(INTnN-INTN) valid time from INTn to INT signal - 4 - 4 s
td(INTnN-INTN) delay time from INTn to INT inactive - 2 - 2 s
tw(rej)L LOW-level rejection time INTn inputs 1 - 1 - s
tw(rej)H HIGH-level rejection time INTn inputs 0.5 - 0.5 - s
RESET
tw(rst)L LOW-level reset time 4 - 4 - ns
trst reset time SDA clear 500 - 500 - ns
tREC;STA recovery time to START condition 0 - 0 - ns
PCA9545A_45B_45C All information provided in this document is subject to legal disclaimers. © NXP Semiconductors N.V. 2014. All rights reserved.
Product data sheet Rev. 9 — 5 May 2014 18 of 32
NXP Semiconductors PCA9545A/45B/45C
4-channel I2C-bus switch with interrupt logic and reset
Fig 17. Definition of timing on the I2C-bus
Fig 18. Definition of RESET timing
SDA
SCL
002aac549
50 %
30 %
50 % 50 %
tREC;STA tw(rst)L
RESET
START
trst
ACK or read cycle
Rise and fall times refer to VIL and VIH.
Fig 19. I2C-bus timing diagram
PCA9545A_45B_45C All information provided in this document is subject to legal disclaimers. © NXP Semiconductors N.V. 2014. All rights reserved.
Product data sheet Rev. 9 — 5 May 2014 19 of 32
NXP Semiconductors PCA9545A/45B/45C
4-channel I2C-bus switch with interrupt logic and reset
13. Test information
Fig 20. Expanded view of read input port register
SCL
002aab176
2 1 0 A P
70 %
30 %
SDA
INPUT 50 %
INT
tv(INTnN−INTN) td(INTnN−INTN)
Definitions test circuit:
RL = Load resistance.
CL = Load capacitance including jig and probe capacitance.
RT = Termination resistance should be equal to the output impedance Zo of the pulse generator.
Fig 21. Test circuitry for switching times
PULSE
GENERATOR
VO
CL
50 pF
RL
500 Ω
002aab177
RT
VI
VDD
VDD
D.U.T.
PCA9545A_45B_45C All information provided in this document is subject to legal disclaimers. © NXP Semiconductors N.V. 2014. All rights reserved.
Product data sheet Rev. 9 — 5 May 2014 20 of 32
NXP Semiconductors PCA9545A/45B/45C
4-channel I2C-bus switch with interrupt logic and reset
14. Package outline
Fig 22. Package outline SOT163-1 (SO20)
PCA9545A_45B_45C All information provided in this document is subject to legal disclaimers. © NXP Semiconductors N.V. 2014. All rights reserved.
Product data sheet Rev. 9 — 5 May 2014 21 of 32
NXP Semiconductors PCA9545A/45B/45C
4-channel I2C-bus switch with interrupt logic and reset
Fig 23. Package outline SOT360-1 (TSSOP20)
PCA9545A_45B_45C All information provided in this document is subject to legal disclaimers. © NXP Semiconductors N.V. 2014. All rights reserved.
Product data sheet Rev. 9 — 5 May 2014 22 of 32
NXP Semiconductors PCA9545A/45B/45C
4-channel I2C-bus switch with interrupt logic and reset
Fig 24. Package outline SOT662-1 (HVQFN20)
PCA9545A_45B_45C All information provided in this document is subject to legal disclaimers. © NXP Semiconductors N.V. 2014. All rights reserved.
Product data sheet Rev. 9 — 5 May 2014 23 of 32
NXP Semiconductors PCA9545A/45B/45C
4-channel I2C-bus switch with interrupt logic and reset
15. Soldering of SMD packages
This text provides a very brief insight into a complex technology. A more in-depth account
of soldering ICs can be found in Application Note AN10365 “Surface mount reflow
soldering description”.
15.1 Introduction to soldering
Soldering is one of the most common methods through which packages are attached to
Printed Circuit Boards (PCBs), to form electrical circuits. The soldered joint provides both
the mechanical and the electrical connection. There is no single soldering method that is
ideal for all IC packages. Wave soldering is often preferred when through-hole and
Surface Mount Devices (SMDs) are mixed on one printed wiring board; however, it is not
suitable for fine pitch SMDs. Reflow soldering is ideal for the small pitches and high
densities that come with increased miniaturization.
15.2 Wave and reflow soldering
Wave soldering is a joining technology in which the joints are made by solder coming from
a standing wave of liquid solder. The wave soldering process is suitable for the following:
• Through-hole components
• Leaded or leadless SMDs, which are glued to the surface of the printed circuit board
Not all SMDs can be wave soldered. Packages with solder balls, and some leadless
packages which have solder lands underneath the body, cannot be wave soldered. Also,
leaded SMDs with leads having a pitch smaller than ~0.6 mm cannot be wave soldered,
due to an increased probability of bridging.
The reflow soldering process involves applying solder paste to a board, followed by
component placement and exposure to a temperature profile. Leaded packages,
packages with solder balls, and leadless packages are all reflow solderable.
Key characteristics in both wave and reflow soldering are:
• Board specifications, including the board finish, solder masks and vias
• Package footprints, including solder thieves and orientation
• The moisture sensitivity level of the packages
• Package placement
• Inspection and repair
• Lead-free soldering versus SnPb soldering
15.3 Wave soldering
Key characteristics in wave soldering are:
• Process issues, such as application of adhesive and flux, clinching of leads, board
transport, the solder wave parameters, and the time during which components are
exposed to the wave
• Solder bath specifications, including temperature and impurities
PCA9545A_45B_45C All information provided in this document is subject to legal disclaimers. © NXP Semiconductors N.V. 2014. All rights reserved.
Product data sheet Rev. 9 — 5 May 2014 24 of 32
NXP Semiconductors PCA9545A/45B/45C
4-channel I2C-bus switch with interrupt logic and reset
15.4 Reflow soldering
Key characteristics in reflow soldering are:
• Lead-free versus SnPb soldering; note that a lead-free reflow process usually leads to
higher minimum peak temperatures (see Figure 25) than a SnPb process, thus
reducing the process window
• Solder paste printing issues including smearing, release, and adjusting the process
window for a mix of large and small components on one board
• Reflow temperature profile; this profile includes preheat, reflow (in which the board is
heated to the peak temperature) and cooling down. It is imperative that the peak
temperature is high enough for the solder to make reliable solder joints (a solder paste
characteristic). In addition, the peak temperature must be low enough that the
packages and/or boards are not damaged. The peak temperature of the package
depends on package thickness and volume and is classified in accordance with
Table 11 and 12
Moisture sensitivity precautions, as indicated on the packing, must be respected at all
times.
Studies have shown that small packages reach higher temperatures during reflow
soldering, see Figure 25.
Table 11. SnPb eutectic process (from J-STD-020D)
Package thickness (mm) Package reflow temperature (C)
Volume (mm3)
< 350 350
< 2.5 235 220
2.5 220 220
Table 12. Lead-free process (from J-STD-020D)
Package thickness (mm) Package reflow temperature (C)
Volume (mm3)
< 350 350 to 2000 > 2000
< 1.6 260 260 260
1.6 to 2.5 260 250 245
> 2.5 250 245 245
PCA9545A_45B_45C All information provided in this document is subject to legal disclaimers. © NXP Semiconductors N.V. 2014. All rights reserved.
Product data sheet Rev. 9 — 5 May 2014 25 of 32
NXP Semiconductors PCA9545A/45B/45C
4-channel I2C-bus switch with interrupt logic and reset
For further information on temperature profiles, refer to Application Note AN10365
“Surface mount reflow soldering description”.
MSL: Moisture Sensitivity Level
Fig 25. Temperature profiles for large and small components
001aac844
temperature
time
minimum peak temperature
= minimum soldering temperature
maximum peak temperature
= MSL limit, damage level
peak
temperature
PCA9545A_45B_45C All information provided in this document is subject to legal disclaimers. © NXP Semiconductors N.V. 2014. All rights reserved.
Product data sheet Rev. 9 — 5 May 2014 26 of 32
NXP Semiconductors PCA9545A/45B/45C
4-channel I2C-bus switch with interrupt logic and reset
16. Soldering: PCB footprints
Fig 26. PCB footprint for SOT163-1 (SO20); reflow soldering
Fig 27. PCB footprint for SOT163-1 (SO20); wave soldering
PCA9545A_45B_45C All information provided in this document is subject to legal disclaimers. © NXP Semiconductors N.V. 2014. All rights reserved.
Product data sheet Rev. 9 — 5 May 2014 27 of 32
NXP Semiconductors PCA9545A/45B/45C
4-channel I2C-bus switch with interrupt logic and reset
Fig 28. PCB footprint for SOT360-1 (TSSOP20); reflow soldering
PCA9545A_45B_45C All information provided in this document is subject to legal disclaimers. © NXP Semiconductors N.V. 2014. All rights reserved.
Product data sheet Rev. 9 — 5 May 2014 28 of 32
NXP Semiconductors PCA9545A/45B/45C
4-channel I2C-bus switch with interrupt logic and reset
Fig 29. PCB footprint for SOT662-1 (HVQFN20); reflow soldering
PCA9545A_45B_45C All information provided in this document is subject to legal disclaimers. © NXP Semiconductors N.V. 2014. All rights reserved.
Product data sheet Rev. 9 — 5 May 2014 29 of 32
NXP Semiconductors PCA9545A/45B/45C
4-channel I2C-bus switch with interrupt logic and reset
17. Abbreviations
18. Revision history
Table 13. Abbreviations
Acronym Description
CDM Charged-Device Model
DUT Device Under Test
ESD ElectroStatic Discharge
HBM Human Body Model
IC Integrated Circuit
I2C-bus Inter-Integrated Circuit bus
LSB Least Significant Bit
MSB Most Significant Bit
PCB Printed-Circuit Board
POR Power-On Reset
SMBus System Management Bus
Table 14. Revision history
Document ID Release date Data sheet status Change notice Supersedes
PCA9545A_45B_45C v.9 20140505 Product data sheet - PCA9545A_45B_45C v.8
Modifications: • Section 6.4 “Power-on reset”, first paragraph, third sentence corrected from “Thereafter,
VDD must be lowered below 0.2 V to reset the device.”
to “Thereafter, VDD must be lowered below 0.2 V for at least 5 s in order to reset the
device.” (this is a correction to documentation only; no change to device)
• Table 8 “Static characteristics at VDD = 2.3 V to 3.6 V”: Table note [2] corrected by inserting
phrase “for at least 5 s” (this is a correction to documentation only; no change to device)
• Table 9 “Static characteristics at VDD = 4.5 V to 5.5 V”: Table note [2] corrected by inserting
phrase “for at least 5 s” (this is a correction to documentation only; no change to device)
PCA9545A_45B_45C v.8 20130514 Product data sheet - PCA9545A_45B_45C v.7
PCA9545A_45B_45C v.7 20090619 Product data sheet - PCA9545A_45B_45C v.6
PCA9545A_45B_45C v.6 20070319 Product data sheet - PCA9545A_45B_45C v.5
PCA9545A_45B_45C v.5 20061017 Product data sheet - PCA9545A v.4
PCA9545A v.4 20060925 Product data sheet - PCA9545A v.3
PCA9545A v.3 20050303 Product data sheet - PCA9545A v.2
PCA9545A v.2 20040929 Objective data sheet - PCA9545A v.1
PCA9545A v.1 20040728 Objective data sheet - -
PCA9545A_45B_45C All information provided in this document is subject to legal disclaimers. © NXP Semiconductors N.V. 2014. All rights reserved.
Product data sheet Rev. 9 — 5 May 2014 30 of 32
NXP Semiconductors PCA9545A/45B/45C
4-channel I2C-bus switch with interrupt logic and reset
19. Legal information
19.1 Data sheet status
[1] Please consult the most recently issued document before initiating or completing a design.
[2] The term ‘short data sheet’ is explained in section “Definitions”.
[3] The product status of device(s) described in this document may have changed since this document was published and may differ in case of multiple devices. The latest product status
information is available on the Internet at URL http://www.nxp.com.
19.2 Definitions
Draft — The document is a draft version only. The content is still under
internal review and subject to formal approval, which may result in
modifications or additions. NXP Semiconductors does not give any
representations or warranties as to the accuracy or completeness of
information included herein and shall have no liability for the consequences of
use of such information.
Short data sheet — A short data sheet is an extract from a full data sheet
with the same product type number(s) and title. A short data sheet is intended
for quick reference only and should not be relied upon to contain detailed and
full information. For detailed and full information see the relevant full data
sheet, which is available on request via the local NXP Semiconductors sales
office. In case of any inconsistency or conflict with the short data sheet, the
full data sheet shall prevail.
Product specification — The information and data provided in a Product
data sheet shall define the specification of the product as agreed between
NXP Semiconductors and its customer, unless NXP Semiconductors and
customer have explicitly agreed otherwise in writing. In no event however,
shall an agreement be valid in which the NXP Semiconductors product is
deemed to offer functions and qualities beyond those described in the
Product data sheet.
19.3 Disclaimers
Limited warranty and liability — Information in this document is believed to
be accurate and reliable. However, NXP Semiconductors does not give any
representations or warranties, expressed or implied, as to the accuracy or
completeness of such information and shall have no liability for the
consequences of use of such information. NXP Semiconductors takes no
responsibility for the content in this document if provided by an information
source outside of NXP Semiconductors.
In no event shall NXP Semiconductors be liable for any indirect, incidental,
punitive, special or consequential damages (including - without limitation - lost
profits, lost savings, business interruption, costs related to the removal or
replacement of any products or rework charges) whether or not such
damages are based on tort (including negligence), warranty, breach of
contract or any other legal theory.
Notwithstanding any damages that customer might incur for any reason
whatsoever, NXP Semiconductors’ aggregate and cumulative liability towards
customer for the products described herein shall be limited in accordance
with the Terms and conditions of commercial sale of NXP Semiconductors.
Right to make changes — NXP Semiconductors reserves the right to make
changes to information published in this document, including without
limitation specifications and product descriptions, at any time and without
notice. This document supersedes and replaces all information supplied prior
to the publication hereof.
Suitability for use — NXP Semiconductors products are not designed,
authorized or warranted to be suitable for use in life support, life-critical or
safety-critical systems or equipment, nor in applications where failure or
malfunction of an NXP Semiconductors product can reasonably be expected
to result in personal injury, death or severe property or environmental
damage. NXP Semiconductors and its suppliers accept no liability for
inclusion and/or use of NXP Semiconductors products in such equipment or
applications and therefore such inclusion and/or use is at the customer’s own
risk.
Applications — Applications that are described herein for any of these
products are for illustrative purposes only. NXP Semiconductors makes no
representation or warranty that such applications will be suitable for the
specified use without further testing or modification.
Customers are responsible for the design and operation of their applications
and products using NXP Semiconductors products, and NXP Semiconductors
accepts no liability for any assistance with applications or customer product
design. It is customer’s sole responsibility to determine whether the NXP
Semiconductors product is suitable and fit for the customer’s applications and
products planned, as well as for the planned application and use of
customer’s third party customer(s). Customers should provide appropriate
design and operating safeguards to minimize the risks associated with their
applications and products.
NXP Semiconductors does not accept any liability related to any default,
damage, costs or problem which is based on any weakness or default in the
customer’s applications or products, or the application or use by customer’s
third party customer(s). Customer is responsible for doing all necessary
testing for the customer’s applications and products using NXP
Semiconductors products in order to avoid a default of the applications and
the products or of the application or use by customer’s third party
customer(s). NXP does not accept any liability in this respect.
Limiting values — Stress above one or more limiting values (as defined in
the Absolute Maximum Ratings System of IEC 60134) will cause permanent
damage to the device. Limiting values are stress ratings only and (proper)
operation of the device at these or any other conditions above those given in
the Recommended operating conditions section (if present) or the
Characteristics sections of this document is not warranted. Constant or
repeated exposure to limiting values will permanently and irreversibly affect
the quality and reliability of the device.
Terms and conditions of commercial sale — NXP Semiconductors
products are sold subject to the general terms and conditions of commercial
sale, as published at http://www.nxp.com/profile/terms, unless otherwise
agreed in a valid written individual agreement. In case an individual
agreement is concluded only the terms and conditions of the respective
agreement shall apply. NXP Semiconductors hereby expressly objects to
applying the customer’s general terms and conditions with regard to the
purchase of NXP Semiconductors products by customer.
No offer to sell or license — Nothing in this document may be interpreted or
construed as an offer to sell products that is open for acceptance or the grant,
conveyance or implication of any license under any copyrights, patents or
other industrial or intellectual property rights.
Document status[1][2] Product status[3] Definition
Objective [short] data sheet Development This document contains data from the objective specification for product development.
Preliminary [short] data sheet Qualification This document contains data from the preliminary specification.
Product [short] data sheet Production This document contains the product specification.
PCA9545A_45B_45C All information provided in this document is subject to legal disclaimers. © NXP Semiconductors N.V. 2014. All rights reserved.
Product data sheet Rev. 9 — 5 May 2014 31 of 32
NXP Semiconductors PCA9545A/45B/45C
4-channel I2C-bus switch with interrupt logic and reset
Export control — This document as well as the item(s) described herein
may be subject to export control regulations. Export might require a prior
authorization from competent authorities.
Non-automotive qualified products — Unless this data sheet expressly
states that this specific NXP Semiconductors product is automotive qualified,
the product is not suitable for automotive use. It is neither qualified nor tested
in accordance with automotive testing or application requirements. NXP
Semiconductors accepts no liability for inclusion and/or use of
non-automotive qualified products in automotive equipment or applications.
In the event that customer uses the product for design-in and use in
automotive applications to automotive specifications and standards, customer
(a) shall use the product without NXP Semiconductors’ warranty of the
product for such automotive applications, use and specifications, and (b)
whenever customer uses the product for automotive applications beyond
NXP Semiconductors’ specifications such use shall be solely at customer’s
own risk, and (c) customer fully indemnifies NXP Semiconductors for any
liability, damages or failed product claims resulting from customer design and
use of the product for automotive applications beyond NXP Semiconductors’
standard warranty and NXP Semiconductors’ product specifications.
Translations — A non-English (translated) version of a document is for
reference only. The English version shall prevail in case of any discrepancy
between the translated and English versions.
19.4 Trademarks
Notice: All referenced brands, product names, service names and trademarks
are the property of their respective owners.
I2C-bus — logo is a trademark of NXP Semiconductors N.V.
20. Contact information
For more information, please visit: http://www.nxp.com
For sales office addresses, please send an email to: salesaddresses@nxp.com
NXP Semiconductors PCA9545A/45B/45C
4-channel I2C-bus switch with interrupt logic and reset
© NXP Semiconductors N.V. 2014. All rights reserved.
For more information, please visit: http://www.nxp.com
For sales office addresses, please send an email to: salesaddresses@nxp.com
Date of release: 5 May 2014
Document identifier: PCA9545A_45B_45C
Please be aware that important notices concerning this document and the product(s)
described herein, have been included in section ‘Legal information’.
21. Contents
1 General description . . . . . . . . . . . . . . . . . . . . . . 1
2 Features and benefits . . . . . . . . . . . . . . . . . . . . 1
3 Ordering information. . . . . . . . . . . . . . . . . . . . . 2
3.1 Ordering options . . . . . . . . . . . . . . . . . . . . . . . . 2
4 Block diagram . . . . . . . . . . . . . . . . . . . . . . . . . . 3
5 Pinning information. . . . . . . . . . . . . . . . . . . . . . 4
5.1 Pinning . . . . . . . . . . . . . . . . . . . . . . . . . . . . . . . 4
5.2 Pin description . . . . . . . . . . . . . . . . . . . . . . . . . 5
6 Functional description . . . . . . . . . . . . . . . . . . . 6
6.1 Device address. . . . . . . . . . . . . . . . . . . . . . . . . 6
6.2 Control register . . . . . . . . . . . . . . . . . . . . . . . . . 7
6.2.1 Control register definition . . . . . . . . . . . . . . . . . 7
6.2.2 Interrupt handling . . . . . . . . . . . . . . . . . . . . . . . 8
6.3 RESET input . . . . . . . . . . . . . . . . . . . . . . . . . . . 8
6.4 Power-on reset . . . . . . . . . . . . . . . . . . . . . . . . . 9
6.5 Voltage translation . . . . . . . . . . . . . . . . . . . . . . 9
7 Characteristics of the I2C-bus . . . . . . . . . . . . 10
7.1 Bit transfer . . . . . . . . . . . . . . . . . . . . . . . . . . . 10
7.2 START and STOP conditions . . . . . . . . . . . . . 10
7.3 System configuration . . . . . . . . . . . . . . . . . . . 11
7.4 Acknowledge . . . . . . . . . . . . . . . . . . . . . . . . . 11
7.5 Bus transactions . . . . . . . . . . . . . . . . . . . . . . . 12
8 Application design-in information . . . . . . . . . 13
9 Limiting values. . . . . . . . . . . . . . . . . . . . . . . . . 14
10 Thermal characteristics . . . . . . . . . . . . . . . . . 14
11 Static characteristics. . . . . . . . . . . . . . . . . . . . 15
12 Dynamic characteristics . . . . . . . . . . . . . . . . . 17
13 Test information. . . . . . . . . . . . . . . . . . . . . . . . 19
14 Package outline . . . . . . . . . . . . . . . . . . . . . . . . 20
15 Soldering of SMD packages . . . . . . . . . . . . . . 23
15.1 Introduction to soldering . . . . . . . . . . . . . . . . . 23
15.2 Wave and reflow soldering . . . . . . . . . . . . . . . 23
15.3 Wave soldering . . . . . . . . . . . . . . . . . . . . . . . . 23
15.4 Reflow soldering . . . . . . . . . . . . . . . . . . . . . . . 24
16 Soldering: PCB footprints. . . . . . . . . . . . . . . . 26
17 Abbreviations. . . . . . . . . . . . . . . . . . . . . . . . . . 29
18 Revision history. . . . . . . . . . . . . . . . . . . . . . . . 29
19 Legal information. . . . . . . . . . . . . . . . . . . . . . . 30
19.1 Data sheet status . . . . . . . . . . . . . . . . . . . . . . 30
19.2 Definitions. . . . . . . . . . . . . . . . . . . . . . . . . . . . 30
19.3 Disclaimers . . . . . . . . . . . . . . . . . . . . . . . . . . . 30
19.4 Trademarks. . . . . . . . . . . . . . . . . . . . . . . . . . . 31
20 Contact information. . . . . . . . . . . . . . . . . . . . . 31
21 Contents . . . . . . . . . . . . . . . . . . . . . . . . . . . . . . 32
1. General description
The LPC3220/30/40/50 embedded microcontrollers were designed for low power, high
performance applications. NXP achieved their performance goals using a 90 nanometer
process to implement an ARM926EJ-S CPU core with a vector floating point co-processor
and a large set of standard peripherals including USB On-The-Go. The
LPC3220/30/40/50 operates at CPU frequencies of up to 266 MHz.
The NXP implementation uses a ARM926EJ-S CPU core with a Harvard architecture,
5-stage pipeline, and an integral Memory Management Unit (MMU). The MMU provides
the virtual memory capabilities needed to support the multi-programming demands of
modern operating systems. The ARM926EJ-S also has a hardware based set of DSP
instruction extensions, which includes single cycle MAC operations, and hardware based
native Jazelle Java Byte-code execution. The NXP implementation has a 32 kB
instruction cache and a 32 kB data cache.
For low power consumption, the LPC3220/30/40/50 takes advantage of NXP’s advanced
technology development to optimize intrinsic power and uses software controlled
architectural enhancements to optimize application based power management.
The LPC3220/30/40/50 also includes 256 kB of on-chip static RAM, a NAND flash
interface, an Ethernet MAC, an LCD controller that supports STN and TFT panels, and an
external bus interface that supports SDR and DDR SDRAM as well as static devices. In
addition, the LPC3220/30/40/50 includes a USB 2.0 full-speed interface, seven UARTs,
two I2C-bus interfaces, two SPI/SSP ports, two I2S-bus interfaces, two single output
PWMs, a motor control PWM, six general purpose timers with capture inputs and compare
outputs, a Secure Digital (SD) interface, and a 10-bit Analog-to-Digital Converter (ADC)
with a touch screen sense option.
2. Features and benefits
ARM926EJ-S processor, running at CPU clock speeds up to 266 MHz.
Vector Floating Point (VFP) coprocessor.
32 kB instruction cache and 32 kB data cache.
Up to 256 kB of Internal SRAM (IRAM).
Selectable boot-up from various external devices: NAND flash, SPI memory, USB,
UART, or static memory.
Multi-layer AHB system that provides a separate bus for each AHB master, including
both an instruction and data bus for the CPU, two data busses for the DMA controller,
and another bus for the USB controller, one for the LCD, and a final one for the
Ethernet MAC. There are no arbitration delays in the system unless two masters
attempt to access the same slave at the same time.
LPC3220/30/40/50
16/32-bit ARM microcontrollers; hardware floating-point
coprocessor, USB On-The-Go, and EMC memory interface
Rev. 2 — 20 October 2011 Product data sheet
LPC3220_30_40_50 All information provided in this document is subject to legal disclaimers. © NXP B.V. 2011. All rights reserved.
Product data sheet Rev. 2 — 20 October 2011 2 of 79
NXP Semiconductors LPC3220/30/40/50
16/32-bit ARM microcontrollers
External memory controller for DDR and SDR SDRAM as well as for static devices.
Two NAND flash controllers: One for single-level NAND flash devices and the other for
multi-level NAND flash devices.
Master Interrupt Controller (MIC) and two Slave Interrupt Controllers (SIC), supporting
74 interrupt sources.
Eight channel General Purpose DMA (GPDMA) controller on the AHB that can be
used with the SD card port, the high-speed UARTs, I2S-bus interfaces, and SPI
interfaces, as well as memory-to-memory transfers.
Serial interfaces:
10/100 Ethernet MAC with dedicated DMA Controller.
USB interface supporting either device, host (OHCI compliant), or On-The-Go
(OTG) with an integral DMA controller and dedicated PLL to generate the required
48 MHz USB clock.
Four standard UARTs with fractional baud rate generation and 64 byte FIFOs. One
of the standard UARTs supports IrDA.
Three additional high-speed UARTs intended for on-board communications that
support baud rates up to 921 600 when using a 13 MHz main oscillator. All
high-speed UARTs provide 64 byte FIFOs.
Two SPI controllers.
Two SSP controllers.
Two I2C-bus interfaces with standard open-drain pins. The I2C-bus interfaces
support single master, slave, and multi-master I2C-bus configurations.
Two I2S-bus interfaces, each with separate input and output channels. Each
channel can be operated independently on three pins, or both input and output
channels can be used with only four pins and a shared clock.
Additional peripherals:
LCD controller supporting both STN and TFT panels, with dedicated DMA
controller. Programmable display resolution up to 1024 768.
Secure Digital (SD) memory card interface, which conforms to the SD Memory
Card Specification Version 1.01.
General Purpose (GP) input, output, and I/O pins. Includes 12 GP input pins, 24
GP output pins, and 51 GP I/O pins.
10-bit, 400 kHz Analog-to-Digital Converter (ADC) with input multiplexing from
three pins. Optionally, the ADC can operate as a touch screen controller.
Real-Time Clock (RTC) with separate power pin and dedicated 32 kHz oscillator.
NXP implemented the RTC in an independent on-chip power domain so it can
remain active while the rest of the chip is not powered. The RTC also includes a
32-byte scratch pad memory.
32-bit general purpose high-speed timer with a 16-bit pre-scaler. This timer
includes one external capture input pin and a capture connection to the RTC clock.
Interrupts may be generated using three match registers.
Six enhanced timer/counters which are architecturally identical except for the
peripheral base address. Two capture inputs and two match outputs are pinned out
to four timers. Timer 1 brings out a third match output, timers 2 and 3 bring out all
four match outputs, timer 4 has one match output, and timer 5 has no inputs or
outputs.
32-bit millisecond timer driven from the RTC clock. This timer can generate
interrupts using two match registers.
LPC3220_30_40_50 All information provided in this document is subject to legal disclaimers. © NXP B.V. 2011. All rights reserved.
Product data sheet Rev. 2 — 20 October 2011 3 of 79
NXP Semiconductors LPC3220/30/40/50
16/32-bit ARM microcontrollers
WatchDog timer clocked by the peripheral clock.
Two single-output PWM blocks.
Motor control PWM.
Keyboard scanner function allows automatic scanning of an up to 8 8 key matrix.
Up to 18 external interrupts.
Standard ARM test/debug interface for compatibility with existing tools.
Emulation Trace Buffer (ETB) with 2048 24 bit RAM allows trace via JTAG.
Stop mode saves power while allowing many peripheral functions to restart CPU
activity.
On-chip crystal oscillator.
An on-chip PLL allows CPU operation up to the maximum CPU rate without the
requirement for a high frequency crystal. Another PLL allows operation from the
32 kHz RTC clock rather than the external crystal.
Boundary scan for simplified board testing.
User-accessible unique serial ID number for each chip.
TFBGA296 package with a 15 mm 15 mm 0.7 mm body.
3. Applications
Consumer
Medical
Industrial
Network control
LPC3220_30_40_50 All information provided in this document is subject to legal disclaimers. © NXP B.V. 2011. All rights reserved.
Product data sheet Rev. 2 — 20 October 2011 4 of 79
NXP Semiconductors LPC3220/30/40/50
16/32-bit ARM microcontrollers
4. Ordering information
[1] F = 40 C to +85 C temperature range. Note that Revision “A” parts with and without the /01 suffix are identical. For example,
LPC3220FET296 Revision “A” is identical to LPC3220FET296/01 Revision “A”.
[2] Available starting with Revision “A”.
4.1 Ordering options
Table 1. Ordering information
Type number[1] Package
Name Description Version
LPC3220FET296/01[2] TFBGA296 plastic thin fine-pitch ball grid array package; 296 balls SOT1048-1
LPC3230FET296/01[2] TFBGA296 plastic thin fine-pitch ball grid array package; 296 balls SOT1048-1
LPC3240FET296/01[2] TFBGA296 plastic thin fine-pitch ball grid array package; 296 balls SOT1048-1
LPC3250FET296/01[2] TFBGA296 plastic thin fine-pitch ball grid array package; 296 balls SOT1048-1
Table 2. Part options
Type number SRAM (kB) 10/100 Ethernet LCD
controller
Temperature range (C) Package
LPC3220FET296/01 128 0 0 40 to +85 TFBGA296
LPC3230FET296/01 256 0 1 40 to +85 TFBGA296
LPC3240FET296/01 256 1 0 40 to +85 TFBGA296
LPC3250FET296/01 256 1 1 40 to +85 TFBGA296
LPC3220_30_40_50 All information provided in this document is subject to legal disclaimers. © NXP B.V. 2011. All rights reserved.
Product data sheet Rev. 2 — 20 October 2011 5 of 79
NXP Semiconductors LPC3220/30/40/50
16/32-bit ARM microcontrollers
5. Block diagram
Fig 1. Block diagram of LPC3220/30/40/50
ARM
9EJS
D-CACHE
32 kB
I-CACHE
32 kB
DATA INSTRUCTION
ethernet
PHY
interface
USB
transceiver
interface
LCD
panel
interface
EXTERNAL
MEMORY
CONTROLLER
ROM
16 kB
SRAM
256 kB
DMA USB SDRAM ETB
STANDARD
UART × 4
I2C
× 2
TIMERS
× 6
WATCHDOG
TIMER
DEBUG
SYSTEM
CONTROL
HS UART
× 3
KEY
SCANNER
10-BIT
ADC/TS
UART
CONTROL
RTC
PWM
× 2
GPIO
M0 M1
AHB
TO
APB
BRIDGE
AHB
TO
APB
BRIDGE
AHB
TO
APB
BRIDGE
master layer 0 1 2 3 4 5 6
slave port 0
1
7
6
5
3
2
= Master/Slave connection supported
by the multilayer AHB matrix
32-bit AHB matrix
APB slaves
FAB slaves
AHB slaves APB slaves
port 3
port 4
port 0
32-bit wide
external
memory
VFP9 ETB ETM 9
ETHERNET LCD
MOTOR
CONTROL PWM
002aae397
MMU
D-SIDE
CONTROLLER
I-SIDE
CONTROLLER
DMA
CONTROLLER
ETHERNET
10/100
MAC
USB OTG
CONTROLLER
LCD
CONTROLLER
MLC
NAND
SLC
NAND
SD
CARD
SPI
× 2
I2S
× 2
SSP
× 2
INTERRUPT
CONTROL
register interfaces
LPC3220_30_40_50 All information provided in this document is subject to legal disclaimers. © NXP B.V. 2011. All rights reserved.
Product data sheet Rev. 2 — 20 October 2011 6 of 79
NXP Semiconductors LPC3220/30/40/50
16/32-bit ARM microcontrollers
6. Pinning information
6.1 Pinning
Fig 2. Pin configuration for SOT1048-1 (TFBGA296)
002aae398
Transparent top view
V
U
T
R
P
N
L
J
M
K
H
G
F
E
D
B
C
A
2 4 6 8 10 12
13
14
15 17
16 18
1 3 5 7 9 11
ball A1
index area
Table 3. Pin allocation table (TFBGA296)
Pin Symbol Pin Symbol Pin Symbol
Row A
A3 I2C2_SCL
A4 I2S1TX_CLK/MAT3[0] A5 I2C1_SCL A6 MS_BS/MAT2[1]
A7 MS_DIO1/MAT0[1] A8 MS_DIO0/MAT0[0] A9 SPI2_DATIO/MOSI1/LCDVD[20][1]
A10 SPI2_DATIN/MISO1/
LCDVD[21][1]/GPI_27
A11 GPIO_1 A12 GPIO_0
A13 GPO_21/U4_TX/LCDVD[3][1] A14 GPO_15/MCOA1/LCDFP[1] A15 GPO_7/LCDVD[2][1]
A16 GPO_6/LCDVD[18][1]
Row B
B2 GPO_20 B3 GPO_5
B4 I2S1TX_WS/CAP3[0] B5 P0[0]/I2S1RX_CLK B6 I2C1_SDA
B7 MS_SCLK/MAT2[0] B8 MS_DIO2/MAT0[2] B9 SPI1_DATIO/MOSI0/MCI2
B10 SPI2_CLK/SCK1/LCDVD[23][1] B11 GPIO_4/SSEL1/LCDVD[22][1] B12 GPO_12/MCOA2/LCDLE[1]
B13 GPO_13/MCOB1/LCDDCLK[1] B14 GPO_2/MAT1[0]/LCDVD[0][1] B15 GPI_19/U4_RX
B16 GPI_8/KEY_COL6/
SPI2_BUSY/ENET_RX_DV[2]
B17 n.c.
Row C
C1 FLASH_RD C2 GPO_19 C3 GPO_0/TST_CLK1
C4 USB_ATX_INT C5 USB_SE0_VM/U5_TX C6 TST_CLK2
C7 GPI_6/HSTIM_CAP/
ENET_RXD2[2]
C8 MS_DIO3/MAT0[3] C9 SPI1_CLK/SCK0
LPC3220_30_40_50 All information provided in this document is subject to legal disclaimers. © NXP B.V. 2011. All rights reserved.
Product data sheet Rev. 2 — 20 October 2011 7 of 79
NXP Semiconductors LPC3220/30/40/50
16/32-bit ARM microcontrollers
C10 SPI1_DATIN/MISO0/GPI_25/
MCI1
C11 GPIO_3/KEY_ROW7/
ENET_MDIO[2]
C12 GPO_9/LCDVD[9][1]
C13 GPO_8/LCDVD[8][1] C14 GPI_2/CAP2[0]/
ENET_RXD3[2]
C15 GPI_1/SERVICE
C16 GPI_0/I2S1RX_SDA C17 KEY_ROW4/ENET_TXD0[2] C18 KEY_ROW5/ENET_TXD1[2]
Row D
D1 FLASH_RDY D2 FLASH_ALE D3 GPO_14
D4 GPO_1 D5 USB_DAT_VP/U5_RX D6 USB_OE_TP
D7 P0[1]/I2S1RX_WS D8 GPO_4 D9 GPIO_2/KEY_ROW6/ENET_MDC[2]
D10 GPO_16/MCOB0/LCDENAB[1]/
LCDM[1]
D11 GPO_18/MCOA0/LCDLP[1] D12 GPO_3/LCDVD[1][1]
D13 GPI_7/CAP4[0]/MCABORT D14 PWM_OUT1/LCDVD[16][1] D15 PWM_OUT2/INTSTAT/LCDVD[19][1]
D16 KEY_ROW3/ENET_TX_EN[2] D17 KEY_COL2/ENET_RX_ER[2] D18 KEY_COL3/ENET_CRS[2]
Row E
E1 FLASH_IO[3] E2 FLASH_IO[7] E3 FLASH_CE
E4 I2C2_SDA E5 USB_I2C_SCL E6 USB_I2C_SDA
E7 I2S1TX_SDA/MAT3[1] E8 GPO_11 E9 GPIO_5/SSEL0/MCI0
E10 GPO_22/U7_HRTS/
LCDVD[14][1]
E11 GPO_10/MCOB2/LCDPWR[1] E12 GPI_9/KEY_COL7/ENET_COL[2]
E13 GPI_4/SPI1_BUSY E14 KEY_ROW1/ENET_TXD2[2] E15 KEY_ROW0/ENET_TX_ER[2]
E16 KEY_COL1/ENET_RX_CLK[2]/
ENET_REF_CLK[2]
E17 U7_RX/CAP0[0]/
LCDVD[10][1]/GPI_23
E18 U7_TX/MAT1[1]/LCDVD[11][1]
Row F
F1 FLASH_IO[2] F2 FLASH_WR F3 FLASH_CLE
F4 GPI_3 F5 VSS_IOC F6 VSS_IOB
F7 VDD_IOC F8 VDD_IOB F9 VDD_IOD
F10 VSS_IOD F11 VSS_IOD F12 VSS_IOD
F13 VDD_IOD F14 KEY_ROW2/ENET_TXD3[2] F15 KEY_COL0/ENET_TX_CLK[2]
F16 KEY_COL5/ENET_RXD1[2] F17 U6_IRRX/GPI_21 F18 U5_RX/GPI_20
Row G
G1 EMC_DYCS1 G2 FLASH_IO[5] G3 FLASH_IO[6]
G4 RESOUT G5 VSS_IOC G6 VDD_IOC
G7 VDD_CORE G8 VSS_CORE G9 VDD_CORE
G10 VSS_CORE G11 VDD_CORE G12 VSS_CORE
G13 U7_HCTS/CAP0[1]/
LCDCLKIN[1]/GPI_22
G14 DBGEN G15 KEY_COL4/ENET_RXD0[2]
G16 U6_IRTX G17 SYSCLKEN/LCDVD[15][1] G18 JTAG_TMS
Row H
H1 EMC_OE H2 FLASH_IO[0] H3 FLASH_IO[1]
H4 FLASH_IO[4] H5 VSS_IOC H6 VDD_IOC
H7 VSS_CORE
H12 VSS_IOD
H13 VDD_IOA H14 JTAG_TCK H15 U5_TX
Table 3. Pin allocation table (TFBGA296)
Pin Symbol Pin Symbol Pin Symbol
LPC3220_30_40_50 All information provided in this document is subject to legal disclaimers. © NXP B.V. 2011. All rights reserved.
Product data sheet Rev. 2 — 20 October 2011 8 of 79
NXP Semiconductors LPC3220/30/40/50
16/32-bit ARM microcontrollers
H16 HIGHCORE/LCDVD[17][1] H17 JTAG_NTRST H18 JTAG_RTCK
Row J
J1 EMC_A[20]/P1[20] J2 EMC_A[21]/P1[21] J3 EMC_A[22]/P1[22]
J4 EMC_A[23]/P1[23] J5 VDD_IOC J6 VDD_EMC
J7 VDD_CORE
J12 VDD_CORE
J13 VDD_IOA J14 U3_RX/GPI_18 J15 JTAG_TDO
J16 JTAG_TDI J17 U3_TX J18 U2_HCTS/U3_CTS/GPI_16
Row K
K1 EMC_A[19]/P1[19] K2 EMC_A[18]/P1[18] K3 EMC_A[16]/P1[16]
K4 EMC_A[17]/P1[17] K5 VSS_EMC K6 VDD_EMC
K7 VDD_EMC
K12 VSS_CORE
K13 VSS_IOA K14 VDD_RTC K15 U1_RX/CAP1[0]/GPI_15
K16 U1_TX K17 U2_TX/U3_DTR K18 U2_RX/U3_DSR/GPI_17
Row L
L1 EMC_A[15]/P1[15] L2 EMC_CKE1 L3 EMC_A[0]/P1[0]
L4 EMC_A[1]/P1[1] L5 VSS_EMC L6 VDD_EMC
L7 VSS_CORE
L12 VDD_COREFXD
L13 VDD_RTCCORE L14 VSS_RTCCORE L15 P0[4]/I2S0RX_WS/LCDVD[6][1]
L16 P0[5]/I2S0TX_SDA/LCDVD[7][1] L17 P0[6]/I2S0TX_CLK/
LCDVD[12][1]
L18 P0[7]/I2S0TX_WS/LCDVD[13][1]
Row M
M1 EMC_A[2]/P1[2] M2 EMC_A[3]/P1[3] M3 EMC_A[4]/P1[4]
M4 EMC_A[8]/P1[8] M5 VSS_EMC M6 VDD_EMC
M7 VDD_CORE M8 VDD_EMC M9 VSS_CORE
M10 VSS_CORE M11 VDD_CORE M12 VSS_CORE
M13 VDD_COREFXD M14 RESET M15 ONSW
M16 GPO_23/U2_HRTS/U3_RTS M17 P0[2]/I2S0RX_SDA/
LCDVD[4][1]
M18 P0[3]/I2S0RX_CLK/LCDVD[5][1]
Row N
N1 EMC_A[5]/P1[5] N2 EMC_A[6]/P1[6] N3 EMC_A[7/P1[7]
N4 EMC_A[12]/P1[12] N5 VSS_EMC N6 VSS_EMC
N7 VDD_EMC N8 VDD_EMC N9 VDD_EMC
N10 VDD_EMC N11 VDD_EMC N12 VDD_AD
N13 VDD_AD N14 VDD_FUSE N15 VDD_RTCOSC
N16 GPI_5/U3_DCD N17 GPI_28/U3_RI N18 GPO_17
Row P
P1 EMC_A[9]/P1[9] P2 EMC_A[10]/P1[10] P3 EMC_A[11]/P1[11]
P4 EMC_DQM[1] P5 EMC_DQM[3] P6 VSS_EMC
Table 3. Pin allocation table (TFBGA296)
Pin Symbol Pin Symbol Pin Symbol
LPC3220_30_40_50 All information provided in this document is subject to legal disclaimers. © NXP B.V. 2011. All rights reserved.
Product data sheet Rev. 2 — 20 October 2011 9 of 79
NXP Semiconductors LPC3220/30/40/50
16/32-bit ARM microcontrollers
[1] LCD on LPC3230 and LPC3250 only.
[2] Ethernet on LPC3240 and LPC3250 only.
P7 VSS_EMC P8 VSS_EMC P9 VSS_EMC
P10 VSS_EMC P11 VSS_EMC P12 EMC_BLS[3]
P13 VSS_AD P14 VSS_OSC P15 VDD_PLLUSB
P16 RTCX_IN P17 RTCX_OUT P18 VSS_RTCOSC
Row R
R1 EMC_A[13]/P1[13] R2 EMC_A[14]/P1[14] R3 EMC_DQM[0]
R4 EMC_WR R5 EMC_CAS R6 EMC_DYCS0
R7 EMC_D[1] R8 EMC_D[7] R9 EMC_D[17]/EMC_DQS1
R10 EMC_D[24]/P2[5] R11 EMC_CS1 R12 EMC_BLS[2]
R13 TS_XP R14 PLL397_LOOP R15 SYSX_OUT
R16 VSS_PLLUSB R17 VDD_PLLHCLK R18 VSS_PLLHCLK
Row T
T1 EMC_DQM[2] T2 EMC_RAS T3 EMC_CLK
T4 EMC_CLKIN T5 EMC_D[2] T6 EMC_D[6]
T7 EMC_D[11] T8 EMC_D[14] T9 EMC_D[20]/P2[1]
T10 EMC_D[23]/P2[4] T11 EMC_D[27]/P2[8] T12 EMC_CS2
T13 EMC_BLS[1] T14 ADIN1/TS_XM T15 VSS_PLL397
T16 VDD_PLL397 T17 SYSX_IN T18 VDD_OSC
Row U
U2 n.c. U3 EMC_CKE0
U4 EMC_D[0] U5 EMC_D[3] U6 EMC_D[9]
U7 EMC_D[12] U8 EMC_D[15] U9 EMC_D[19]/P2[0]
U10 EMC_D[22]/P2[3] U11 EMC_D[26]/P2[7] U12 EMC_D[30]/P2[11]
U13 EMC_CS0 U14 EMC_BLS[0] U15 ADIN0/TS_YM
U16 TS_YP U17 n.c.
Row V
V3 EMC_D[4]
V4 EMC_D[5] V5 EMC_D[8] V6 EMC_D[10]
V7 EMC_D[13] V8 EMC_D[16]/EMC_DQS0 V9 EMC_D[18]/EMC_CLK
V10 EMC_D[21]/P2[2] V11 EMC_D[25]/P2[6] V12 EMC_D[28]/P2[9]
V13 EMC_D[29]/P2[10] V14 EMC_D[31]/P2[12] V15 EMC_CS3
V16 ADIN2/TS_AUX_IN
Table 3. Pin allocation table (TFBGA296)
Pin Symbol Pin Symbol Pin Symbol
LPC3220_30_40_50 All information provided in this document is subject to legal disclaimers. © NXP B.V. 2011. All rights reserved.
Product data sheet Rev. 2 — 20 October 2011 10 of 79
NXP Semiconductors LPC3220/30/40/50
16/32-bit ARM microcontrollers
6.2 Pin description
Table 4. Pin description
Symbol Pin Power supply
domain
Type Description
ADIN0/TS_YM U15 VDD_AD analog in ADC input 0/touch screen Y minus
ADIN1/TS_XM T14 VDD_AD analog in ADC input 0/touch screen X minus
ADIN2/TS_AUX_IN V16 VDD_AD analog in ADC input 2/touch screen AUX input
DBGEN G14 VDD_IOD I: PD Device test input
LOW = JTAG in-circuit debug available; normal
operation.
HIGH = I/O cell boundary scan test; for board
assembly BSDL test.
EMC_A[0]/P1[0] L3 VDD_EMC I/O EMC address bit 0
I/O Port 1 GPIO bit 0
EMC_A[1]/P1[1] L4 VDD_EMC I/O EMC address bit 1
I/O Port 1 GPIO bit 1
EMC_A[2]/P1[2] M1 VDD_EMC I/O EMC address bit 2
I/O Port 1 GPIO bit 2
EMC_A[3]/P1[3] M2 VDD_EMC I/O EMC address bit 3
I/O Port 1 GPIO bit 3
EMC_A[4]/P1[4] M3 VDD_EMC I/O EMC address bit 4
I/O Port 1 GPIO bit 4
EMC_A[5]/P1[5] N1 VDD_EMC I/O EMC address bit 5
I/O Port 1 GPIO bit 5
EMC_A[6]/P1[6] N2 VDD_EMC I/O EMC address bit 6
I/O Port 1 GPIO bit 6
EMC_A[7/P1[7] N3 VDD_EMC I/O EMC address bit 7
I/O Port 1 GPIO bit 7
EMC_A[8]/P1[8] M4 VDD_EMC I/O EMC address bit 8
I/O Port 1 GPIO bit 8
EMC_A[9]/P1[9] P1 VDD_EMC I/O EMC address bit 9
I/O Port 1 GPIO bit 9
EMC_A[10]/P1[10] P2 VDD_EMC I/O EMC address bit 10
I/O Port 1 GPIO bit 10
EMC_A[11]/P1[11] P3 VDD_EMC I/O EMC address bit 11
I/O Port 1 GPIO bit 11
EMC_A[12]/P1[12] N4 VDD_EMC I/O EMC address bit 12
I/O Port 1 GPIO bit 12
EMC_A[13]/P1[13] R1 VDD_EMC I/O EMC address bit 13
I/O Port 1 GPIO bit 13
EMC_A[14]/P1[14] R2 VDD_EMC I/O EMC address bit 14
I/O Port 1 GPIO bit 14
LPC3220_30_40_50 All information provided in this document is subject to legal disclaimers. © NXP B.V. 2011. All rights reserved.
Product data sheet Rev. 2 — 20 October 2011 11 of 79
NXP Semiconductors LPC3220/30/40/50
16/32-bit ARM microcontrollers
EMC_A[15]/P1[15] L1 VDD_EMC I/O EMC address bit 15
I/O Port 1 GPIO bit 15
EMC_A[16]/P1[16] K3 VDD_EMC I/O EMC address bit 16
I/O Port 1 GPIO bit 16
EMC_A[17]/P1[17] K4 VDD_EMC I/O EMC address bit 17
I/O Port 1 GPIO bit 17
EMC_A[18]/P1[18] K2 VDD_EMC I/O EMC address bit 18
I/O Port 1 GPIO bit 18
EMC_A[19]/P1[19] K1 VDD_EMC I/O EMC address bit 19
I/O Port 1 GPIO bit 19
EMC_A[20]/P1[20] J1 VDD_EMC I/O EMC address bit 20
I/O Port 1 GPIO bit 20
EMC_A[21]/P1[21] J2 VDD_EMC I/O EMC address bit 21
I/O Port 1 GPIO bit 21
EMC_A[22]/P1[22] J3 VDD_EMC I/O EMC address bit 22
I/O Port 1 GPIO bit 22
EMC_A[23]/P1[23] J4 VDD_EMC I/O EMC address bit 23
I/O Port 1 GPIO bit 23
EMC_BLS[0] U14 VDD_EMC O Static memory byte lane 0 select
EMC_BLS[1] T13 VDD_EMC O Static memory byte lane 1 select
EMC_BLS[2] R12 VDD_EMC O Static memory byte lane 2 select
EMC_BLS[3] P12 VDD_EMC O Static memory byte lane 3 select
EMC_CAS R5 VDD_EMC O SDRAM column address strobe out, active LOW
EMC_CKE0 U3 VDD_EMC O Clock enable out for SDRAM bank 0
EMC_CKE1 L2 VDD_EMC O Clock enable out for SDRAM bank 1
EMC_CLK T3 VDD_EMC O SDRAM clock out
EMC_CLKIN T4 VDD_EMC I SDRAM clock feedback
EMC_CS0 U13 VDD_EMC O EMC static memory chip select 0
EMC_CS1 R11 VDD_EMC O EMC static memory chip select 1
EMC_CS2 T12 VDD_EMC O EMC static memory chip select 2
EMC_CS3 V15 VDD_EMC O EMC static memory chip select 3
EMC_D[0] U4 VDD_EMC I/O: BK EMC data bit 0
EMC_D[1] R7 VDD_EMC I/O: BK EMC data bit 1
EMC_D[2] T5 VDD_EMC I/O: BK EMC data bit 2
EMC_D[3] U5 VDD_EMC I/O: BK EMC data bit 3
EMC_D[4] V3 VDD_EMC I/O: BK EMC data bit 4
EMC_D[5] V4 VDD_EMC I/O: BK EMC data bit 5
EMC_D[6] T6 VDD_EMC I/O: BK EMC data bit 6
EMC_D[7] R8 VDD_EMC I/O: BK EMC data bit 7
EMC_D[8] V5 VDD_EMC I/O: BK EMC data bit 8
Table 4. Pin description …continued
Symbol Pin Power supply
domain
Type Description
LPC3220_30_40_50 All information provided in this document is subject to legal disclaimers. © NXP B.V. 2011. All rights reserved.
Product data sheet Rev. 2 — 20 October 2011 12 of 79
NXP Semiconductors LPC3220/30/40/50
16/32-bit ARM microcontrollers
EMC_D[9] U6 VDD_EMC I/O: BK EMC data bit 9
EMC_D[10] V6 VDD_EMC I/O: BK EMC data bit 10
EMC_D[11] T7 VDD_EMC I/O: BK EMC data bit 11
EMC_D[12] U7 VDD_EMC I/O: BK EMC data bit 12
EMC_D[13] V7 VDD_EMC I/O: BK EMC data bit 13
EMC_D[14] T8 VDD_EMC I/O: BK EMC data bit 14
EMC_D[15] U8 VDD_EMC I/O: BK EMC data bit 15
EMC_D[16]/
EMC_DQS0
V8 VDD_EMC I/O: BK EMC data bit 16
I/O: BK DDR data strobe 0
EMC_D[17]/
EMC_DQS1
R9 VDD_EMC I/O: BK EMC data bit 17
I/O: BK DDR data strobe 1
EMC_D[18]/
EMC_CLK
V9 VDD_EMC I/O: P EMC data bit 18
I/O: P DDR inverted clock output
EMC_D[19]/P2[0] U9 VDD_EMC I/O: P EMC data bit 19
I/O: P Port 2 GPIO bit 0
EMC_D[20]/P2[1] T9 VDD_EMC I/O: P EMC data bit 20
I/O: P Port 2 GPIO bit 1
EMC_D[21]/P2[2] V10 VDD_EMC I/O: P EMC data bit 21
I/O: P Port 2 GPIO bit 2
EMC_D[22]/P2[3] U10 VDD_EMC I/O: P EMC data bit 22
I/O: P Port 2 GPIO bit 3
EMC_D[23]/P2[4] T10 VDD_EMC I/O: P EMC data bit 23
I/O: P Port 2 GPIO bit 4
EMC_D[24]/P2[5] R10 VDD_EMC I/O: P EMC data bit 24
I/O: P Port 2 GPIO bit 5
EMC_D[25]/P2[6] V11 VDD_EMC I/O: P EMC data bit 25
I/O: P Port 2 GPIO bit 6
EMC_D[26]/P2[7] U11 VDD_EMC I/O: P EMC data bit 26
I/O: P Port 2 GPIO bit 7
EMC_D[27]/P2[8] T11 VDD_EMC I/O: P EMC data bit 27
I/O: P Port 2 GPIO bit 8
EMC_D[28]/P2[9] V12 VDD_EMC I/O: P EMC data bit 28
I/O: P Port 2 GPIO bit 9
EMC_D[29]/P2[10] V13 VDD_EMC I/O: P EMC data bit 29
I/O: P Port 2 GPIO bit 10
EMC_D[30]/P2[11] U12 VDD_EMC I/O: P EMC data bit 30
I/O: P Port 2 GPIO bit 11
EMC_D[31]/P2[12] V14 VDD_EMC I/O: P EMC data bit 31
I/O: P Port 2 GPIO bit 12
EMC_DQM[0] R3 VDD_EMC O SDRAM data mask 0 out
Table 4. Pin description …continued
Symbol Pin Power supply
domain
Type Description
LPC3220_30_40_50 All information provided in this document is subject to legal disclaimers. © NXP B.V. 2011. All rights reserved.
Product data sheet Rev. 2 — 20 October 2011 13 of 79
NXP Semiconductors LPC3220/30/40/50
16/32-bit ARM microcontrollers
EMC_DQM[1] P4 VDD_EMC O SDRAM data mask 1 out
EMC_DQM[2] T1 VDD_EMC O SDRAM data mask 2 out
EMC_DQM[3] P5 VDD_EMC O SDRAM data mask 3 out
EMC_DYCS0 R6 VDD_EMC O SDRAM active LOW chip select 0
EMC_DYCS1 G1 VDD_EMC O SDRAM active LOW chip select 1
EMC_OE H1 VDD_EMC O EMC static memory output enable
EMC_RAS T2 VDD_EMC O SDRAM row address strobe, active LOW
EMC_WR R4 VDD_EMC O EMC write strobe, active LOW
FLASH_ALE D2 VDD_IOC O Flash address latch enable
FLASH_CE E3 VDD_IOC O Flash chip enable
FLASH_CLE F3 VDD_IOC O Flash command latch enable
FLASH_IO[0] H2 VDD_IOC I/O: BK Flash data bus, bit 0
FLASH_IO[1] H3 VDD_IOC I/O: BK Flash data bus, bit 1
FLASH_IO[2] F1 VDD_IOC I/O: BK Flash data bus, bit 2
FLASH_IO[3] E1 VDD_IOC I/O: BK Flash data bus, bit 3
FLASH_IO[4] H4 VDD_IOC I/O: BK Flash data bus, bit 4
FLASH_IO[5] G2 VDD_IOC I/O: BK Flash data bus, bit 5
FLASH_IO[6] G3 VDD_IOC I/O: BK Flash data bus, bit 6
FLASH_IO[7] E2 VDD_IOC I/O: BK Flash data bus, bit 7
FLASH_RD C1 VDD_IOC O Flash read enable
FLASH_RDY D1 VDD_IOC I Flash ready (from flash device)
FLASH_WR F2 VDD_IOC O Flash write enable
GPI_0/I2S1RX_SDA C16 VDD_IOD I General purpose input 0
I I2S1 Receive data
GPI_1/SERVICE C15 VDD_IOD I General purpose input 1
I Boot select input
GPI_2/CAP2[0]/
ENET_RXD3
C14 VDD_IOD I General purpose input 2
I Timer 2 capture input 0
I Ethernet receive data 3 (LPC3240 and LPC3250
only)
GPI_3 F4 VDD_IOC I General purpose input 3
GPI_4/SPI1_BUSY E13 VDD_IOD I General purpose input 4
I SPI1 busy input
GPI_5/U3_DCD N16 VDD_IOA I General purpose input 5
I UART 3 data carrier detect input
GPI_6/
HSTIM_CAP/
ENET_RXD2
C7 VDD_IOB I: BK General purpose input 6
I: BK High-speed timer capture input
I : BK Ethernet receive data 2 (LPC3240 and LPC3250
only)
Table 4. Pin description …continued
Symbol Pin Power supply
domain
Type Description
LPC3220_30_40_50 All information provided in this document is subject to legal disclaimers. © NXP B.V. 2011. All rights reserved.
Product data sheet Rev. 2 — 20 October 2011 14 of 79
NXP Semiconductors LPC3220/30/40/50
16/32-bit ARM microcontrollers
GPI_7/CAP4[0]/
MCABORT
D13 VDD_IOD I General purpose input 7
I Timer 4 capture input 0
I Motor control PWM LOW-active fast abort input
GPI_8/KEY_COL6/
SPI2_BUSY/
ENET_RX_DV
B16 VDD_IOD I General purpose input 8
I Keyscan column 6 input
I SPI2 busy input
I Ethernet receive data valid input (LPC3240 and
LPC3250 only)
GPI_9/KEY_COL7/
ENET_COL
E12 VDD_IOD I General purpose input 9
I Keyscan column 7 input
I Ethernet collision input (LPC3240 and LPC3250
only)
GPI_19/U4_RX B15 VDD_IOD I General purpose input 19
I UART 4 receive
GPI_28/U3_RI N17 VDD_IOA I General purpose input 28
I UART 3 ring indicator input
GPIO_0 A12 VDD_IOD I/O General purpose input/output 0
GPIO_1 A11 VDD_IOD I/O General purpose input/output 1
GPIO_2/
KEY_ROW6/
ENET_MDC
D9 VDD_IOD I/O General purpose input/output 2
O Keyscan row 6 output
O Ethernet PHY interface clock (LPC3240 and
LPC3250 only)
GPIO_3/
KEY_ROW7/
ENET_MDIO
C11 VDD_IOD I/O General purpose input/output 3
I/O Keyscan row 7 output
I/O Ethernet PHY interface data (LPC3240 and
LPC3250 only)
GPIO_4/
SSEL1/
LCDVD[22]
B11 VDD_IOD I/O General purpose input/output 4
I/O SSP1 Slave Select
I/O LCD data bit 22 (LPC3230 and LPC3250 only)
GPIO_5/
SSEL0/
MCI0
E9 VDD_IOD I/O General purpose input/output 5
I/O SSP0 Slave Select
I/O Motor control channel 0 input
GPO_0/
TST_CLK1
C3 VDD_IOC O General purpose output 0
O Test clock 1 out
GPO_1 D4 VDD_IOC O General purpose output 1
GPO_2/
MAT1[0]/
LCDVD[0]
B14 VDD_IOD O General purpose output 2
O Timer 1 match output 0
O LCD data bit 0 (LPC3230 and LPC3250 only)
GPO_3/
LCDVD[1]
D12 VDD_IOD O General purpose output 3
O LCD data bit 1 (LPC3230 and LPC3250 only)
GPO_4 D8 VDD_IOB O General purpose output 4
Table 4. Pin description …continued
Symbol Pin Power supply
domain
Type Description
LPC3220_30_40_50 All information provided in this document is subject to legal disclaimers. © NXP B.V. 2011. All rights reserved.
Product data sheet Rev. 2 — 20 October 2011 15 of 79
NXP Semiconductors LPC3220/30/40/50
16/32-bit ARM microcontrollers
GPO_5 B3 VDD_IOC O General purpose output 5
GPO_6/
LCDVD[18]
A16 VDD_IOD O General purpose output 6
O LCD data bit 18 (LPC3230 and LPC3250 only)
GPO_7/
LCDVD[2]
A15 VDD_IOD O General purpose output 7
O LCD data bit 2 (LPC3230 and LPC3250 only)
GPO_8/
LCDVD[8]
C13 VDD_IOD O General purpose output 8
O LCD data bit 8 (LPC3230 and LPC3250 only)
GPO_9/
LCDVD[9]
C12 VDD_IOD O General purpose output 9
O LCD data bit 9 (LPC3230 and LPC3250 only)
GPO_10/
MCOB2/
LCDPWR
E11 VDD_IOD O General purpose output 10
O Motor control PWM channel 2, output B
O LCD panel power enable (LPC3230 and LPC3250
only)
GPO_11 E8 VDD_IOB O General purpose output 11
GPO_12/
MCOA2/
LCDLE
B12 VDD_IOD O General purpose output 12
O Motor control PWM channel 2, output A
O LCD line end signal (LPC3230 and LPC3250 only)
GPO_13/
MCOB1/
LCDDCLK
B13 VDD_IOD O General purpose output 13
O Motor control PWM channel 1, output B
O LCD clock output (LPC3230 and LPC3250 only)
GPO_14 D3 VDD_IOC O General purpose output 14
GPO_15/
MCOA1/
LCDFP
A14 VDD_IOD O General purpose output 15
O Motor control PWM channel 1, output A
O LCD frame/sync pulse (LPC3230 and LPC3250
only)
GPO_16/
MCOB0/
LCDENAB/LCDM
D10 VDD_IOD O General purpose output 16
O Motor control PWM channel 0, output B
O LCD STN AC bias/TFT data enable (LPC3230 and
LPC3250 only)
GPO_17 N18 VDD_IOA O General purpose output 17
GPO_18/
MCOA0/
LCDLP
D11 VDD_IOD O General purpose output 18
O Motor control PWM channel 0, output A
O LCD line sync/horizontal sync (LPC3230 and
LPC3250 only)
GPO_19 C2 VDD_IOC O General purpose output 19
GPO_20 B2 VDD_IOC O General purpose output 20
GPO_21/
U4_TX/
LCDVD[3]
A13 VDD_IOD O General purpose output 21
O UART 4 transmit
O LCD data bit 3 (LPC3230 and LPC3250 only)
Table 4. Pin description …continued
Symbol Pin Power supply
domain
Type Description
LPC3220_30_40_50 All information provided in this document is subject to legal disclaimers. © NXP B.V. 2011. All rights reserved.
Product data sheet Rev. 2 — 20 October 2011 16 of 79
NXP Semiconductors LPC3220/30/40/50
16/32-bit ARM microcontrollers
GPO_22/
U7_HRTS/
LCDVD[14]
E10 VDD_IOD O General purpose output 22
O HS UART 7 RTS out
O LCD data bit 14 (LPC3230 and LPC3250 only)
GPO_23/
U2_HRTS/
U3_RTS
M16 VDD_IOA O General purpose output 23
O HS U ART 2 RTS out
O UART 3 RTS out
HIGHCORE/
LCDVD[17]
H16 VDD_IOD O Core voltage control out
O LCD data bit 17 (LPC3230 and LPC3250 only)
I2C1_SCL A5 VDD_IOB I/O T I2C1 serial clock input/output
I2C1_SDA B6 VDD_IOB I/O T I2C1 serial data input/output
I2C2_SCL A3 VDD_IOC I/O T I2C2 serial clock input/output
I2C2_SDA E4 VDD_IOC I/O T I2C2 serial data input/output
I2S1TX_CLK/
MAT3[0]
A4 VDD_IOB I/O I2S1 transmit clock
O Timer 3 match output 0
I2S1TX_SDA/
MAT3[1]
E7 VDD_IOB I/O I2S1 transmit data
O Timer 3 match output 1
I2S1TX_WS/
CAP3[0]
B4 VDD_IOB I/O I2S1 transmit word select
I/O Timer 3 capture input 0
JTAG_NTRST H17 VDD_IOD I: PU JTAG1 reset input. Must be LOW during power-on
reset.
JTAG_RTCK H18 VDD_IOD O JTAG1 return clock out
JTAG_TCK H14 VDD_IOD I JTAG1 clock input
JTAG_TDI J16 VDD_IOD I: PU JTAG1 data input
JTAG_TDO J15 VDD_IOD O JTAG1 data out
JTAG_TMS G18 VDD_IOD I: PU TAG1 test mode select input
KEY_COL0/
ENET_TX_CLK
F15 VDD_IOD I Keyscan column 0 input
I Ethernet transmit clock (LPC3240 and LPC3250
only)
KEY_COL1/
ENET_RX_CLK/
ENET_REF_CLK
E16 VDD_IOD I Keyscan column 1 input
I Ethernet receive clock (MII mode, LPC3240 and
LPC3250 only)
I Ethernet reference clock (RMII mode, LPC3240 and
LPC3250 only)
KEY_COL2/
ENET_RX_ER
D17 VDD_IOD I Keyscan column 2 input
I Ethernet receive error input (LPC3240 and
LPC3250 only)
KEY_COL3/
ENET_CRS
D18 VDD_IOD I Keyscan column 3 input
I Ethernet carrier sense input (LPC3240 and
LPC3250 only)
KEY_COL4/
ENET_RXD0
G15 VDD_IOD I Keyscan column 4 input
I Ethernet receive data 0 (LPC3240 and LPC3250
only)
Table 4. Pin description …continued
Symbol Pin Power supply
domain
Type Description
LPC3220_30_40_50 All information provided in this document is subject to legal disclaimers. © NXP B.V. 2011. All rights reserved.
Product data sheet Rev. 2 — 20 October 2011 17 of 79
NXP Semiconductors LPC3220/30/40/50
16/32-bit ARM microcontrollers
KEY_COL5/
ENET_RXD1
F16 VDD_IOD I Keyscan column 5 input
I Ethernet receive data 1 (LPC3240 and LPC3250
only)
KEY_ROW0/
ENET_TX_ER
E15 VDD_IOD I/O T Keyscan row 0 out
I/O T Ethernet transmit error (LPC3240 and LPC3250
only)
KEY_ROW1/
ENET_TXD2
E14 VDD_IOD I/O T Keyscan row 1 out
I/O T Ethernet transmit data 2 (LPC3240 and LPC3250
only)
KEY_ROW2/
ENET_TXD3
F14 VDD_IOD I/O T Keyscan row 2 out
I/O T Ethernet transmit data 3 (LPC3240 and LPC3250
only)
KEY_ROW3/
ENET_TX_EN
D16 VDD_IOD I/O T Keyscan row 3 out
I/O T Ethernet transmit enable (LPC3240 and LPC3250
only)
KEY_ROW4/
ENET_TXD0
C17 VDD_IOD I/O T Keyscan row 4 out
I/O T Ethernet transmit data 0 (LPC3240 and LPC3250
only)
KEY_ROW5/
ENET_TXD1
C18 VDD_IOD I/O T Keyscan row 5 out
I/O T Ethernet transmit data 1 (LPC3240 and LPC3250
only)
MS_BS/MAT2[1] A6 VDD_IOD I/O: P MS/SD card command out
O Timer 2 match output 1
MS_DIO0/MAT0[0] A8 VDD_IOD I/O: P MS/SD card data 0
O Timer 0 match output 0
MS_DIO1/
MAT0[1]
A7 VDD_IOD I/O: P MS/SD card data 1
O Timer 0 match output 1
MS_DIO2/
MAT0[2]
B8 VDD_IOD I/O: P MS/SD card data 2
O Timer 0 match output 2
MS_DIO3/
MAT0[3]
C8 VDD_IOD I/O: P MS/SD card data 3
O Timer 0 match output 3
MS_SCLK/
MAT2[0]
B7 VDD_IOD I/O MS/SD card clock output
O Timer 2 match output 0
n.c. B17,
U17,
U2
- - not connected
ONSW M15 VDD_RTC O RTC match output for external power control
P0[0]/
I2S1RX_CLK
B5 VDD_IOB I/O Port 0 GPIO bit 0
I/O I2S1 receive clock
P0[1]/
I2S1RX_WS
D7 VDD_IOB I/O Port 0 GPIO bit 1
I/O I2S1 receive word select
Table 4. Pin description …continued
Symbol Pin Power supply
domain
Type Description
LPC3220_30_40_50 All information provided in this document is subject to legal disclaimers. © NXP B.V. 2011. All rights reserved.
Product data sheet Rev. 2 — 20 October 2011 18 of 79
NXP Semiconductors LPC3220/30/40/50
16/32-bit ARM microcontrollers
P0[2]/
I2S0RX_SDA/
LCDVD[4]
M17 VDD_IOA I/O Port 0 GPIO bit 2
I/O I2S0 receive data
I/O LCD data bit 4 (LPC3230 and LPC3250 only)
P0[3]/
I2S0RX_CLK/
LCDVD[5]
M18 VDD_IOA I/O Port 0 GPIO bit 3
I/O I2S0 receive clock
I/O LCD data bit 5 (LPC3230 and LPC3250 only)
P0[4]/
I2S0RX_WS/
LCDVD[6]
L15 VDD_IOA I/O Port 0 GPIO bit 4
I/O I2S0 receive word select
I/O LCD data bit 6 (LPC3230 and LPC3250 only)
P0[5]/
I2S0TX_SDA/
LCDVD[7]
L16 VDD_IOA I/O Port 0 GPIO bit 5
I/O I2S0 transmit data
I/O LCD data bit 7 (LPC3230 and LPC3250 only)
P0[6]/
I2S0TX_CLK/
LCDVD[12]
L17 VDD_IOA I/O Port 0 GPIO bit 6
I/O I2S0 transmit clock
I/O LCD data bit 12 (LPC3230 and LPC3250 only)
P0[7]/
I2S0TX_WS/
LCDVD[13]
L18 VDD_IOA I/O Port 0 GPIO bit 7
I/O I2S0 transmit word select
I/O LCD data bit 13 (LPC3230 and LPC3250 only)
PLL397_LOOP R14 VDD_PLL397 analog filter PLL397 loop filter
(for external components)
PWM_OUT1/
LCDVD[16]
D14 VDD_IOD O PWM1 out
O LCD data bit 16 (LPC3230 and LPC3250 only)
PWM_OUT2/INTSTAT/
LCDVD[19]
D15 VDD_IOD O PWM2 output/internal interrupt status[1]
O LCD data bit 19 (LPC3230 and LPC3250 only)
RESET M14 VDD_RTC I Reset input, active LOW
RESOUT G4 VDD_IOC O Reset out. Reflects external and WDT reset
RTCX_IN P16 VDD_RTC analog in RTC oscillator input
RTCX_OUT P17 VDD_RTC analog out RTC oscillator output
SPI1_CLK/
SCK0
C9 VDD_IOD O SPI1 clock out
O SSP0 clock out
SPI1_DATIN/
MISO0/
GPI_25/
MCI1
C10 VDD_IOD I/O SPI1 data in
I/O SSP0 MISO
I/O General purpose input bit 25
I Motor control channel 1 input
SPI1_DATIO/
MOSI0/
MCI2
B9 VDD_IOD I/O SPI1 data out (and optional input)
I/O SSP0 MOSI
I Motor control channel 2 input
SPI2_CLK/
SCK1/
LCDVD[23]
B10 VDD_IOD I/O SPI2 clock out
I/O SSP1 clock out
I/O LCD data bit 23 (LPC3230 and LPC3250 only)
Table 4. Pin description …continued
Symbol Pin Power supply
domain
Type Description
LPC3220_30_40_50 All information provided in this document is subject to legal disclaimers. © NXP B.V. 2011. All rights reserved.
Product data sheet Rev. 2 — 20 October 2011 19 of 79
NXP Semiconductors LPC3220/30/40/50
16/32-bit ARM microcontrollers
SPI2_DATIO/
MOSI1/
LCDVD[20]
A9 VDD_IOD I/O SPI2 data out (and optional input)
I/O SSP1 MOSI
I/O LCD data bit 20 (LPC3230 and LPC3250 only)
SPI2_DATIN/
MISO1/
LCDVD[21]/
GPI_27
A10 VDD_IOD I/O SPI2 data in
I/O SSP1 MISO
I/O LCD data 21 (LPC3230 and LPC3250 only)
I/O General purpose input bit 27
SYSCLKEN/
LCDVD[15]
G17 VDD_IOD I/O T Clock request out for external clock source
I/O T LCD data bit 15 (LPC3230 and LPC3250 only)
SYSX_IN T17 VDD_OSC analog in System clock oscillator input
SYSX_OUT R15 VDD_OSC analog out System clock oscillator output
TS_XP R13 VDD_AD I/O Touchscreen X output
TS_YP U16 VDD_AD I/O Touchscreen Y output
TST_CLK2 C6 VDD_IOB O Test clock 2 out
U1_RX/CAP1[0]/
GPI_15
K15 VDD_IOA I/O HS UART 1 receive
I/O Timer 1 capture input 0
I/O General purpose input bit 15
U1_TX K16 VDD_IOA O HS UART 1 transmit
U2_HCTS/
U3_CTS/GPI_16
J18 VDD_IOA I/O HS UART 2 Clear to Send input
I UART 3 Clear to Send
I/O General purpose input bit 16
U2_RX/
U3_DSR/GPI_17
K18 VDD_IOA I/O HS UART 2 receive
I/O UART 3 data set ready
I/O General purpose input bit 17
U2_TX/U3_DTR K17 VDD_IOA O HS UART 2 transmit
O UART 3 data terminal ready out
U3_RX/
GPI_18
J14 VDD_IOD I/O UART 3 receive
I/O General purpose input bit 18
U3_TX J17 VDD_IOD O UART 3 transmit
U5_RX/
GPI_20
F18 VDD_IOD I/O UART 5 receive
I General purpose input bit 20
U5_TX H15 VDD_IOD O UART 5 transmit
U6_IRRX/
GPI_21
F17 VDD_IOD I/O UART 6 receive (with IrDA)
I General purpose input bit 21
U6_IRTX G16 VDD_IOD O UART 6 transmit (with IrDA)
U7_HCTS/
CAP0[1]/
LCDCLKIN/
GPI_22
G13 VDD_IOD I HS UART 7 CTS in
I Timer 0 capture input 1
I LCD panel clock in (LPC3230 and LPC3250 only)
I General purpose input bit 22
Table 4. Pin description …continued
Symbol Pin Power supply
domain
Type Description
LPC3220_30_40_50 All information provided in this document is subject to legal disclaimers. © NXP B.V. 2011. All rights reserved.
Product data sheet Rev. 2 — 20 October 2011 20 of 79
NXP Semiconductors LPC3220/30/40/50
16/32-bit ARM microcontrollers
U7_RX/
CAP0[0]/
LCDVD[10]/
GPI_23
E17 VDD_IOD I/O HS UART 7 receive
I/O Timer 0 capture input 0
I/O LCD data bit 10 (LPC3230 and LPC3250 only)
I/O General purpose input bit 23
U7_TX/
MAT1[1]/
LCDVD[11]
E18 VDD_IOD O HS UART 7 transmit
O Timer 1 match output 1
O LCD data bit 11 (LPC3230 and LPC3250 only)
USB_ATX_INT C4 VDD_IOC I Interrupt from USB ATX
USB_DAT_VP/
U5_RX
D5 VDD_IOC I/O: P USB transmit data, D+ receive
I/O: P UART 5 receive
USB_I2C_SCL E5 VDD_IOC I/O T I2C clock for USB ATX interface
USB_I2C_SDA E6 VDD_IOC I/O T I2C data for USB ATX interface
USB_OE_TP D6 VDD_IOC I/O USB transmit enable for DAT/SE0
USB_SE0_VM/
U5_TX
C5 VDD_IOC I/O: P USB single ended zero transmit, D Receive
I/O: P UART 5 transmit
VDD_AD N12,
N13
VDD_AD power 3.3 V supply for ADC/touch screen
VDD_CORE G7,
G9,
G11,
J7,
J12,
M7,
M11
VDD_CORE power 1.2 V or 0.9 V supply for core
VDD_COREFXD L12,
M13
VDD_COREFXD power Fixed 1.2 V supply for digital portion of the analog
block
VDD_EMC J6,
K6,
K7,
L6,
M6,
M8,
N7,
N8,
N9,
N10,
N11
VDD_EMC power 1.8 V or 2.5 V or 3.3 V supply for
External Memory Controller (EMC)
VDD_IOA H13,
J13
VDD_IOA power 1.8 V or 3.3 V supply for IOA domain
VDD_IOB F8 VDD_IOB power 1.8 V or 3.3 V supply for IOB domain
VDD_IOC F7,
G6,
H6, J5
VDD_IOC power 1.8 V or 3.3 V supply for IOC domain
VDD_IOD F13,
F9
VDD_IOD power 1.8 V to 3.3 V supply for IOD domain
VDD_OSC T18 VDD_OSC power 1.2 V supply for main oscillator
Table 4. Pin description …continued
Symbol Pin Power supply
domain
Type Description
LPC3220_30_40_50 All information provided in this document is subject to legal disclaimers. © NXP B.V. 2011. All rights reserved.
Product data sheet Rev. 2 — 20 October 2011 21 of 79
NXP Semiconductors LPC3220/30/40/50
16/32-bit ARM microcontrollers
[1] The PWM2_CTRL register controls this pin function (see LPC32x0 User manual).
VDD_PLL397 T16 VDD_PLL397 power 1.2 V supply for 397x PLL
VDD_PLLHCLK R17 VDD_PLLHCLK power 1.2 V supply for HCLK PLL
VDD_PLLUSB P15 VDD_PLLUSB power 1.2 V supply for USB PLL
VDD_FUSE N14 VDD_FUSE power 1.2 V supply
VDD_RTC K14 VDD_RTC power 1.2 V supply for RTC I/O
VDD_RTCCORE L13 VDD_RTCCORE power 1.2 V supply for RTC
VDD_RTCOSC N15 VDD_RTCOSC power 1.2 V supply for RTC oscillator
VSS_AD P13 - power Ground for ADC/touch screen
VSS_CORE G8,
G10,
G12,
H7,
K12,
L7,
M9,
M10,
M12
- power Ground for core
VSS_EMC K5,
L5,
M5,
N5,
N6,
P6,
P7,
P8,
P9,
P10,
P11
- power Ground for EMC
VSS_IOA K13 - power Ground VDD_IOA domain
VSS_IOB F6 - power Ground VDD_IOB domain
VSS_IOC F5,
G5,
H5
- power Ground VDD_IOC domain
VSS_IOD F10,
F11,
F12,
H12
- power Ground VDD_IOD domain
VSS_OSC P14 - power Ground for main oscillator
VSS_PLL397 T15 - power Ground for 397x PLL
VSS_PLLHCLK R18 - power Ground for HCLK PLL
VSS_PLLUSB R16 - power Ground for USB PLL
VSS_RTCCORE L14 - power Ground for RTC
VSS_RTCOSC P18 - power Ground for RTC oscillator
Table 4. Pin description …continued
Symbol Pin Power supply
domain
Type Description
LPC3220_30_40_50 All information provided in this document is subject to legal disclaimers. © NXP B.V. 2011. All rights reserved.
Product data sheet Rev. 2 — 20 October 2011 22 of 79
NXP Semiconductors LPC3220/30/40/50
16/32-bit ARM microcontrollers
[1] See LPC32x0 User manual for details.
Table 5. Digital I/O pad types[1]
Parameter Abbreviation
I/O type I = input.
O = output.
I/O = bidirectional.
I/O T = bidirectional or high impedance.
Pin detail BK: pin has a bus keeper function that weakly retains the last logic
level driven on an I/O pin.
Bus keeper current for different I/O pin voltages:
0 V= 1 A (max)
VDD_x = 1 A (max)
2/3 VDD_x = 55 A (max)
1/3 VDD_x = 60 A (max)
PU: pin has a nominal 50 A internal pull-up connected.
PD: pin has a nominal 50 A internal pull-down connected.
P: pin has programmable input characteristics.
Table 6. Supply domains
Supply domain Voltage range Related supply
pins
Description
VDD_CORE 0.9 V to 1.39 V VDD_CORE Core power domain.
VDD_COREFXD 1.2 V VDD_COREFXD Fixed 1.2 V supply for digital portion of
the analog block.
other core
domains
1.2 V VDD_PLL397,
VDD_PLLHCLK,
VDD_PLLUSB,
VDD_FUSE,
VDD_OSC
1.2 V supplies, tied to
VDD_COREFXD.
VDD_RTC 0.9 V to 1.39 V VDD_RTC,
VDD_RTCCORE,
VDD_RTCOSC
RTC supply domain. Can be
connected to a battery backed-up
power source.
VDD_AD 2.7 V to 3.6 V VDD_AD 3.3 V supply for ADC and touch
screen.
VDD_EMC 1.7 V to 1.95 V
2.3 V to 2.7 V
2.7 V to 3.6 V
VDD_EMC External memory interface IO pins in
1.8 V range, 2.5 V range, or 3.3 V
range.
VDD_IOA[1] 1.7 V to 1.95 V or
2.7 V to 3.6 V
VDD_IOA Peripheral supply.
VDD_IOB[1] 1.7 V to 1.95 V or
2.7 V to 3.6 V
VDD_IOB Peripheral supply.
VDD_IOC[1] 1.7 V to 1.95 V or
2.3 V to 3.6 V
VDD_IOC Peripheral supply.
VDD_IOD[1] 1.7 V to 1.95 V or
2.7 V to 3.6 V
VDD_IOD Peripheral supply.
LPC3220_30_40_50 All information provided in this document is subject to legal disclaimers. © NXP B.V. 2011. All rights reserved.
Product data sheet Rev. 2 — 20 October 2011 23 of 79
NXP Semiconductors LPC3220/30/40/50
16/32-bit ARM microcontrollers
[1] The VDD_IOA, VDD_IOB, VDD_IOC, and VDD_IOD supply domains can be operated at a voltage
independent of the other domains as long as all pins connected to the same peripheral are at the same
voltage level. There are two special cases for determining supply domain voltages (for details see
application note AN10777):
a) Ethernet configured in MII mode: VDD_IOD must be the same as VDD_IOB.
b) UART 3 when used with hardware flow control or when sharing an RS-232 transceiver with another
UART: VDD_IOA must be the same as VDD_IOD.
LPC3220_30_40_50 All information provided in this document is subject to legal disclaimers. © NXP B.V. 2011. All rights reserved.
Product data sheet Rev. 2 — 20 October 2011 24 of 79
NXP Semiconductors LPC3220/30/40/50
16/32-bit ARM microcontrollers
7. Functional description
7.1 CPU and subsystems
7.1.1 CPU
NXP created the LPC3220/30/40/50 using an ARM926EJ-S CPU core that includes a
Harvard architecture and a 5-stage pipeline. To this ARM core, NXP implemented a 32 kB
instruction cache, a 32 kB data cache and a Vector Floating Point coprocessor. The
ARM926EJ-S core also has an integral Memory Management Unit (MMU) to provide the
virtual memory capabilities required to support the multi-programming demands of
modern operating systems. The basic ARM926EJ-S core V5TE instruction set includes
DSP instruction extensions for native Jazelle Java Byte-code execution in hardware. The
LPC3220/30/40/50 operates at CPU frequencies up to 266 MHz.
7.1.2 Vector Floating Point (VFP) coprocessor
The LPC3220/30/40/50 includes a VFP co-processor providing full support for
single-precision and double-precision add, subtract, multiply, divide, and
multiply-accumulate operations at CPU clock speeds. It is compliant with the IEEE 754
standard for binary Floating-Point Arithmetic. This hardware floating point capability
makes the microcontroller suitable for advanced motor control and DSP applications. The
VFP has 3 separate pipelines for floating-point MAC operations, divide or square root
operations, and Load/Store operations. These pipelines operate in parallel and can
complete execution out of order. All single-precision instructions execute in one cycle,
except the divide and square root instructions. All double-precision multiply and
multiply-accumulate instructions take two cycles. The VFP also provides format
conversions between floating-point and integer word formats.
7.1.3 Emulation and debugging
The LPC3220/30/40/50 supports emulation and debugging via a dedicated JTAG serial
port. An Embedded Trace Buffer allows tracing program execution. The dedicated JTAG
port allows debugging of all chip features without impact to any pins that may be used in
the application.
7.1.3.1 Embedded ICE
Standard ARM EmbeddedICE logic provides on-chip debug support. The debugging of
the target system requires a host computer running the debugger software and an
Embedded ICE protocol converter. The Embedded ICE protocol converter converts the
Remote Debug Protocol commands to the JTAG data needed to access the ARM core.
The ARM core has a Debug Communication Channel (DCC) function built-in. The debug
communication channel allows a program running on the target to communicate with the
host debugger or another separate host without stopping the program flow or entering the
debug state.
LPC3220_30_40_50 All information provided in this document is subject to legal disclaimers. © NXP B.V. 2011. All rights reserved.
Product data sheet Rev. 2 — 20 October 2011 25 of 79
NXP Semiconductors LPC3220/30/40/50
16/32-bit ARM microcontrollers
7.1.3.2 Embedded trace buffer
The Embedded Trace Module (ETM) is connected directly to the ARM core. It compresses
the trace information and exports it through a narrow trace port. An internal Embedded
Trace Buffer (ETB) of 2048 24 bits captures the trace information under software
debugger control. Data from the ETB is recovered by the debug software through the
JTAG port.
The trace contains information about when the ARM core switches between states.
Instruction shows the flow of execution of the processor and provides a list of all the
instructions that were executed. Instruction trace is significantly compressed by only
broadcasting branch addresses as well as a set of status signals that indicate the pipeline
status on a cycle by cycle basis. For data accesses either data or address or both can be
traced.
7.2 AHB matrix
The LPC3220/30/40/50 has a multi-layer AHB matrix for inter-block communication. AHB
is an ARM defined high-speed bus, which is part of the ARM bus architecture. AHB is a
high-bandwidth low-latency bus that supports multi-master arbitration and a bus
grant/request mechanism. For systems that have only one (CPU), or two (CPU and DMA)
bus masters a simple AHB works well. However, if a system requires multiple bus masters
and the CPU needs access to external memory, a single AHB bus can cause a bottleneck.
To increase performance, the LPC3220/30/40/50 uses an expanded AHB architecture
known as Multi-layer AHB. A Multi-layer AHB replaces the request/grant and arbitration
mechanism used in a simple AHB with an interconnect matrix that moves arbitration out
toward the slave devices. Thus, if a CPU and a DMA controller want access to the same
memory, the interconnect matrix arbitrates between the two when granting access to the
memory. This advanced architecture allows simultaneous access by bus masters to
different resources with an increase in arbitration complexity. In this architectural
implementation, removing guaranteed central arbitration and allowing more than one bus
master to be active at the same time provides better overall microcontroller performance.
In the LPC3220/30/40/50, the multi-Layer AHB system has a separate bus for each of
seven AHB Masters:
• CPU data bus
• CPU instruction bus
• General purpose DMA Master 0
• General purpose DMA Master 1
• Ethernet controller
• USB controller
• LCD controller
There are no arbitration delays unless two masters attempt to access the same slave at
the same time.
LPC3220_30_40_50 All information provided in this document is subject to legal disclaimers. © NXP B.V. 2011. All rights reserved.
Product data sheet Rev. 2 — 20 October 2011 26 of 79
NXP Semiconductors LPC3220/30/40/50
16/32-bit ARM microcontrollers
7.2.1 APB
Many peripheral functions are accessed by on-chip APBs that are attached to the higher
speed AHB. The APB performs reads and writes to peripheral registers in three peripheral
clocks.
7.2.2 FAB
Some peripherals are placed on a special bus called FAB that allows faster CPU access
to those peripheral functions. A write access to FAB peripherals takes a single AHB clock
and a read access to FAB peripherals takes two AHB clocks.
7.3 Physical memory map
The physical memory map incorporates several distinct regions, as shown in Figure 3.
When an application is running, the CPU interrupt vectors are re-mapped to allow them to
reside in on-chip SRAM (IRAM).
LPC3220_30_40_50 All information provided in this document is subject to legal disclaimers. © NXP B.V. 2011. All rights reserved.
Product data sheet Rev. 2 — 20 October 2011 27 of 79
NXP Semiconductors LPC3220/30/40/50
16/32-bit ARM microcontrollers
Fig 3. LPC3220/30/40/50 memory map
on-chip memory
0x4000 0000
0x0000 0000
0.0 GB
768 MB
1.0 GB
4.0 GB
peripherals on AHB
matrix slave port 5
0x0FFF FFFF
0x2000 0000
0x3000 0000
0x2FFF FFFF
0x1FFF FFFF
0x8000 0000
0xFFFF FFFF
0x1000 0000
0x3FFF FFFF
0x4FFF FFFF
0x5000 0000
0x7FFF FFFF
peripherals on AHB
matrix slave port 6
peripherals on AHB
matrix slave port 7
off-chip memory
IROM or IRAM 0x0000 0000 to 0x03FF FFFF
dummy space for DMA 0x0400 0000 to 0x07FF FFFF
IRAM 0x0800 0000 to 0x0BFF FFFF
IROM 0x0C00 0000 to 0x0FFF FFFF
AHB peripherals 0x2000 0000 to 0x2007 FFFF
AHB peripherals 0x200A 0000 to 0x200B FFFF
APB peripherals 0x2008 0000 to 0x2009 FFFF
RESERVED
AHB peripherals 0x3000 0000 to 0x31FF FFFF
RESERVED
FAB peripherals 0x4000 0000 to 0x4007 FFFF
APB peripherals 0x4008 0000 to 0x400F FFFF
RESERVED
RESERVED
RESERVED
RESERVED
RESERVED
0x9FFF FFFF
0xA000 0000
0xBFFF FFFF
0xC000 0000
0xDFFF FFFF
0xE000 0000
0xE0FF FFFF
0xE100 0000
0xE1FF FFFF
0xE200 0000
0xE2FF FFFF
0xE300 0000
0xE3FF FFFF
0xE400 0000
2.0 GB
EMC_DYCS0
EMC_DYCS1
EMC_CS0
EMC_CS1
EMC_CS2
EMC_CS3
002aae468
LPC3220_30_40_50 All information provided in this document is subject to legal disclaimers. © NXP B.V. 2011. All rights reserved.
Product data sheet Rev. 2 — 20 October 2011 28 of 79
NXP Semiconductors LPC3220/30/40/50
16/32-bit ARM microcontrollers
7.4 Internal memory
7.4.1 On-chip ROM
The built-in 16 kB ROM contains a program which runs a boot procedure to load code
from one of four external sources, UART 5, SSP0 (SPI mode), EMC Static CS0 memory,
or NAND FLASH.
After reset, execution always begins from the internal ROM. The bootstrap software first
reads the SERVICE input (GPI_1). If SERVICE is LOW, the bootstrap starts a service boot
and can download a program over serial link UART 5 to IRAM and transfer execution to
the downloaded code.
If the SERVICE pin is HIGH, the bootstrap routine jumps to normal boot. The normal boot
process first tests SPI memory for boot information if present it uploads the boot code and
transfers execution to the uploaded software. If the SPI is not present or no software is
loaded, the bootloader will test the EMC Static CS0 memory for the presence of boot code
and if present boots from static memory, If this test fails the boot loader will test external
NAND flash for boot code and boot if code is present.
The boot loader consumes no user memory space because it is in ROM.
7.4.2 On-chip SRAM
On-chip SRAM may be used for code and/or data storage. The SRAM may be accessed
as 8, 16, or 32 bit memory. The LPC3220/30/40/50 provides 256 kB of internal SRAM.
7.5 External memory interfaces
The LPC3220/30/40/50 includes three external memory interfaces, NAND Flash
controllers, Secure Digital Memory Controller, and an external memory controller for
SDRAM, DDR SDRAM, and Static Memory devices.
7.5.1 NAND flash controllers
The LPC3220/30/40/50 includes two NAND flash controllers, one for multi-level cell
NAND flash devices and one for single-level cell NAND flash devices. The two NAND
flash controllers use the same pins to interface to external NAND flash devices, so only
one interface is active at a time.
7.5.1.1 Multi-Level Cell (MLC) NAND flash controller
The MLC NAND flash controller interfaces to either multi-level or single-level NAND flash
devices. An external NAND flash device is used to allow the bootloader to automatically
load a portion of the application code into internal SRAM for execution following reset.
The MLC NAND flash controller supports small (528 byte) and large (2114 byte) pages.
Programmable NAND timing parameters allow support for a variety of NAND flash
devices. A built-in Reed-Solomon encoder/decoder provides error detection and
correction capability. A 528 byte data buffer reduces the need for CPU supervision during
loading. The MLC NAND flash controller also provides DMA support.
LPC3220_30_40_50 All information provided in this document is subject to legal disclaimers. © NXP B.V. 2011. All rights reserved.
Product data sheet Rev. 2 — 20 October 2011 29 of 79
NXP Semiconductors LPC3220/30/40/50
16/32-bit ARM microcontrollers
7.5.1.2 Single-Level Cell (SLC) NAND flash controller
The SLC NAND flash controller interfaces to single-level NAND flash devices. DMA page
transfers are supported, including a 20-byte DMA read and write FIFO. Hardware support
for ECC (Error Checking and Correction) is included for the main data area. Software can
correct a single bit error.
7.5.2 SD card controller
The SD interface allows access to external SD memory cards. The SD card interface
conforms to the SD Memory Card Specification Version 1.01.
7.5.2.1 Features
• 1-bit and 4-bit data line interface support.
• DMA is supported through the system DMA controller.
• Provides all functions specific to the SD memory card. These include the clock
generation unit, power management control, command and data transfer.
7.5.3 External memory controller
The LPC3220/30/40/50 includes a memory controller that supports data bus SDRAM,
DDR SDRAM, and static memory devices. The memory controller provides an interface
between the system bus and external (off-chip) memory devices.
The controller supports 16-bit and 32-bit wide SDR SDRAM devices of 64 Mbit, 128 Mbit,
128 Mbit, 256 Mbit, and 512 Mbit sizes, as well as 16-bit wide data bus DDR SDRAM
devices of 64 Mbit, 128 Mbit, 128 Mbit, 256 Mbit, and 512 Mbit sizes. Two dynamic
memory chip selects are supplied, supporting two groups of SDRAM:
• DYCS0 in the address range 0x8000 0000 to 0x9FFF FFFF
• DYCS1 in the address range 0xA000 0000 to 0xBFFF FFFF
The memory controller also supports 8-bit, 16-bit, and 32-bit wide asynchronous static
memory devices, including RAM, ROM, and flash, with or without asynchronous page
mode. Four static memory chip selects are supplied for SRAM devices:
• CS0 in the address range 0xE000 0000 to 0xE0FF FFFF
• CS1 in the address range 0xE100 0000 to 0xE1FF FFFF
• CS2 in the address range 0xE200 0000 to 0xE2FF FFFF
• CS3 in the address range 0xE300 0000 to 0xE3FF FFFF
The SDRAM controller uses three data ports to allow simultaneous requests from multiple
on-chip AHB bus masters and has the following features.
• Dynamic memory interface supports SDRAM, DDR-SDRAM, and low-power variants.
• Read and write buffers to reduce latency and improve performance.
• Static memory features include
– asynchronous page mode read
– programmable wait states
– bus turnaround cycles
– output enable and write enable delays
LPC3220_30_40_50 All information provided in this document is subject to legal disclaimers. © NXP B.V. 2011. All rights reserved.
Product data sheet Rev. 2 — 20 October 2011 30 of 79
NXP Semiconductors LPC3220/30/40/50
16/32-bit ARM microcontrollers
– extended wait
• Power-saving modes dynamically control EMC_CKE[1:0] and EMC_CLK.
• Dynamic memory self-refresh mode supported by software.
• Controller supports 2 k, 4 k, and 8 k row address synchronous memory parts. That is,
typical 512 MB, 256 MB, 128 MB, and 16 MB parts, with 8, 16, or 32 data bits per
device.
• Two reset domains enable dynamic memory contents to be preserved over a soft
reset.
• This controller does not support synchronous static memory devices (burst mode
devices).
7.6 AHB master peripherals
The LPC3220/30/40/50 implements four AHB master peripherals, which include a
General Purpose Direct Memory Access (GPDMA) controller, a 10/100 Ethernet Media
Access Controller (MAC), a Universal Serial Bus (USB) controller, and an LCD controller.
Each of these four peripherals contain an integral DMA controller optimized to support the
performance demands of the peripheral.
7.6.1 General Purpose DMA (GPDMA) controller
The GPDMA controller allows peripheral-to memory, memory-to-peripheral,
peripheral-to-peripheral, and memory-to-memory transactions. Each DMA stream
provides unidirectional serial DMA transfers for a single source and destination. For
example, a bidirectional port requires one stream for transmit and one for receive. The
source and destination areas can each be either a memory region or a peripheral, and
can be accessed through the same AHB master, or one area by each master. The DMA
controller supports the following peripheral device transfers.
• Secure Digital (SD) Memory interface
• High-speed UARTs
• I2S0 and I2S1 ports
• SPI1 and SPI2 interfaces
• SSP0 and SSP1 interfaces
• Memory
The DMA controls eight DMA channels with hardware prioritization. The DMA controller
interfaces to the system via two AHB bus masters, each with a full 32-bit data bus width.
DMA operations may be set up for 8-bit, 16-bit, and 32-bit data widths, and can be either
big-endian or little-endian. Incrementing or non-incrementing addressing for source and
destination are supported, as well as programmable DMA burst size. Scatter or gather
DMA is supported through the use of linked lists. This means that the source and
destination areas do not have to occupy contiguous areas of memory.
7.6.2 Ethernet MAC
The Ethernet block contains a full featured 10 Mbit/s or 100 Mbit/s Ethernet MAC
designed to provide optimized performance through the use of DMA hardware
acceleration. Features include a generous suite of control registers, half or full duplex
operation, flow control, control frames, hardware acceleration for transmit retry, receive
LPC3220_30_40_50 All information provided in this document is subject to legal disclaimers. © NXP B.V. 2011. All rights reserved.
Product data sheet Rev. 2 — 20 October 2011 31 of 79
NXP Semiconductors LPC3220/30/40/50
16/32-bit ARM microcontrollers
packet filtering and wake-up on LAN activity. Automatic frame transmission and reception
with scatter-gather DMA off-loads many operations from the CPU. The Ethernet DMA can
access off-chip memory via the EMC, as well as the IRAM. The Ethernet block interfaces
between an off-chip Ethernet PHY using the Media Independent Interface (MII) or
Reduced MII (RMII) protocol and the on-chip Media Independent Interface Management
(MIIM) serial bus.
7.6.2.1 Features
• Ethernet standards support:
– Supports 10 Mbit/s or 100 Mbit/s PHY devices including 10 Base-T, 100 Base-TX,
100 Base-FX, and 100 Base-T4.
– Fully compliant with IEEE standard 802.3.
– Fully compliant with 802.3x Full Duplex Flow Control and Half Duplex back
pressure.
– Flexible transmit and receive frame options.
– Virtual Local Area Network (VLAN) frame support.
• Memory management:
– Independent transmit and receive buffers memory mapped to SRAM.
– DMA managers with scatter/gather DMA and arrays of frame descriptors.
– Memory traffic optimized by buffering and pre-fetching.
• Enhanced Ethernet features:
– Receive filtering.
– Multicast and broadcast frame support for both transmit and receive.
– Optional automatic Frame Check Sequence (FCS) insertion with Circular
Redundancy Check (CRC) for transmit.
– Selectable automatic transmit frame padding.
– Over-length frame support for both transmit and receive allows any length frames.
– Promiscuous receive mode.
– Automatic collision back-off and frame retransmission.
– Includes power management by clock switching. Wake-on-LAN power
management support allows system wake-up using the receive filters or a magic
frame detection filter.
• Physical interface
– Attachment of external PHY chip through standard MII or RMII interface.
– PHY register access is available via the MIIM interface.
7.6.3 USB interface
The LPC3220/30/40/50 supports USB in either device, host, or OTG configuration.
7.6.3.1 USB device controller
The USB device controller enables 12 Mbit/s data exchange with a USB host controller. It
consists of register interface, serial interface engine, endpoint buffer memory and DMA
controller. The serial interface engine decodes the USB data stream and writes data to the
appropriate end point buffer memory. The status of a completed USB transfer or error
LPC3220_30_40_50 All information provided in this document is subject to legal disclaimers. © NXP B.V. 2011. All rights reserved.
Product data sheet Rev. 2 — 20 October 2011 32 of 79
NXP Semiconductors LPC3220/30/40/50
16/32-bit ARM microcontrollers
condition is indicated via status registers. An interrupt is also generated if enabled. The
DMA controller when enabled transfers data between the endpoint buffer and the USB
RAM.
Features
• Fully compliant with USB 2.0 full-speed specification.
• Supports 32 physical (16 logical) endpoints.
• Supports control, bulk, interrupt and isochronous endpoints.
• Scalable realization of endpoints at run time.
• Endpoint maximum packet size selection (up to USB maximum specification) by
software at run time.
• RAM message buffer size based on endpoint realization and maximum packet size.
• Supports bus-powered capability with low suspend current.
• Supports DMA transfer on all non-control endpoints.
• One duplex DMA channel serves all endpoints.
• Allows dynamic switching between CPU controlled and DMA modes.
• Double buffer implementation for bulk and isochronous endpoints.
7.6.3.2 USB host controller
The host controller enables data exchange with various USB devices attached to the bus.
It consists of register interface, serial interface engine and DMA controller. The register
interface complies to the OHCI specification.
Features
• OHCI compliant.
• OHCI specifies the operation and interface of the USB host controller and software
driver.
• The host controller has four USB states visible to the software driver:
– USBOperational: Process lists and generate SOF tokens.
– USBReset: Forces reset signaling on the bus, SOF disabled.
– USBSuspend: Monitor USB for wake-up activity.
– USBResume: Forces resume signaling on the bus.
• HCCA register points to interrupt and isochronous descriptors list.
• ControlHeadED and BulkHeadED registers point to control and bulk descriptors list.
7.6.3.3 USB OTG controller
USB OTG (On-The-Go) is a supplement to the USB 2.0 specification that augments the
capability of existing mobile devices and USB peripherals by adding host functionality for
connection to USB peripherals.
Features
• Fully compliant with On-The-Go supplement to the USB Specification 2.0 Revision
1.0.
LPC3220_30_40_50 All information provided in this document is subject to legal disclaimers. © NXP B.V. 2011. All rights reserved.
Product data sheet Rev. 2 — 20 October 2011 33 of 79
NXP Semiconductors LPC3220/30/40/50
16/32-bit ARM microcontrollers
• Supports Host Negotiation Protocol (HNP) and Session Request Protocol (SRP) for
dual-role devices under software control. HNP is partially implemented in hardware.
• Provides programmable timers required for HNP and SRP.
• Supports slave mode operation through AHB slave interface.
• Supports the OTG ATX from NXP (ISP 1302) or any external CEA-2011OTG
specification compliant ATX.
7.6.4 LCD controller
The LCD controller provides all of the necessary control signals to interface directly to a
variety of color and monochrome LCD panels. Both STN (single and dual panel) and TFT
panels can be operated. The display resolution is selectable and can be up to 1024 768
pixels. Several color modes are provided, up to a 24-bit true-color non-palettized mode.
An on-chip 512-byte color palette allows reducing bus utilization (i.e. memory size of the
displayed data) while still supporting a large number of colors.
The LCD interface includes its own DMA controller to allow it to operate independently of
the CPU and other system functions. A built-in FIFO acts as a buffer for display data,
providing flexibility for system timing. Hardware cursor support can further reduce the
amount of CPU time needed to operate the display.
7.6.4.1 Features
• AHB bus master interface to access frame buffer.
• Setup and control via a separate AHB slave interface.
• Dual 16-deep programmable 64-bit wide FIFOs for buffering incoming display data.
• Supports single and dual-panel monochrome Super Twisted Nematic (STN) displays
with 4-bit or 8-bit interfaces.
• Supports single and dual-panel color STN displays.
• Supports Thin Film Transistor (TFT) color displays.
• Programmable display resolution including, but not limited to: 320 200, 320 240,
640 200, 640 240, 640 480, 800 600, and 1024 768.
• Hardware cursor support for single-panel displays.
• 15 gray-level monochrome, 3375 color STN, and 32 k color palettized TFT support.
• 1, 2, or 4 bits-per-pixel (bpp) palettized displays for monochrome STN.
• 1, 2, 4, or 8 bpp palettized color displays for color STN and TFT.
• 16 bpp true-color non-palettized, for color STN and TFT.
• 24 bpp true-color non-palettized, for color TFT.
• Programmable timing for different display panels.
• 256 entry, 16-bit palette RAM, arranged as a 128 32 bit RAM.
• Frame, line, and pixel clock signals.
• AC bias signal for STN, data enable signal for TFT panels.
• Supports little and big-endian, and Windows CE data formats.
• LCD panel clock may be generated from the peripheral clock or from a clock input pin.
LPC3220_30_40_50 All information provided in this document is subject to legal disclaimers. © NXP B.V. 2011. All rights reserved.
Product data sheet Rev. 2 — 20 October 2011 34 of 79
NXP Semiconductors LPC3220/30/40/50
16/32-bit ARM microcontrollers
7.7 System functions
To enhance the performance of the LPC3220/30/40/50 incorporates the following system
functions, an Interrupt Controller (INTC), a watchdog timer, a millisecond timer, and
several power control features. These functions are described in the following sections
7.7.1 Interrupt controller
The interrupt controller is comprised of three basic interrupt controller blocks, supporting a
total of 73 interrupt sources. Each interrupt source can be individually enabled/disabled
and configured for high or low level triggering, or rising or falling edge triggering. Each
interrupt may also be steered to either the FIQ or IRQ input of the ARM9. Raw interrupt
status and masked interrupt status registers allow versatile condition evaluation. In
addition to peripheral functions, each of the six general purpose input/output pins and
12 of the 22 general purpose input pins are connected directly to the interrupt controller.
7.7.2 Watchdog timer
The watchdog timer block is clocked by the main peripheral clock, which clocks a 32-bit
counter. A match register is compared to the Timer. When configured for watchdog
functionality, a match drives the match output low. The match output is gated with an
enable signal that gives the opportunity to generate two type of reset signal: one that only
resets chip internally, and another that goes through a programmable pulse generator
before it goes to the external pin RESOUT and to the internal chip reset.
7.7.2.1 Features
• Programmable 32-bit timer.
• Internally resets the device if not periodically reloaded.
• Flag to indicate that a watchdog reset has occurred.
• Programmable watchdog pulse output on RESOUT pin.
• Can be used as a standard timer if watchdog is not used.
• Pause control to stop counting when core is in debug state.
7.7.3 Millisecond timer
The millisecond timer is clocked by 32 kHz RTC clock, so a prescaler is not needed to
obtain a lower count rate.
The millisecond timer includes three match registers that are compared to the
Timer/Counter value. A match can generate an interrupt and the cause the Timer/Counter
either continue to run, stop, or be reset.
7.7.3.1 Features
• 32-bit Timer/Counter, running from the 32 kHz RTC clock.
• Counter or Timer operation.
• Three 32-bit match registers that allow:
– Continuous operation with optional interrupt generation on match.
– Stop timer on match with optional interrupt generation.
– Reset timer on match with optional interrupt generation.
• Pause control to stop counting when core is in debug state.
LPC3220_30_40_50 All information provided in this document is subject to legal disclaimers. © NXP B.V. 2011. All rights reserved.
Product data sheet Rev. 2 — 20 October 2011 35 of 79
NXP Semiconductors LPC3220/30/40/50
16/32-bit ARM microcontrollers
7.7.4 Clocking and power control features
7.7.4.1 Clocking
Clocking in the LPC3220/30/40/50 is designed to be versatile, so that system and
peripheral requirements may be met, while allowing optimization of power consumption.
Clocks to most functions may be turned off if not needed and some peripherals do this
automatically.
The LPC3220/30/40/50 supports three operational modes, two of which are specifically
designed to reduce power consumption. The modes are: Run mode, Direct run mode, and
Stop mode.These three operational modes give control over processing speed and power
consumption. In addition, clock rates to different functional blocks may be changed by
switching clock sources, changing PLL values, or altering clock divider configurations.
This allows a trade-off of power versus processing speed based on application
requirements.
7.7.4.2 Crystal oscillator
The main oscillator is the basis for the clocks most chip functions use by default.
Optionally, many functions can be clocked instead by the output of a PLL (with a fixed
397x rate multiplication) which runs from the RTC oscillator. In this mode, the main
oscillator may be turned off unless the USB interface is enabled. If a SYSCLK frequency
other than 13 MHz is required in the application, or if the USB block is not used, the main
oscillator may be used with a frequency of between 1 MHz and 20 MHz.
7.7.4.3 PLLs
The LPC3220/30/40/50 includes three PLLs: The 397x PLL allows boosting the RTC
frequency to 13.008896 MHz for use as the primary system clock. The USB PLL provides
the 48 MHz clock required by the USB block, and the HCLK PLL provides the basis for the
CPU clock, the AHB bus clock, and the main peripheral clock.
The 397x PLL multiplies the 32768 Hz RTC clock by 397 to obtain a 13.008896 MHz
clock. The 397x PLL is designed for low power operation and low jitter. This PLL requires
an external RC loop filter for proper operation.
The HCLK PLL accepts an input clock from either the main oscillator or the output of the
397x PLL. The USB PLL only accepts an input clock from the main oscillator.The USB
input clock runs through a divide-by-N pre-divider before entering the USB PLL.
The input to the HCLK and USB PLLs may initially be divided down by a pre-divider value
‘N’, which may have the values 1, 2, 3, or 4. This pre-divider can allow a greater number of
possibilities for the output frequency. Following the PLL input divider is the PLL multiplier.
This can multiply the pre-divider output by a value ‘M’, in the range of 1 through 256. The
resulting frequency must be in the range of 156 MHz to 320 MHz. The multiplier works by
dividing the output of a Current Controlled Oscillator (CCO) by the value of M, then using
a phase detector to compare the divided CCO output to the pre-divider output. The error
value is used to adjust the CCO frequency.
At the PLL output, there is a post-divider that can be used to bring the CCO frequency
down to the desired PLL output frequency. The post-divider value can divide the CCO
output by 1, 2, 4, 8, or 16. The post-divider can also be bypassed, allowing the PLL CCO
LPC3220_30_40_50 All information provided in this document is subject to legal disclaimers. © NXP B.V. 2011. All rights reserved.
Product data sheet Rev. 2 — 20 October 2011 36 of 79
NXP Semiconductors LPC3220/30/40/50
16/32-bit ARM microcontrollers
output to be used directly. The maximum PLL output frequency supported by the CPU is
266 MHz. The only output frequency supported by the USB PLL is 48 MHz, and the clock
has strict requirements for nominal frequency (500 ppm) and jitter (500 ps).
7.7.4.4 Power control modes
The LPC3220/30/40/50 supports three operational modes, two of which are specifically
designed to reduce power consumption. The modes are: Run mode, Direct Run mode,
and Stop mode.
Run mode is the normal operating mode for applications that require the CPU, AHB bus,
or any peripheral function other than the USB block to run faster than the main oscillator
frequency. In Run mode, the CPU can run at up to 266 MHz and the AHB bus can run at
up to 133 MHz.
Direct Run mode allows reducing the CPU and AHB bus rates in order to save power.
Direct Run mode can also be the normal operating mode for applications that do not
require the CPU, AHB bus, or any peripheral function other than the USB block to run
faster than the main oscillator frequency. Direct Run mode is the default mode following
chip reset.
Stop mode causes all CPU and AHB operation to cease, and stops clocks to peripherals
other than the USB block.
7.7.4.5 Reset
Reset is accomplished by an active LOW signal on the RESET input pin. A reset pulse
with a minimum width of 10 main oscillator clocks after the oscillator is stable is required to
guarantee a valid chip reset. At power-up, 10 milliseconds should be allowed for the
oscillator to start up and stabilize after VDD reaches operational voltage. An internal reset
with a minimum duration of 10 clock pulses will also be applied if the watchdog timer
generates an internal device reset.
The RESET pin is located in the RTC power domain. This means that the RTC power
must be present for an external reset to have any effect. The RTC power domain
nominally runs from 1.2 V, but the RESET pin can be driven as high as 1.95 V.
7.8 Communication peripheral interfaces
In addition to the Ethernet MAC and USB interfaces there are many more serial
communication peripheral interfaces available on the LPC3220/30/40/50. Here is a list of
the serial communication interfaces:
• Seven UARTs; four standard UARTs and three high-speed UARTs
• Two SPI serial I/O controllers
• Two SSP serial I/O controllers
• Two I2C serial I/O controllers
• Two I2S audio controllers
A short functional description of each of these peripherals is provided in the following
sections.
LPC3220_30_40_50 All information provided in this document is subject to legal disclaimers. © NXP B.V. 2011. All rights reserved.
Product data sheet Rev. 2 — 20 October 2011 37 of 79
NXP Semiconductors LPC3220/30/40/50
16/32-bit ARM microcontrollers
7.8.1 UARTs
The LPC3220/30/40/50 contains seven UARTs. Four are standard UARTs, and three are
high-speed UARTs.
7.8.1.1 Standard UARTs
The four standard UARTs are compatible with the INS16Cx50. These UARTs support
rates up to 460800 bit/s from a 13 MHz peripheral clock.
Features
• Each standard UART has 64 byte Receive and Transmit FIFOs.
• Receiver FIFO trigger points at 16, 32, 48, and 60 Bytes.
• Transmitter FIFO trigger points at 0, 4, 8, and 16 Bytes.
• Register locations conform to the “550” industry standard.
• Each standard UART has a fractional rate pre-divider and an internal baud rate
generator.
• The standard UARTs support three clocking modes: on, off, and auto-clock. The
auto-clock mode shuts off the clock to the UART when it is idle.
• UART 6 includes an IrDA mode to support infrared communication.
• The standard UARTs are designed to support data rates of (2400, 4800, 9600,
19200, 38400, 57600, 115200, 230400, 460800) bit/s.
• Each UART includes an internal loopback mode.
7.8.1.2 High-speed UARTs
The three high-speed UARTs are designed to support rates up to 921600 bit/s from a
13 MHz peripheral clock for on-board communication in low noise conditions. This is
accomplished by changing the over sampling from 16 to 14 and altering the rate
generation logic.
Features
• Each high-speed UART has 64-byte Receive and Transmit FIFOs.
• Receiver FIFO trigger points at 1, 4, 8, 16, 32, and 48 B.
• Transmitter FIFO trigger points at 0, 4, and 8 B.
• Each high-speed UART has an internal baud rate generator.
• The high-speed UARTs are designed to support data rates of (2400, 4800, 9600,
19200, 38400, 57600, 115200, 230400, 460800, 921600) bit/s.
• The three high speed UARTs only support (8N1) 8-bit data word length, 1-stop bit, no
parity, and no flow control as a the communications protocol.
• Each UART includes an internal loopback mode.
7.8.2 SPI serial I/O controller
The LPC3220/30/40/50 has two Serial Peripheral Interfaces (SPI). The SPI is a 3-wire
serial interface that is able to interface with a large range of serial peripheral or memory
devices (SPI mode 0 to 3 compatible slave devices).
LPC3220_30_40_50 All information provided in this document is subject to legal disclaimers. © NXP B.V. 2011. All rights reserved.
Product data sheet Rev. 2 — 20 October 2011 38 of 79
NXP Semiconductors LPC3220/30/40/50
16/32-bit ARM microcontrollers
Only a single master and a single slave can communicate on the interface during a given
data transfer. During a data transfer the master always sends a byte of data to the slave,
and the slave always sends a byte of data to the master. The SPI implementation on the
LPC3220/30/40/50 does not support operation as a slave.
7.8.2.1 Features
• Supports slaves compatible with SPI modes 0 to 3.
• Half duplex synchronous transfers.
• DMA support for data transmit and receive.
• 1-bit to 16-bit word length.
• Choice of LSB or MSB first data transmission.
• 64 16-bit input or output FIFO.
• Bit rates up to 52 Mbit/s.
• Busy input function.
• DMA time out interrupt to allow detection of end of reception when using DMA.
• Timed interrupt to facilitate emptying the FIFO at the end of a transmission.
• SPI clock and data pins may be used as general purpose pins if the SPI is not used.
• Slave selects can be supported using GPO or GPIO pins
7.8.3 SSP serial I/O controller
The LPC3220/30/40/50 contains two SSP controllers. The SSP controller is capable of
operation on a SPI, 4-wire SSI, or Microwire bus. It can interact with multiple masters and
slaves on the bus. Only a single master and a single slave can communicate on the bus
during a given data transfer. The SSP supports full duplex transfers, with frames of 4 bits
to 16 bits of data flowing from the master to the slave and from the slave to the master. In
practice, often only one of these data flows carries meaningful data.
7.8.3.1 Features
• Compatible with Motorola SPI, 4-wire TI SSI, and National Semiconductor Microwire
buses
• Synchronous serial communication
• Master or slave operation
• 8-frame FIFOs for both transmit and receive
• 4-bit to 16-bit frame
• Maximum SPI bus data bit rate of 1⁄2 (Master mode) and 1⁄2 (Slave mode) of the input
clock rate
• DMA transfers supported by GPDMA
7.8.4 I2C-bus serial I/O controller
There are two I2C-bus interfaces in the LPC32x0 family of controllers. These I2C blocks
can be configured as a master, multi-master or slave supporting up to 400 kHz. The I2C
blocks also support 7 or 10 bit addressing. Each has a four word FIFO for both transmit
and receive. An interrupt signal is available from each block.
LPC3220_30_40_50 All information provided in this document is subject to legal disclaimers. © NXP B.V. 2011. All rights reserved.
Product data sheet Rev. 2 — 20 October 2011 39 of 79
NXP Semiconductors LPC3220/30/40/50
16/32-bit ARM microcontrollers
There is a separate slave transmit FIFO. The slave transmit FIFO (TXS) and its level are
only available when the controller is configured as a Master/Slave device and is operating
in a multi-master environment. Separate TX FIFOs are needed in a multi-master because
a controller might have a message queued for transmission when an external master
addresses it to be come a slave-transmitter, a second source of data is needed.
Note that the I2C clock must be enabled in the I2CCLK_CTRL register before using the
I2C. The I2C clock can be disabled between communications, if used as a single master
I2C-bus interface, software has full control of when I2C communication is taking place on
the bus.
7.8.4.1 Features
• The two I2C-bus blocks are standard I2C-bus compliant interfaces that may be used in
Single-master, Multi-master or Slave modes.
• Programmable clock to allow adjustment of I2C-bus transfer rates.
• Bidirectional data transfer.
• Serial clock synchronization allows devices with different bit rates to communicate via
one serial bus.
• Serial clock synchronization can be used as a handshake mechanism to suspend and
resume serial transfer.
7.8.5 I2S-bus audio controller
The I2S-bus provides a standard communication interface for digital audio applications
The I2S-bus specification defines a 3-wire serial bus using one data line, one clock line,
and one word select signal. Each I2S connection can act as a master or a slave. The
master connection determines the frequency of the clock line and all other slaves are
driven by this clock source. The two I2S-bus interfaces on the LPC3220/30/40/50 provides
a separate transmit and receive channel, providing a total of two transmit channels and
two receive channels. Each I2S channel supports monaural or stereo formatted data.
7.8.5.1 Features
• The interface has separate input/output channels each of which can operate in master
or slave mode.
• Capable of handling 8-bit, 16-bit, and 32-bit word sizes.
• Mono and stereo audio data supported.
• Supports standard sampling frequencies (8 kHz, 11.025 kHz, 16 kHz, 22.05 kHz,
32 kHz, 44.1 kHz, 48 kHz, 96 kHz).
• Word select period can be configured in master mode (separately for I2S input and
output).
• Two eight-word FIFO data buffers are provided, one for transmit and one for receive.
• Generates interrupt requests when buffer levels cross a programmable boundary.
• Two DMA requests, controlled by programmable buffer levels. These are connected
to the GPDMA block.
• Controls include reset, stop, and mute options separately for I2S input and I2S output.
LPC3220_30_40_50 All information provided in this document is subject to legal disclaimers. © NXP B.V. 2011. All rights reserved.
Product data sheet Rev. 2 — 20 October 2011 40 of 79
NXP Semiconductors LPC3220/30/40/50
16/32-bit ARM microcontrollers
7.9 Other peripherals
In addition to the communication peripherals there are many general purpose peripherals
available in the LPC3220/30/40/50. Here is a list of the general purpose peripherals.
• GPI/O
• Keyboard scanner
• Touch screen controller and 10-bit Analog-to-Digital-Converter
• Real-time clock
• High-speed timer
• Four general purpose 32-bit timer/external event counters
• Two simple PWMs
• One motor control PWM
A short functional description of each of these peripherals is provided in the following
sections.
7.9.1 General purpose parallel I/O
Some device pins that are not dedicated to a specific peripheral function have been
designed to be general purpose inputs, outputs, or input/outputs. Also, some pins may be
configured either as a specific peripheral function or a general purpose input, output, or
input/output. A total of 51 pins can potentially be used as general purpose input/outputs,
24 as general purpose outputs, and 22 as general purpose inputs.
GPIO pins may be dynamically configured as inputs or outputs. Separate registers allow
setting or clearing any number of GPIO and GPO outputs controlled by that register
simultaneously. The value of the output register for standard GPIOs and GPO pins may
be read back, as well as the current actual state of the port pins.
In addition to GPIO pins on port 0, port 1, and port 2, there are 22 GPI, 24 GPO, and
six GPIO pins. When the SDRAM bus is configured for 16 data bits, 13 of the remaining
SDRAM data pins may be used as GPIOs.
7.9.1.1 Features
• Bit-level set and clear registers allow a single instruction set or clear of any number of
bits in one port.
• A single register selects direction for pins that support both input and output modes.
• Direction control of individual bits.
• For input/output pins, both the programmed output state and the actual pin state can
be read.
• There are a total of 12 general purpose inputs, 24 general purpose outputs, and six
general purpose input/outputs.
• Additionally, 13 SDRAM data lines may be used as GPIOs if a 16-bit SDRAM
interface is used (rather than a 32-bit interface).
LPC3220_30_40_50 All information provided in this document is subject to legal disclaimers. © NXP B.V. 2011. All rights reserved.
Product data sheet Rev. 2 — 20 October 2011 41 of 79
NXP Semiconductors LPC3220/30/40/50
16/32-bit ARM microcontrollers
7.9.2 Keyboard scanner
The keyboard scanner function can automatically scan a keyboard of up to 64 keys in an
8 8 matrix. In operation, the keyboard scanner’s internal state machine will normally be
in an idle state, with all KEY_ROWn pins set high, waiting for a change in the column
inputs to indicate that one or more keys have been pressed.
When a keypress is detected, the matrix is scanned by setting one output pin high at a
time and reading the column inputs. After de-bouncing, the keypad state is stored and an
interrupt is generated. The keypad is then continuously scanned waiting for ‘extra key
pressed’ or ‘key released’. Any new keypad state is scanned and stored into the matrix
registers followed by a new interrupt request to the interrupt controller. It is possible to
detect and separate up to 64 multiple keys pressed.
7.9.2.1 Features
• Supports up to 64 keys in 8 8 matrix.
• Programmable de-bounce period.
• A key press can wake up the CPU from Stop mode.
7.9.3 Touch screen controller and 10-bit ADC
The LPC3220/30/40/50 microcontrollers includes Touch Screen Controller (TSC)
hardware, which automatically measures and determines the X and Y coordinates where
a touch screen is pressed. In addition, the TSC can measure an analog input signal on the
AUX_IN pin.
Optionally, the TSC can operate as an Analog-to-Digital Converter (ADC). The ADC
supports three channels and uses 10-bit successive approximation to produce results with
a resolution of 10 bits in 11 clock cycles.
The analog portion of the ADC has its own power supply to enhance the low noise
characteristics of the converter. This voltage is only supplied internally when the core has
voltage. However, the ADC block is not affected by any difference in ramp-up time for
VDD_AD and VDD_CORE voltage supplies.
7.9.3.1 Features
• Measurement range of 0 V to VDD_AD (nominally 3.3 V).
• Low-noise ADC.
• 10-bit resolution.
• Three input channels.
• Uses 32 kHz RTC clock or peripheral clock.
7.9.4 Real-Time Clock (RTC) and battery RAM
The RTC runs at 32768 Hz using a very low power oscillator. The RTC counts seconds
and can generate alarm interrupts that can wake up the device from Stop mode. The RTC
clock can also clock the 397x PLL, the Millisecond Timer, the ADC, the Keyboard Scanner
and the PWMs. The RTC up-counter value represents a number of seconds elapsed since
second 0, which is an application determined time. The RTC counter will reach maximum
value after about 136 years. The RTC down-counter is initiated with all ones.
LPC3220_30_40_50 All information provided in this document is subject to legal disclaimers. © NXP B.V. 2011. All rights reserved.
Product data sheet Rev. 2 — 20 October 2011 42 of 79
NXP Semiconductors LPC3220/30/40/50
16/32-bit ARM microcontrollers
Two 32-bit match registers are readable and writable by the processor. A match will result
in an interrupt provided that the interrupt is enabled. The ONSW output pin can also be
triggered by a match event and cause an external power supply to turn on all of the
operating voltages, as a way to startup after power has been removed.
The RTC block is implemented in a separate voltage domain. The block is supplied via a
separate supply pin from a battery or other power source.
The RTC block also contains 32 words (128 bytes) of very low voltage SRAM. This SRAM
is able to hold its contents down to the minimum RTC operating voltage.
7.9.4.1 Features
• Measures the passage of time in seconds.
• 32-bit up and down seconds counters.
• Ultra-low power design to support battery powered systems.
• Dedicated 32 kHz oscillator.
• An output pin is included to assist in waking up when the chip has had power removed
to all functions except the RTC.
• Two 32-bit match registers with interrupt option.
• 32 words (128 bytes) of very low voltage SRAM.
• The RTC and battery RAM power have an independent power domain and dedicated
supply pins, which can be powered from a battery or power supply.
Remark: The LPC3220/30/40/50 will run at voltages down to 0.9 V at frequencies below
14 MHz. However, the ARM core cannot access the RTC registers and battery RAM when
the core supply voltage is at 0.9 V and the RTC supply is at 1.2 V.
7.9.5 Enhanced 32-bit timers/external event counters
The LPC3220/30/40/50 includes six 32-bit Timer/Counters. The Timer/Counter is
designed to count cycles of the system derived clock or an externally-supplied clock. It
can optionally generate interrupts or perform other actions at specified timer values,
based on four match registers. The Timer/Counter also includes four capture inputs to trap
the timer value when an input signal transitions, optionally generating an interrupt.
7.9.5.1 Features
• A 32-bit Timer/Counter with a programmable 32-bit pre-scaler.
• Counter or Timer operation.
• Up to four 32-bit capture channels per timer, that can take a snapshot of the timer
value when an input signal transitions. A capture event may also optionally generate
an interrupt.
• Four 32-bit match registers that allow:
– continuous operation with optional interrupt generation on match
– stop timer on match with optional interrupt generation
– reset timer on match with optional interrupt generation
• Up to four external outputs corresponding to match registers, with the following
capabilities:
LPC3220_30_40_50 All information provided in this document is subject to legal disclaimers. © NXP B.V. 2011. All rights reserved.
Product data sheet Rev. 2 — 20 October 2011 43 of 79
NXP Semiconductors LPC3220/30/40/50
16/32-bit ARM microcontrollers
– set LOW on match
– set HIGH on match
– toggle on match
– do nothing on match
7.9.6 High-speed timer
The high-speed timer block is clocked by the main peripheral clock. The clock is first
divided down in a 16-bit programmable pre-scale counter which clocks a 32-bit
timer/counter.
The high-speed timer includes three match registers that are compared to the
timer/counter value. A match can generate an interrupt and cause the timer/counter to
either continue to run, stop, or be reset. The high-speed timer also includes two capture
registers that can take a snapshot of the timer/counter value when an input signal
transitions. A capture event may also generate an interrupt.
7.9.6.1 Features
• 32-bit timer/counter with programmable 16-bit pre-scaler.
• Counter or timer operation.
• Two 32-bit capture registers.
• Three 32-bit match registers that allow:
– Continuous operation with optional interrupt generation on match.
– Stop timer on match with optional interrupt generation.
– Reset timer on match with optional interrupt generation.
• Pause control to stop counting when core is in debug state.
7.9.7 Pulse Width Modulators (PWMs)
The LPC3220/30/40/50 provides two simple PWMs. They are clocked separately by either
the main peripheral clock or the 32 kHz RTC clock. Both PWMs have a duty cycle
programmable in 255 steps.
7.9.7.1 Features
• Clocked by the main peripheral clock or the 32 kHz RTC clock.
• Programmable 4-bit pre-scaler.
• Duty cycle programmable in 255 steps.
• Output frequency up to 50 kHz when using a 13 MHz peripheral clock.
7.9.8 Motor control pulse width modulator
The Motor Control PWM (MCPWM) provides a set of features for three-phase AC and DC
motor control applications in a single peripheral. The MCPWM can also be configured for
use in other generalized timing, counting, capture, and compare applications.
7.9.8.1 Features
• 32-bit timer
• 32-bit period register
LPC3220_30_40_50 All information provided in this document is subject to legal disclaimers. © NXP B.V. 2011. All rights reserved.
Product data sheet Rev. 2 — 20 October 2011 44 of 79
NXP Semiconductors LPC3220/30/40/50
16/32-bit ARM microcontrollers
• 32-bit pulse-width (match) register
• 10-bit dead-time register and an associated 10-bit dead-time counter
• 32-bit capture register
• Two PWM (match) outputs (pins MCOA0/1/2 and MCOB0/1/2) with opposite polarities
• Period interrupt, pulse-width interrupt, and capture interrupt
8. Basic architecture
The LPC3220/30/40/50 is a general purpose ARM926EJ-S 32-bit microprocessor with a
32 kB instruction cache and a 32 kB data cache. The microcontroller offers high
performance and very low power consumption. The ARM architecture is based on RISC
principles, which results in the instruction set and related decode mechanism being much
simpler than equivalent micro programmed CISCs. This simplicity results in a high
instruction throughput and impressive real-time interrupt response from a small and
cost-effective processor core.
The ARM926EJ-S core employs a 5-stage pipeline so processing and memory system
accesses can occur continuously. At any one point in time, several operations are in
progress: subsequent instruction fetch, next instruction decode, instruction execution,
memory access, and write-back. The combination of architectural enhancements gives
the ARM9 about 30 % better performance than an ARM7 running at the same clock rate:
• Approximately 1.3 clocks per instruction for the ARM926EJ-S compared to 1.9 clocks
per instruction for ARM7TDMI.
• Approximately 1.1 Dhrystone MIPS/MHz for the ARM926EJ-S compared to 0.9
Dhrystone MIPS/MHz for ARM7TDMI.
The ARM926EJ-S processor also employs an operational state known as Thumb, which
makes it ideally suited to high-volume applications with memory restrictions, or
applications where code density is an issue.
The key idea behind Thumb state is the use of a super-reduced instruction set.
Essentially, the ARM926EJ-S processor core has two instruction sets:
1. The standard 32-bit ARM set
2. The 16-bit Thumb set
The Thumb set’s smaller 16-bit instruction length allows it to approach twice the density of
standard ARM code while retaining many of ARM’s 32-bit performance advantage over a
traditional 16-bit processor using 16-bit registers. This is possible because Thumb code
operates using the same 32-bit register set as ARM code. Thumb code size is up to 65 %
smaller than ARM code size, and 160 % of the performance of an equivalent ARM
processor connected to a 16-bit memory system. Additionally, the ARM926EJ-S core
includes enhanced DSP instructions and multiplier, as well as an enhanced 32-bit MAC
block.
LPC3220_30_40_50 All information provided in this document is subject to legal disclaimers. © NXP B.V. 2011. All rights reserved.
Product data sheet Rev. 2 — 20 October 2011 45 of 79
NXP Semiconductors LPC3220/30/40/50
16/32-bit ARM microcontrollers
9. Limiting values
[1] The following applies to Table 7:
a) This product includes circuitry specifically designed for the protection of its internal devices from the damaging effects of excessive
static charge. Nonetheless, it is suggested that conventional precautions be taken to avoid applying greater than the rated
maximum.
b) Parameters are valid over operating temperature range unless otherwise specified. All voltages are with respect to VSS unless
otherwise noted.
[2] Core, PLL, oscillator, and RTC supplies; applies to pins VDD_CORE, VDD_COREFXD, VDD_OSC, VDD_PLL397, VDD_PLLHCLK,
VDD_PLLUSB, VDD_RTC, VDD_RTCCORE, and VDD_RTCOSC.
[3] I/O pad supply; applies to domains VDD_EMC.
[4] Applies to VDD_AD pins.
[5] Applies to pins in the following domains VDD_IOA, VDD_IOB, VDD_IOC, and VDD_IOD.
[6] Including voltage on outputs in 3-state mode.
[7] Based on package heat transfer, not device power consumption. Calculated package thermal resistance (ThetaJA): 35.766 C/W (with
JEDEC Test Board and 0 m/s airflow, 15 % accuracy).
[8] Human body model: equivalent to discharging a 100 pF capacitor through a 1.5 k series resistor.
[9] Charge device model per AEC-Q100-011.
Table 7. Limiting values
In accordance with the Absolute Maximum Rating System (IEC 60134).[1]
Symbol Parameter Conditions Notes Min Max Unit
VDD(1V2) supply voltage (1.2 V) [2] 0.5 +1.4 V
VDD(EMC) external memory controller
supply voltage
[3] 0.5 +4.6 V
VDDA(3V3) analog supply voltage (3.3 V) [4] 0.5 +4.6 V
VDD(IO) input/output supply voltage [5] 0.5 +4.6 V
VIA analog input voltage 0.5 +4.6 V
VI input voltage 1.8 V pins [6] 0.5 +2.4 V
3.3 V pins [6] 0.5 +4.6 V
IDD supply current per supply pin - 100 mA
ISS ground current per ground pin - 100 mA
Tstg storage temperature 65 +150 C
Ptot(pack) total power dissipation
(per package)
max. junction temp 125 C
max. ambient temp 85 C
[7]- 1.12 W
VESD electrostatic discharge voltage HBM [8] - 2500 V
CDM [9] - 1000 V
LPC3220_30_40_50 All information provided in this document is subject to legal disclaimers. © NXP B.V. 2011. All rights reserved.
Product data sheet Rev. 2 — 20 October 2011 46 of 79
NXP Semiconductors LPC3220/30/40/50
16/32-bit ARM microcontrollers
10. Static characteristics
Table 8. Static characteristics
Tamb = 40 C to +85 C, unless otherwise specified.
Symbol Parameter Conditions Min Typ[1] Max Unit
VDD(1V2) supply voltage (1.2 V) core supply voltage for
full performance;
266 MHz (see Figure 4);
VDD_CORE supply domain
[2] 1.31 1.35 1.39 V
core supply voltage for
normal performance;
208 MHz (see Figure 4);
VDD_CORE supply domain
[2] 1.1 1.2 1.39 V
core supply voltage for
reduced power;
up to 14 MHz CPU;
VDD_CORE supply domain
[2] 0.9 - 1.39 V
RTC supply voltage;
VDD_RTC supply domain
[3] 0.9 - 1.39 V
PLL and oscillator supply
voltage
[4] 1.1 1.2 1.39 V
VDD(EMC) external memory
controller supply voltage
in 1.8 V range [5] 1.7 1.8 1.95 V
in 2.5 V range [6] 2.3 2.5 2.7 V
in 3.3 V range [7] 2.7 3.3 3.6 V
VDD(IO) input/output supply
voltage
VDD_IOA, VDD_IOB, and
VDD_IOD supply domain
in 1.8 V range
1.7 1.8 1.95 V
in 3.3 V range 2.7 3.3 3.6 V
VDD_IOC supply domain
in 1.8 V range
1.7 1.8 1.95 V
in 3.3 V range 2.3 3.3 3.6 V
VDDA(3V3) analog supply voltage
(3.3 V)
applies to pins in VDD_AD
power domain
2.7 3.3 3.6 V
LPC3220_30_40_50 All information provided in this document is subject to legal disclaimers. © NXP B.V. 2011. All rights reserved.
Product data sheet Rev. 2 — 20 October 2011 47 of 79
NXP Semiconductors LPC3220/30/40/50
16/32-bit ARM microcontrollers
Power consumption in Run, direct Run, and Stop modes
IDD(run) Run mode supply
current
Tamb = 25 C;
code
while(1){}
executed from IRAM; all
peripherals enabled
I-cache/D-cache, MMU
enabled; CPU
clock = 208 MHz;
VDD_CORE = 1.2 V
- 150 - mA
I-cache/D-cache, MMU
enabled; CPU
clock = 266 MHz;
VDD_CORE = 1.35 V
- 218 - mA
I-cache/D-cache, MMU
disabled; CPU
clock = 208 MHz;
VDD_CORE = 1.2 V
- 78 - mA
I-cache/D-cache, MMU
disabled; CPU
clock = 266 MHz;
VDD_CORE = 1.35 V
- 111 - mA
IDD(drun) direct Run mode supply
current
Tamb = 25 C; CPU
clock = 13 MHz;
code
while(1){}
executed from IRAM; all
peripherals disabled
I-cache/D-cache, MMU
enabled;
VDD_CORE = 1.2 V
- 7.8 - mA
I-cache/D-cache, MMU
enabled;
VDD_CORE = 0.9 V
- 5.6 - mA
I-cache/D-cache, MMU
disabled;
VDD_CORE = 1.2 V
- 5 - mA
I-cache/D-cache, MMU
disabled;
VDD_CORE = 0.9 V
- 3.5 - mA
IDD(stop) Stop mode supply
current
Tamb = 25 C; CPU
clock stopped internally; all
peripherals disabled
VDD_CORE = 1.2 V - 400 - A
VDD_CORE = 0.9 V - 400 - A
Table 8. Static characteristics …continued
Tamb = 40 C to +85 C, unless otherwise specified.
Symbol Parameter Conditions Min Typ[1] Max Unit
LPC3220_30_40_50 All information provided in this document is subject to legal disclaimers. © NXP B.V. 2011. All rights reserved.
Product data sheet Rev. 2 — 20 October 2011 48 of 79
NXP Semiconductors LPC3220/30/40/50
16/32-bit ARM microcontrollers
IDD(RTC) RTC supply current normal operation;
VDD_RTC =
VDD_RTCCORE =
VDD_RTCOSC = 1.2 V;
Tamb = 25 C
[8]- 13 - A
RTC back up operation;
Rev “-” silicon
[9]- 30 - A
Rev “A” silicon [9]- 4 -
IDD supply current for HCLK; PLL output
frequency = 266 MHz;
VDD_PLLHCLK = 1.2 V
- 2 - mA
for USB; VDD_PLLUSB =
1.2 V
- 2 - mA
for ADC; interrupt driven
loop converting ADIN[2:0];
VDD_AD = 3.3 V
- 1 - mA
Input pins and I/O pins configured as input
VI input voltage [10][12]0 - VDD(IO) V
VIH HIGH-level
input voltage
1.8 V inputs 0.7 VDD(IO) - - V
3.3 V inputs 0.7 VDD(IO) - - V
VIL LOW-level
input voltage
1.8 V inputs - - 0.3 VDD(IO) V
3.3 V inputs - - 0.3 VDD(IO) V
Vhys hysteresis voltage 1.8 V inputs 0.1 VDD(IO) - - V
3.3 V inputs 0.1 VDD(IO) - - V
IIL LOW-level
input current
VI = 0 V; no pull-up - - 1 A
IIH HIGH-level
input current
VI = VDD(IO); no pull-down [10]- - 1 A
Ilatch I/O latch-up current (1.5VDD(IO)) < VI <
(1.5VDD(IO))
[10]- - 100 mA
Ipu pull-up current 1.8 V inputs with pull-up;
VI = 0 V
6 12 22 A
3.3 V inputs with pull-up;
VI = 0 V
25 50 80 A
Ipd pull-down current 1.8 V inputs with pull-down;
VI = VDD(IO)
5 12 22 A
3.3 V inputs with pull-down;
VI = VDD(IO)
25 50 85 A
II input current bus keeper inputs;
VI = VDD
- - 1 A
VI = 0.67 VDD - - 55 A
VI = 0.33 VDD - - 60 A
VI = 0 V - - 1 A
Ci input capacitance Excluding bonding
pad capacitance
- - 3.3 pF
Table 8. Static characteristics …continued
Tamb = 40 C to +85 C, unless otherwise specified.
Symbol Parameter Conditions Min Typ[1] Max Unit
LPC3220_30_40_50 All information provided in this document is subject to legal disclaimers. © NXP B.V. 2011. All rights reserved.
Product data sheet Rev. 2 — 20 October 2011 49 of 79
NXP Semiconductors LPC3220/30/40/50
16/32-bit ARM microcontrollers
Output pins and I/O pins configured as output
VO output voltage [10][11]
[12][13]
0 - VDD(IO) V
VOH HIGH-level output
voltage
1.8 V outputs; IOH = 1 mA [14] VDD(IO) 0.4 - - V
3.3 V outputs; IOH = 4 mA [14] VDD(IO) 0.4 - - V
VOL LOW-level
output voltage
1.8 V outputs; IOL = 4 mA [14]- - 0.4 V
3.3 V outputs; IOL = 4 mA [14]- - 0.4 V
IOH HIGH-level
output current
VDD(IO) = 1.8 V;
VOH = VDD(IO) 0.4 V
[10][14] 3.3 - - mA
VDD(IO) = 3.3 V;
VOH = VDD(IO) 0.4 V
6.5 - - mA
IOL LOW-level
output current
VDD(IO) = 1.8 V; VOL = 0.4 V [10][14] 1.5 - - mA
VDD(IO) = 3.3 V; VOL = 0.4 V 3 - - mA
IOZ OFF-state
output current
VO = 0 V; VO = VDD(IO);
no pull-up/down
[10]- - 1 A
IOHS HIGH-level short-circuit
output current
VDD(IO) = 1.8 V; VOH = 0 V [15]- - 66 mA
VDD(IO) = 3.3 V; VOH = 0 V - - 183 mA
IOLS LOW-level short-circuit
output current
VDD(IO) = 1.8 V; VOL =
VDD(IO)
[10][15]- - 34 mA
VDD(IO) = 3.3 V; VOL =
VDD(IO)
- - 105 mA
Zo output impedance VDD(IO) = 1.8 V 40 - 60
VDD(IO) = 3.3 V 40 - 60
EMC pins
VI input voltage [12]0 - VDD(EMC) V
VIH HIGH-level input voltage 1.8 V inputs 0.7 VDD(EMC) - - V
3.3 V inputs 0.7 VDD(EMC) - - V
VIL LOW-level input voltage 1.8 V inputs - - 0.3 VDD(EMC) V
3.3 V inputs - - 0.3 VDD(EMC) V
Vhys hysteresis voltage 1.8 V inputs 0.4 - 0.6 V
3.3 V inputs 0.55 - 0.85 V
IIL LOW-level
input current
VI = 0 V; no pull-up - - 0.3 A
IIH HIGH-level
input current
VI = VDD(EMC); no pull-down - - 0.3 A
Ilatch I/O latch-up current (1.5VDD(EMC)) < VI <
(1.5VDD(EMC))
- - 100 mA
Ipu pull-up current 1.8 V inputs with pull-up;
VI = 0
34 62 107 A
3.3 V inputs with pull-up;
VI = 0
97 169 271 A
Table 8. Static characteristics …continued
Tamb = 40 C to +85 C, unless otherwise specified.
Symbol Parameter Conditions Min Typ[1] Max Unit
LPC3220_30_40_50 All information provided in this document is subject to legal disclaimers. © NXP B.V. 2011. All rights reserved.
Product data sheet Rev. 2 — 20 October 2011 50 of 79
NXP Semiconductors LPC3220/30/40/50
16/32-bit ARM microcontrollers
Ipd pull-down current 1.8 V inputs with pull-down;
VI = VDD(EMC)
23 51 93 A
3.3 V inputs with pull-down;
VI = VDD(EMC)
73 155 266 A
Ci input capacitance Excluding bonding
pad capacitance
- - 2.1 pF
VO output voltage [11]
[12][13]
0 - VDD(EMC) V
VOH HIGH-level
output voltage
1.8 V outputs; IOH = 1 mA [14] VDD(EMC) 0.3 - - V
3.3 V outputs; IOH = 4 mA [14] VDD(EMC) 0.3 - - V
VOL LOW-level
output voltage
1.8 V outputs; IOL = 4 mA [14]- - 0.3 V
3.3 V outputs; IOL = 4 mA [14]- - 0.3 V
IOH HIGH-level
output current
VDD(EMC) = 1.8 V;
VOH = VDD(EMC) 0.4 V
[14] 6 - - mA
VDD(EMC) = 3.3 V;
VOH = VDD(EMC) 0.4 V
6 - - mA
IOL LOW-level
output current
VDD(EMC) = 1.8 V;
VOL = 0.4 V
[14]6 - - mA
VDD(EMC) = 3.3 V;
VOL = 0.4 V
6 - - mA
IOZ OFF-state
output current
VO = 0 V; VO = VDD(EMC);
no pull-up/down
- - 0.3 A
IOHS HIGH-level short-circuit
output current
VDD(EMC) = 1.8 V; VOH = 0 V [15]- - 49 mA
VDD(EMC) = 3.3 V; VOH = 0 V - - 81 mA
IOLS LOW-level short-circuit
output current
VDD(EMC) = 1.8 V;
VOL = VDD(EMC)
[14]- - 49 mA
VDD(EMC) = 3.3 V;
VOL = VDD(EMC)
- - 86 mA
Zo output impedance VDD(EMC) = 1.8 V 35 40 58
VDD(EMC) = 3.3 V 32 35 45
I2C pins
VI input voltage [10]
[12]
0 - 5.5 V
VIH HIGH-level
input voltage
1.8 V inputs 0.7 VDD(IO) - - V
3.3 V inputs 0.7 VDD(IO) - - V
VIL LOW-level
input voltage
1.8 V inputs - - 0.3 VDD(IO) V
3.3 V inputs - - 0.3 VDD(IO) V
IIL LOW-level
input current
VI = 0 V; no pull-up - - 10 A
IIH HIGH-level
input current
VI = VDD(IO); no pull-down [10]- - 10 A
Ilatch I/O latch-up current (1.5VDD(IO)) < VI <
(1.5VDD(IO))
[10]- - 100 mA
Table 8. Static characteristics …continued
Tamb = 40 C to +85 C, unless otherwise specified.
Symbol Parameter Conditions Min Typ[1] Max Unit
LPC3220_30_40_50 All information provided in this document is subject to legal disclaimers. © NXP B.V. 2011. All rights reserved.
Product data sheet Rev. 2 — 20 October 2011 51 of 79
NXP Semiconductors LPC3220/30/40/50
16/32-bit ARM microcontrollers
Ci input capacitance Excluding bonding
pad capacitance
- - 1.6 pF
VOL LOW-level
output voltage
1.8 V outputs; IOL = 4 mA [14]- - 0.4 V
3.3 V outputs; IOL = 4 mA [14]- - 0.4 V
IOL LOW-level
output current
VDD(IO) = 1.8 V; VOL = 0.4 V [10][14]3 - - mA
VDD(IO) = 3.3 V; VOL = 0.4 V 3 - - mA
IOZ OFF-state
output current
VO = 0 V; VO = VDD(IO);
no pull-up/down
[10]- - 10 A
IOLS LOW-level short-circuit
output current
VDD(IO) = 1.8 V; VOL =
VDD(IO)
[10][15]- - 40 mA
VDD(IO) = 3.3 V; VOL =
VDD(IO)
- - 40 mA
ONSW pin
VO output voltage [10][11]
[12][13]
0 - VDD(1V2) V
VOH HIGH-level
output voltage
1.2 V outputs; IOH = 1 mA [14] VDD(1V2) 0.4 - - V
VOL LOW-level
output voltage
1.2 V outputs; IOL = 4 mA [14]- - 0.4 V
IOH HIGH-level
output current
VOH = VDD(1V2) 0.4 V [10][14] 4 - - mA
IOL LOW-level
output current
VOL = 0.4 V [10][14]3 - - mA
IOZ OFF-state
output current
VO = 0 V; VO = VDD(1V2);
no pull-up/down
[10]- - 1.5 A
IOHS HIGH-level short-circuit
output current
VDD(1V2) = 1.8 V; VOH = 0 V [15]- - 135 mA
IOLS LOW-level short-circuit
output current
VOL = VDD(1V2) [10][15]- - 135 mA
Zo output impedance VDD(1V2) = 1.2 V 40 - 60
Oscillator input/output pins
Vi(xtal) crystal input voltage on pins RTCX_IN and
SYSX_IN
0.5 - +1.3 V
Vo(xtal) crystal output voltage on pins RTCX_OUT and
SYSX_OUT
0.5 - +1.3 V
RESET pin
VI input voltage [10]
[12]
0 - 1.95 V
VIH HIGH-level input voltage 1.2 V inputs 0.7 VDD(1V2) - - V
VIL LOW-level input voltage 1.2 V inputs - - 0.3 VDD(1V2) V
IIL LOW-level input current VI = 0 V; no pull-up - - 1 A
Table 8. Static characteristics …continued
Tamb = 40 C to +85 C, unless otherwise specified.
Symbol Parameter Conditions Min Typ[1] Max Unit
LPC3220_30_40_50 All information provided in this document is subject to legal disclaimers. © NXP B.V. 2011. All rights reserved.
Product data sheet Rev. 2 — 20 October 2011 52 of 79
NXP Semiconductors LPC3220/30/40/50
16/32-bit ARM microcontrollers
[1] Typical ratings are not guaranteed. The values listed are at room temperature (25 C), nominal supply voltages.
[2] Applies to VDD_CORE pins.
[3] Applies to pins VDD_RTC, VDD_RTCCORE, and VDD_RTCOSC.
[4] Applies to pins VDD_COREFXD, VDD_OSC, VDD_PLL397, VDD_PLLHCLK, and VDD_PLLUSB.
[5] Applies when using 1.8 V Mobile DDR or Mobile SDR SDRAM.
[6] Applies when using 2.5 V DDR memory.
[7] Applies when using 3.3 V SDR SDRAM and SRAM.
[8] Specifies current on combined VDD_RTCx during normal chip operation: VDD_RTC, VDD_CORE, VDD_OSC = 1.2 V and
VDD_CORE, VDD_IOx at typical voltage.
[9] Specifies current on combined VDD_RTCx during backup operation: VDD_RTC, VDD_CORE, VDD_OSC = 1.2 V and all other VDD_x
at 0 V.
[10] Referenced to the applicable VDD for the pin.
[11] Including voltage on outputs in 3-state mode.
[12] The applicable VDD voltage for the pin must be present.
[13] 3-state outputs go into 3-state mode when the applicable VDD voltage for the pin is grounded.
[14] Accounts for 100 mV voltage drop in all supply lines.
[15] Allowed as long as the current limit does not exceed the maximum current allowed by the device.
IIH HIGH-level input current VI = VDD; no pull-down [10]- - 1 A
IOZ OFF-state output current VO = 0 V; VO = VDD;
no pull-up/down
[10]- - 1 A
Ilatch I/O latch-up current (1.5VDD) < VI < (1.5VDD) [10]- - 100 mA
Table 8. Static characteristics …continued
Tamb = 40 C to +85 C, unless otherwise specified.
Symbol Parameter Conditions Min Typ[1] Max Unit
LPC3220_30_40_50 All information provided in this document is subject to legal disclaimers. © NXP B.V. 2011. All rights reserved.
Product data sheet Rev. 2 — 20 October 2011 53 of 79
NXP Semiconductors LPC3220/30/40/50
16/32-bit ARM microcontrollers
10.1 Minimum core voltage requirements
Figure 4 shows the minimum core supply voltage that should be applied for a given core
frequency on pin VDD_CORE to ensure stable operation of the LPC3220/30/40/50.
10.2 Power supply sequencing
The LPC32x0 has no power sequencing requirements, that is, VDD(1V2), VDD(EMC), VDD(IO),
and VDDA(3V3) can be switched on or off independent of each other. An internal circuit
ensures that the system correctly powers up in the absence of core power. During IO
power-up this circuit takes care that the system is powered in a defined mode. The same
is valid for core power-down.
10.3 Power consumption per peripheral
[1] All three Ethernet clocks are in enabled in the MAC_CLK_CTRL register (see LPC32x0 User manual).
Fig 4. Minimum required core supply voltage for different core frequencies
core frequency (MHz)
160 200 240 280
002aae872
1.0
1.2
1.4
0.8
VDD_CORE
(V)
Table 9. Power consumption per peripheral
Tamb = 25 C; CPU clock = 208 MHz; I-cache/D-cache, MMU disabled; VDD_CORE = 1.2 V;
VDD(IO) = 1.8 V; USB AHB, IRAM, and IROM clocks always on; all peripherals are at their default
state at reset. Peripheral clocks are disabled except for peripheral measured.
Peripheral IDD(run) / mA
High-speed UART (set to 115 200 Bd (8N1)) 0.3
I2C-bus 0.3
SSP 0.6
I2S 0.5
DMA 6.3
EMC 7.3
Multi-level NAND controller 1.4
Single-level NAND controller 0.3
LCD 5.6
Ethernet MAC[1] 2.9
LPC3220_30_40_50 All information provided in this document is subject to legal disclaimers. © NXP B.V. 2011. All rights reserved.
Product data sheet Rev. 2 — 20 October 2011 54 of 79
NXP Semiconductors LPC3220/30/40/50
16/32-bit ARM microcontrollers
10.4 Power consumption in Run mode
Power consumption is shown in Figure 5 for WinCE applications running under typical
conditions from SDRAM. MMU and I-cache/D-cache are enabled. The VFP is turned on
but not used. I2S-interface (channel 1), LCD, SLC NAND controller, I2C1-bus, SD card,
touchscreen ADC, and UART 3 are turned on. All other peripherals are turned off.
The AHB clock HCLK is identical to the core clock for frequencies up to 133 MHz, which is
the maximum allowed HCLK frequency. For higher core frequencies, the HCLK PLL
output must be divided by 2 to obtain an HCLK frequency lower than or equal to 133 MHz
resulting in correspondingly lower power consumption by the AHB peripherals.
Conditions: Tamb = 25 C; VDD_CORE = 1.2 V for core frequencies 208 MHz;
VDD_CORE = 1.35 V for core frequencies > 208 MHz; VDD(IO) = 1.8 V.
(1) WinCE running from SDRAM; playing wmv file at 20 frames/s, 32 kHz mono.
(2) WinCE running from SDRAM; playing mp3 file at 128 kbit/s, stereo.
(3) WinCE running from SDRAM; no application running.
Fig 5. Core current versus core frequency for WinCE applications
core frequency (MHz)
40 120 200 280
002aae762
80
40
120
160
IDD(run)
(mA)
0
(1)
(2)
(3)
HCLK = 133 MHz
HCLK = 72 MHz
VDD_CORE =
1.2 V
VDD_CORE =
1.35 V
LPC3220_30_40_50 All information provided in this document is subject to legal disclaimers. © NXP B.V. 2011. All rights reserved.
Product data sheet Rev. 2 — 20 October 2011 55 of 79
NXP Semiconductors LPC3220/30/40/50
16/32-bit ARM microcontrollers
10.5 ADC static characteristics
[1] Conditions: VSSA = 0 V (on pin VSS_AD); VDDA(3V3) = 3.3 V (on pin VDD_AD).
[2] The ADC is monotonic; there are no missing codes.
[3] The differential linearity error (ED) is the difference between the actual step width and the ideal step width. See Figure 6.
[4] The integral non-linearity (EL(adj)) is the peak difference between the center of the steps of the actual and the ideal transfer curve after
appropriate adjustment of gain and offset errors. See Figure 6.
[5] The offset error (EO) is the absolute difference between the straight line which fits the actual curve and the straight line which fits the
ideal curve. See Figure 6.
[6] The gain error (EG) is the relative difference in percent between the straight line fitting the actual transfer curve after removing offset
error, and the straight line which fits the ideal transfer curve. See Figure 6.
[7] The absolute error (ET) is the maximum difference between the center of the steps of the actual transfer curve of the non-calibrated
ADC and the ideal transfer curve. See Figure 6.
Table 10. ADC static characteristics
VDDA(3V3) = 3.3 V; Tamb = 25C unless otherwise specified; ADC clock frequency 4.5 MHz.
Symbol Parameter Conditions Min Typ Max Unit
VIA analog input voltage 0 - VDDA(3V3) V
Cia analog input capacitance - - 1 pF
ED differential linearity error [1][2][3] - 0.5 1 LSB
EL(adj) integral non-linearity [1][4] - 0.6 1 LSB
EO offset error [1][5] - 1 3 LSB
EG gain error [1][6] - 0.3 0.6 %
ET absolute error [1][7] - 4 LSB
Rvsi voltage source interface resistance - - 40 k
LPC3220_30_40_50 All information provided in this document is subject to legal disclaimers. © NXP B.V. 2011. All rights reserved.
Product data sheet Rev. 2 — 20 October 2011 56 of 79
NXP Semiconductors LPC3220/30/40/50
16/32-bit ARM microcontrollers
(1) Example of an actual transfer curve.
(2) The ideal transfer curve.
(3) Differential linearity error (ED).
(4) Integral non-linearity (EL(adj)).
(5) Center of a step of the actual transfer curve.
Fig 6. ADC characteristics
002aae434
1023
1022
1021
1020
1019
(2)
(1)
1 2 3 4 5 6 7 1018 1019 1020 1021 1022 1023 1024
7
6
5
4
3
2
1
0
1018
(5)
(4)
(3)
1 LSB
(ideal)
code
out
VDDA(3V3) − VSSA
1024
offset
error
EO
gain
error
EG
offset error
EO
VIA (LSBideal)
1 LSB =
LPC3220_30_40_50 All information provided in this document is subject to legal disclaimers. © NXP B.V. 2011. All rights reserved.
Product data sheet Rev. 2 — 20 October 2011 57 of 79
NXP Semiconductors LPC3220/30/40/50
16/32-bit ARM microcontrollers
11. Dynamic characteristics
11.1 Clocking and I/O port pins
[1] Parameters are valid over operating temperature range unless otherwise specified.
[2] After supply voltages are stable
[3] Supplied by an external crystal.
11.2 Static memory controller
Table 11. Dynamic characteristics
Tamb = 40 C to +85 C, unless otherwise specified.[1]
Symbol Parameter Conditions Min Typ Max Unit
Reset
tw(RESET)ext external RESET pulse width [2] 10 - - ms
External clock
fext external clock frequency [3]1 13 20 MHz
Port pins
tr rise time - 5 - ns
tf fall time - 5 - ns
Table 12. Dynamic characteristics: static external memory interface
CL = 25 pF, Tamb = 20C, VDD(EMC) = 1.8 V, 2.5 V, or 3.3 V.
Symbol Parameter Notes Min Typ Max Unit
Common to read and write cycles
TCLCL clock cycle time [1] 7.5 9.6 - ns
tCSLAV CS LOW to address valid time - 0 - ns
Read cycle parameters
tOELAV OE LOW to address valid time [2]- 0 WAITOEN TCLCL - ns
tBLSLAV BLS LOW to address valid time [2]- 0 WAITOEN TCLCL - ns
tCSLOEL CS LOW to OE LOW time - 0 + WAITOEN TCLCL - ns
tCSLBLSL CS LOW to BLS LOW time [2] - 0 + WAITOEN TCLCL - ns
tOELOEH OE LOW to OE HIGH time [2][3]- (WAITRD WAITOEN + 1) TCLCL - ns
tBLSLBLSH BLS LOW to BLS HIGH time [2][3]- (WAITRD WAITOEN + 1) TCLCL - ns
tsu(DQ) data input/output set-up time [6]- 8.4 - ns
th(DQ) data input/output hold time [6]- 0 - ns
tCSHOEH CS HIGH to OE HIGH time - 0 - ns
tCSHBLSH CS HIGH to BLS HIGH time - 0 - ns
tOEHANV OE HIGH to address invalid time - 1 TCLCL - ns
tBLSHANV BLS HIGH to address invalid time - 1 TCLCL - ns
Write cycle parameters
tCSLDV CS LOW to data valid time - 0 - ns
tCSLWEL CS LOW to WE LOW time [4]- (WAITWEN+1) TCLCL - ns
tCSLBLSL CS LOW to BLS LOW time [4]- (WAITWEN+ 1) TCLCL - ns
tWELDV WE LOW to data valid time [4]- 0 (WAITWEN + 1) TCLCL - ns
LPC3220_30_40_50 All information provided in this document is subject to legal disclaimers. © NXP B.V. 2011. All rights reserved.
Product data sheet Rev. 2 — 20 October 2011 58 of 79
NXP Semiconductors LPC3220/30/40/50
16/32-bit ARM microcontrollers
[1] TCLCL = 1/HCLK
[2] Refer to the LPC32x0 User manual EMCStaticWaitOen0-3 register for the programming of WAITOEN value.
[3] Refer to the LPC32x0 User manual EMCStaticWaitRd0-3 register for the programming of WAITRD value.
[4] Refer to the LPC32x0 User manual EMCStaticWaitWen0-3 register for the programming of WAITWEN value.
[5] Refer to the LPC32x0 User manual EMCStaticWaitWr0-3 register for the programming of WAITWR value.
[6] Earliest of CS HIGH, OE HIGH, address change to data invalid.
tWELWEH WE LOW to WE HIGH time [4][5]- (WAITWR WAITWEN + 1) TCLCL - ns
tBLSLBLSH BLS LOW to BLS HIGH time [4][5]- (WAITWR WAITWEN + 1) TCLCL - ns
tWEHANV WE HIGH to address invalid time - 1 TCLCL - ns
tWEHDNV WE HIGH to data invalid time - 1 TCLCL - ns
tBLSHANV BLS HIGH to address invalid time - 1 TCLCL - ns
tBLSHDNV BLS HIGH to data invalid time - 1 TCLCL - ns
Table 12. Dynamic characteristics: static external memory interface …continued
CL = 25 pF, Tamb = 20C, VDD(EMC) = 1.8 V, 2.5 V, or 3.3 V.
Symbol Parameter Notes Min Typ Max Unit
Fig 7. External memory read access
EMC_CS[3:0]
EMC_A[23:0]
EMC_D[31:0]
EMC_OE
EMC_BLS[3:0]
tCSLAV
tOELAV
tOELOEH
tCSLOEL
tsu(DQ)
th(DQ)
tCSHOEH
tOEHANV
002aae402
tBLSLAV tCSHBLSH
tBLSLBLSH
tCSLBLSL tBLSHANV
LPC3220_30_40_50 All information provided in this document is subject to legal disclaimers. © NXP B.V. 2011. All rights reserved.
Product data sheet Rev. 2 — 20 October 2011 59 of 79
NXP Semiconductors LPC3220/30/40/50
16/32-bit ARM microcontrollers
Fig 8. External memory write access
EMC_A[23:0]
EMC_D[31:0]
tCSLWEL
tCSLBLSL
tWELDV
tCSLDV
tWELWEH
tWEHANV
tBLSHANV
tWEHDNV
tBLSHDNV
002aae469
tCSLAV
EMC_CS[3:0]
tBLSLBLSH
EMC_BLS[3:0]
EMC_WR
LPC3220_30_40_50 All information provided in this document is subject to legal disclaimers. © NXP B.V. 2011. All rights reserved.
Product data sheet Rev. 2 — 20 October 2011 60 of 79
NXP Semiconductors LPC3220/30/40/50
16/32-bit ARM microcontrollers
11.3 SDR SDRAM Controller
[1] Parameters are valid over operating temperature range unless otherwise specified.
[2] Typical values valid for EMC pads set to fast slew rate: VDD_EMC = 1.8 V, VDD_CORE = 1.2 V or slower slew rate: VDD_EMC = 3.3 V,
VDD_CORE = 1.2 V (see SDRAMCLK_CTRL register in the LPC32x0 User manual).
[3] All min or max values valid for EMC pads set to fast slew rate: VDD_EMC = 1.8 V, VDD_CORE = 1.2 V or slower slew rate: VDD_EMC
= 3.3 V, VDD_CORE = 1.2 V.
[4] foper = 1/tCK.
[5] Applies to signals: EMC_DQM[3:0], EMC_DYCS[1:0], EMC_RAS, EMC_CAS, EMC_WR, EMC_CKE[1:0].
[6] CMD_DLY = COMMAND_DELAY bitfield in SDRAMCLK_CTRL[18:14] register, see External Memory Controller (EMC) chapter in
LPC32x0 User manual.
Table 13. EMC SDR SDRAM memory interface dynamic characteristics
CL = 25 pF, Tamb = 40 C to +85 C, unless otherwise specified.[1][3]
Symbol Parameter Min Typical[2] Max Unit
foper operating frequency [4] 104 133 MHz
tCK clock cycle time 7.5 9.6 - ns
tCL CK LOW-level width - 4.8 - ns
tCH CK HIGH-level width - 4.8 - ns
td(V)ctrl control valid delay time [5][6] - (CMD_DLY 0.25) + 2.7 ns
th(ctrl) control hold time [5][6] (CMD_DLY 0.25) + 1.2 - ns
td(AV) address valid delay time [6] - (CMD_DLY 0.25) + 3.2 ns
th(A) address hold time [6] (CMD_DLY 0.25) + 1.2 - ns
td(QV) data output valid delay time [6] - (CMD_DLY 0.25) + 3.5 ns
th(Q) data output hold time [6] (CMD_DLY 0.25) + 1.2 - ns
tsu(D) data input set-up time - 0.6 - ns
th(D) data input hold time - 0.9 - ns
tQZ data output high-impedance time - - tCK ns
Fig 9. SDR SDRAM signal timing
002aae420
EMC_CLK
output signal (O)
input signal (I)
td(V)ctrl, td(AV), td(QV) th(ctrl), th(Q), th(A)
tsu(D) th(D)
tCK
tCH tCL
tQZ
LPC3220_30_40_50 All information provided in this document is subject to legal disclaimers. © NXP B.V. 2011. All rights reserved.
Product data sheet Rev. 2 — 20 October 2011 61 of 79
NXP Semiconductors LPC3220/30/40/50
16/32-bit ARM microcontrollers
11.4 DDR SDRAM controller
[1] All values valid for EMC pads set to fast slew rate at 1.8 V unless otherwise specified (see SDRAMCLK_CTRL register in the LPC32x0
User manual).
[2] CMD_DLY = COMMAND_DELAY bitfield in SDRAMCLK_CTRL[18:14] register, see External Memory Controller (EMC) chapter in
LPC32x0 User manual.
[3] Applies to signals EMC_DQM[3:0], EMC_DYCS[1:0], EMC_RAS, EMC_CAS, EMC_WR, EMC_CKE[1:0].
[4] DQS_DELAY, see LPC32x0 User manual, External Memory Controller Chapter, Section 8 DDR DQS delay calibration for details on
configuring this value.
[5] Test conditions for measurements: Tamb = 40 C to +85 C; operating frequency range foper = 52 MHz to 133 MHz; EMC_DQM[3:0] and
EMC_D[31:0] driving 2 inches of 50 characteristic impedance trace with 10 pF capacitive load; no external source series termination
resistors used. EMC pads set to fast slew rate at 1.8 V or 2.5 V (see SDRAMCLK_CTRL register in the LPC32x0 User manual).
Table 14. EMC DDR SDRAM memory interface dynamic characteristics[1]
CL = 25 pF, Tamb = 25C, unless otherwise specified.
Symbol Parameter Conditions Min Typical Max Unit
foper operating frequency - 104 133 MHz
tCK clock cycle time 7.5 9.6 - ns
tCL CK LOW-level width - 0.5 tCK - ns
tCH CK HIGH-level width - 0.5 tCK - ns
td(V)ctrl control valid delay time [2][3] - (CMD_DLY 0.25) + 1.5 - ns
th(ctrl) control hold time [2][3] - (CMD_DLY 0.25) 1.5 - ns
td(AV) address valid delay time [2] - (CMD_DLY 0.25) + 1.5 - ns
th(A) address hold time [2] - (CMD_DLY 0.25) 1.5 - ns
tsu(Q) data output set-up time EMC_D[31:0]
and
EMC_DQM[3:0]
to
EMC_DQS[1:0]
out
[5] 0.08
tCK
0.15 tCK 0.25
tCK
ns
th(Q) data output hold time EMC_D[31:0]
and
EMC_DQM[3:0]
to
EMC_DQS[1:0]
out
[5] 0.25
tCK
0.35 tCK 0.42
tCK
ns
tDQSH DQS HIGH time for WRITE
command
- 0.5 tCK - ns
tDQSL DQS LOW time for WRITE
command
- 0.5 tCK - ns
tDQSS WRITE command to first DQS latching
transition time
for DQS out - tCK + 0.7 - ns
tDSS DQS falling edge to CK set-up time for DQS in - 0.5 tCK - ns
tDSH DQS falling edge hold time from CK for DQS in - 0.5 tCK - ns
td(DQS) DQS delay time for DQS in [4] - DQS_DELAY - ns
tsu(D) data input set-up time - 0.3 - ns
th(D) data input hold time - 0.5 - ns
LPC3220_30_40_50 All information provided in this document is subject to legal disclaimers. © NXP B.V. 2011. All rights reserved.
Product data sheet Rev. 2 — 20 October 2011 62 of 79
NXP Semiconductors LPC3220/30/40/50
16/32-bit ARM microcontrollers
Fig 10. DDR control timing parameters
EMC_CLK
EMC control
and address
signals
002aae436
tCK tCH tCL
td(AV); td(V)ctrl th(A); th(ctl)
valid
Fig 11. DDR write timing parameters
command
EMC_D[31:0],
EMC_DQM[3:0]
tDQSS tDQSL tDQSH
th(Q)
EMC_DQS[1:0]
EMC_CLK
002aae437
WRITE
tsu(Q)
tDSS tDSH
(1) The delay of the EMC_DQS[1:0] signal is determined by the DQS_DELAY settings. See LPC32x0 User manual, External
Memory Controller Chapter, section DDR DQS delay calibration for details on configuring this value.
Fig 12. DDR read timing parameters
EMC_CLK
command
EMC_D[31:0]
tsu(D)
EMC_DQS[1:0]
002aae438
th(D)
READ
delayed EMC_DQS[1:0](1)
td(DQS)
LPC3220_30_40_50 All information provided in this document is subject to legal disclaimers. © NXP B.V. 2011. All rights reserved.
Product data sheet Rev. 2 — 20 October 2011 63 of 79
NXP Semiconductors LPC3220/30/40/50
16/32-bit ARM microcontrollers
11.5 USB controller
[1] Parameters are valid over operating temperature range unless otherwise specified.
11.6 Secure Digital (SD) card interface
[1] Parameters are valid over operating temperature range unless otherwise specified.
[2] Typical ratings are not guaranteed. The values listed are at room temperature (25 C), nominal supply voltages.
Table 15. Dynamic characteristics USB digital I/O pins
VDD(IO) = 3.3 V; Tamb = 40 C to +85 C, unless otherwise specified.[1]
Symbol Parameter Conditions Min Typ Max Unit
tTIO bus turnaround time (I/O) OE_N/INT_N to DAT/VP and SE0/VM - 7 - ns
tTOI bus turnaround time (O/I) OE_N/INT_N to DAT/VP and SE0/VM - 0 - ns
Fig 13. USB bus turnaround time
002aae440
USB_DAT_VP
tTIO tTOI
USB_OE_TP
USB_SE0_VM
input output input
Table 16. Dynamic characteristics: SD card pin interface
Tamb = 40 C to +85 C for industrial applications; VDD(IO) over specified ranges.[1]
Symbol Parameter Conditions Min Typ[2] Max Unit
Tcy(clk) clock cycle time on pin MS_SCLK; Data transfer mode - - 25 MHz
on pin MS_SCLK; Identification mode - - 400 kHz
tsu(D) data input set-up time on pins MS_BS, MS_DIO[3:0] as inputs - 2.7 - ns
th(D) data input hold time on pins MS_BS, MS_DIO[3:0] as inputs - 0 - ns
td(QV) data output valid delay time on pins MS_BS, MS_DIO[3:0] as outputs - 9.7 - ns
th(Q) data output hold time on pins MS_BS, MS_DIO[3:0] as outputs - 7.7 - ns
LPC3220_30_40_50 All information provided in this document is subject to legal disclaimers. © NXP B.V. 2011. All rights reserved.
Product data sheet Rev. 2 — 20 October 2011 64 of 79
NXP Semiconductors LPC3220/30/40/50
16/32-bit ARM microcontrollers
11.7 MLC NAND flash memory controller
[1] THCLK = 1/HCLK
[2] CEAD = bitfield TCEA_DELAY[1:0] in register MLC_TIME_REG[25:24]
[3] WL = bitfield WR_LOW[3:0] in register MLC_TIME_REG[3:0]
[4] WH = bitfield WR_HIGH[3:0] in register MLC_TIME_REG[7:4]
[5] RL = bitfield RD_LOW[3:0] in register MLC_TIME_REG[11:8]
[6] RH = bitfield RD_HIGH [3:0] in register MLC_TIME_REG[15:12]
[7] RHZ = bitfield NAND_TA[2:0] in register MLC_TIME_REG[18:16]
[8] BD = bitfield BUSY_DELAY[4:0] in register MLC_TIME_REG[23:19]
Fig 14. SD card pin interface timing
002aae441
MS_SCLK
MS_DIO[3:0](O)
MS_DIO[3:0] (I)
td(QV)
tsu(D) th(D)
Tcy(clk)
th(Q)
MS_BS (O)
MS_BS (I)
Table 17. Dynamic characteristics of the MLC NAND flash memory controller
Tamb = 40 C to +85 C.
Symbol Parameter Min Typ Max Unit
tCELREL CE LOW to RE LOW time [1][2] - THCLK CEAD - ns
tRC RE cycle time [1][5][6] - THCLK (RL + 1) + THCLK (RH RL) - ns
tREH RE HIGH hold time [1][5][6] - THCLK (RH RL) - ns
tRHZ RE HIGH to output high-impedance time [1][5][7] - THCLK (RH RL) + THCLK RHZ - ns
tRP RE pulse width [1][5] - THCLK (RL + 1) - ns
tREHRBL RE HIGH to R/B LOW time [1][8] - THCLK BD - ns
tWB WE HIGH to R/B LOW time [1][8] - THCLK BD - ns
tWC WE cycle time [1][3][4] - THCLK (WL + 1) + THCLK (WH WL) - ns
tWH WE HIGH hold time [1][3][4] - THCLK (WH WL) - ns
tWP WE pulse width [1][3] - THCLK (WL + 1) - ns
LPC3220_30_40_50 All information provided in this document is subject to legal disclaimers. © NXP B.V. 2011. All rights reserved.
Product data sheet Rev. 2 — 20 October 2011 65 of 79
NXP Semiconductors LPC3220/30/40/50
16/32-bit ARM microcontrollers
11.8 SLC NAND flash memory controller
Fig 15. MLC NAND flash controller write timing (writing to NAND flash)
Fig 16. MLC NAND flash controller read timing (reading from NAND flash)
tWB
FLASH_IO[7:0]
FLASH_WR
tWP
tWC
FLASH_RDY (R/B)
FLASH_CE
D0 D1 Dn 10h
tWH
002aae442
FLASH_IO[7:0]
tRP tREH
tRC
FLASH_RD
FLASH_CE
tCELREL
D0 D1 D2 D3
tRHZ
002aae443
Table 18. Dynamic characteristics of SLC NAND flash memory controller
Tamb = 40 C to +85 C.
Symbol Parameter Conditions Min Typ Max Unit
tALS ALE set-up time read [1][2][4][6] - THCLK (Rsu + Rw) - ns
write - THCLK (Wsu + Ww) - ns
tALH ALE hold time read [1][7] - THCLK Rh - ns
write - THCLK Wh - ns
tAR ALE to RE delay time read [1][2][6] - THCLK Rsu - ns
write - THCLK Wsu - ns
LPC3220_30_40_50 All information provided in this document is subject to legal disclaimers. © NXP B.V. 2011. All rights reserved.
Product data sheet Rev. 2 — 20 October 2011 66 of 79
NXP Semiconductors LPC3220/30/40/50
16/32-bit ARM microcontrollers
tCEA CE access time read [1][2][4][6][8] - THCLK (Rsu + Rw) - ns
write - THCLK (Wsu + Ww) - ns
tCS CE set-up time read [1][2][4][6][8] - THCLK (Rsu + Rw) - ns
write - THCLK (Wsu + Ww) - ns
tCH CE hold time read [1][3] - THCLK Rh - ns
write - THCLK Wh - ns
tCLS CLE set-up time read [1][2][4][6][8] - THCLK (Rsu + Rw) - ns
write - THCLK (Wsu + Ww) - ns
tCLH CLE hold time read [1][3] - THCLK Rh - ns
write - THCLK Wh - ns
tCLR CLE to RE delay time read [1][2][6] - THCLK Rsu - ns
write - THCLK Wsu - ns
tDH data hold time output from
NAND
controller; read
[1][3][7] - THCLK Rh - ns
output from
NAND
controller;
write
- THCLK Wh - ns
tDS data set-up time output from
NAND
controller; read
[1][2][4][6][8] - THCLK (Rsu + Rw) - ns
output from
NAND
controller;
write
- THCLK (Wsu + Ww) -
tIR output high-impedance to RE
LOW time
read [1][2][6] - THCLK Rsu - ns
write - THCLK Wsu - ns
tRC RE cycle time read [1][2] - THCLK (Rsu + Rw + Rh) - ns
tREA RE access time read [1][4] - THCLK Rw - ns
tREH RE high hold time read [1][2][3] - THCLK (Rsu + Rh) - ns
tRHOH RE HIGH to output hold time input hold for
flash
controller; read
- 0 - -
input hold for
flash
controller;
write
- 0 - -
tRHZ RE HIGH to output
high-impedance time
read [1] - THCLK Rh - ns
tRP RE pulse width read [1][4] - THCLK Rw - ns
tRR ready to RE LOW time read [1][2][3] - THCLK Rsu - ns
tWB WE HIGH to R/B LOW time write [1][7][9] - (THCLK Wh) + (2 THCLK
Wb)
- ns
tWC WE cycle time write [1][6][7][8] - THCLK (Wsu + Ww + Wh) - ns
Table 18. Dynamic characteristics of SLC NAND flash memory controller …continued
Tamb = 40 C to +85 C.
Symbol Parameter Conditions Min Typ Max Unit
LPC3220_30_40_50 All information provided in this document is subject to legal disclaimers. © NXP B.V. 2011. All rights reserved.
Product data sheet Rev. 2 — 20 October 2011 67 of 79
NXP Semiconductors LPC3220/30/40/50
16/32-bit ARM microcontrollers
[1] THCLK = 1/HCLK
[2] Rsu = bitfield R_SETUP[3:0] in register SLC_TAC[3:0] for reads
[3] Rh = bitfield R_HOLD[3:0] in register SLC_TAC[7:4] for reads
[4] Rw = bitfield R_WIDTH[3:0] in register SLC_TAC[11:8] for reads
[5] Rb = bitfield R_RDY[3:0] in register SLC_TAC[15:12] for reads
[6] Wsu = bitfield W_SETUP[3:0] in register SLC_TAC[19:16] for writes
[7] Wh = bitfield W_HOLD[3:0] in register SLC_TAC[23:20] for writes
[8] Ww = bitfield W_WIDTH[3:0] in register SLC_TAC[27:24] for writes
[9] Wb = bitfield W_RDY[3:0] in register SLC_TAC[31:28] for writes
tWH WE HIGH hold time write [1][6][7] - THCLK (Wsu + Wh) - ns
tWHR WE HIGH to RE LOW time write [1][7][9] - (THCLK Wh) + (2 THCLK
Wb)
- ns
tWP WE pulse width write [1][8] - THCLK Ww - ns
tREHRBL RE HIGH to R/B LOW time write [1][3][5] - (THCLK Rh) + (2 THCLK
Rb)
- ns
Table 18. Dynamic characteristics of SLC NAND flash memory controller …continued
Tamb = 40 C to +85 C.
Symbol Parameter Conditions Min Typ Max Unit
Fig 17. MLC NAND flash memory write timing (writing to NAND flash)
command
tDS tDH
tWB
FLASH_IO[7:0] address
tDS tDH
tALS tALH
tDS tDH
tWP tWH tWC
tCS tCH tCH
tCLS tCLH
command address data
D0 D1 Dn
tALS
tCLH
tALS tALH
tWP tWP tWH
tCLS
tCS
002aae444
FLASH_CE
FLASH_CLE
FLASH_WR
FLASH_ALE
FLASH_RDY
LPC3220_30_40_50 All information provided in this document is subject to legal disclaimers. © NXP B.V. 2011. All rights reserved.
Product data sheet Rev. 2 — 20 October 2011 68 of 79
NXP Semiconductors LPC3220/30/40/50
16/32-bit ARM microcontrollers
Fig 18. MLC NAND Flash memory read timing (reading from NAND flash)
command
tDS tDH
tWB
FLASH_IO[7:0] address
tDS tDH
tALS tALH
tDS tDH
tRP tREH tRC
tRR
tAR
tCS tCH tCEA
tCLR
tCLS tCLH
command address data
tCOH
tREA
D0 D1 D2 D3
tRHZ
tRHOH
tALS tALH
tCLS
tCS
tWP tWP tWH
002aae445
FLASH_ALE
FLASH_CLE
FLASH_RDY
FLASH_WR
FLASH_RD
FLASH_CE
LPC3220_30_40_50 All information provided in this document is subject to legal disclaimers. © NXP B.V. 2011. All rights reserved.
Product data sheet Rev. 2 — 20 October 2011 69 of 79
NXP Semiconductors LPC3220/30/40/50
16/32-bit ARM microcontrollers
11.9 SPI and SSP Controller
11.9.1 SPI
[1] THCLK = period time of SPI IP block input clock (HCLK)
Fig 19. MLC NAND flash memory status timing
tCS tCH tCEA
70 h
tDS tDH
status
tRHOH
tCLS tCLH
command data
tCLR
tCOH
tREA
tIR
FLASH_IO[7:0]
tWHR
tWP
tRHZ
FLASH_CLE
FLASH_WR
FLASH_CE
FLASH_RD
002aae446
Table 19. Dynamic characteristics of SPI pins on SPI master controller
Tamb = 40 C to +85 C.
Symbol Parameter Min Typ Max Unit
Common to SPI1 and SPI2
TSPICYC SPI cycle time [1] 2 THCLK - 256 THCLK ns
SPI1
tSPIDSU SPI data set-up time - 6 - ns
tSPIDH SPI data hold time - 0 - ns
tSPIDV SPI enable to output data valid time - 2 - ns
tSPIOH SPI output data hold time - 0 - ns
SPI2
tSPIDSU SPI data set-up time - 10 - ns
tSPIDH SPI data hold time - 0 - ns
tSPIDV SPI enable to output data valid time - 2 - ns
tSPIOH SPI output data hold time - 0 - ns
LPC3220_30_40_50 All information provided in this document is subject to legal disclaimers. © NXP B.V. 2011. All rights reserved.
Product data sheet Rev. 2 — 20 October 2011 70 of 79
NXP Semiconductors LPC3220/30/40/50
16/32-bit ARM microcontrollers
11.9.2 Timing diagrams for SPI and SSP (in SPI mode)
Fig 20. SPI master timing (CPHA = 0)
Fig 21. SPI master timing (CPHA = 1)
002aae457
TSPICYC tSPICLKH tSPICLKL
tSPIDSU tSPIDH
DATA VALID DATA VALID
tSPIOH
DATA VALID DATA VALID
tSPIQV
SPI1/2_CLK or
SCK0/1 (CPOL = 0)
SPI1/2_CLK or
SCK0/1 (CPOL = 1)
SPI1/2_DATAIO or
MOSI0/1
SPI1/2_DATAIN or
MISO0/1
002aae454
TSPICYC tSPICLKH tSPICLKL
tSPIDSU tSPIDH
tSPIQV
DATA VALID DATA VALID
tSPIOH
DATA VALID DATA VALID
SPI1/2_CLK or
SCK0/1 (CPOL = 0)
SPI1/2_CLK or
SCK0/1 (CPOL = 1)
SPI1/2_DATAIO or
MOSI0/1
SPI1/2_DATAIN or
MISO0/1
LPC3220_30_40_50 All information provided in this document is subject to legal disclaimers. © NXP B.V. 2011. All rights reserved.
Product data sheet Rev. 2 — 20 October 2011 71 of 79
NXP Semiconductors LPC3220/30/40/50
16/32-bit ARM microcontrollers
Fig 22. SPI slave timing (CPHA = 0)
Fig 23. SPI slave timing (CPHA = 1)
002aae458
TSPICYC tSPICLKH tSPICLKL
tSPIDSU tSPIDH
tSPIQV
DATA VALID DATA VALID
tSPIOH
DATA VALID DATA VALID
SPI1/2_CLK or
SCK0/1 (CPOL = 0)
SPI1/2_CLK or
SCK0/1 (CPOL = 1)
SPI1/2_DATAIO or
MOSI0/1
SPI1/2_DATAIN or
MISO0/1
002aae459
TSPICYC tSPICLKH tSPICLKL
tSPIDSU tSPIDH
tSPIQV
DATA VALID DATA VALID
tSPIOH
DATA VALID DATA VALID
SPI1/2_CLK or
SCK0/1 (CPOL = 0)
SPI1/2_CLK or
SCK0/1 (CPOL = 1)
SPI1/2_DATAIO or
MOSI0/1
SPI1/2_DATAIN or
MISO0/1
LPC3220_30_40_50 All information provided in this document is subject to legal disclaimers. © NXP B.V. 2011. All rights reserved.
Product data sheet Rev. 2 — 20 October 2011 72 of 79
NXP Semiconductors LPC3220/30/40/50
16/32-bit ARM microcontrollers
12. Package outline
Fig 24. Package outline SOT1048-1 (TFBGA296)
OUTLINE REFERENCES
VERSION
EUROPEAN
PROJECTION
ISSUE DATE
IEC JEDEC JEITA
SOT1048-1 MO-216
SOT1048-1
07-10-19
07-11-02
UNIT A
max
mm 1.2 0.4
0.3
0.80
0.65
15.1
14.9
15.1
14.9 0.8 13.6 0.15 0.08 0.1
A1
DIMENSIONS (mm are the original dimensions)
TFBGA296: plastic thin fine-pitch ball grid array package; 296 balls
0 5 10 mm
scale
A2 b
0.5
0.4
D E e e1 e2
13.6
v w y
0.12
y1
C
y1 C y
X
A
B
C
D
E
F
H
K
G
L
J
M
N
P
R
T
U
2 4 6 8 10 12 14 16
1 3 5 7 9 11 13 15
18
17
b
e2
e1
e
e
1/2 e
1/2 e
∅ v M C A B
∅ w M C
ball A1
index area
V
B A
ball A1
index area
D
E
detail X
A
A2
A1
LPC3220_30_40_50 All information provided in this document is subject to legal disclaimers. © NXP B.V. 2011. All rights reserved.
Product data sheet Rev. 2 — 20 October 2011 73 of 79
NXP Semiconductors LPC3220/30/40/50
16/32-bit ARM microcontrollers
13. Abbreviations
Table 20. Abbreviations
Acronym Description
ADC Analog-to-Digital Converter
AHB Advanced High-performance Bus
AMBA Advanced Microcontroller Bus Architecture
APB Advanced Peripheral Bus
BSDL Boundary Scan Description Language
CISC Complex Instruction Set Computer
DDR SDRAM Double Data Rate Synchronous Dynamic Random Access Memory
DMA Direct Memory Access
DSP Digital Signal Processing
ETM Embedded Trace Macrocell
FAB Fast Access Bus
FIFO First In, First Out
FIQ Fast Interrupt Request
GPIO General Purpose Input/Output
I/O Input/Output
IRQ Interrupt Request
HS High-Speed
IrDA Infrared Data Association
JTAG Joint Test Action Group
LCD Liquid Crystal Display
MAC Media Access Control
MIIM Media Independent Interface Management
OHCI Open Host Controller Interface
OTG On-The-Go
PHY Physical Layer
PLL Phase-Locked Loop
PWM Pulse Width Modulator
RAM Random Access Memory
RMII Reduced Media Independent Interface
SE0 Single Ended Zero
SDR SDRAM Single Data Rate Synchronous Dynamic Random Access Memory
SPI Serial Peripheral Interface
SSI Serial Synchronous Interface
SSP Synchronous Serial Port
TFT Thin Film Transistor
TTL Transistor-Transistor Logic
STN Super Twisted Nematic
LPC3220_30_40_50 All information provided in this document is subject to legal disclaimers. © NXP B.V. 2011. All rights reserved.
Product data sheet Rev. 2 — 20 October 2011 74 of 79
NXP Semiconductors LPC3220/30/40/50
16/32-bit ARM microcontrollers
UART Universal Asynchronous Receiver/Transmitter
USB Universal Serial Bus
VFP Vector Floating Point processor
Table 20. Abbreviations …continued
Acronym Description
LPC3220_30_40_50 All information provided in this document is subject to legal disclaimers. © NXP B.V. 2011. All rights reserved.
Product data sheet Rev. 2 — 20 October 2011 75 of 79
NXP Semiconductors LPC3220/30/40/50
16/32-bit ARM microcontrollers
14. Revision history
Table 21. Revision history
Document ID Release date Data sheet status Change notice Supersedes
LPC3220_30_40_50 v.2 20111020 Product data sheet - LPC3220_30_40_50 v.1
Modifications: • Corrected pin functions for pin T14 (ADIN1/TS_XM) and pin U15 (ADIN0/TS_YM) in
Table 3 and Table 4.
• Power domain for pin PLL397_LOOP corrected in Table 4.
• Power supply domain for pins SYSX_IN and SYSX_OUT pins corrected in Table 4.
• Power supply domain for pin VDD_OSC corrected in Table 4.
• Description of DEBUG pin updated in Table 4.
• Added Table 6 “Supply domains”.
• Changed VESD to 2500 V (HBM) and 1000 V (CDM) in Table 7.
• Power consumption for HCLK, USB, and ADC added in Table 8.
• Parameter IDD(RTC) updated in Table 8.
• Parameter VDD(EMC) table notes updated in Table 8.
• Input current for bus keeper inputs added in Table 8.
• Added power consumption data (Table 8, Table 9, and Figure 5).
• Static memory controller: added tsu(DQ) value in Table 12.
• DDR SDRAM controller: updated tDQSS value in Table 14.
• Minimum and maximum characterization data added for parameters tsu(Q) and th(Q) over
temperature range 40 C to +85 C (see Table 14).
• DDR SDRAM characteristics extended to maximum operating frequency foper = 133 MHz
(see Table 14).
• Parameters tWB, tWHR, and tREHRBL updated in Table 18.
• Changed data sheet status to Product data sheet.
• Parts LPC3220FET296/01, LPC3230FET296/01, LPC3240FET296/01,
LPC3250FET296/01 added.
LPC3220_30_40_50 v.1 20090206 Preliminary data sheet - -
LPC3220_30_40_50 All information provided in this document is subject to legal disclaimers. © NXP B.V. 2011. All rights reserved.
Product data sheet Rev. 2 — 20 October 2011 76 of 79
NXP Semiconductors LPC3220/30/40/50
16/32-bit ARM microcontrollers
15. Legal information
15.1 Data sheet status
[1] Please consult the most recently issued document before initiating or completing a design.
[2] The term ‘short data sheet’ is explained in section “Definitions”.
[3] The product status of device(s) described in this document may have changed since this document was published and may differ in case of multiple devices. The latest product status
information is available on the Internet at URL http://www.nxp.com.
15.2 Definitions
Draft — The document is a draft version only. The content is still under
internal review and subject to formal approval, which may result in
modifications or additions. NXP Semiconductors does not give any
representations or warranties as to the accuracy or completeness of
information included herein and shall have no liability for the consequences of
use of such information.
Short data sheet — A short data sheet is an extract from a full data sheet
with the same product type number(s) and title. A short data sheet is intended
for quick reference only and should not be relied upon to contain detailed and
full information. For detailed and full information see the relevant full data
sheet, which is available on request via the local NXP Semiconductors sales
office. In case of any inconsistency or conflict with the short data sheet, the
full data sheet shall prevail.
Product specification — The information and data provided in a Product
data sheet shall define the specification of the product as agreed between
NXP Semiconductors and its customer, unless NXP Semiconductors and
customer have explicitly agreed otherwise in writing. In no event however,
shall an agreement be valid in which the NXP Semiconductors product is
deemed to offer functions and qualities beyond those described in the
Product data sheet.
15.3 Disclaimers
Limited warranty and liability — Information in this document is believed to
be accurate and reliable. However, NXP Semiconductors does not give any
representations or warranties, expressed or implied, as to the accuracy or
completeness of such information and shall have no liability for the
consequences of use of such information.
In no event shall NXP Semiconductors be liable for any indirect, incidental,
punitive, special or consequential damages (including - without limitation - lost
profits, lost savings, business interruption, costs related to the removal or
replacement of any products or rework charges) whether or not such
damages are based on tort (including negligence), warranty, breach of
contract or any other legal theory.
Notwithstanding any damages that customer might incur for any reason
whatsoever, NXP Semiconductors’ aggregate and cumulative liability towards
customer for the products described herein shall be limited in accordance
with the Terms and conditions of commercial sale of NXP Semiconductors.
Right to make changes — NXP Semiconductors reserves the right to make
changes to information published in this document, including without
limitation specifications and product descriptions, at any time and without
notice. This document supersedes and replaces all information supplied prior
to the publication hereof.
Suitability for use — NXP Semiconductors products are not designed,
authorized or warranted to be suitable for use in life support, life-critical or
safety-critical systems or equipment, nor in applications where failure or
malfunction of an NXP Semiconductors product can reasonably be expected
to result in personal injury, death or severe property or environmental
damage. NXP Semiconductors accepts no liability for inclusion and/or use of
NXP Semiconductors products in such equipment or applications and
therefore such inclusion and/or use is at the customer’s own risk.
Applications — Applications that are described herein for any of these
products are for illustrative purposes only. NXP Semiconductors makes no
representation or warranty that such applications will be suitable for the
specified use without further testing or modification.
Customers are responsible for the design and operation of their applications
and products using NXP Semiconductors products, and NXP Semiconductors
accepts no liability for any assistance with applications or customer product
design. It is customer’s sole responsibility to determine whether the NXP
Semiconductors product is suitable and fit for the customer’s applications and
products planned, as well as for the planned application and use of
customer’s third party customer(s). Customers should provide appropriate
design and operating safeguards to minimize the risks associated with their
applications and products.
NXP Semiconductors does not accept any liability related to any default,
damage, costs or problem which is based on any weakness or default in the
customer’s applications or products, or the application or use by customer’s
third party customer(s). Customer is responsible for doing all necessary
testing for the customer’s applications and products using NXP
Semiconductors products in order to avoid a default of the applications and
the products or of the application or use by customer’s third party
customer(s). NXP does not accept any liability in this respect.
Limiting values — Stress above one or more limiting values (as defined in
the Absolute Maximum Ratings System of IEC 60134) will cause permanent
damage to the device. Limiting values are stress ratings only and (proper)
operation of the device at these or any other conditions above those given in
the Recommended operating conditions section (if present) or the
Characteristics sections of this document is not warranted. Constant or
repeated exposure to limiting values will permanently and irreversibly affect
the quality and reliability of the device.
Terms and conditions of commercial sale — NXP Semiconductors
products are sold subject to the general terms and conditions of commercial
sale, as published at http://www.nxp.com/profile/terms, unless otherwise
agreed in a valid written individual agreement. In case an individual
agreement is concluded only the terms and conditions of the respective
agreement shall apply. NXP Semiconductors hereby expressly objects to
applying the customer’s general terms and conditions with regard to the
purchase of NXP Semiconductors products by customer.
No offer to sell or license — Nothing in this document may be interpreted or
construed as an offer to sell products that is open for acceptance or the grant,
conveyance or implication of any license under any copyrights, patents or
other industrial or intellectual property rights.
Export control — This document as well as the item(s) described herein
may be subject to export control regulations. Export might require a prior
authorization from competent authorities.
Document status[1][2] Product status[3] Definition
Objective [short] data sheet Development This document contains data from the objective specification for product development.
Preliminary [short] data sheet Qualification This document contains data from the preliminary specification.
Product [short] data sheet Production This document contains the product specification.
LPC3220_30_40_50 All information provided in this document is subject to legal disclaimers. © NXP B.V. 2011. All rights reserved.
Product data sheet Rev. 2 — 20 October 2011 77 of 79
NXP Semiconductors LPC3220/30/40/50
16/32-bit ARM microcontrollers
Non-automotive qualified products — Unless this data sheet expressly
states that this specific NXP Semiconductors product is automotive qualified,
the product is not suitable for automotive use. It is neither qualified nor tested
in accordance with automotive testing or application requirements. NXP
Semiconductors accepts no liability for inclusion and/or use of
non-automotive qualified products in automotive equipment or applications.
In the event that customer uses the product for design-in and use in
automotive applications to automotive specifications and standards, customer
(a) shall use the product without NXP Semiconductors’ warranty of the
product for such automotive applications, use and specifications, and (b)
whenever customer uses the product for automotive applications beyond
NXP Semiconductors’ specifications such use shall be solely at customer’s
own risk, and (c) customer fully indemnifies NXP Semiconductors for any
liability, damages or failed product claims resulting from customer design and
use of the product for automotive applications beyond NXP Semiconductors’
standard warranty and NXP Semiconductors’ product specifications.
15.4 Trademarks
Notice: All referenced brands, product names, service names and trademarks
are the property of their respective owners.
I2C-bus — logo is a trademark of NXP B.V.
16. Contact information
For more information, please visit: http://www.nxp.com
For sales office addresses, please send an email to: salesaddresses@nxp.com
LPC3220_30_40_50 All information provided in this document is subject to legal disclaimers. © NXP B.V. 2011. All rights reserved.
Product data sheet Rev. 2 — 20 October 2011 78 of 79
continued >>
NXP Semiconductors LPC3220/30/40/50
16/32-bit ARM microcontrollers
17. Contents
1 General description . . . . . . . . . . . . . . . . . . . . . . 1
2 Features and benefits . . . . . . . . . . . . . . . . . . . . 1
3 Applications . . . . . . . . . . . . . . . . . . . . . . . . . . . . 3
4 Ordering information. . . . . . . . . . . . . . . . . . . . . 4
4.1 Ordering options . . . . . . . . . . . . . . . . . . . . . . . . 4
5 Block diagram . . . . . . . . . . . . . . . . . . . . . . . . . . 5
6 Pinning information. . . . . . . . . . . . . . . . . . . . . . 6
6.1 Pinning . . . . . . . . . . . . . . . . . . . . . . . . . . . . . . . 6
6.2 Pin description . . . . . . . . . . . . . . . . . . . . . . . . 10
7 Functional description . . . . . . . . . . . . . . . . . . 24
7.1 CPU and subsystems . . . . . . . . . . . . . . . . . . . 24
7.1.1 CPU . . . . . . . . . . . . . . . . . . . . . . . . . . . . . . . . 24
7.1.2 Vector Floating Point (VFP) coprocessor . . . . 24
7.1.3 Emulation and debugging. . . . . . . . . . . . . . . . 24
7.1.3.1 Embedded ICE . . . . . . . . . . . . . . . . . . . . . . . . 24
7.1.3.2 Embedded trace buffer . . . . . . . . . . . . . . . . . . 25
7.2 AHB matrix . . . . . . . . . . . . . . . . . . . . . . . . . . . 25
7.2.1 APB . . . . . . . . . . . . . . . . . . . . . . . . . . . . . . . . 26
7.2.2 FAB. . . . . . . . . . . . . . . . . . . . . . . . . . . . . . . . . 26
7.3 Physical memory map . . . . . . . . . . . . . . . . . . 26
7.4 Internal memory . . . . . . . . . . . . . . . . . . . . . . . 28
7.4.1 On-chip ROM . . . . . . . . . . . . . . . . . . . . . . . . . 28
7.4.2 On-chip SRAM . . . . . . . . . . . . . . . . . . . . . . . . 28
7.5 External memory interfaces . . . . . . . . . . . . . . 28
7.5.1 NAND flash controllers . . . . . . . . . . . . . . . . . . 28
7.5.1.1 Multi-Level Cell (MLC) NAND flash
controller. . . . . . . . . . . . . . . . . . . . . . . . . . . . . 28
7.5.1.2 Single-Level Cell (SLC) NAND flash
controller. . . . . . . . . . . . . . . . . . . . . . . . . . . . . 29
7.5.2 SD card controller. . . . . . . . . . . . . . . . . . . . . . 29
7.5.2.1 Features . . . . . . . . . . . . . . . . . . . . . . . . . . . . . 29
7.5.3 External memory controller. . . . . . . . . . . . . . . 29
7.6 AHB master peripherals . . . . . . . . . . . . . . . . . 30
7.6.1 General Purpose DMA (GPDMA)
controller . . . . . . . . . . . . . . . . . . . . . . . . . . . . 30
7.6.2 Ethernet MAC . . . . . . . . . . . . . . . . . . . . . . . . . 30
7.6.2.1 Features . . . . . . . . . . . . . . . . . . . . . . . . . . . . . 31
7.6.3 USB interface . . . . . . . . . . . . . . . . . . . . . . . . . 31
7.6.3.1 USB device controller . . . . . . . . . . . . . . . . . . . 31
7.6.3.2 USB host controller. . . . . . . . . . . . . . . . . . . . . 32
7.6.3.3 USB OTG controller . . . . . . . . . . . . . . . . . . . . 32
7.6.4 LCD controller. . . . . . . . . . . . . . . . . . . . . . . . . 33
7.6.4.1 Features . . . . . . . . . . . . . . . . . . . . . . . . . . . . . 33
7.7 System functions . . . . . . . . . . . . . . . . . . . . . . 34
7.7.1 Interrupt controller . . . . . . . . . . . . . . . . . . . . . 34
7.7.2 Watchdog timer. . . . . . . . . . . . . . . . . . . . . . . . 34
7.7.2.1 Features . . . . . . . . . . . . . . . . . . . . . . . . . . . . . 34
7.7.3 Millisecond timer . . . . . . . . . . . . . . . . . . . . . . 34
7.7.3.1 Features. . . . . . . . . . . . . . . . . . . . . . . . . . . . . 34
7.7.4 Clocking and power control features . . . . . . . 35
7.7.4.1 Clocking . . . . . . . . . . . . . . . . . . . . . . . . . . . . . 35
7.7.4.2 Crystal oscillator. . . . . . . . . . . . . . . . . . . . . . . 35
7.7.4.3 PLLs. . . . . . . . . . . . . . . . . . . . . . . . . . . . . . . . 35
7.7.4.4 Power control modes . . . . . . . . . . . . . . . . . . . 36
7.7.4.5 Reset . . . . . . . . . . . . . . . . . . . . . . . . . . . . . . . 36
7.8 Communication peripheral interfaces . . . . . . 36
7.8.1 UARTs . . . . . . . . . . . . . . . . . . . . . . . . . . . . . . 37
7.8.1.1 Standard UARTs. . . . . . . . . . . . . . . . . . . . . . . 37
7.8.1.2 High-speed UARTs . . . . . . . . . . . . . . . . . . . . 37
7.8.2 SPI serial I/O controller . . . . . . . . . . . . . . . . . 37
7.8.2.1 Features. . . . . . . . . . . . . . . . . . . . . . . . . . . . . 38
7.8.3 SSP serial I/O controller. . . . . . . . . . . . . . . . . 38
7.8.3.1 Features. . . . . . . . . . . . . . . . . . . . . . . . . . . . . 38
7.8.4 I2C-bus serial I/O controller . . . . . . . . . . . . . . 38
7.8.4.1 Features. . . . . . . . . . . . . . . . . . . . . . . . . . . . . 39
7.8.5 I2S-bus audio controller . . . . . . . . . . . . . . . . . 39
7.8.5.1 Features. . . . . . . . . . . . . . . . . . . . . . . . . . . . . 39
7.9 Other peripherals . . . . . . . . . . . . . . . . . . . . . . 40
7.9.1 General purpose parallel I/O . . . . . . . . . . . . . 40
7.9.1.1 Features. . . . . . . . . . . . . . . . . . . . . . . . . . . . . 40
7.9.2 Keyboard scanner . . . . . . . . . . . . . . . . . . . . . 41
7.9.2.1 Features. . . . . . . . . . . . . . . . . . . . . . . . . . . . . 41
7.9.3 Touch screen controller and 10-bit ADC . . . . 41
7.9.3.1 Features. . . . . . . . . . . . . . . . . . . . . . . . . . . . . 41
7.9.4 Real-Time Clock (RTC)
and battery RAM . . . . . . . . . . . . . . . . . . . . . . 41
7.9.4.1 Features. . . . . . . . . . . . . . . . . . . . . . . . . . . . . 42
7.9.5 Enhanced 32-bit timers/external
event counters . . . . . . . . . . . . . . . . . . . . . . . . 42
7.9.5.1 Features. . . . . . . . . . . . . . . . . . . . . . . . . . . . . 42
7.9.6 High-speed timer . . . . . . . . . . . . . . . . . . . . . . 43
7.9.6.1 Features. . . . . . . . . . . . . . . . . . . . . . . . . . . . . 43
7.9.7 Pulse Width Modulators (PWMs) . . . . . . . . . . 43
7.9.7.1 Features. . . . . . . . . . . . . . . . . . . . . . . . . . . . . 43
7.9.8 Motor control pulse width modulator . . . . . . . 43
7.9.8.1 Features. . . . . . . . . . . . . . . . . . . . . . . . . . . . . 43
8 Basic architecture . . . . . . . . . . . . . . . . . . . . . . 44
9 Limiting values . . . . . . . . . . . . . . . . . . . . . . . . 45
10 Static characteristics . . . . . . . . . . . . . . . . . . . 46
10.1 Minimum core voltage requirements . . . . . . . 53
10.2 Power supply sequencing . . . . . . . . . . . . . . . 53
10.3 Power consumption per peripheral . . . . . . . . 53
10.4 Power consumption in Run mode . . . . . . . . . 54
10.5 ADC static characteristics . . . . . . . . . . . . . . . 55
NXP Semiconductors LPC3220/30/40/50
16/32-bit ARM microcontrollers
© NXP B.V. 2011. All rights reserved.
For more information, please visit: http://www.nxp.com
For sales office addresses, please send an email to: salesaddresses@nxp.com
Date of release: 20 October 2011
Document identifier: LPC3220_30_40_50
Please be aware that important notices concerning this document and the product(s)
described herein, have been included in section ‘Legal information’.
11 Dynamic characteristics . . . . . . . . . . . . . . . . . 57
11.1 Clocking and I/O port pins . . . . . . . . . . . . . . . 57
11.2 Static memory controller . . . . . . . . . . . . . . . . . 57
11.3 SDR SDRAM Controller . . . . . . . . . . . . . . . . . 60
11.4 DDR SDRAM controller . . . . . . . . . . . . . . . . . 61
11.5 USB controller . . . . . . . . . . . . . . . . . . . . . . . . 63
11.6 Secure Digital (SD) card interface . . . . . . . . . 63
11.7 MLC NAND flash memory controller. . . . . . . . 64
11.8 SLC NAND flash memory controller . . . . . . . . 65
11.9 SPI and SSP Controller . . . . . . . . . . . . . . . . . 69
11.9.1 SPI . . . . . . . . . . . . . . . . . . . . . . . . . . . . . . . . . 69
11.9.2 Timing diagrams for SPI and SSP
(in SPI mode) . . . . . . . . . . . . . . . . . . . . . . . . . 70
12 Package outline . . . . . . . . . . . . . . . . . . . . . . . . 72
13 Abbreviations. . . . . . . . . . . . . . . . . . . . . . . . . . 73
14 Revision history. . . . . . . . . . . . . . . . . . . . . . . . 75
15 Legal information. . . . . . . . . . . . . . . . . . . . . . . 76
15.1 Data sheet status . . . . . . . . . . . . . . . . . . . . . . 76
15.2 Definitions. . . . . . . . . . . . . . . . . . . . . . . . . . . . 76
15.3 Disclaimers . . . . . . . . . . . . . . . . . . . . . . . . . . . 76
15.4 Trademarks. . . . . . . . . . . . . . . . . . . . . . . . . . . 77
16 Contact information. . . . . . . . . . . . . . . . . . . . . 77
17 Contents . . . . . . . . . . . . . . . . . . . . . . . . . . . . . . 78
1. General description
The LPC81xM are an ARM Cortex-M0+ based, low-cost 32-bit MCU family operating at
CPU frequencies of up to 30 MHz. The LPC81xM support up to 16 kB of flash memory
and 4 kB of SRAM.
The peripheral complement of the LPC81xM includes a CRC engine, one I2C-bus
interface, up to three USARTs, up to two SPI interfaces, one multi-rate timer, self wake-up
timer, and state-configurable timer, one comparator, function-configurable I/O ports
through a switch matrix, an input pattern match engine, and up to 18 general-purpose I/O
pins.
2. Features and benefits
System:
ARM Cortex-M0+ processor, running at frequencies of up to 30 MHz with
single-cycle multiplier and fast single-cycle I/O port.
ARM Cortex-M0+ built-in Nested Vectored Interrupt Controller (NVIC).
System tick timer.
Serial Wire Debug (SWD) and JTAG boundary scan modes supported.
Micro Trace Buffer (MTB) supported.
Memory:
Up to 16 kB on-chip flash programming memory with 64 Byte page write and erase.
Up to 4 kB SRAM.
ROM API support:
Boot loader.
USART drivers.
I2C drivers.
Power profiles.
Flash In-Application Programming (IAP) and In-System Programming (ISP).
Digital peripherals:
High-speed GPIO interface connected to the ARM Cortex-M0+ IO bus with up to 18
General-Purpose I/O (GPIO) pins with configurable pull-up/pull-down resistors,
programmable open-drain mode, input inverter, and glitch filter.
High-current source output driver (20 mA) on four pins.
High-current sink driver (20 mA) on two true open-drain pins.
GPIO interrupt generation capability with boolean pattern-matching feature on eight
GPIO inputs.
Switch matrix for flexible configuration of each I/O pin function.
LPC81xM
32-bit ARM Cortex-M0+ microcontroller; up to 16 kB flash and
4 kB SRAM
Rev. 4.3 — 22 April 2014 Product data sheet
LPC81XM All information provided in this document is subject to legal disclaimers. © NXP Semiconductors N.V. 2014. All rights reserved.
Product data sheet Rev. 4.3 — 22 April 2014 2 of 76
NXP Semiconductors LPC81xM
32-bit ARM Cortex-M0+ microcontroller
State Configurable Timer/PWM (SCTimer/PWM) with input and output functions
(including capture and match) assigned to pins through the switch matrix.
Multiple-channel multi-rate timer (MRT) for repetitive interrupt generation at up to
four programmable, fixed rates.
Self Wake-up Timer (WKT) clocked from either the IRC or a low-power,
low-frequency internal oscillator.
CRC engine.
Windowed Watchdog timer (WWDT).
Analog peripherals:
Comparator with internal and external voltage references with pin functions
assigned or enabled through the switch matrix.
Serial interfaces:
Three USART interfaces with pin functions assigned through the switch matrix.
Two SPI controllers with pin functions assigned through the switch matrix.
One I2C-bus interface with pin functions assigned through the switch matrix.
Clock generation:
12 MHz internal RC oscillator trimmed to 1.5 % accuracy that can optionally be
used as a system clock.
Crystal oscillator with an operating range of 1 MHz to 25 MHz.
Programmable watchdog oscillator with a frequency range of 9.4 kHz to 2.3 MHz.
10 kHz low-power oscillator for the WKT.
PLL allows CPU operation up to the maximum CPU rate without the need for a
high-frequency crystal. May be run from the system oscillator, the external clock
input CLKIN, or the internal RC oscillator.
Clock output function with divider that can reflect the crystal oscillator, the main
clock, the IRC, or the watchdog oscillator.
Power control:
Integrated PMU (Power Management Unit) to minimize power consumption.
Reduced power modes: Sleep mode, Deep-sleep mode, Power-down mode, and
Deep power-down mode.
Wake-up from Deep-sleep and Power-down modes on activity on USART, SPI, and
I2C peripherals.
Timer-controlled self wake-up from Deep power-down mode.
Power-On Reset (POR).
Brownout detect.
Unique device serial number for identification.
Single power supply.
Operating temperature range 40 °C to 105 °C except for the DIP8 package, which is
available for a temperature range of 40 °C to 85 °C.
Available as DIP8, TSSOP16, SO20, TSSOP20, and XSON16 package.
3. Applications
8/16-bit applications Lighting
Consumer Motor control
Climate control Fire and security applications
LPC81XM All information provided in this document is subject to legal disclaimers. © NXP Semiconductors N.V. 2014. All rights reserved.
Product data sheet Rev. 4.3 — 22 April 2014 3 of 76
NXP Semiconductors LPC81xM
32-bit ARM Cortex-M0+ microcontroller
4. Ordering information
4.1 Ordering options
Table 1. Ordering information
Type number Package
Name Description Version
LPC810M021FN8 DIP8 plastic dual in-line package; 8 leads (300 mil) SOT097-2
LPC811M001JDH16 TSSOP16 plastic thin shrink small outline package; 16 leads; body width 4.4 mm SOT403-1
LPC812M101JDH16 TSSOP16 plastic thin shrink small outline package; 16 leads; body width 4.4 mm SOT403-1
LPC812M101JD20 SO20 plastic small outline package; 20 leads; body width 7.5 mm SOT163-1
LPC812M101JDH20 TSSOP20 plastic thin shrink small outline package; 20 leads; body width 4.4 mm SOT360-1
LPC812M101JTB16 XSON16 plastic extremely thin small outline package; no leads; 16 terminals;
body 2.5 3.2 0.5 mm
SOT1341-1
Table 2. Ordering options
Type number Flash/kB SRAM/kB USART I2C-bus SPI Comparator GPIO Package
LPC810M021FN8 4 1 2 1 1 1 6 DIP8
LPC811M001JDH16 8 2 2 1 1 1 14 TSSOP16
LPC812M101JDH16 16 4 3 1 2 1 14 TSSOP16
LPC812M101JD20 16 4 2 1 1 1 18 SO20
LPC812M101JDH20 16 4 3 1 2 1 18 TSSOP20
LPC812M101JTB16 16 4 3 1 2 1 14 XSON16
LPC81XM All information provided in this document is subject to legal disclaimers. © NXP Semiconductors N.V. 2014. All rights reserved.
Product data sheet Rev. 4.3 — 22 April 2014 4 of 76
NXP Semiconductors LPC81xM
32-bit ARM Cortex-M0+ microcontroller
5. Marking
The LPC81xM devices typically have the following top-side marking:
LPC81x
xxxxx
xxxxxxxx
xxYWWxR[x]
The last two letters in the last line (field ‘xR’) identify the boot code version and device
revision.
Field ‘Y’ states the year the device was manufactured. Field ‘WW’ states the week the
device was manufactured during that year.
Remark: On the TSSOP16 package, the last line includes only the date code xxYWW.
Table 3. Device revision table
Revision identifier (xR) Revision description
‘1A’ Initial device revision with boot code version 13.1
‘2A’ Device revision with boot code version 13.2
’4C’ Device revision with boot code version 13.4
LPC81XM All information provided in this document is subject to legal disclaimers. © NXP Semiconductors N.V. 2014. All rights reserved.
Product data sheet Rev. 4.3 — 22 April 2014 5 of 76
NXP Semiconductors LPC81xM
32-bit ARM Cortex-M0+ microcontroller
6. Block diagram
Fig 1. LPC81xM block diagram
LPC81XM All information provided in this document is subject to legal disclaimers. © NXP Semiconductors N.V. 2014. All rights reserved.
Product data sheet Rev. 4.3 — 22 April 2014 6 of 76
NXP Semiconductors LPC81xM
32-bit ARM Cortex-M0+ microcontroller
7. Pinning information
7.1 Pinning
Fig 2. Pin configuration DIP8 package (LPC810M021JN8)
Fig 3. Pin configuration TSSOP16 package (LPC811M001JDH16 and LPC812M101JDH16)
Fig 4. Pin configuration SO20 package (LPC812M101JD20)
LPC81XM All information provided in this document is subject to legal disclaimers. © NXP Semiconductors N.V. 2014. All rights reserved.
Product data sheet Rev. 4.3 — 22 April 2014 7 of 76
NXP Semiconductors LPC81xM
32-bit ARM Cortex-M0+ microcontroller
Fig 5. Pin configuration TSSOP20 package (LPC812M101JDH20)
Fig 6. Pin configuration XSON16 package (LPC812M101JTB16)
terminal 1
index area XSON16
16
aaa-009570
Transparent top view
15
14
13
12
11
10
9
1
2
3
4
5
6
7
8
PIO0_13
PIO0_12
RESET/PIO0_5
PIO0_4/WAKEUP/TRST
SWCLK/PIO0_3/TCK
SWDIO/PIO0_2/TMS
PIO0_11
PIO0_10
PIO0_0/ACMP_I1/TDO
PIO0_6/VDDCMP
PIO0_7
VSS
VDD
PIO0_8/XTALIN
PIO0_9/XTALOUT
PIO0_1/ACMP_I2/CLKIN/TDI
LPC81XM All information provided in this document is subject to legal disclaimers. © NXP Semiconductors N.V. 2014. All rights reserved.
Product data sheet Rev. 4.3 — 22 April 2014 8 of 76
NXP Semiconductors LPC81xM
32-bit ARM Cortex-M0+ microcontroller
7.2 Pin description
The pin description consists of two parts showing pin functions that are fixed to a certain
package pin (see Table 4) and showing pin functions that can be assigned to any pin on
the package through the switch matrix (see Table 5).
The pin description table in Table 4 shows the pin functions that are fixed to specific pins
on each package. These fixed-pin functions are selectable between GPIO and the
comparator inputs, SWD, RESET, and the XTAL pins. By default, the GPIO function is
selected except on pins PIO0_2, PIO0_3, and PIO0_5. JTAG functions are available in
boundary scan mode only.
Table 5 shows the the I2C, USART, SPI, and SCT pin functions, which can be assigned
through the switch matrix to any pin that is not power or ground in place of the pin’s fixed
functions.
The following exceptions apply:
For full I2C-bus compatibility, assign the I2C functions to the open-drain pins PIO0_11 and
PIO0_10.
Do not assign more than one output to any pin. However, more than one input can be
assigned to a pin. Once any function is assigned to a pin, the pin’s GPIO functionality is
disabled.
Pin PIO0_4 triggers a wake-up from Deep power-down mode. If you need to wake up
from Deep power-down mode via an external pin, do not assign any movable function to
this pin.
The JTAG functions TDO, TDI, TCK, TMS, and TRST are selected on pins PIO0_0 to
PIO0_4 by hardware when the part is in boundary scan mode.
Table 4. Pin description table (fixed pins)
Symbol
SO20/
TSSOP20
TSSOP16
XSON16
DIP8
Type Reset
state
[1]
Description
PIO0_0/ACMP_I1/
TDO
19 16 16 8 [5] I/O I; PU PIO0_0 — General purpose digital input/output port 0 pin 0.
In ISP mode, this is the USART0 receive pin U0_RXD.
In boundary scan mode: TDO (Test Data Out).
AI - ACMP_I1 — Analog comparator input 1.
PIO0_1/ACMP_I2/
CLKIN/TDI
12 9 9 5 [5] I/O I; PU PIO0_1 — General purpose digital input/output pin.
In boundary scan mode: TDI (Test Data In).
ISP entry pin on chip versions 1A and 2A and on the DIP8
package (see Table 6). For these chip versions and
packages, a LOW level on this pin during reset starts the
ISP command handler.
See PIO0_12 for all other packages.
AI - ACMP_I2 — Analog comparator input 2.
I - CLKIN — External clock input.
LPC81XM All information provided in this document is subject to legal disclaimers. © NXP Semiconductors N.V. 2014. All rights reserved.
Product data sheet Rev. 4.3 — 22 April 2014 9 of 76
NXP Semiconductors LPC81xM
32-bit ARM Cortex-M0+ microcontroller
SWDIO/PIO0_2/TMS 7 6 6 4 [2] I/O I; PU SWDIO — Serial Wire Debug I/O. SWDIO is enabled by
default on this pin.
In boundary scan mode: TMS (Test Mode Select).
I/O - PIO0_2 — General purpose digital input/output pin.
SWCLK/PIO0_3/
TCK
6 5 5 3 [2] I/O I; PU SWCLK — Serial Wire Clock. SWCLK is enabled by default
on this pin.
In boundary scan mode: TCK (Test Clock).
I/O - PIO0_3 — General purpose digital input/output pin.
PIO0_4/WAKEUP/
TRST
5 4 4 2 [6] I/O I; PU PIO0_4 — General purpose digital input/output pin.
In ISP mode, this is the USART0 transmit pin U0_TXD.
In boundary scan mode: TRST (Test Reset).
This pin triggers a wake-up from Deep power-down mode. If
you need to wake up from Deep power-down mode via an
external pin, do not assign any movable function to this pin.
This pin should be pulled HIGH externally before entering
Deep power-down mode. A LOW-going pulse as short as 50
ns causes the chip to exit Deep power-down mode and
wakes up the part.
RESET/PIO0_5 4 3 3 1 [4] I/O I; PU RESET — External reset input: A LOW-going pulse as short
as 50 ns on this pin resets the device, causing I/O ports and
peripherals to take on their default states, and processor
execution to begin at address 0.
In deep power-down mode, this pin must be pulled HIGH
externally. The RESET pin can be left unconnected or be
used as a GPIO or for any movable function if an external
RESET function is not needed and the Deep power-down
mode is not used.
I - PIO0_5 — General purpose digital input/output pin.
PIO0_6/VDDCMP 18 15 15 - [9] I/O I; PU PIO0_6 — General purpose digital input/output pin.
AI - VDDCMP — Alternate reference voltage for the analog
comparator.
PIO0_7 17 14 14 - [2] I/O I; PU PIO0_7 — General purpose digital input/output pin.
PIO0_8/XTALIN 14 11 11 - [8] I/O I; PU PIO0_8 — General purpose digital input/output pin.
I - XTALIN — Input to the oscillator circuit and internal clock
generator circuits. Input voltage must not exceed 1.95 V.
PIO0_9/XTALOUT 13 10 10 - [8] I/O I; PU PIO0_9 — General purpose digital input/output pin.
O - XTALOUT — Output from the oscillator circuit.
PIO0_10 9 8 8 - [3]I IA PIO0_10 — General purpose digital input/output pin. Assign
I2C functions to this pin when true open-drain pins are
needed for a signal compliant with the full I2C specification.
PIO0_11 8 7 7 - [3]I IA PIO0_11 — General purpose digital input/output pin. Assign
I2C functions to this pin when true open-drain pins are
needed for a signal compliant with the full I2C specification.
Table 4. Pin description table (fixed pins)
Symbol
SO20/
TSSOP20
TSSOP16
XSON16
DIP8
Type Reset
state
[1]
Description
LPC81XM All information provided in this document is subject to legal disclaimers. © NXP Semiconductors N.V. 2014. All rights reserved.
Product data sheet Rev. 4.3 — 22 April 2014 10 of 76
NXP Semiconductors LPC81xM
32-bit ARM Cortex-M0+ microcontroller
[1] Pin state at reset for default function: I = Input; AI = Analog Input; O = Output; PU = internal pull-up enabled (pins pulled up to full VDD
level); IA = inactive, no pull-up/down enabled.
[2] 5 V tolerant pad providing digital I/O functions with configurable pull-up/pull-down resistors and configurable hysteresis; includes
high-current output driver.
[3] True open-drain pin. I2C-bus pins compliant with the I2C-bus specification for I2C standard mode, I2C Fast-mode, and I2C Fast-mode
Plus. Do not use this pad for high-speed applications such as SPI or USART. The pin requires an external pull-up to provide output
functionality. When power is switched off, this pin is floating and does not disturb the I2C lines. Open-drain configuration applies to all
functions on this pin.
Remark: If this pin is not available on the package, prevent it from internally floating as follows: Set bits 10 and 11 in the GPIO DIR0
register to 1 to enable the output driver and write 1 to bits 10 and 11 in the GPIO CLR0 register to drive the outputs LOW internally.
[4] See Figure 11 for the reset pad configuration. RESET functionality is not available in Deep power-down mode. Use the WAKEUP pin to
reset the chip and wake up from Deep power-down mode. An external pull-up resistor is required on this pin for the Deep power-down
mode.
[5] 5 V tolerant pin providing standard digital I/O functions with configurable modes, configurable hysteresis, and analog input. When
configured as an analog input, the digital section of the pin is disabled, and the pin is not 5 V tolerant.
[6] 5 V tolerant pad providing digital I/O functions with configurable pull-up/pull-down resistors and configurable hysteresis. In Deep
power-down mode, pulling this pin LOW wakes up the chip. The wake-up pin function can be disabled and the pin can be used for other
purposes, if the WKT low power oscillator is enabled for waking up the part from Deep power-down mode.
[7] 5 V tolerant pad providing digital I/O functions with configurable pull-up/pull-down resistors and configurable hysteresis.
[8] 5 V tolerant pin providing standard digital I/O functions with configurable modes, configurable hysteresis, and analog I/O for the system
oscillator. When configured as an analog I/O, the digital section of the pin is disabled, and the pin is not 5 V tolerant.
[9] The digital part of this pin is 3 V tolerant pin due to special analog functionality. Pin provides standard digital I/O functions with
configurable modes, configurable hysteresis, and an analog input. When configured as an analog input, the digital section of the pin is
disabled.
PIO0_12 3 2 2 - [2] I/O I; PU PIO0_12 — General purpose digital input/output pin. ISP
entry pin on the SO20/TSSOP20/TSSOP16/XSON16
packages starting with chip version 4C (see Table 6). A
LOW level on this pin during reset starts the ISP command
handler.
See pin PIO0_1 for the DIP8 package and chip versions 1A
and 2A.
PIO0_13 2 1 1 - [2] I/O I; PU PIO0_13 — General purpose digital input/output pin.
PIO0_14 20 - - - [7] I/O I; PU PIO0_14 — General purpose digital input/output pin.
PIO0_15 11 - - - [7] I/O I; PU PIO0_15 — General purpose digital input/output pin.
PIO0_16 10 - - - [7] I/O I; PU PIO0_16 — General purpose digital input/output pin.
PIO0_17 1 - - - [7] I/O I; PU PIO0_17 — General purpose digital input/output pin.
VDD 15 12 12 6 - - 3.3 V supply voltage.
VSS 16 13 13 7 - - Ground.
Table 4. Pin description table (fixed pins)
Symbol
SO20/
TSSOP20
TSSOP16
XSON16
DIP8
Type Reset
state
[1]
Description
Table 5. Movable functions (assign to pins PIO0_0 to PIO_17 through switch matrix)
Function name Type Description
U0_TXD O Transmitter output for USART0.
U0_RXD I Receiver input for USART0.
U0_RTS O Request To Send output for USART0.
U0_CTS I Clear To Send input for USART0.
LPC81XM All information provided in this document is subject to legal disclaimers. © NXP Semiconductors N.V. 2014. All rights reserved.
Product data sheet Rev. 4.3 — 22 April 2014 11 of 76
NXP Semiconductors LPC81xM
32-bit ARM Cortex-M0+ microcontroller
U0_SCLK I/O Serial clock input/output for USART0 in synchronous mode.
U1_TXD O Transmitter output for USART1.
U1_RXD I Receiver input for USART1.
U1_RTS O Request To Send output for USART1.
U1_CTS I Clear To Send input for USART1.
U1_SCLK I/O Serial clock input/output for USART1 in synchronous mode.
U2_TXD O Transmitter output for USART2.
U2_RXD I Receiver input for USART2.
U2_RTS O Request To Send output for USART2.
U2_CTS I Clear To Send input for USART2.
U2_SCLK I/O Serial clock input/output for USART2 in synchronous mode.
SPI0_SCK I/O Serial clock for SPI0.
SPI0_MOSI I/O Master Out Slave In for SPI0.
SPI0_MISO I/O Master In Slave Out for SPI0.
SPI0_SSEL I/O Slave select for SPI0.
SPI1_SCK I/O Serial clock for SPI1.
SPI1_MOSI I/O Master Out Slave In for SPI1.
SPI1_MISO I/O Master In Slave Out for SPI1.
SPI1_SSEL I/O Slave select for SPI1.
CTIN_0 I SCT input 0.
CTIN_1 I SCT input 1.
CTIN_2 I SCT input 2.
CTIN_3 I SCT input 3.
CTOUT_0 O SCT output 0.
CTOUT_1 O SCT output 1.
CTOUT_2 O SCT output 2.
CTOUT_3 O SCT output 3.
I2C0_SCL I/O I2C-bus clock input/output (open-drain if assigned to pin PIO0_10).
High-current sink only if assigned to PIO0_10 and if I2C Fast-mode
Plus is selected in the I/O configuration register.
I2C0_SDA I/O I2C-bus data input/output (open-drain if assigned to pin PIO0_11).
High-current sink only if assigned to pin PIO0_11 and if I2C
Fast-mode Plus is selected in the I/O configuration register.
ACMP_O O Analog comparator digital output.
CLKOUT O Clock output.
GPIO_INT_BMAT O Output of the pattern match engine.
Table 5. Movable functions (assign to pins PIO0_0 to PIO_17 through switch matrix)
Function name Type Description
LPC81XM All information provided in this document is subject to legal disclaimers. © NXP Semiconductors N.V. 2014. All rights reserved.
Product data sheet Rev. 4.3 — 22 April 2014 12 of 76
NXP Semiconductors LPC81xM
32-bit ARM Cortex-M0+ microcontroller
Table 6. Pin location in ISP mode
ISP entry pin USART RXD USART TXD Marking Boot loader
version
Package
PIO0_1 PIO0_0 PIO0_4 1A v 13.1 TSSOP20; SO20;
TSSOP16; DIP8;
XSON16
PIO0_1 PIO0_0 PIO0_4 2A v 13.2 TSSOP20; SO20;
TSSOP16; DIP8;
XSON16
PIO0_1 PIO0_0 PIO0_4 4C and
later
v 13.4 and
later
DIP8
PIO0_12 PIO0_0 PIO0_4 4C and
later
v 13.4 and
later
TSSOP20; SO20;
TSSOP16;
XSON16
LPC81XM All information provided in this document is subject to legal disclaimers. © NXP Semiconductors N.V. 2014. All rights reserved.
Product data sheet Rev. 4.3 — 22 April 2014 13 of 76
NXP Semiconductors LPC81xM
32-bit ARM Cortex-M0+ microcontroller
8. Functional description
8.1 ARM Cortex-M0+ core
The ARM Cortex-M0+ core runs at an operating frequency of up to 30 MHz using a
two-stage pipeline. Integrated in the core are the NVIC and Serial Wire Debug with four
breakpoints and two watchpoints. The ARM Cortex-M0+ core supports a single-cycle I/O
enabled port for fast GPIO access.
The core includes a single-cycle multiplier and a system tick timer.
8.2 On-chip flash program memory
The LPC81xM contain up to 16 kB of on-chip flash program memory. The flash memory
supports a 64 Byte page size with page write and erase.
8.3 On-chip SRAM
The LPC81xM contain a total of up to 4 kB on-chip static RAM data memory.
8.4 On-chip ROM
The 8 kB on-chip ROM contains the boot loader and the following Application
Programming Interfaces (API):
• In-System Programming (ISP) and In-Application Programming (IAP) support for flash
programming
• Power profiles for configuring power consumption and PLL settings
• USART driver API routines
• I2C-bus driver API routines
8.5 Nested Vectored Interrupt Controller (NVIC)
The Nested Vectored Interrupt Controller (NVIC) is an integral part of the Cortex-M0+. The
tight coupling to the CPU allows for low interrupt latency and efficient processing of late
arriving interrupts.
8.5.1 Features
• Controls system exceptions and peripheral interrupts.
• On the LPC81xM, the NVIC supports 32 vectored interrupts including up to 8 external
interrupt inputs selectable from all GPIO pins.
• Four programmable interrupt priority levels with hardware priority level masking.
• Software interrupt generation using the ARM exceptions SVCall and PendSV.
• Relocatable interrupt vector table using vector table offset register.
8.5.2 Interrupt sources
Each peripheral device has one interrupt line connected to the NVIC but may have several
interrupt flags. Individual interrupt flags may also represent more than one interrupt
source.
LPC81XM All information provided in this document is subject to legal disclaimers. © NXP Semiconductors N.V. 2014. All rights reserved.
Product data sheet Rev. 4.3 — 22 April 2014 14 of 76
NXP Semiconductors LPC81xM
32-bit ARM Cortex-M0+ microcontroller
Up to eight pins, regardless of the selected function, can be programmed to generate an
interrupt on a level, a rising or falling edge, or both. The interrupt generating pins can be
selected from all digital or mixed digital/analog pins. The pin interrupt/pattern match block
controls the edge or level detection mechanism.
8.6 System tick timer
The ARM Cortex-M0+ includes a 24-bit system tick timer (SysTick) that is intended to
generate a dedicated SysTick exception at a fixed time interval (typically 10 ms).
8.7 Memory map
The LPC81xM incorporates several distinct memory regions. Figure 7 shows the overall
map of the entire address space from the user program viewpoint following reset. The
interrupt vector area supports address remapping.
The ARM private peripheral bus includes the ARM core registers for controlling the NVIC,
the system tick timer (SysTick), and the reduced power modes.
LPC81XM All information provided in this document is subject to legal disclaimers. © NXP Semiconductors N.V. 2014. All rights reserved.
Product data sheet Rev. 4.3 — 22 April 2014 15 of 76
NXP Semiconductors LPC81xM
32-bit ARM Cortex-M0+ microcontroller
8.8 I/O configuration
The IOCON block controls the configuration of the I/O pins. Each digital or mixed
digital/analog pin with the PIO0_n designator (except the true open-drain pins PIO0_10
and PIO0_11) in Table 4 can be configured as follows:
• Enable or disable the weak internal pull-up and pull-down resistors.
• Select a pseudo open-drain mode. The input cannot be pulled up above VDD. This pin
is not 5 V tolerant when VDD = 0.
Fig 7. LPC81xM Memory map
LPC81XM All information provided in this document is subject to legal disclaimers. © NXP Semiconductors N.V. 2014. All rights reserved.
Product data sheet Rev. 4.3 — 22 April 2014 16 of 76
NXP Semiconductors LPC81xM
32-bit ARM Cortex-M0+ microcontroller
• Program the input glitch filter with different filter constants using one of the IOCON
divided clock signals (IOCONCLKCDIV, see Figure 10 “LPC81xM clock generation”).
You can also bypass the glitch filter.
• Invert the input signal.
• Hysteresis can be enabled or disabled.
• For pins PIO0_10 and PIO0_11, select the I2C-mode and output driver for standard
digital operation, for I2C standard and fast modes, or for I2C Fast mode+.
• On mixed digital/analog pins, enable the analog input mode. Enabling the analog
mode disconnects the digital functionality.
Remark: The functionality of each I/O pin is flexible and is determined entirely through the
switch matrix. See Section 8.9 for details.
8.8.1 Standard I/O pad configuration
Figure 8 shows the possible pin modes for standard I/O pins with analog input function:
• Digital output driver with configurable open-drain output
• Digital input: Weak pull-up resistor (PMOS device) enabled/disabled
• Digital input: Weak pull-down resistor (NMOS device) enabled/disabled
• Digital input: Repeater mode enabled/disabled
• Digital input: Input glitch filter selectable on all pins
• Analog input
LPC81XM All information provided in this document is subject to legal disclaimers. © NXP Semiconductors N.V. 2014. All rights reserved.
Product data sheet Rev. 4.3 — 22 April 2014 17 of 76
NXP Semiconductors LPC81xM
32-bit ARM Cortex-M0+ microcontroller
8.9 Switch Matrix (SWM)
The switch matrix controls the function of each digital or mixed analog/digital pin in a
highly flexible way by allowing to connect many functions like the USART, SPI, SCT, and
I2C functions to any pin that is not power or ground. These functions are called movable
functions and are listed in Table 5.
Functions that need specialized pads like the oscillator pins XTALIN and XTALOUT can
be enabled or disabled through the switch matrix. These functions are called fixed-pin
functions and cannot move to other pins. The fixed-pin functions are listed in Table 4. If a
fixed-pin function is disabled, any other movable function can be assigned to this pin.
8.10 Fast General-Purpose parallel I/O (GPIO)
Device pins that are not connected to a specific peripheral function are controlled by the
GPIO registers. Pins may be dynamically configured as inputs or outputs. Multiple outputs
can be set or cleared in one write operation.
LPC81xM use accelerated GPIO functions:
• GPIO registers are located on the ARM Cortex M0+ IO bus for fastest possible
single-cycle I/O timing, allowing GPIO toggling with rates of up to 15 MHz.
Fig 8. Standard I/O pad configuration
LPC81XM All information provided in this document is subject to legal disclaimers. © NXP Semiconductors N.V. 2014. All rights reserved.
Product data sheet Rev. 4.3 — 22 April 2014 18 of 76
NXP Semiconductors LPC81xM
32-bit ARM Cortex-M0+ microcontroller
• An entire port value can be written in one instruction.
• Mask, set, and clear operations are supported for the entire port.
All GPIO port pins are fixed-pin functions that are enabled or disabled on the pins by the
switch matrix. Therefore each GPIO port pin is assigned to one specific pin and cannot be
moved to another pin. Except for pins SWDIO/PIO0_2, SWCLK/PIO0_3, and
RESET/PIO0_5, the switch matrix enables the GPIO port pin function by default.
8.10.1 Features
• Bit level port registers allow a single instruction to set and clear any number of bits in
one write operation.
• Direction control of individual bits.
• All I/O default to inputs with internal pull-up resistors enabled after reset - except for
the I2C-bus true open-drain pins PIO0_2 and PIO0_3.
• Pull-up/pull-down configuration, repeater, and open-drain modes can be programmed
through the IOCON block for each GPIO pin (see Figure 8).
•
8.11 Pin interrupt/pattern match engine
The pin interrupt block configures up to eight pins from all digital pins for providing eight
external interrupts connected to the NVIC.
The pattern match engine can be used, in conjunction with software, to create complex
state machines based on pin inputs.
Any digital pin, independently of the function selected through the switch matrix, can be
configured through the SYSCON block as input to the pin interrupt or pattern match
engine. The registers that control the pin interrupt or pattern match engine are located on
the IO+ bus for fast single-cycle access.
8.11.1 Features
• Pin interrupts
– Up to eight pins can be selected from all digital pins as edge- or level-sensitive
interrupt requests. Each request creates a separate interrupt in the NVIC.
– Edge-sensitive interrupt pins can interrupt on rising or falling edges or both.
– Level-sensitive interrupt pins can be HIGH- or LOW-active.
– Pin interrupts can wake up the LPC81xM from sleep mode, deep-sleep mode, and
power-down mode.
• Pin interrupt pattern match engine
– Up to eight pins can be selected from all digital pins to contribute to a boolean
expression. The boolean expression consists of specified levels and/or transitions
on various combinations of these pins.
– Each minterm (product term) comprising the specified boolean expression can
generate its own, dedicated interrupt request.
– Any occurrence of a pattern match can be programmed to also generate an RXEV
notification to the ARM CPU. The RXEV signal can be connected to a pin.
LPC81XM All information provided in this document is subject to legal disclaimers. © NXP Semiconductors N.V. 2014. All rights reserved.
Product data sheet Rev. 4.3 — 22 April 2014 19 of 76
NXP Semiconductors LPC81xM
32-bit ARM Cortex-M0+ microcontroller
– The pattern match engine does not facilitate wake-up.
8.12 USART0/1/2
Remark: USART0 and USART1 are available on all LPC800 parts. USART2 is available
on parts LPC812M101JDH16 and LPC812M101JDH20 only.
All USART functions are movable functions and are assigned to pins through the switch
matrix.
8.12.1 Features
• Maximum bit rates of 1.875 Mbit/s in asynchronous mode and 10 Mbit/s in
synchronous mode for USART functions connected to all digital pins except PIO0_10
and PIO0_11.
• 7, 8, or 9 data bits and 1 or 2 stop bits
• Synchronous mode with master or slave operation. Includes data phase selection and
continuous clock option.
• Multiprocessor/multidrop (9-bit) mode with software address compare. (RS-485
possible with software address detection and transceiver direction control.)
• Parity generation and checking: odd, even, or none.
• One transmit and one receive data buffer.
• RTS/CTS for hardware signaling for automatic flow control. Software flow control can
be performed using Delta CTS detect, Transmit Disable control, and any GPIO as an
RTS output.
• Received data and status can optionally be read from a single register
• Break generation and detection.
• Receive data is 2 of 3 sample "voting". Status flag set when one sample differs.
• Built-in Baud Rate Generator.
• A fractional rate divider is shared among all UARTs.
• Interrupts available for Receiver Ready, Transmitter Ready, Receiver Idle, change in
receiver break detect, Framing error, Parity error, Overrun, Underrun, Delta CTS
detect, and receiver sample noise detected.
• Separate data and flow control loopback modes for testing.
• Supported by on-chip ROM API.
8.13 SPI0/1
Remark: SPI0 is available on all LPC800 parts. SPI1 is available on parts
LPC812M101JDH16 and LPC812M101JDH20 only.
All SPI functions are movable functions and are assigned to pins through the switch
matrix.
8.13.1 Features
• Maximum data rates of 30 Mbit/s in master mode and 25 Mbit/s in slave mode for SPI
functions connected to all digital pins except PIO0_10 and PIO0_11.
LPC81XM All information provided in this document is subject to legal disclaimers. © NXP Semiconductors N.V. 2014. All rights reserved.
Product data sheet Rev. 4.3 — 22 April 2014 20 of 76
NXP Semiconductors LPC81xM
32-bit ARM Cortex-M0+ microcontroller
• Data frames of 1 to 16 bits supported directly. Larger frames supported by software.
• Master and slave operation.
• Data can be transmitted to a slave without the need to read incoming data. This can
be useful while setting up an SPI memory.
• Control information can optionally be written along with data. This allows very
versatile operation, including “any length” frames.
• One Slave Select input/output with selectable polarity and flexible usage.
Remark: Texas Instruments SSI and National Microwire modes are not supported.
8.14 I2C-bus interface
The I2C-bus is bidirectional for inter-IC control using only two wires: a serial clock line
(SCL) and a serial data line (SDA). Each device is recognized by a unique address and
can operate as either a receiver-only device (e.g., an LCD driver) or a transmitter with the
capability to both receive and send information (such as memory). Transmitters and/or
receivers can operate in either master or slave mode, depending on whether the chip has
to initiate a data transfer or is only addressed. The I2C is a multi-master bus and can be
controlled by more than one bus master connected to it.
The I2C-bus functions are movable functions and can be assigned through the switch
matrix to any pin. However, only the true open-drain PIO0_10 and PIO0_11 provide the
electrical characteristics to support the full I2C-bus specification (see Ref. 1).
8.14.1 Features
• Supports standard and fast mode with data rates of up to 400 kbit/s.
• Independent Master, Slave, and Monitor functions.
• Supports both Multi-master and Multi-master with Slave functions.
• Multiple I2C slave addresses supported in hardware.
• One slave address can be selectively qualified with a bit mask or an address range in
order to respond to multiple I2C bus addresses.
• 10-bit addressing supported with software assist.
• Supports SMBus.
• Supported by on-chip ROM API.
• If the I2C functions are connected to the true open-drain pins (PIO0_10 and
PIO0_11), the I2C supports the full I2C-bus specification:
– Fail-safe operation: When the power to an I2C-bus device is switched off, the SDA
and SCL pins connected to the I2C-bus are floating and do not disturb the bus.
– Supports Fast-mode Plus with bit rates up to 1 Mbit/s.
8.15 State-Configurable Timer/PWM (SCTimer/PWM)
The state configurable timer (SCTimer/PWM or SCT) can perform basic 16-bit and 32-bit
timer/counter functions with match outputs and external and internal capture inputs. In
addition, the SCTimer/PWM can employ up to two different programmable states, which
can change under the control of events, to provide complex timing patterns.
LPC81XM All information provided in this document is subject to legal disclaimers. © NXP Semiconductors N.V. 2014. All rights reserved.
Product data sheet Rev. 4.3 — 22 April 2014 21 of 76
NXP Semiconductors LPC81xM
32-bit ARM Cortex-M0+ microcontroller
All inputs and outputs of the SCTimer/PWM are movable functions and are assigned to
pins through the switch matrix.
8.15.1 Features
• Two 16-bit counters or one 32-bit counter.
• Counters clocked by bus clock or selected input.
• Up counters or up-down counters.
• State variable allows sequencing across multiple counter cycles.
• The following conditions define an event: a counter match condition, an input (or
output) condition, a combination of a match and/or and input/output condition in a
specified state, and the count direction.
• Events control outputs, interrupts, and the SCT states.
– Match register 0 can be used as an automatic limit.
– In bi-directional mode, events can be enabled based on the count direction.
– Match events can be held until another qualifying event occurs.
• Selected events can limit, halt, start, or stop a counter.
• Supports:
– 4 inputs
– 4 outputs
– 5 match/capture registers
– 6 events
– 2 states
8.16 Multi-Rate Timer (MRT)
The Multi-Rate Timer (MRT) provides a repetitive interrupt timer with four channels. Each
channel can be programmed with an independent time interval, and each channel
operates independently from the other channels.
8.16.1 Features
• 31-bit interrupt timer
• Four channels independently counting down from individually set values
• Bus stall, repeat and one-shot interrupt modes
8.17 Windowed WatchDog Timer (WWDT)
The watchdog timer resets the controller if software fails to periodically service it within a
programmable time window.
8.17.1 Features
• Internally resets chip if not periodically reloaded during the programmable time-out
period.
• Optional windowed operation requires reload to occur between a minimum and
maximum time period, both programmable.
LPC81XM All information provided in this document is subject to legal disclaimers. © NXP Semiconductors N.V. 2014. All rights reserved.
Product data sheet Rev. 4.3 — 22 April 2014 22 of 76
NXP Semiconductors LPC81xM
32-bit ARM Cortex-M0+ microcontroller
• Optional warning interrupt can be generated at a programmable time prior to
watchdog time-out.
• Enabled by software but requires a hardware reset or a watchdog reset/interrupt to be
disabled.
• Incorrect feed sequence causes reset or interrupt if enabled.
• Flag to indicate watchdog reset.
• Programmable 24-bit timer with internal prescaler.
• Selectable time period from (Tcy(WDCLK) 256 4) to (Tcy(WDCLK) 224 4) in
multiples of Tcy(WDCLK) 4.
• The Watchdog Clock (WDCLK)is generated by a the dedicated watchdog oscillator
(WDOSC).
8.18 Self Wake-up Timer (WKT)
The self wake-up timer is a 32-bit, loadable down-counter. Writing any non-zero value to
this timer automatically enables the counter and launches a count-down sequence. When
the counter is used as a wake-up timer, this write can occur just prior to entering a
reduced power mode.
8.18.1 Features
• 32-bit loadable down-counter. Counter starts automatically when a count value is
loaded. Time-out generates an interrupt/wake up request.
• The WKT resides in a separate, always-on power domain.
• The WKT supports two clock sources: the low-power oscillator and the IRC. The
low-power oscillator is located in the always-on power domain, so it can be used as
the clock source in Deep power-down mode.
• The WKT can be used for waking up the part from any reduced power mode,
including Deep power-down mode, or for general-purpose timing.
8.19 Analog comparator (ACMP)
The analog comparator with selectable hysteresis can compare voltage levels on external
pins and internal voltages.
After power-up and after switching the input channels of the comparator, the output of the
voltage ladder must be allowed to settle to its stable value before it can be used as a
comparator reference input. Settling times are given in Table 22.
The analog comparator output is a movable function and is assigned to a pin through the
switch matrix. The comparator inputs and the voltage reference are enabled or disabled
on pins PIO0_0 and PIO0_1 through the switch matrix.
LPC81XM All information provided in this document is subject to legal disclaimers. © NXP Semiconductors N.V. 2014. All rights reserved.
Product data sheet Rev. 4.3 — 22 April 2014 23 of 76
NXP Semiconductors LPC81xM
32-bit ARM Cortex-M0+ microcontroller
8.19.1 Features
• Selectable 0 mV, 10 mV ( 5 mV), and 20 mV ( 10 mV), 40 mV ( 20 mV) input
hysteresis.
• Two selectable external voltages (VDD or VDDCMP on pin PIO0_6); fully configurable
on either positive or negative input channel.
• Internal voltage reference from band gap selectable on either positive or negative
input channel.
• 32-stage voltage ladder with the internal reference voltage selectable on either the
positive or the negative input channel.
• Voltage ladder source voltage is selectable from an external pin or the main 3.3 V
supply voltage rail.
• Voltage ladder can be separately powered down for applications only requiring the
comparator function.
• Interrupt output is connected to NVIC.
• Comparator level output is connected to output pin ACMP_O.
• The comparator output can be routed internally to the SCT input through the switch
matrix.
Fig 9. Comparator block diagram
LPC81XM All information provided in this document is subject to legal disclaimers. © NXP Semiconductors N.V. 2014. All rights reserved.
Product data sheet Rev. 4.3 — 22 April 2014 24 of 76
NXP Semiconductors LPC81xM
32-bit ARM Cortex-M0+ microcontroller
8.20 Clocking and power control
8.20.1 Crystal and internal oscillators
The LPC81xM include four independent oscillators:
1. The crystal oscillator (SysOsc) operating at frequencies between 1 MHz and 25 MHz.
2. The internal RC Oscillator (IRC) with a fixed frequency of 12 MHz, trimmed to 1%
accuracy.
3. The internal low-power, low-frequency Oscillator with a nominal frequency of 10 kHz
with 40% accuracy for use with the self wake-up timer.
4. The dedicated Watchdog Oscillator (WDOsc) with a programmable nominal
frequency between 9.4 kHz and 2.3 MHz with 40% accuracy.
Fig 10. LPC81xM clock generation
LPC81XM All information provided in this document is subject to legal disclaimers. © NXP Semiconductors N.V. 2014. All rights reserved.
Product data sheet Rev. 4.3 — 22 April 2014 25 of 76
NXP Semiconductors LPC81xM
32-bit ARM Cortex-M0+ microcontroller
Each oscillator, except the low-frequency oscillator, can be used for more than one
purpose as required in a particular application.
Following reset, the LPC81xM will operate from the IRC until switched by software. This
allows systems to operate without any external crystal and the bootloader code to operate
at a known frequency.
See Figure 10 for an overview of the LPC81xM clock generation.
8.20.1.1 Internal RC Oscillator (IRC)
The IRC may be used as the clock source for the WWDT, and/or as the clock that drives
the PLL and subsequently the CPU. The nominal IRC frequency is 12 MHz. The IRC is
trimmed to 1.5 % accuracy over the entire voltage and temperature range.
The IRC can be used as a clock source for the CPU with or without using the PLL. The
IRC frequency can be boosted to a higher frequency, up to the maximum CPU operating
frequency, by the system PLL.
Upon power-up or any chip reset, the LPC81xM use the IRC as the clock source.
Software may later switch to one of the other available clock sources.
8.20.1.2 Crystal Oscillator (SysOsc)
The crystal oscillator can be used as the clock source for the CPU, with or without using
the PLL.
The SysOsc operates at frequencies of 1 MHz to 25 MHz. This frequency can be boosted
to a higher frequency, up to the maximum CPU operating frequency, by the system PLL.
8.20.1.3 Internal Low-power Oscillator and Watchdog Oscillator (WDOsc)
The nominal frequency of the WDOsc is programmable between 9.4 kHz and 2.3 MHz.
The frequency spread over silicon process variations is 40%.
The WDOsc is a dedicated oscillator for the windowed WWDT.
The internal low-power 10 kHz ( 40% accuracy) oscillator serves a the clock input to the
WKT. This oscillator can be configured to run in all low power modes.
8.20.2 Clock input
An external clock source can be supplied on the selected CLKIN pin. When selecting a
clock signal for the CLKIN pin, follow the specifications for digital I/O pins in Table 9 “Static
characteristics” and Table 15 “Dynamic characteristics: I/O pins[1]”.
An 1.8 V external clock source can be supplied on the XTALIN pins to the system
oscillator limiting the voltage of this signal ((see Section 14.2).
The maximum frequency for both clock signals is 25 MHz.
8.20.3 System PLL
The PLL accepts an input clock frequency in the range of 10 MHz to 25 MHz. The input
frequency is multiplied up to a high frequency with a Current Controlled Oscillator (CCO).
The multiplier can be an integer value from 1 to 32. The CCO operates in the range of
156 MHz to 320 MHz, so there is an additional divider in the loop to keep the CCO within
its frequency range while the PLL is providing the desired output frequency. The output
LPC81XM All information provided in this document is subject to legal disclaimers. © NXP Semiconductors N.V. 2014. All rights reserved.
Product data sheet Rev. 4.3 — 22 April 2014 26 of 76
NXP Semiconductors LPC81xM
32-bit ARM Cortex-M0+ microcontroller
divider may be set to divide by 2, 4, 8, or 16 to produce the output clock. Since the
minimum output divider value is 2, it is insured that the PLL output has a 50 % duty cycle.
The PLL is turned off and bypassed following a chip reset and may be enabled by
software. The program must configure and activate the PLL, wait for the PLL to lock, and
then connect to the PLL as a clock source. The PLL settling time is nominally 100 s.
8.20.4 Clock output
The LPC81xM features a clock output function that routes the IRC, the SysOsc, the
watchdog oscillator, or the main clock to the CLKOUT function. The CLKOUT function can
be connected to any digital pin through the switch matrix.
8.20.5 Wake-up process
The LPC81xM begin operation at power-up by using the IRC as the clock source. This
allows chip operation to resume quickly. If the SysOsc, the external clock source, or the
PLL is needed by the application, software must enable these features and wait for them
to stabilize before they are used as a clock source.
8.20.6 Power control
The LPC81xM supports the ARM Cortex-M0 Sleep mode. The CPU clock rate may also
be controlled as needed by changing clock sources, reconfiguring PLL values, and/or
altering the CPU clock divider value. This allows a trade-off of power versus processing
speed based on application requirements. In addition, a register is provided for shutting
down the clocks to individual on-chip peripherals, allowing to fine-tune power
consumption by eliminating all dynamic power use in any peripherals that are not required
for the application. Selected peripherals have their own clock divider which provides even
better power control.
8.20.6.1 Power profiles
The power consumption in Active and Sleep modes can be optimized for the application
through simple calls to the power profile API. The API is accessible through the on-chip
ROM.
The power configuration routine configures the LPC81xM for one of the following power
modes:
• Default mode corresponding to power configuration after reset.
• CPU performance mode corresponding to optimized processing capability.
• Efficiency mode corresponding to optimized balance of current consumption and CPU
performance.
• Low-current mode corresponding to lowest power consumption.
In addition, the power profile includes routines to select the optimal PLL settings for a
given system clock and PLL input clock.
8.20.6.2 Sleep mode
When Sleep mode is entered, the clock to the core is stopped. Resumption from the Sleep
mode does not need any special sequence but re-enabling the clock to the ARM core.
LPC81XM All information provided in this document is subject to legal disclaimers. © NXP Semiconductors N.V. 2014. All rights reserved.
Product data sheet Rev. 4.3 — 22 April 2014 27 of 76
NXP Semiconductors LPC81xM
32-bit ARM Cortex-M0+ microcontroller
In Sleep mode, execution of instructions is suspended until either a reset or interrupt
occurs. Peripheral functions continue operation during Sleep mode and may generate
interrupts to cause the processor to resume execution. Sleep mode eliminates dynamic
power used by the processor itself, memory systems and related controllers, and internal
buses.
8.20.6.3 Deep-sleep mode
In Deep-sleep mode, the LPC81xM is in Sleep-mode and all peripheral clocks and all
clock sources are off except for the IRC and watchdog oscillator or low-power oscillator if
selected. The IRC output is disabled. In addition all analog blocks are shut down and the
flash is in stand-by mode. In Deep-sleep mode, the application can keep the watchdog
oscillator and the BOD circuit running for self-timed wake-up and BOD protection.
The LPC81xM can wake up from Deep-sleep mode via a reset, digital pins selected as
inputs to the pin interrupt block, a watchdog timer interrupt, or an interrupt from the
USART (if the USART is configured in synchronous slave mode), the SPI, or the I2C
blocks (in slave mode).
Any interrupt used for waking up from Deep-sleep mode must be enabled in one of the
SYSCON wake-up enable registers and the NVIC.
Deep-sleep mode saves power and allows for short wake-up times.
8.20.6.4 Power-down mode
In Power-down mode, the LPC81xM is in Sleep-mode and all peripheral clocks and all
clock sources are off except for watchdog oscillator or low-power oscillator if selected. In
addition all analog blocks and the flash are shut down. In Power-down mode, the
application can keep the watchdog oscillator and the BOD circuit running for self-timed
wake-up and BOD protection.
The LPC81xM can wake up from Power-down mode via a reset, digital pins selected as
inputs to the pin interrupt block, a watchdog timer interrupt, or an interrupt from the
USART (if the USART is configured in synchronous slave mode), the SPI, or the I2C
blocks (in slave mode).
Any interrupt used for waking up from Power-down mode must be enabled in one of the
SYSCON wake-up enable registers and the NVIC.
Power-down mode reduces power consumption compared to Deep-sleep mode at the
expense of longer wake-up times.
8.20.6.5 Deep power-down mode
In Deep power-down mode, power is shut off to the entire chip except for the WAKEUP
pin and the self wake-up timer if enabled. Four general-purpose registers are available to
store information during Deep power-down mode. The LPC81xM can wake up from Deep
power-down mode via the WAKEUP pin, or without an external signal by using the
time-out of the self wake-up timer (see Section 8.18).
The LPC81xM can be prevented from entering Deep power-down mode by setting a lock
bit in the PMU block. Locking out Deep power-down mode enables the application to keep
the watchdog timer or the BOD running at all times.
LPC81XM All information provided in this document is subject to legal disclaimers. © NXP Semiconductors N.V. 2014. All rights reserved.
Product data sheet Rev. 4.3 — 22 April 2014 28 of 76
NXP Semiconductors LPC81xM
32-bit ARM Cortex-M0+ microcontroller
When entering Deep power-down mode, an external pull-up resistor is required on the
WAKEUP pin to hold it HIGH. Pull the RESET pin HIGH to prevent it from floating while in
Deep power-down mode.
8.21 System control
8.21.1 Reset
Reset has four sources on the LPC81xM: the RESET pin, the Watchdog reset, power-on
reset (POR), and the BrownOut Detection (BOD) circuit. The RESET pin is a Schmitt
trigger input pin. Assertion of chip reset by any source, once the operating voltage attains
a usable level, starts the IRC and initializes the flash controller.
A LOW-going pulse as short as 50 ns resets the part.
When the internal Reset is removed, the processor begins executing at address 0, which
is initially the Reset vector mapped from the boot block. At that point, all of the processor
and peripheral registers have been initialized to predetermined values.
In Deep power-down mode, an external pull-up resistor is required on the RESET pin.
8.21.2 Brownout detection
The LPC81xM includes up to four levels for monitoring the voltage on the VDD pin. If this
voltage falls below one of the selected levels, the BOD asserts an interrupt signal to the
NVIC. This signal can be enabled for interrupt in the Interrupt Enable Register in the NVIC
to cause a CPU interrupt. Alternatively, software can monitor the signal by reading a
dedicated status register. Four threshold levels can be selected to cause a forced reset of
the chip.
Fig 11. Reset pad configuration
LPC81XM All information provided in this document is subject to legal disclaimers. © NXP Semiconductors N.V. 2014. All rights reserved.
Product data sheet Rev. 4.3 — 22 April 2014 29 of 76
NXP Semiconductors LPC81xM
32-bit ARM Cortex-M0+ microcontroller
8.21.3 Code security (Code Read Protection - CRP)
CRP provides different levels of security in the system so that access to the on-chip flash
and use of the Serial Wire Debugger (SWD) and In-System Programming (ISP) can be
restricted. Programming a specific pattern into a dedicated flash location invokes CRP.
IAP commands are not affected by the CRP.
In addition, ISP entry via the ISP entry pin can be disabled without enabling CRP. For
details, see the LPC800 user manual.
There are three levels of Code Read Protection:
1. CRP1 disables access to the chip via the SWD and allows partial flash update
(excluding flash sector 0) using a limited set of the ISP commands. This mode is
useful when CRP is required and flash field updates are needed but all sectors cannot
be erased.
2. CRP2 disables access to the chip via the SWD and only allows full flash erase and
update using a reduced set of the ISP commands.
3. Running an application with level CRP3 selected, fully disables any access to the chip
via the SWD pins and the ISP. This mode effectively disables ISP override using the
ISP entry pin as well. If necessary, the application must provide a flash update
mechanism using IAP calls or using a call to the reinvoke ISP command to enable
flash update via the USART.
In addition to the three CRP levels, sampling of the ISP entry pin for valid user code can
be disabled. For details, see the LPC800 user manual.
8.21.4 APB interface
The APB peripherals are located on one APB bus.
8.21.5 AHBLite
The AHBLite connects the CPU bus of the ARM Cortex-M0+ to the flash memory, the
main static RAM, the CRC, and the ROM.
CAUTION
If level three Code Read Protection (CRP3) is selected, no future factory testing can be
performed on the device.
LPC81XM All information provided in this document is subject to legal disclaimers. © NXP Semiconductors N.V. 2014. All rights reserved.
Product data sheet Rev. 4.3 — 22 April 2014 30 of 76
NXP Semiconductors LPC81xM
32-bit ARM Cortex-M0+ microcontroller
8.22 Emulation and debugging
Debug functions are integrated into the ARM Cortex-M0+. Serial wire debug functions are
supported in addition to a standard JTAG boundary scan. The ARM Cortex-M0+ is
configured to support up to four breakpoints and two watch points.
The Micro Trace Buffer is implemented on the LPC81xM.
The RESET pin selects between the JTAG boundary scan (RESET = LOW) and the ARM
SWD debug (RESET = HIGH). The ARM SWD debug port is disabled while the LPC81xM
is in reset. The JTAG boundary scan pins are selected by hardware when the part is in
boundary scan mode on pins PIO0_0 to PIO0_3 (see Table 4).
To perform boundary scan testing, follow these steps:
1. Erase any user code residing in flash.
2. Power up the part with the RESET pin pulled HIGH externally.
3. Wait for at least 250 s.
4. Pull the RESET pin LOW externally.
5. Perform boundary scan operations.
6. Once the boundary scan operations are completed, assert the TRST pin to enable the
SWD debug mode, and release the RESET pin (pull HIGH).
Remark: The JTAG interface cannot be used for debug purposes.
Fig 12. Connecting the SWD pins to a standard SWD connector
LPC81XM All information provided in this document is subject to legal disclaimers. © NXP Semiconductors N.V. 2014. All rights reserved.
Product data sheet Rev. 4.3 — 22 April 2014 31 of 76
NXP Semiconductors LPC81xM
32-bit ARM Cortex-M0+ microcontroller
9. Limiting values
[1] The following applies to the limiting values:
a) This product includes circuitry specifically designed for the protection of its internal devices from the damaging effects of excessive
static charge. Nonetheless, it is suggested that conventional precautions be taken to avoid applying greater than the rated
maximum.
b) Parameters are valid over operating temperature range unless otherwise specified. All voltages are with respect to VSS unless
otherwise noted.
c) The limiting values are stress ratings only. Operating the part at these values is not recommended and proper operation is not
guaranteed. The conditions for functional operation are specified in Table 9.
[2] Maximum/minimum voltage above the maximum operating voltage (see Table 9) and below ground that can be applied for a short time
(< 10 ms) to a device without leading to irrecoverable failure. Failure includes the loss of reliability and shorter lifetime of the device.
[3] Including voltage on outputs in tri-state mode. Does not apply to pin PIO0_6.
[4] VDD present or not present. Compliant with the I2C-bus standard. 5.5 V can be applied to this pin when VDD is powered down.
[5] VDD present or not present.
[6] If the comparator is configured with the common mode input VIC = VDD, the other comparator input can be up to 0.2 V above or below
VDD without affecting the hysteresis range of the comparator function.
[7] It is recommended to connect an overvoltage protection diode between the analog input pin and the voltage supply pin.
[8] The maximum non-operating storage temperature is different than the temperature for required shelf life which should be determined
based on required shelf lifetime. Please refer to the JEDEC spec (J-STD-033B.1) for further details.
[9] Human body model: equivalent to discharging a 100 pF capacitor through a 1.5 k series resistor.
Table 7. Limiting values
In accordance with the Absolute Maximum Rating System (IEC 60134).[1]
Symbol Parameter Conditions Min Max Unit
VDD supply voltage (core and external rail) [2] 0.5 +4.6 V
VI input voltage 5 V tolerant I/O pins; VDD 1.8 V [3] 0.5 +5.5 V
5 V tolerant open-drain pins PIO0_10
and PIO0_11
[4] 0.5 +5.5 V
3 V tolerant I/O pin PIO0_6 [5] 0.5 +3.6 V
VIA analog input voltage [6]
[7]
0.5 4.6 V
Vi(xtal) crystal input voltage [2] 0.5 +2.5 V
IDD supply current per supply pin - 100 mA
ISS ground current per ground pin - 100 mA
Ilatch I/O latch-up current (0.5VDD) < VI < (1.5VDD);
Tj < 125 C
- 100 mA
Tstg storage temperature non-operating [8] 65 +150 C
Tj(max) maximum junction temperature - 150 C
Ptot(pack) total power dissipation (per package) based on package heat transfer, not
device power consumption
- 1.5 W
VESD electrostatic discharge voltage human body model; all pins [9] - 5500 V
charged device model; TSSOP20 and
SOP20 packages
- 1200 V
charged device model; TSSOP16
package
- 1000 V
charged device model; XSON16
package
- 800 V
LPC81XM All information provided in this document is subject to legal disclaimers. © NXP Semiconductors N.V. 2014. All rights reserved.
Product data sheet Rev. 4.3 — 22 April 2014 32 of 76
NXP Semiconductors LPC81xM
32-bit ARM Cortex-M0+ microcontroller
10. Thermal characteristics
The average chip junction temperature, Tj (C), can be calculated using the following
equation:
(1)
• Tamb = ambient temperature (C),
• Rth(j-a) = the package junction-to-ambient thermal resistance (C/W)
• PD = sum of internal and I/O power dissipation
The internal power dissipation is the product of IDD and VDD. The I/O power dissipation of
the I/O pins is often small and many times can be negligible. However it can be significant
in some applications.
Table 8. Thermal resistance
Symbol Parameter Conditions Max/Min Unit
DIP8
Rth(j-a) thermal resistance from
junction to ambient
JEDEC (4.5 in 4 in); still air 60 ± 15 % C/W
Single-layer (4.5 in 3 in); still air 81 ± 15 % C/W
Rth(j-c) thermal resistance from
junction to case
38 ± 15 % C/W
TSSOP16
Rth(j-a) thermal resistance from
junction to ambient
JEDEC (4.5 in 4 in); still air 133 ± 15 % C/W
Single-layer (4.5 in 3 in); still air 182 ± 15 % C/W
Rth(j-c) thermal resistance from
junction to case
33 ± 15 % C/W
TSSOP20
Rth(j-a) thermal resistance from
junction to ambient
JEDEC (4.5 in 4 in); still air 110 ± 15 % C/W
Single-layer (4.5 in 3 in); still air 153 ± 15 % C/W
Rth(j-c) thermal resistance from
junction to case
23 ± 15 % C/W
SO20
Rth(j-a) thermal resistance from
junction to ambient
JEDEC (4.5 in 4 in); still air 87 ± 15 % C/W
Single-layer (4.5 in 3 in); still air 112 ± 15 % C/W
Rth(j-c) thermal resistance from
junction to case
50 ± 15 % C/W
XSON16
Rth(j-a) thermal resistance from
junction to ambient
JEDEC (4.5 in 4 in); still air 92 ± 15 % C/W
Single-layer (4.5 in 3 in); still air 180 ± 15 % C/W
Rth(j-c) thermal resistance from
junction to case
27 ± 15 % C/W
Tj = Tamb + PD Rthj – a
LPC81XM All information provided in this document is subject to legal disclaimers. © NXP Semiconductors N.V. 2014. All rights reserved.
Product data sheet Rev. 4.3 — 22 April 2014 33 of 76
NXP Semiconductors LPC81xM
32-bit ARM Cortex-M0+ microcontroller
11. Static characteristics
Table 9. Static characteristics
Tamb = 40 C to +105 C, unless otherwise specified.
Symbol Parameter Conditions Min Typ[1] Max Unit
VDD supply voltage (core
and external rail)
1.8 3.3 3.6 V
IDD supply current Active mode; code
while(1){}
executed from flash;
system clock = 12 MHz; default
mode; VDD = 3.3 V
[2][3][4][5]- 1.4 - mA
system clock = 12 MHz;
low-current mode; VDD = 3.3 V
[2][3][4][5]
[6]
- 1.0 - mA
system clock = 24 MHz;
low-current mode; VDD = 3.3 V
[2][4][5][6]
[7]
- 2.2 - mA
system clock = 30 MHz; default
mode; VDD = 3.3 V
[2][4][5][8]- 3.3 - mA
system clock = 30 MHz;
low-current mode; VDD = 3.3 V
[2][4][5][6]
[8]
- 3 - mA
Sleep mode
system clock = 12 MHz; default
mode; VDD = 3.3 V
[2][3][4][5]- 0.8 - mA
system clock = 12 MHz;
low-current mode; VDD = 3.3 V
[2][3][4][5]
[6]
- 0.7 - mA
system clock = 24 MHz;
low-current mode; VDD = 3.3 V
[2][4][5][6]
[7]
- 1.3 - mA
system clock = 30 MHz; default
mode; VDD = 3.3 V
[2][4][5][8]- 1.8 - mA
system clock = 30 MHz;
low-current mode; VDD = 3.3 V
[2][4][5][6]
[8]
- 1.7 - mA
Deep-sleep mode
VDD = 3.3 V, Tamb = 25 °C [2][9] - 150 300 A
VDD = 3.3 V, Tamb = 105 °C [2][9] - - 400 A
Power-down mode
VDD = 3.3 V, Tamb = 25 °C [2][9]- 0.9 5 A
VDD = 3.3 V, Tamb = 105 °C [2][9]- - 40 A
Deep power-down mode;
Low-power oscillator and self
wakeup timer (WKT) disabled
VDD = 3.3 V, Tamb = 25 °C [10] - 170 1000 nA
VDD = 3.3 V, Tamb = 105 °C [10] - - 4 A
Deep power-down mode;
Low-power oscillator and self
wakeup timer (WKT) enabled
- 1 - A
LPC81XM All information provided in this document is subject to legal disclaimers. © NXP Semiconductors N.V. 2014. All rights reserved.
Product data sheet Rev. 4.3 — 22 April 2014 34 of 76
NXP Semiconductors LPC81xM
32-bit ARM Cortex-M0+ microcontroller
Standard port pins configured as digital pins, RESET; see Figure 13
IIL LOW-level input current VI = 0 V; on-chip pull-up resistor
disabled
- 0.5 10 nA
IIH HIGH-level input
current
VI = VDD; on-chip pull-down
resistor disabled
- 0.5 10 nA
IOZ OFF-state output
current
VO = 0 V; VO = VDD; on-chip
pull-up/down resistors disabled
- 0.5 10 nA
VI input voltage VDD 1.8 V; 5 V tolerant pins
except PIO0_6
[11]
[12]
0 - 5.0 V
VDD 1.8 V; on 3 V tolerant pin
PIO0_6
0 - 3.6
VDD = 0 V 0 - 3.6 V
VO output voltage output active 0 - VDD V
VIH HIGH-level input
voltage
0.7VDD - - V
VIL LOW-level input voltage - - 0.3VDD V
Vhys hysteresis voltage - 0.4 - V
VOH HIGH-level output
voltage
2.5 V VDD 3.6 V; IOH = 4 mA VDD 0.4 - - V
1.8 V VDD < 2.5 V; IOH = 3 mA VDD 0.4 - - V
VOL LOW-level output
voltage
2.5 V VDD 3.6 V; IOL = 4 mA - - 0.4 V
1.8 V VDD < 2.5 V; IOL = 3 mA - - 0.4 V
IOH HIGH-level output
current
VOH = VDD 0.4 V;
2.5 V VDD 3.6 V
4 - - mA
1.8 V VDD < 2.5 V 3 - - mA
IOL LOW-level output
current
VOL = 0.4 V
2.5 V VDD 3.6 V
4 - - mA
1.8 V VDD < 2.5 V 3 - - mA
IOHS HIGH-level short-circuit
output current
VOH = 0 V [13] - - 45 mA
IOLS LOW-level short-circuit
output current
VOL = VDD [13] - - 50 mA
Ipd pull-down current VI = 5 V 10 50 150 A
Ipu pull-up current VI = 0 V;
2.0 V VDD 3.6 V
15 50 85 A
1.8 V VDD < 2.0 V 10 50 85 A
VDD < VI < 5 V 0 0 0 A
High-drive output pins configured as digital pins (PIO0_2, PIO0_3, PIO0_7, PIO0_12, PIO0_13); see Figure 13
IIL LOW-level input current VI = 0 V; on-chip pull-up resistor
disabled
- 0.5 10 nA
IIH HIGH-level input
current
VI = VDD; on-chip pull-down
resistor disabled
- 0.5 10 nA
IOZ OFF-state output
current
VO = 0 V; VO = VDD; on-chip
pull-up/down resistors disabled
- 0.5 10 nA
Table 9. Static characteristics …continued
Tamb = 40 C to +105 C, unless otherwise specified.
Symbol Parameter Conditions Min Typ[1] Max Unit
LPC81XM All information provided in this document is subject to legal disclaimers. © NXP Semiconductors N.V. 2014. All rights reserved.
Product data sheet Rev. 4.3 — 22 April 2014 35 of 76
NXP Semiconductors LPC81xM
32-bit ARM Cortex-M0+ microcontroller
VI input voltage VDD 1.8 V [11]
[12]
0 - 5.0 V
VDD = 0 V 0 - 3.6 V
VO output voltage output active 0 - VDD V
VIH HIGH-level input
voltage
0.7VDD - - V
VIL LOW-level input voltage - - 0.3VDD V
Vhys hysteresis voltage 0.4 - - V
VOH HIGH-level output
voltage
2.5 V VDD 3.6 V; IOH = 20 mA VDD 0.4 - - V
1.8 V VDD < 2.5 V; IOH = 12 mA VDD 0.4 - - V
VOL LOW-level output
voltage
2.5 V VDD 3.6 V; IOL = 4 mA - - 0.4 V
1.8 V VDD < 2.5 V; IOL = 3 mA - - 0.4 V
IOH HIGH-level output
current
VOH = VDD 0.4 V;
2.5 V VDD 3.6 V
20 - - mA
1.8 V VDD < 2.5 V 12 - - mA
IOL LOW-level output
current
VOL = 0.4 V
2.5 V VDD 3.6 V
4 - - mA
1.8 V VDD < 2.5 V 3 - - mA
IOLS LOW-level short-circuit
output current
VOL = VDD [13] - - 50 mA
Ipd pull-down current VI = 5 V [14] 10 50 150 A
Ipu pull-up current VI = 0 V
2.0 V VDD 3.6 V
[14] 15 50 85 A
1.8 V VDD < 2.0 V 10 50 85 A
VDD < VI < 5 V 0 0 0 A
I2C-bus pins (PIO0_10 and PIO0_11); see Figure 13
VIH HIGH-level input
voltage
0.7VDD - - V
VIL LOW-level input voltage - - 0.3VDD V
Vhys hysteresis voltage - 0.05VDD - V
IOL LOW-level output
current
VOL = 0.4 V; I2C-bus pins
configured as standard mode pins
2.5 V VDD 3.6 V
3.5 - - mA
1.8 V VDD < 2.5 V 3 - -
IOL LOW-level output
current
VOL = 0.4 V; I2C-bus pins
configured as Fast-mode Plus
pins
2.5 V VDD 3.6 V
20 - - mA
1.8 V VDD < 2.5 V 16 - -
ILI input leakage current VI = VDD [15]- 2 4 A
VI = 5 V - 10 22 A
Table 9. Static characteristics …continued
Tamb = 40 C to +105 C, unless otherwise specified.
Symbol Parameter Conditions Min Typ[1] Max Unit
LPC81XM All information provided in this document is subject to legal disclaimers. © NXP Semiconductors N.V. 2014. All rights reserved.
Product data sheet Rev. 4.3 — 22 April 2014 36 of 76
NXP Semiconductors LPC81xM
32-bit ARM Cortex-M0+ microcontroller
[1] Typical ratings are not guaranteed. The values listed are for room temperature (25 C), nominal supply voltages.
[2] IDD measurements were performed with all pins configured as GPIO outputs driven LOW and pull-up resistors disabled.
[3] IRC enabled; system oscillator disabled; system PLL disabled.
[4] BOD disabled.
[5] All peripherals disabled in the SYSAHBCLKCTRL register. Peripheral clocks to USART, CLKOUT, and IOCON disabled in system
configuration block.
[6] Low-current mode PWR_LOW_CURRENT selected when running the set_power routine in the power profiles.
[7] IRC enabled; system oscillator disabled; system PLL enabled.
[8] IRC disabled; system oscillator enabled; system PLL enabled.
[9] All oscillators and analog blocks turned off in the PDSLEEPCFG register; PDSLEEPCFG = 0x0000 18FF.
[10] WAKEUP pin pulled HIGH externally.
[11] Including voltage on outputs in tri-state mode.
[12] 3-state outputs go into tri-state mode in Deep power-down mode.
[13] Allowed as long as the current limit does not exceed the maximum current allowed by the device.
[14] Pull-up and pull-down currents are measured across the weak internal pull-up/pull-down resistors. See Figure 8.
[15] To VSS.
Oscillator input pins (PIO0_8 and PIO0_9)
Vi(xtal) crystal input voltage 0.5 1.8 1.95 V
Vo(xtal) crystal output voltage 0.5 1.8 1.95 V
Table 9. Static characteristics …continued
Tamb = 40 C to +105 C, unless otherwise specified.
Symbol Parameter Conditions Min Typ[1] Max Unit
Fig 13. Pin input/output current measurement
LPC81XM All information provided in this document is subject to legal disclaimers. © NXP Semiconductors N.V. 2014. All rights reserved.
Product data sheet Rev. 4.3 — 22 April 2014 37 of 76
NXP Semiconductors LPC81xM
32-bit ARM Cortex-M0+ microcontroller
11.1 Power consumption
Power measurements in Active, Sleep, Deep-sleep,and Power-down modes were
performed under the following conditions:
• Configure all pins as GPIO with pull-up resistor disabled in the IOCON block.
• Configure GPIO pins as outputs using the GPIO DIR register.
• Write 1 to the GPIO CLR register to drive the outputs LOW.
Conditions: Tamb = 25 C; active mode entered executing code while(1){} from flash; all
peripherals disabled in the SYSAHBCLKCTRL register (SYSAHBCLKCTRL =0x1F); all peripheral
clocks disabled; internal pull-up resistors disabled; BOD disabled; low-current mode.
1 MHz - 6 MHz: IRC enabled; PLL disabled.
12 MHz: IRC enabled; PLL disabled.
24 MHz: IRC enabled; PLL enabled.
30 MHz: IRC disabled; SYSOSC enabled; PLL enabled.
Fig 14. Active mode: Typical supply current IDD versus supply voltage VDD
LPC81XM All information provided in this document is subject to legal disclaimers. © NXP Semiconductors N.V. 2014. All rights reserved.
Product data sheet Rev. 4.3 — 22 April 2014 38 of 76
NXP Semiconductors LPC81xM
32-bit ARM Cortex-M0+ microcontroller
Conditions: VDD = 3.3 V; active mode entered executing code while(1){} from flash; all
peripherals disabled in the SYSAHBCLKCTRL register (SYSAHBCLKCTRL = 0x1F); all peripheral
clocks disabled; internal pull-up resistors disabled; BOD disabled; low-current mode.
1 MHz - 6 MHz: IRC enabled; PLL disabled.
12 MHz: IRC enabled; PLL disabled.
24 MHz: IRC enabled; PLL enabled.
30 MHz: IRC disabled; SYSOSC enabled; PLL enabled.
Fig 15. Active mode: Typical supply current IDD versus temperature
LPC81XM All information provided in this document is subject to legal disclaimers. © NXP Semiconductors N.V. 2014. All rights reserved.
Product data sheet Rev. 4.3 — 22 April 2014 39 of 76
NXP Semiconductors LPC81xM
32-bit ARM Cortex-M0+ microcontroller
Conditions: VDD = 3.3 V; sleep mode entered from flash; all peripherals disabled in the
SYSAHBCLKCTRL register (SYSAHBCLKCTRL = 0x1F); all peripheral clocks disabled; internal
pull-up resistors disabled; BOD disabled; low-current mode.
1 MHz - 6 MHz: IRC enabled; PLL disabled.
12 MHz: IRC enabled; PLL disabled.
24 MHz: IRC enabled; PLL enabled.
30 MHz: IRC disabled; SYSOSC enabled; PLL enabled.
Fig 16. Sleep mode: Typical supply current IDD versus temperature for different system
clock frequencies
Conditions: BOD disabled; all oscillators and analog blocks disabled in the PDSLEEPCFG register
(PDSLEEPCFG = 0x0000 18FF).
Fig 17. Deep-sleep mode: Typical supply current IDD versus temperature for different
supply voltages VDD
LPC81XM All information provided in this document is subject to legal disclaimers. © NXP Semiconductors N.V. 2014. All rights reserved.
Product data sheet Rev. 4.3 — 22 April 2014 40 of 76
NXP Semiconductors LPC81xM
32-bit ARM Cortex-M0+ microcontroller
Conditions: BOD disabled; all oscillators and analog blocks disabled in the PDSLEEPCFG register
(PDSLEEPCFG = 0x0000 18FF).
Fig 18. Power-down mode: Typical supply current IDD versus temperature for different
supply voltages VDD
WKT not running.
Fig 19. Deep power-down mode: Typical supply current IDD versus temperature for
different supply voltages VDD
LPC81XM All information provided in this document is subject to legal disclaimers. © NXP Semiconductors N.V. 2014. All rights reserved.
Product data sheet Rev. 4.3 — 22 April 2014 41 of 76
NXP Semiconductors LPC81xM
32-bit ARM Cortex-M0+ microcontroller
11.2 CoreMark data
Conditions: VDD = 3.3 V; Tamb = 25 C; active mode; all peripherals except one UART and the SCT
disabled in the SYSAHBCLKCTRL register; system clock derived from the IRC; system oscillator
disabled; internal pull-up resistors enabled; BOD disabled. Measured with Keil uVision v.4.7.
Fig 20. Active mode: CoreMark power consumption IDD
Conditions: VDD = 3.3 V; active mode; all peripherals except one UART and the SCT disabled in
the SYSAHBCLKCTRL register; internal pull-up resistors enabled; BOD disabled. Measured with
Keil uVision v.4.7.
Fig 21. CoreMark score
LPC81XM All information provided in this document is subject to legal disclaimers. © NXP Semiconductors N.V. 2014. All rights reserved.
Product data sheet Rev. 4.3 — 22 April 2014 42 of 76
NXP Semiconductors LPC81xM
32-bit ARM Cortex-M0+ microcontroller
11.3 Peripheral power consumption
The supply current per peripheral is measured as the difference in supply current between
the peripheral block enabled and the peripheral block disabled in the SYSAHBCLKCFG
and PDRUNCFG (for analog blocks) registers. All other blocks are disabled in both
registers and no code is executed. Measured on a typical sample at Tamb = 25 C. Unless
noted otherwise, the system oscillator and PLL are running in both measurements.
The supply currents are shown for system clock frequencies of 12 MHz and 30 MHz.
Table 10. Power consumption for individual analog and digital blocks
Peripheral Typical supply current in mA Notes
n/a 12 MHz 30 MHz
IRC 0.21 - - System oscillator running; PLL off; independent
of main clock frequency.
System oscillator at 12 MHz 0.28 - - IRC running; PLL off; independent of main clock
frequency.
Watchdog oscillator at
500 kHz/2
0.002 - - System oscillator running; PLL off; independent
of main clock frequency.
BOD 0.05 - - Independent of main clock frequency.
Main PLL - 0.31 - -
CLKOUT - 0.06 0.09 Main clock divided by 4 in the CLKOUTDIV
register.
ROM - 0.08 0.19 -
I2C - 0.06 0.15 -
GPIO + pin interrupt/pattern
match
- 0.09 0.23 GPIO pins configured as outputs and set to
LOW. Direction and pin state are maintained if
the GPIO is disabled in the SYSAHBCLKCFG
register.
SWM - 0.03 0.07 -
SCT - 0.17 0.42 -
WKT - 0.01 0.03 -
MRT - 0.09 0.21 -
SPI0 - 0.05 0.13 -
SPI1 - 0.06 0.14 -
CRC - 0.03 0.07 -
USART0 - 0.04 0.10 -
USART1 - 0.04 0.11 -
USART2 - 0.04 0.10 -
WWDT - 0.04 0.10 Main clock selected as clock source for the
WDT.
IOCON - 0.03 0.08 -
Comparator - 0.04 0.09 -
LPC81XM All information provided in this document is subject to legal disclaimers. © NXP Semiconductors N.V. 2014. All rights reserved.
Product data sheet Rev. 4.3 — 22 April 2014 43 of 76
NXP Semiconductors LPC81xM
32-bit ARM Cortex-M0+ microcontroller
11.4 Electrical pin characteristics
Conditions: VDD = 3.3 V and VDD = 1.8 V; on pins PIO0_2, PIO0_3, PIO0_7, PIO0_12, PIO0_13.
Fig 22. High-drive output: Typical HIGH-level output voltage VOH versus HIGH-level
output current IOH
Conditions: VDD = 3.3 V and VDD = 1.8 V; on pins PIO0_10 and PIO0_11.
Fig 23. I2C-bus pins (high current sink): Typical LOW-level output current IOL versus
LOW-level output voltage VOL
LPC81XM All information provided in this document is subject to legal disclaimers. © NXP Semiconductors N.V. 2014. All rights reserved.
Product data sheet Rev. 4.3 — 22 April 2014 44 of 76
NXP Semiconductors LPC81xM
32-bit ARM Cortex-M0+ microcontroller
Conditions: VDD = 3.3 V and VDD = 1.8 V; standard port pins and high-drive pins PIO0_2, PIO0_3,
PIO0_7, PIO0_12, PIO0_13.
Fig 24. Typical LOW-level output current IOL versus LOW-level output voltage VOL
Conditions: VDD = 3.3 V and VDD = 1.8 V; standard port pins.
Fig 25. Typical HIGH-level output voltage VOH versus HIGH-level output source current
IOH
LPC81XM All information provided in this document is subject to legal disclaimers. © NXP Semiconductors N.V. 2014. All rights reserved.
Product data sheet Rev. 4.3 — 22 April 2014 45 of 76
NXP Semiconductors LPC81xM
32-bit ARM Cortex-M0+ microcontroller
Conditions: VDD = 3.3 V and VDD = 1.8 V; standard port pins.
Fig 26. Typical pull-up current Ipu versus input voltage VI
Conditions: VDD = 3.3 V and VDD = 1.8 V; standard port pins.
Fig 27. Typical pull-down current Ipd versus input voltage VI
LPC81XM All information provided in this document is subject to legal disclaimers. © NXP Semiconductors N.V. 2014. All rights reserved.
Product data sheet Rev. 4.3 — 22 April 2014 46 of 76
NXP Semiconductors LPC81xM
32-bit ARM Cortex-M0+ microcontroller
12. Dynamic characteristics
12.1 Flash memory
[1] Number of program/erase cycles.
[2] Programming times are given for writing 64 bytes to the flash. Tamb +85 C. Flash programming with IAP
calls (see LPC800 user manual).
12.2 External clock for the oscillator in slave mode
Remark: The input voltage on the XTAL1/2 pins must be 1.95 V (see Table 9). For
connecting the oscillator to the XTAL pins, also see Section 14.2.
[1] Parameters are valid over operating temperature range unless otherwise specified.
[2] Typical ratings are not guaranteed. The values listed are for room temperature (25 C), nominal supply
voltages.
Table 11. Flash characteristics
Tamb = 40 C to +105 C. Based on JEDEC NVM qualification. Failure rate < 10 ppm for parts as
specified below.
Symbol Parameter Conditions Min Typ Max Unit
Nendu endurance [1] 10000 100000 - cycles
tret retention time powered 10 20 - years
unpowered 20 40 - years
ter erase time page or multiple
consecutive pages,
sector or multiple
consecutive
sectors
95 100 105 ms
tprog programming
time
[2] 0.95 1 1.05 ms
Table 12. Dynamic characteristic: external clock (XTALIN inputs)
Tamb = 40 C to +105 C; VDD over specified ranges.[1]
Symbol Parameter Conditions Min Typ[2] Max Unit
fosc oscillator frequency 1 - 25 MHz
Tcy(clk) clock cycle time 40 - 1000 ns
tCHCX clock HIGH time Tcy(clk) 0.4 - - ns
tCLCX clock LOW time Tcy(clk) 0.4 - - ns
tCLCH clock rise time - - 5 ns
tCHCL clock fall time - - 5 ns
Fig 28. External clock timing (with an amplitude of at least Vi(RMS) = 200 mV)
LPC81XM All information provided in this document is subject to legal disclaimers. © NXP Semiconductors N.V. 2014. All rights reserved.
Product data sheet Rev. 4.3 — 22 April 2014 47 of 76
NXP Semiconductors LPC81xM
32-bit ARM Cortex-M0+ microcontroller
12.3 Internal oscillators
[1] Parameters are valid over operating temperature range unless otherwise specified.
[2] Typical ratings are not guaranteed. The values listed are for room temperature (25 C), nominal supply
voltages.
[1] Typical ratings are not guaranteed. The values listed are at nominal supply voltages.
[2] The typical frequency spread over processing and temperature (Tamb = 40 C to +105 C) is 40 %.
[3] See the LPC81xM user manual.
Table 13. Dynamic characteristics: IRC
Tamb = 40 C to +105 C; 2.7 V VDD 3.6 V[1].
Symbol Parameter Conditions Min Typ[2] Max Unit
fosc(RC) internal RC oscillator
frequency
Tamb = 40 C to
+105 C
11.82 12 12.18 MHz
Conditions: Frequency values are typical values. 12 MHz 1.5 % accuracy is guaranteed for
2.7 V VDD 3.6 V and Tamb = 40 C to +105 C. Variations between parts may cause the IRC to
fall outside the 12 MHz 1.5 % accuracy specification for voltages below 2.7 V.
Fig 29. Typical Internal RC oscillator frequency versus temperature
Table 14. Dynamic characteristics: Watchdog oscillator
Symbol Parameter Conditions Min Typ[1] Max Unit
fosc(int) internal oscillator
frequency
DIVSEL = 0x1F, FREQSEL = 0x1
in the WDTOSCCTRL register;
[2][3]- 9.4 - kHz
DIVSEL = 0x00, FREQSEL = 0xF
in the WDTOSCCTRL register
[2][3] - 2300 - kHz
LPC81XM All information provided in this document is subject to legal disclaimers. © NXP Semiconductors N.V. 2014. All rights reserved.
Product data sheet Rev. 4.3 — 22 April 2014 48 of 76
NXP Semiconductors LPC81xM
32-bit ARM Cortex-M0+ microcontroller
12.4 I/O pins
[1] Applies to standard port pins and RESET pin.
12.5 I2C-bus
[1] See the I2C-bus specification UM10204 for details.
[2] Parameters are valid over operating temperature range unless otherwise specified.
Table 15. Dynamic characteristics: I/O pins[1]
Tamb = 40 C to +105 C; 3.0 V VDD 3.6 V.
Symbol Parameter Conditions Min Typ Max Unit
tr rise time pin configured as output 3.0 - 5.0 ns
tf fall time pin configured as output 2.5 - 5.0 ns
Table 16. Dynamic characteristic: I2C-bus pins[1]
Tamb = 40 C to +105 C.[2]
Symbol Parameter Conditions Min Max Unit
fSCL SCL clock
frequency
Standard-mode 0 100 kHz
Fast-mode 0 400 kHz
Fast-mode Plus; on
pins PIO0_10 and
PIO0_11
0 1 MHz
tf fall time [4][5][6][7] of both SDA and
SCL signals
Standard-mode
- 300 ns
Fast-mode 20 + 0.1 Cb 300 ns
Fast-mode Plus;
on pins PIO0_10
and PIO0_11
- 120 ns
tLOW LOW period of
the SCL clock
Standard-mode 4.7 - s
Fast-mode 1.3 - s
Fast-mode Plus; on
pins PIO0_10 and
PIO0_11
0.5 - s
tHIGH HIGH period of
the SCL clock
Standard-mode 4.0 - s
Fast-mode 0.6 - s
Fast-mode Plus; on
pins PIO0_10 and
PIO0_11
0.26 - s
tHD;DAT data hold time [3][4][8] Standard-mode 0 - s
Fast-mode 0 - s
Fast-mode Plus; on
pins PIO0_10 and
PIO0_11
0 - s
tSU;DAT data set-up
time
[9][10] Standard-mode 250 - ns
Fast-mode 100 - ns
Fast-mode Plus; on
pins PIO0_10 and
PIO0_11
50 - ns
LPC81XM All information provided in this document is subject to legal disclaimers. © NXP Semiconductors N.V. 2014. All rights reserved.
Product data sheet Rev. 4.3 — 22 April 2014 49 of 76
NXP Semiconductors LPC81xM
32-bit ARM Cortex-M0+ microcontroller
[3] tHD;DAT is the data hold time that is measured from the falling edge of SCL; applies to data in transmission
and the acknowledge.
[4] A device must internally provide a hold time of at least 300 ns for the SDA signal (with respect to the
VIH(min) of the SCL signal) to bridge the undefined region of the falling edge of SCL.
[5] Cb = total capacitance of one bus line in pF.
[6] The maximum tf for the SDA and SCL bus lines is specified at 300 ns. The maximum fall time for the SDA
output stage tf is specified at 250 ns. This allows series protection resistors to be connected in between the
SDA and the SCL pins and the SDA/SCL bus lines without exceeding the maximum specified tf.
[7] In Fast-mode Plus, fall time is specified the same for both output stage and bus timing. If series resistors
are used, designers should allow for this when considering bus timing.
[8] The maximum tHD;DAT could be 3.45 s and 0.9 s for Standard-mode and Fast-mode but must be less than
the maximum of tVD;DAT or tVD;ACK by a transition time (see UM10204). This maximum must only be met if
the device does not stretch the LOW period (tLOW) of the SCL signal. If the clock stretches the SCL, the
data must be valid by the set-up time before it releases the clock.
[9] tSU;DAT is the data set-up time that is measured with respect to the rising edge of SCL; applies to data in
transmission and the acknowledge.
[10] A Fast-mode I2C-bus device can be used in a Standard-mode I2C-bus system but the requirement
tSU;DAT = 250 ns must then be met. This will automatically be the case if the device does not stretch the
LOW period of the SCL signal. If such a device does stretch the LOW period of the SCL signal, it must
output the next data bit to the SDA line tr(max) + tSU;DAT = 1000 + 250 = 1250 ns (according to the
Standard-mode I2C-bus specification) before the SCL line is released. Also the acknowledge timing must
meet this set-up time.
Fig 30. I2C-bus pins clock timing
LPC81XM All information provided in this document is subject to legal disclaimers. © NXP Semiconductors N.V. 2014. All rights reserved.
Product data sheet Rev. 4.3 — 22 April 2014 50 of 76
NXP Semiconductors LPC81xM
32-bit ARM Cortex-M0+ microcontroller
12.6 SPI interfaces
The maximum data bit rate is 30 Mbit/s in master mode and 25 Mbit/s in slave mode.
Remark: SPI functions can be assigned to all digital pins. The characteristics are valid for
all digital pins except the open-drain pins PIO0_10 and PIO0_11.
[1] Capacitance on pin SPIn_SCK CSCK < 5 pF.
[2] Tcy(clk) = DIVVAL/CCLK with CCLK = system clock frequency. DIVVAL is the SPI clock divider. See the
LPC800 User manual UM10601.
Table 17. SPI dynamic characteristics
Tamb = 40 C to 105 C; 1.8 V VDD 3.6 V. Simulated parameters sampled at the 50 % level of
the rising or falling edge; values guaranteed by design.
Symbol Parameter Conditions Min Max Unit
SPI master[1]
Tcy(clk) clock cycle time [2] 33 - ns
tDS data set-up time 0 - ns
tDH data hold time 16 - ns
tv(Q) data output valid time CL = 10 pF - 0.5 ns
th(Q) data output hold time CL = 10 pF 0.5 - ns
SPI slave
Tcy(clk) 40 ns
tDS data set-up time 0 - ns
tDH data hold time 16 - ns
tv(Q) data output valid time CL = 10 pF - 10 ns
th(Q) data output hold time CL = 10 pF 10 - ns
LPC81XM All information provided in this document is subject to legal disclaimers. © NXP Semiconductors N.V. 2014. All rights reserved.
Product data sheet Rev. 4.3 — 22 April 2014 51 of 76
NXP Semiconductors LPC81xM
32-bit ARM Cortex-M0+ microcontroller
Pin names SCK, MISO, and MOSI refer to pins for both SPI peripherals, SPI0 and SPI1.
Fig 31. SPI master timing
LPC81XM All information provided in this document is subject to legal disclaimers. © NXP Semiconductors N.V. 2014. All rights reserved.
Product data sheet Rev. 4.3 — 22 April 2014 52 of 76
NXP Semiconductors LPC81xM
32-bit ARM Cortex-M0+ microcontroller
Pin names SCK, MISO, and MOSI refer to pins for both SPI peripherals, SPI0 and SPI1.
Fig 32. SPI slave timing
LPC81XM All information provided in this document is subject to legal disclaimers. © NXP Semiconductors N.V. 2014. All rights reserved.
Product data sheet Rev. 4.3 — 22 April 2014 53 of 76
NXP Semiconductors LPC81xM
32-bit ARM Cortex-M0+ microcontroller
12.7 USART interface
The maximum USART bit rate is 1.875 Mbit/s in asynchronous mode and 10 Mbit/s in
synchronous mode slave and master mode.
Remark: USART functions can be assigned to all digital pins. The characteristics are valid
for all digital pins except the open-drain pins PIO0_10 and PIO0_11.
[1] Typical ratings are not guaranteed. The values listed are for room temperature (25 C), VDD = 3.3 V, typical
samples.
[2] Tcy(clk) = U_PCLK/BRGVAL. See the LPC800 User manual UM10601.
[3] Capacitance on pin Un_SCLK CSCLK < 5 pF.
Table 18. USART dynamic characteristics
Tamb = 40 C to 105 C; 1.8 V VDD 3.6 V. Simulated parameters sampled at the 50 % level of
the falling or rising edge; values guaranteed by design.
Symbol Parameter Conditions Min Max Unit
Tcy(clk) clock cycle time [2] 100 - ns
USART master (in synchronous mode)[3]
tsu(D) data input set-up
time
44 - ns
th(D) data input hold time 0 - ns
tv(Q) data output valid time - -8 ns
th(Q) data output hold time -8 - ns
USART slave (in synchronous mode)
tsu(D) data input set-up
time
5 - ns
th(D) data input hold time 0 - ns
tv(Q) data output valid time CL = 10 pF - 40 ns
th(Q) data output hold time CL = 10 pF 40 - ns
Fig 33. USART timing
LPC81XM All information provided in this document is subject to legal disclaimers. © NXP Semiconductors N.V. 2014. All rights reserved.
Product data sheet Rev. 4.3 — 22 April 2014 54 of 76
NXP Semiconductors LPC81xM
32-bit ARM Cortex-M0+ microcontroller
13. Analog characteristics
13.1 BOD
[1] Interrupt levels are selected by writing the level value to the BOD control register BODCTRL.
[2] Typical ratings are not guaranteed. The values listed are for room temperature (25 C), VDD = 3.3 V, typical
samples.
13.2 Internal voltage reference
[1] Characterized through simulation.
[2] Characterized on a typical silicon sample.
Table 19. BOD static characteristics[1]
Tamb = 40 C to +105 C.
Symbol Parameter Conditions Typ[2] Unit
Vth threshold voltage interrupt level 1
assertion 2.3 V
de-assertion 2.4 V
interrupt level 2
assertion 2.6 V
de-assertion 2.7 V
interrupt level 3
assertion 2.8 V
de-assertion 2.9 V
reset level 1
assertion 2.1 V
de-assertion 2.2 V
reset level 2
assertion 2.4 V
de-assertion 2.5 V
reset level 3
assertion 2.6 V
de-assertion 2.8 V
Table 20. Internal voltage reference static and dynamic characteristics
Symbol Parameter Conditions Min Typ Max Unit
VO output voltage Tamb = 40 C to +105 C [1] 0.855 0.900 0.945 V
Tamb = 70 C to 105 C [2] - 0.906 - V
Tamb = 50 C [2] - 0.905 - V
Tamb = 25 C [4] 0.893 0.903 0.913 V
Tamb = 0 C [2] - 0.902 - V
Tamb = 20 C [2] - 0.899 - V
Tamb = 40 C [2] - 0.896 - V
ts(pu) power-up
settling time
to 99% of VO [3] - 155 195 s
LPC81XM All information provided in this document is subject to legal disclaimers. © NXP Semiconductors N.V. 2014. All rights reserved.
Product data sheet Rev. 4.3 — 22 April 2014 55 of 76
NXP Semiconductors LPC81xM
32-bit ARM Cortex-M0+ microcontroller
[3] Typical values are derived from nominal simulation (VDD = 3.3 V; Tamb = 27 C; nominal process models).
Maximum values are derived from worst case simulation (VDD = 2.6 V; Tamb = 105 C; slow process
models).
[4] Maximum and minimum values are measured on samples from the corners of the process matrix lot.
13.3 Comparator
VDD = 3.3 V
Fig 34. Typical internal voltage reference output voltage
Table 21. Comparator characteristics
VDD = 3.0 V and Tamb = 27 C unless noted otherwise.
Symbol Parameter Conditions Min Typ Max Unit
Static characteristics
Vref(cmp) comparator reference
voltage
pin PIO0_6/VDDCMP configured for
function VDDCMP
1.5 - 3.6 V
IDD supply current - 55 - A
VIC common-mode input
voltage
0 - VDD V
DVO output voltage variation 0 - VDD V
Voffset offset voltage VIC = 0.1 V - 1.9 - mV
VIC = 1.5 V - 2.1 - mV
VIC = 2.8 V - 2.0 mV
Dynamic characteristics
tstartup start-up time nominal process - 4 - s
LPC81XM All information provided in this document is subject to legal disclaimers. © NXP Semiconductors N.V. 2014. All rights reserved.
Product data sheet Rev. 4.3 — 22 April 2014 56 of 76
NXP Semiconductors LPC81xM
32-bit ARM Cortex-M0+ microcontroller
[1] CL = 10 pF; results from measurements on silicon samples over process corners and over the full temperature range Tamb = 40 C to
+105 C. Typical data are for Tamb = 27 C.
[2] Input hysteresis is relative to the reference input channel and is software programmable to three levels.
[1] Maximum values are derived from worst case simulation (VDD = 2.6 V; Tamb = 105 C; slow process
models).
[2] Settling time applies to switching between comparator channels.
tPD propagation delay HIGH to LOW; VDD = 3.0 V;
VIC = 0.1 V; 50 mV overdrive input [1]
- 109 121
ns
VIC = 0.1 V; rail-to-rail input [1] - 155 164 ns
VIC = 1.5 V; 50 mV overdrive input [1] - 95 105 ns
VIC = 1.5 V; rail-to-rail input [1] - 101 108 ns
VIC = 2.9 V; 50 mV overdrive input [1] - 122 129 ns
VIC = 2.9 V; rail-to-rail input [1] - 74 82 ns
tPD propagation delay LOW to HIGH; VDD = 3.0 V;
VIC = 0.1 V; 50 mV overdrive input [1]
- 246 260
ns
VIC = 0.1 V; rail-to-rail input [1] - 57 59 ns
VIC = 1.5 V; 50 mV overdrive input [1] - 218 ns
VIC = 1.5 V; rail-to-rail input [1] - 146 155 ns
VIC = 2.9 V; 50 mV overdrive input [1] - 184 206 ns
VIC = 2.9 V; rail-to-rail input [1] - 250 286 ns
Vhys hysteresis voltage positive hysteresis; VDD = 3.0 V;
VIC = 1.5 V
[2] - 6, 11, 21 - mV
Vhys hysteresis voltage negative hysteresis; VDD = 3.0 V;
VIC = 1.5 V
[2][2] - 4, 9, 19 - mV
Rlad ladder resistance - - 1.034 - M
Table 21. Comparator characteristics …continued
VDD = 3.0 V and Tamb = 27 C unless noted otherwise.
Symbol Parameter Conditions Min Typ Max Unit
Table 22. Comparator voltage ladder dynamic characteristics
Symbol Parameter Conditions Min Typ Max Unit
ts(pu) power-up settling
time
to 99% of voltage
ladder output
value
[1]- - 30 s
ts(sw) switching settling
time
to 99% of voltage
ladder output
value
[1]
[2]
- - 15 s
LPC81XM All information provided in this document is subject to legal disclaimers. © NXP Semiconductors N.V. 2014. All rights reserved.
Product data sheet Rev. 4.3 — 22 April 2014 57 of 76
NXP Semiconductors LPC81xM
32-bit ARM Cortex-M0+ microcontroller
[1] Measured over a polyresistor matrix lot with a 2 kHz input signal and overdrive < 100 V.
[2] All peripherals except comparator and IRC turned off.
Table 23. Comparator voltage ladder reference static characteristics
VDD = 3.3 V; Tamb = 40 C to + 105C.
Symbol Parameter Conditions Min Typ Max[1] Unit
EV(O) output voltage error Internal VDD supply
decimal code = 00 [2]- 0 0 %
decimal code = 08 - 0 0.4 %
decimal code = 16 - 0.2 0.2 %
decimal code = 24 - 0.2 0.2 %
decimal code = 30 - 0.1 0.1 %
decimal code = 31 - 0.1 0.1 %
EV(O) output voltage error External VDDCMP
supply
decimal code = 00 - 0 0 %
decimal code = 08 - 0.1 0.5 %
decimal code = 16 - 0.2 0.4 %
decimal code = 24 - 0.2 0.3 %
decimal code = 30 - 0.2 0.2 %
decimal code = 31 - 0.1 0.1 %
LPC81XM All information provided in this document is subject to legal disclaimers. © NXP Semiconductors N.V. 2014. All rights reserved.
Product data sheet Rev. 4.3 — 22 April 2014 58 of 76
NXP Semiconductors LPC81xM
32-bit ARM Cortex-M0+ microcontroller
14. Application information
14.1 Typical wake-up times
[1] The wake-up time measured is the time between when a GPIO input pin is triggered to wake the device up
from the low power modes and from when a GPIO output pin is set in the interrupt service routine (ISR)
wake-up handler.
[2] IRC enabled, all peripherals off.
[3] Watchdog oscillator disabled, Brown-Out Detect (BOD) disabled.
[4] Self wakeup-timer disabled. Wake-up from deep power-down causes the LPC800 to go through entire reset
process. The wake-up time measured is the time between when a wake-up pin is triggered to wake the
device up from the low power modes and from when a GPIO output pin is set in the reset handler.
14.2 XTAL input
The input voltage to the on-chip oscillators is limited to 1.8 V. If the oscillator is driven by a
clock in slave mode, it is recommended that the input be coupled through a capacitor with
Ci = 100 pF. To limit the input voltage to the specified range, choose an additional
capacitor to ground Cg which attenuates the input voltage by a factor Ci/(Ci + Cg). In slave
mode, a minimum of 200 mV(RMS) is needed.
In slave mode the input clock signal should be coupled by means of a capacitor of 100 pF
(Figure 35), with an amplitude between 200 mV (RMS) and 1000 mV (RMS). This
corresponds to a square wave signal with a signal swing of between 280 mV and 1.4 V.
The XTALOUT pin in this configuration can be left unconnected.
External components and models used in oscillation mode are shown in Figure 36 and in
Table 25 and Table 26. Since the feedback resistance is integrated on chip, only a crystal
and the capacitances CX1 and CX2 need to be connected externally in case of
fundamental mode oscillation (the fundamental frequency is represented by L, CL and
Table 24. Typical wake-up times (3.3 V, Temp = 25 °C)
Power modes VDD current Wake-up time
Sleep mode (12 MHz)[1][2] 0.7 mA 2.6 s
Deep-sleep mode[1][3] 150 A 4 s
Power-down mode[1][3] 0.9 A 50 s
Deep Power-down mode[4] 170 nA 215 s
Fig 35. Slave mode operation of the on-chip oscillator
LPC81XM All information provided in this document is subject to legal disclaimers. © NXP Semiconductors N.V. 2014. All rights reserved.
Product data sheet Rev. 4.3 — 22 April 2014 59 of 76
NXP Semiconductors LPC81xM
32-bit ARM Cortex-M0+ microcontroller
RS). Capacitance CP in Figure 36 represents the parallel package capacitance and should
not be larger than 7 pF. Parameters FOSC, CL, RS and CP are supplied by the crystal
manufacturer (see Table 25).
Fig 36. Oscillator modes and models: oscillation mode of operation and external crystal
model used for CX1/CX2 evaluation
Table 25. Recommended values for CX1/CX2 in oscillation mode (crystal and external
components parameters) low frequency mode
Fundamental oscillation
frequency FOSC
Crystal load
capacitance CL
Maximum crystal
series resistance RS
External load
capacitors CX1, CX2
1 MHz to 5 MHz 10 pF < 300 18 pF, 18 pF
20 pF < 300 39 pF, 39 pF
30 pF < 300 57 pF, 57 pF
5 MHz to 10 MHz 10 pF < 300 18 pF, 18 pF
20 pF < 200 39 pF, 39 pF
30 pF < 100 57 pF, 57 pF
10 MHz to 15 MHz 10 pF < 160 18 pF, 18 pF
20 pF < 60 39 pF, 39 pF
15 MHz to 20 MHz 10 pF < 80 18 pF, 18 pF
Table 26. Recommended values for CX1/CX2 in oscillation mode (crystal and external
components parameters) high frequency mode
Fundamental oscillation
frequency FOSC
Crystal load
capacitance CL
Maximum crystal
series resistance RS
External load
capacitors CX1, CX2
15 MHz to 20 MHz 10 pF < 180 18 pF, 18 pF
20 pF < 100 39 pF, 39 pF
20 MHz to 25 MHz 10 pF < 160 18 pF, 18 pF
20 pF < 80 39 pF, 39 pF
LPC81XM All information provided in this document is subject to legal disclaimers. © NXP Semiconductors N.V. 2014. All rights reserved.
Product data sheet Rev. 4.3 — 22 April 2014 60 of 76
NXP Semiconductors LPC81xM
32-bit ARM Cortex-M0+ microcontroller
14.3 XTAL Printed Circuit Board (PCB) layout guidelines
The crystal should be connected on the PCB as close as possible to the oscillator input
and output pins of the chip. Take care that the load capacitors Cx1,Cx2, and Cx3 in case of
third overtone crystal usage have a common ground plane. The external components
must also be connected to the ground plain. Loops must be made as small as possible in
order to keep the noise coupled in via the PCB as small as possible. Also parasitics
should stay as small as possible. Values of Cx1 and Cx2 should be chosen smaller
accordingly to the increase in parasitics of the PCB layout.
LPC81XM All information provided in this document is subject to legal disclaimers. © NXP Semiconductors N.V. 2014. All rights reserved.
Product data sheet Rev. 4.3 — 22 April 2014 61 of 76
NXP Semiconductors LPC81xM
32-bit ARM Cortex-M0+ microcontroller
15. Package outline
Fig 37. Package outline SOT097-2 (DIP8)
Outline References
version
European
projection
Issue date
IEC JEDEC JEITA
SOT97-2 MO-001
sot097-2_po
10-10-15
10-10-18
Unit(1)
mm
max
nom
min
4.2
0.51
0.53
0.38
1.07
0.89
0.38
0.20
6.48
6.20
9.8
9.2
2.54 7.62
A
Dimensions (inch dimensions are derived from the original dimensions)
Note
1. Plastic or metal protrusions of 0.15 mm (0.006 inch) maximum per side are not included
DIP8: plastic dual in-line package; 8 leads (300 mil) SOT97-2
A1 b
1.73
1.14
b1 b2 c D(1) E(1) e e1 L ME MH w
0.254
Z(1)
1.15
inches
max
nom
min
0.17
0.02
3.43
A2
0.14 0.021
0.015
0.042
0.035
0.015
0.008
9.40
7.88
0.37
0.31
7.88
7.62
0.31
0.30
0.26
0.24
0.39
0.36
3.60
3.05
0.14
0.12
0.1
0.3
0.068
0.045
0.01
0.045
0 2.5 5 mm
scale
Z e w
b1
D
seating plane
A2
A1
A
L
pin 1 index
b b2
E
1 4
8 5
(e1)
MH
ME
c
- - - - - -
LPC81XM All information provided in this document is subject to legal disclaimers. © NXP Semiconductors N.V. 2014. All rights reserved.
Product data sheet Rev. 4.3 — 22 April 2014 62 of 76
NXP Semiconductors LPC81xM
32-bit ARM Cortex-M0+ microcontroller
Fig 38. Package outline SOT403-1 (TSSOP16)
UNIT A1 A2 A3 bp c D(1) E (2) e HE L Lp Q v w y Z (1) θ
OUTLINE REFERENCES
VERSION
EUROPEAN
PROJECTION ISSUE DATE
IEC JEDEC JEITA
mm 0.15
0.05
0.95
0.80
0.30
0.19
0.2
0.1
5.1
4.9
4.5
4.3
0.65
6.6
6.2
0.4
0.3
0.40
0.06
8
0
o
1 0.2 0.13 0.1 o
DIMENSIONS (mm are the original dimensions)
Notes
1. Plastic or metal protrusions of 0.15 mm maximum per side are not included.
2. Plastic interlead protrusions of 0.25 mm maximum per side are not included.
0.75
0.50
SOT403-1 MO-153
99-12-27
03-02-18
w M
bp
D
Z
e
0.25
1 8
16 9
θ
A
A1
A2
Lp
Q
detail X
L
(A 3 )
HE
E
c
v M A
X
A
y
0 2.5 5 mm
scale
TSSOP16: plastic thin shrink small outline package; 16 leads; body width 4.4 mm SOT403-1
A
max.
1.1
pin 1 index
LPC81XM All information provided in this document is subject to legal disclaimers. © NXP Semiconductors N.V. 2014. All rights reserved.
Product data sheet Rev. 4.3 — 22 April 2014 63 of 76
NXP Semiconductors LPC81xM
32-bit ARM Cortex-M0+ microcontroller
Fig 39. Package outline SOT163-1 (SO20)
UNIT
A
max. A1 A2 A3 bp c D(1) E(1) e HE L Lp Q v w y Z (1) θ
OUTLINE REFERENCES
VERSION
EUROPEAN
PROJECTION ISSUE DATE
IEC JEDEC JEITA
mm
inches
2.65 0.3
0.1
2.45
2.25
0.49
0.36
0.32
0.23
13.0
12.6
7.6
7.4
1.27
10.65
10.00
1.1
1.0
0.9
0.4 8
0
o
o
0.25 0.1
DIMENSIONS (inch dimensions are derived from the original mm dimensions)
Note
1. Plastic or metal protrusions of 0.15 mm (0.006 inch) maximum per side are not included.
1.1
0.4
SOT163-1
10
20
w M
bp
detail X
Z
e
11
1
D
y
0.25
075E04 MS-013
pin 1 index
0.1 0.012
0.004
0.096
0.089
0.019
0.014
0.013
0.009
0.51
0.49
0.30
0.29
0.05
1.4
0.055
0.419
0.394
0.043
0.039
0.035
0.016
0.01
0.25
0.01 0.004
0.043
0.016
0.01
0 5 10 mm
scale
X
θ
A
A1
A2
HE
Lp
Q
E
c
L
v M A
(A 3 )
A
SO20: plastic small outline package; 20 leads; body width 7.5 mm SOT163-1
99-12-27
03-02-19
LPC81XM All information provided in this document is subject to legal disclaimers. © NXP Semiconductors N.V. 2014. All rights reserved.
Product data sheet Rev. 4.3 — 22 April 2014 64 of 76
NXP Semiconductors LPC81xM
32-bit ARM Cortex-M0+ microcontroller
Fig 40. Package outline SOT360-1 (TSSOP20)
UNIT A1 A2 A3 bp c D(1) E (2) e HE L Lp Q v w y Z (1) θ
OUTLINE REFERENCES
VERSION
EUROPEAN
PROJECTION ISSUE DATE
IEC JEDEC JEITA
mm 0.15
0.05
0.95
0.80
0.30
0.19
0.2
0.1
6.6
6.4
4.5
4.3
0.65
6.6
6.2
0.4
0.3
0.5
0.2
8
0
o
1 0.2 0.13 0.1 o
DIMENSIONS (mm are the original dimensions)
Notes
1. Plastic or metal protrusions of 0.15 mm maximum per side are not included.
2. Plastic interlead protrusions of 0.25 mm maximum per side are not included.
0.75
0.50
SOT360-1 MO-153
99-12-27
03-02-19
w M
bp
D
Z
e
0.25
1 10
20 11
pin 1 index
θ
A
A1
A2
Lp
Q
detail X
L
(A 3 )
HE
E
c
v M A
X
A
y
0 2.5 5 mm
scale
TSSOP20: plastic thin shrink small outline package; 20 leads; body width 4.4 mm SOT360-1
A
max.
1.1
LPC81XM All information provided in this document is subject to legal disclaimers. © NXP Semiconductors N.V. 2014. All rights reserved.
Product data sheet Rev. 4.3 — 22 April 2014 65 of 76
NXP Semiconductors LPC81xM
32-bit ARM Cortex-M0+ microcontroller
Fig 41. Package outline SOT1341-1 (XSON16)
Outline References
version
European
projection Issue date
IEC JEDEC JEITA
SOT1341-1 MO-252
sot1341-1_po
12-09-05
13-02-13
Unit(1)
mm
max
nom
min
0.5 0.05
0.00
A
Dimensions (mm are the original dimensions)
XSON16: plastic extremely thin small outline package; no leads; 16 terminals; body 2.5 x 3.2 x 0.5 mm S OT1341-1
A1
0.25
0.20
0.15
2.6
2.5
2.4
0.9
0.8
0.7
3.3
3.2
3.1
0.4 2.8
0.2
b c
0.152
0.050
D E e e1 k L
1.0
0.9
0.8
L1 v
0.1 0.05
w y
0.05
y1
0.05
0 1 2 3 mm
scale
Note
1. Plastic or metal protrusions of 0.075 mm maximum per side are not included.
e1
e
terminal 1
index area
terminal 1
index area
D B A
E
detail X
c
A A1
L1
k
L
- - - - - -
X
C
b y1 C y
v C A B
w C
1 8
16 9
LPC81XM All information provided in this document is subject to legal disclaimers. © NXP Semiconductors N.V. 2014. All rights reserved.
Product data sheet Rev. 4.3 — 22 April 2014 66 of 76
NXP Semiconductors LPC81xM
32-bit ARM Cortex-M0+ microcontroller
16. Soldering
Fig 42. Reflow soldering of the TSSOP16 package
DIMENSIONS in mm
P1 Ay By C D1 D2 Gx Gy Hy
sot403-1_fr
Hx
SOT403-1
solder land
occupied area
Footprint information for reflow soldering of TSSOP16 package
Gy By Ay
C
Hy
Hx
Gx
P1
Generic footprint pattern
Refer to the package outline drawing for actual layout
P2
(0.125) (0.125)
D2 (4x) D1
P2
0.650 0.750 7.200 4.500 1.350 0.400 0.600 5.600 5.300 5.800 7.450
LPC81XM All information provided in this document is subject to legal disclaimers. © NXP Semiconductors N.V. 2014. All rights reserved.
Product data sheet Rev. 4.3 — 22 April 2014 67 of 76
NXP Semiconductors LPC81xM
32-bit ARM Cortex-M0+ microcontroller
Fig 43. Reflow soldering of the SO20 package
occupied area sot163-1_fr
solder lands
placement accuracy ± 0.25 Dimensions in mm
1.50
0.60 (20×)
1.27 (18×)
8.00 11.00
13.40
11.40
LPC81XM All information provided in this document is subject to legal disclaimers. © NXP Semiconductors N.V. 2014. All rights reserved.
Product data sheet Rev. 4.3 — 22 April 2014 68 of 76
NXP Semiconductors LPC81xM
32-bit ARM Cortex-M0+ microcontroller
Fig 44. Reflow soldering of the TSSOP20 package
DIMENSIONS in mm
P1 Ay By C D1 D2 Gx Gy Hy
sot360-1_fr
Hx
SOT360-1
solder land
occupied area
Footprint information for reflow soldering of TSSOP20 package
Gy By Ay
C
Hy
Hx
Gx
P1
Generic footprint pattern
Refer to the package outline drawing for actual layout
P2
(0.125) (0.125)
D2 (4x) D1
P2
0.650 0.750 7.200 4.500 1.350 0.400 0.600 6.900 5.300 7.300 7.450
LPC81XM All information provided in this document is subject to legal disclaimers. © NXP Semiconductors N.V. 2014. All rights reserved.
Product data sheet Rev. 4.3 — 22 April 2014 69 of 76
NXP Semiconductors LPC81xM
32-bit ARM Cortex-M0+ microcontroller
Fig 45. Reflow soldering of the XSON16 package
LPC81XM All information provided in this document is subject to legal disclaimers. © NXP Semiconductors N.V. 2014. All rights reserved.
Product data sheet Rev. 4.3 — 22 April 2014 70 of 76
NXP Semiconductors LPC81xM
32-bit ARM Cortex-M0+ microcontroller
17. Abbreviations
18. References
[1] I2C-bus specification UM10204.
Table 27. Abbreviations
Acronym Description
AHB Advanced High-performance Bus
APB Advanced Peripheral Bus
BOD BrownOut Detection
GPIO General-Purpose Input/Output
PLL Phase-Locked Loop
RC Resistor-Capacitor
SPI Serial Peripheral Interface
SMBus System Management Bus
TEM Transverse ElectroMagnetic
UART Universal Asynchronous Receiver/Transmitter
LPC81XM All information provided in this document is subject to legal disclaimers. © NXP Semiconductors N.V. 2014. All rights reserved.
Product data sheet Rev. 4.3 — 22 April 2014 71 of 76
NXP Semiconductors LPC81xM
32-bit ARM Cortex-M0+ microcontroller
19. Revision history
Table 28. Revision history
Document ID Release date Data sheet status Change notice Supersedes
LPC81XM v.4.3 20140422 Product data sheet - LPC81XM v.4.2
Modifications: • Section 8.20.2 “Clock input” updated for clarity.
• CLKIN signal removed from Table 12 “Dynamic characteristic: external clock (XTALIN
inputs)”.
• Name “SCT” changed to “SCTimer/PWM” for clarity.
• Remove slew rate control from GPIO features for clarity.
• MRT bus stall mode added.
• WWDT clock source corrected in Section 8.17.1.
• Pin description table updated for clarification (I2C-bus pins, WAKEUP, RESET).
• Added reflow solder diagram and thermal resistance numbers for XSON16
(SOT1341-1).
• Table 21: Added Vref(cmp) spec for PIO0_6/VDDCMP.
LPC81XM v.4.2 20131210 Product data sheet - LPC81XM v.4.1
Modifications: Corrected vertical axis marker in Figure 21 “CoreMark score”.
LPC81XM v.4.1 20131112 Product data sheet - LPC81XM v.4
Modifications: • Corrected XSON16 pin information in Figure 6 and Table 4.
LPC81XM v.4 20131025 Product data sheet - LPC81XM v.3.1
Modifications: • Added Section 14.1 “Typical wake-up times”.
• Added LPC812M101JTB16 and XSON16 package.
LPC81XM v.3.1 20130916 Product data sheet - LPC81XM v.3
Modifications: • Correct the pin interrupt features: Pin interrupts can wake up the part from Sleep
mode, Deep-sleep mode, and Power-down mode. See Section 8.11.1.
• Table 9 “Static characteristics”: Updated power numbers for Deep-sleep, Power-down,
and Deep power-down.
• Added 30 MHz data to Figure 13 “Active mode: Typical supply current IDD versus
supply voltage VDD”, Figure 14 “Active mode: Typical supply current IDD versus
temperature”, and Figure 15 “Sleep mode: Typical supply current IDD versus
temperature for different system clock frequencies”.
LPC81XM v.3 20130729 Product data sheet - LPC81XM v.2.1
• Operating temperature range changed to 40 °C to 105 °C.
• Type numbers updated to reflect the new operating temperature range. See Table 1
“Ordering information” and Table 2 “Ordering options”.
• ISP entry pin moved from PIO0_1 to PIO0_12 for TSSOP, and SSOP packages. See
Table 4 and Table 6.
• Propagation delay values updated in Table 21 “Comparator characteristics”.
• SPI characteristics updated. See Section 12.6.
• IRC characteristics updated. See Section 12.3.
• CoreMark data updated. See Figure 19 and Figure 20.
• IRC frequency changed to 12 MHz +/- 1.5 %. See Table 13.
• Data sheet status updated to Product data sheet.
LPC81XM v.2.1 20130325 Preliminary data sheet - LPC81XM v.2
LPC81XM All information provided in this document is subject to legal disclaimers. © NXP Semiconductors N.V. 2014. All rights reserved.
Product data sheet Rev. 4.3 — 22 April 2014 72 of 76
NXP Semiconductors LPC81xM
32-bit ARM Cortex-M0+ microcontroller
• Editorial updates (temperature sensor removed).
• CoreMark data added. See Figure 19 “Active mode: CoreMark power consumption
IDD” and Figure 20 “CoreMark score”.
• IDD in Deep power-down mode added for condition Low-power oscillator on/WKT
wake-up enabled. See Table 10.
• Table note 3 updated for Table 4 “Pin description table (fixed pins)”.
• Conditions for ter and tprog updated in Table 12 “Flash characteristics”.
• Section 13.3 “Internal voltage reference” added.
• Typical timing data added for SPI. See Section 12.6.
• Typical timing data added for USART in synchronous mode. See Section 12.7.
• BOD characterization added. See Section 13.1.
• IRC characterization added. See Section 12.3.
• Internal voltage reference characteristics added. See Section 13.3.
• Data sheet status changed to Preliminary data sheet.
LPC81XM v.2 20130128 Objective data sheet - LPC81XM v.1
Modifications: • MTB memory space changed to 1 kB in Figure 6.
• Electrical pin characteristics added in Table 10.
• Figure 11 “Connecting the SWD pins to a standard SWD connector” added.
• Peripheral power consumption added in Table 11.
• Table 7 updated.
• MRT implementation changed to 31-bit timer.
• Power consumption data in active and sleep mode with IRC added. See Figure 13 to
Figure 15.
• Power consumption (parameter IDD) in active and sleep mode for low-power mode at
12 MHz corrected in Table 10.
• Power consumption (parameter IDD) in active and sleep mode at 24 MHz added in
Table 10.
• Maximum USART speed in synchronous mode changed to 10 Mbit/s.
• Section 5 “Marking” added.
LPC81XM v.1 20121112 Objective data sheet - -
Table 28. Revision history …continued
Document ID Release date Data sheet status Change notice Supersedes
LPC81XM All information provided in this document is subject to legal disclaimers. © NXP Semiconductors N.V. 2014. All rights reserved.
Product data sheet Rev. 4.3 — 22 April 2014 73 of 76
NXP Semiconductors LPC81xM
32-bit ARM Cortex-M0+ microcontroller
20. Legal information
20.1 Data sheet status
[1] Please consult the most recently issued document before initiating or completing a design.
[2] The term ‘short data sheet’ is explained in section “Definitions”.
[3] The product status of device(s) described in this document may have changed since this document was published and may differ in case of multiple devices. The latest product status
information is available on the Internet at URL http://www.nxp.com.
20.2 Definitions
Draft — The document is a draft version only. The content is still under
internal review and subject to formal approval, which may result in
modifications or additions. NXP Semiconductors does not give any
representations or warranties as to the accuracy or completeness of
information included herein and shall have no liability for the consequences of
use of such information.
Short data sheet — A short data sheet is an extract from a full data sheet
with the same product type number(s) and title. A short data sheet is intended
for quick reference only and should not be relied upon to contain detailed and
full information. For detailed and full information see the relevant full data
sheet, which is available on request via the local NXP Semiconductors sales
office. In case of any inconsistency or conflict with the short data sheet, the
full data sheet shall prevail.
Product specification — The information and data provided in a Product
data sheet shall define the specification of the product as agreed between
NXP Semiconductors and its customer, unless NXP Semiconductors and
customer have explicitly agreed otherwise in writing. In no event however,
shall an agreement be valid in which the NXP Semiconductors product is
deemed to offer functions and qualities beyond those described in the
Product data sheet.
20.3 Disclaimers
Limited warranty and liability — Information in this document is believed to
be accurate and reliable. However, NXP Semiconductors does not give any
representations or warranties, expressed or implied, as to the accuracy or
completeness of such information and shall have no liability for the
consequences of use of such information. NXP Semiconductors takes no
responsibility for the content in this document if provided by an information
source outside of NXP Semiconductors.
In no event shall NXP Semiconductors be liable for any indirect, incidental,
punitive, special or consequential damages (including - without limitation - lost
profits, lost savings, business interruption, costs related to the removal or
replacement of any products or rework charges) whether or not such
damages are based on tort (including negligence), warranty, breach of
contract or any other legal theory.
Notwithstanding any damages that customer might incur for any reason
whatsoever, NXP Semiconductors’ aggregate and cumulative liability towards
customer for the products described herein shall be limited in accordance
with the Terms and conditions of commercial sale of NXP Semiconductors.
Right to make changes — NXP Semiconductors reserves the right to make
changes to information published in this document, including without
limitation specifications and product descriptions, at any time and without
notice. This document supersedes and replaces all information supplied prior
to the publication hereof.
Suitability for use — NXP Semiconductors products are not designed,
authorized or warranted to be suitable for use in life support, life-critical or
safety-critical systems or equipment, nor in applications where failure or
malfunction of an NXP Semiconductors product can reasonably be expected
to result in personal injury, death or severe property or environmental
damage. NXP Semiconductors and its suppliers accept no liability for
inclusion and/or use of NXP Semiconductors products in such equipment or
applications and therefore such inclusion and/or use is at the customer’s own
risk.
Applications — Applications that are described herein for any of these
products are for illustrative purposes only. NXP Semiconductors makes no
representation or warranty that such applications will be suitable for the
specified use without further testing or modification.
Customers are responsible for the design and operation of their applications
and products using NXP Semiconductors products, and NXP Semiconductors
accepts no liability for any assistance with applications or customer product
design. It is customer’s sole responsibility to determine whether the NXP
Semiconductors product is suitable and fit for the customer’s applications and
products planned, as well as for the planned application and use of
customer’s third party customer(s). Customers should provide appropriate
design and operating safeguards to minimize the risks associated with their
applications and products.
NXP Semiconductors does not accept any liability related to any default,
damage, costs or problem which is based on any weakness or default in the
customer’s applications or products, or the application or use by customer’s
third party customer(s). Customer is responsible for doing all necessary
testing for the customer’s applications and products using NXP
Semiconductors products in order to avoid a default of the applications and
the products or of the application or use by customer’s third party
customer(s). NXP does not accept any liability in this respect.
Limiting values — Stress above one or more limiting values (as defined in
the Absolute Maximum Ratings System of IEC 60134) will cause permanent
damage to the device. Limiting values are stress ratings only and (proper)
operation of the device at these or any other conditions above those given in
the Recommended operating conditions section (if present) or the
Characteristics sections of this document is not warranted. Constant or
repeated exposure to limiting values will permanently and irreversibly affect
the quality and reliability of the device.
Terms and conditions of commercial sale — NXP Semiconductors
products are sold subject to the general terms and conditions of commercial
sale, as published at http://www.nxp.com/profile/terms, unless otherwise
agreed in a valid written individual agreement. In case an individual
agreement is concluded only the terms and conditions of the respective
agreement shall apply. NXP Semiconductors hereby expressly objects to
applying the customer’s general terms and conditions with regard to the
purchase of NXP Semiconductors products by customer.
No offer to sell or license — Nothing in this document may be interpreted or
construed as an offer to sell products that is open for acceptance or the grant,
conveyance or implication of any license under any copyrights, patents or
other industrial or intellectual property rights.
Document status[1][2] Product status[3] Definition
Objective [short] data sheet Development This document contains data from the objective specification for product development.
Preliminary [short] data sheet Qualification This document contains data from the preliminary specification.
Product [short] data sheet Production This document contains the product specification.
LPC81XM All information provided in this document is subject to legal disclaimers. © NXP Semiconductors N.V. 2014. All rights reserved.
Product data sheet Rev. 4.3 — 22 April 2014 74 of 76
NXP Semiconductors LPC81xM
32-bit ARM Cortex-M0+ microcontroller
Export control — This document as well as the item(s) described herein
may be subject to export control regulations. Export might require a prior
authorization from competent authorities.
Non-automotive qualified products — Unless this data sheet expressly
states that this specific NXP Semiconductors product is automotive qualified,
the product is not suitable for automotive use. It is neither qualified nor tested
in accordance with automotive testing or application requirements. NXP
Semiconductors accepts no liability for inclusion and/or use of
non-automotive qualified products in automotive equipment or applications.
In the event that customer uses the product for design-in and use in
automotive applications to automotive specifications and standards, customer
(a) shall use the product without NXP Semiconductors’ warranty of the
product for such automotive applications, use and specifications, and (b)
whenever customer uses the product for automotive applications beyond
NXP Semiconductors’ specifications such use shall be solely at customer’s
own risk, and (c) customer fully indemnifies NXP Semiconductors for any
liability, damages or failed product claims resulting from customer design and
use of the product for automotive applications beyond NXP Semiconductors’
standard warranty and NXP Semiconductors’ product specifications.
20.4 Trademarks
Notice: All referenced brands, product names, service names and trademarks
are the property of their respective owners.
I2C-bus — logo is a trademark of NXP Semiconductors N.V.
21. Contact information
For more information, please visit: http://www.nxp.com
For sales office addresses, please send an email to: salesaddresses@nxp.com
LPC81XM All information provided in this document is subject to legal disclaimers. © NXP Semiconductors N.V. 2014. All rights reserved.
Product data sheet Rev. 4.3 — 22 April 2014 75 of 76
continued >>
NXP Semiconductors LPC81xM
32-bit ARM Cortex-M0+ microcontroller
22. Contents
1 General description . . . . . . . . . . . . . . . . . . . . . . 1
2 Features and benefits . . . . . . . . . . . . . . . . . . . . 1
3 Applications . . . . . . . . . . . . . . . . . . . . . . . . . . . . 2
4 Ordering information. . . . . . . . . . . . . . . . . . . . . 3
4.1 Ordering options . . . . . . . . . . . . . . . . . . . . . . . . 3
5 Marking . . . . . . . . . . . . . . . . . . . . . . . . . . . . . . . . 4
6 Block diagram . . . . . . . . . . . . . . . . . . . . . . . . . . 5
7 Pinning information. . . . . . . . . . . . . . . . . . . . . . 6
7.1 Pinning . . . . . . . . . . . . . . . . . . . . . . . . . . . . . . . 6
7.2 Pin description . . . . . . . . . . . . . . . . . . . . . . . . . 8
8 Functional description . . . . . . . . . . . . . . . . . . 13
8.1 ARM Cortex-M0+ core . . . . . . . . . . . . . . . . . . 13
8.2 On-chip flash program memory . . . . . . . . . . . 13
8.3 On-chip SRAM . . . . . . . . . . . . . . . . . . . . . . . . 13
8.4 On-chip ROM . . . . . . . . . . . . . . . . . . . . . . . . . 13
8.5 Nested Vectored Interrupt Controller (NVIC) . 13
8.5.1 Features . . . . . . . . . . . . . . . . . . . . . . . . . . . . . 13
8.5.2 Interrupt sources. . . . . . . . . . . . . . . . . . . . . . . 13
8.6 System tick timer . . . . . . . . . . . . . . . . . . . . . . 14
8.7 Memory map. . . . . . . . . . . . . . . . . . . . . . . . . . 14
8.8 I/O configuration . . . . . . . . . . . . . . . . . . . . . . . 15
8.8.1 Standard I/O pad configuration . . . . . . . . . . . . 16
8.9 Switch Matrix (SWM) . . . . . . . . . . . . . . . . . . . 17
8.10 Fast General-Purpose parallel I/O (GPIO) . . . 17
8.10.1 Features . . . . . . . . . . . . . . . . . . . . . . . . . . . . . 18
8.11 Pin interrupt/pattern match engine . . . . . . . . . 18
8.11.1 Features . . . . . . . . . . . . . . . . . . . . . . . . . . . . . 18
8.12 USART0/1/2 . . . . . . . . . . . . . . . . . . . . . . . . . . 19
8.12.1 Features . . . . . . . . . . . . . . . . . . . . . . . . . . . . . 19
8.13 SPI0/1. . . . . . . . . . . . . . . . . . . . . . . . . . . . . . . 19
8.13.1 Features . . . . . . . . . . . . . . . . . . . . . . . . . . . . . 19
8.14 I2C-bus interface . . . . . . . . . . . . . . . . . . . . . . 20
8.14.1 Features . . . . . . . . . . . . . . . . . . . . . . . . . . . . . 20
8.15 State-Configurable Timer/PWM
(SCTimer/PWM) . . . . . . . . . . . . . . . . . . . . . . . 20
8.15.1 Features . . . . . . . . . . . . . . . . . . . . . . . . . . . . . 21
8.16 Multi-Rate Timer (MRT) . . . . . . . . . . . . . . . . . 21
8.16.1 Features . . . . . . . . . . . . . . . . . . . . . . . . . . . . . 21
8.17 Windowed WatchDog Timer (WWDT) . . . . . . 21
8.17.1 Features . . . . . . . . . . . . . . . . . . . . . . . . . . . . . 21
8.18 Self Wake-up Timer (WKT). . . . . . . . . . . . . . . 22
8.18.1 Features . . . . . . . . . . . . . . . . . . . . . . . . . . . . . 22
8.19 Analog comparator (ACMP) . . . . . . . . . . . . . . 22
8.19.1 Features . . . . . . . . . . . . . . . . . . . . . . . . . . . . . 23
8.20 Clocking and power control . . . . . . . . . . . . . . 24
8.20.1 Crystal and internal oscillators . . . . . . . . . . . . 24
8.20.1.1 Internal RC Oscillator (IRC) . . . . . . . . . . . . . . 25
8.20.1.2 Crystal Oscillator (SysOsc) . . . . . . . . . . . . . . 25
8.20.1.3 Internal Low-power Oscillator and Watchdog
Oscillator (WDOsc) . . . . . . . . . . . . . . . . . . . . 25
8.20.2 Clock input . . . . . . . . . . . . . . . . . . . . . . . . . . . 25
8.20.3 System PLL . . . . . . . . . . . . . . . . . . . . . . . . . . 25
8.20.4 Clock output . . . . . . . . . . . . . . . . . . . . . . . . . . 26
8.20.5 Wake-up process . . . . . . . . . . . . . . . . . . . . . . 26
8.20.6 Power control . . . . . . . . . . . . . . . . . . . . . . . . . 26
8.20.6.1 Power profiles . . . . . . . . . . . . . . . . . . . . . . . . 26
8.20.6.2 Sleep mode . . . . . . . . . . . . . . . . . . . . . . . . . . 26
8.20.6.3 Deep-sleep mode. . . . . . . . . . . . . . . . . . . . . . 27
8.20.6.4 Power-down mode. . . . . . . . . . . . . . . . . . . . . 27
8.20.6.5 Deep power-down mode . . . . . . . . . . . . . . . . 27
8.21 System control . . . . . . . . . . . . . . . . . . . . . . . . 28
8.21.1 Reset . . . . . . . . . . . . . . . . . . . . . . . . . . . . . . . 28
8.21.2 Brownout detection . . . . . . . . . . . . . . . . . . . . 28
8.21.3 Code security (Code Read Protection - CRP) 29
8.21.4 APB interface . . . . . . . . . . . . . . . . . . . . . . . . . 29
8.21.5 AHBLite . . . . . . . . . . . . . . . . . . . . . . . . . . . . . 29
8.22 Emulation and debugging . . . . . . . . . . . . . . . 30
9 Limiting values . . . . . . . . . . . . . . . . . . . . . . . . 31
10 Thermal characteristics . . . . . . . . . . . . . . . . . 32
11 Static characteristics . . . . . . . . . . . . . . . . . . . 33
11.1 Power consumption . . . . . . . . . . . . . . . . . . . . 37
11.2 CoreMark data . . . . . . . . . . . . . . . . . . . . . . . . 41
11.3 Peripheral power consumption . . . . . . . . . . . 42
11.4 Electrical pin characteristics. . . . . . . . . . . . . . 43
12 Dynamic characteristics. . . . . . . . . . . . . . . . . 46
12.1 Flash memory . . . . . . . . . . . . . . . . . . . . . . . . 46
12.2 External clock for the oscillator in slave mode 46
12.3 Internal oscillators . . . . . . . . . . . . . . . . . . . . . 47
12.4 I/O pins. . . . . . . . . . . . . . . . . . . . . . . . . . . . . . 48
12.5 I2C-bus. . . . . . . . . . . . . . . . . . . . . . . . . . . . . . 48
12.6 SPI interfaces. . . . . . . . . . . . . . . . . . . . . . . . . 50
12.7 USART interface . . . . . . . . . . . . . . . . . . . . . . 53
13 Analog characteristics . . . . . . . . . . . . . . . . . . 54
13.1 BOD . . . . . . . . . . . . . . . . . . . . . . . . . . . . . . . . 54
13.2 Internal voltage reference . . . . . . . . . . . . . . . 54
13.3 Comparator . . . . . . . . . . . . . . . . . . . . . . . . . . 55
14 Application information . . . . . . . . . . . . . . . . . 58
14.1 Typical wake-up times . . . . . . . . . . . . . . . . . . 58
14.2 XTAL input . . . . . . . . . . . . . . . . . . . . . . . . . . . 58
14.3 XTAL Printed Circuit Board (PCB) layout
guidelines. . . . . . . . . . . . . . . . . . . . . . . . . . . . 60
15 Package outline. . . . . . . . . . . . . . . . . . . . . . . . 61
NXP Semiconductors LPC81xM
32-bit ARM Cortex-M0+ microcontroller
© NXP Semiconductors N.V. 2014. All rights reserved.
For more information, please visit: http://www.nxp.com
For sales office addresses, please send an email to: salesaddresses@nxp.com
Date of release: 22 April 2014
Document identifier: LPC81XM
Please be aware that important notices concerning this document and the product(s)
described herein, have been included in section ‘Legal information’.
16 Soldering . . . . . . . . . . . . . . . . . . . . . . . . . . . . . 66
17 Abbreviations. . . . . . . . . . . . . . . . . . . . . . . . . . 70
18 References . . . . . . . . . . . . . . . . . . . . . . . . . . . . 70
19 Revision history. . . . . . . . . . . . . . . . . . . . . . . . 71
20 Legal information. . . . . . . . . . . . . . . . . . . . . . . 73
20.1 Data sheet status . . . . . . . . . . . . . . . . . . . . . . 73
20.2 Definitions. . . . . . . . . . . . . . . . . . . . . . . . . . . . 73
20.3 Disclaimers . . . . . . . . . . . . . . . . . . . . . . . . . . . 73
20.4 Trademarks. . . . . . . . . . . . . . . . . . . . . . . . . . . 74
21 Contact information. . . . . . . . . . . . . . . . . . . . . 74
22 Contents . . . . . . . . . . . . . . . . . . . . . . . . . . . . . . 75
1. General description
The LPC1769/68/67/66/65/64/63 are ARM Cortex-M3 based microcontrollers for
embedded applications featuring a high level of integration and low power consumption.
The ARM Cortex-M3 is a next generation core that offers system enhancements such as
enhanced debug features and a higher level of support block integration.
The LPC1768/67/66/65/64/63 operate at CPU frequencies of up to 100 MHz. The
LPC1769 operates at CPU frequencies of up to 120 MHz. The ARM Cortex-M3 CPU
incorporates a 3-stage pipeline and uses a Harvard architecture with separate local
instruction and data buses as well as a third bus for peripherals. The ARM Cortex-M3
CPU also includes an internal prefetch unit that supports speculative branching.
The peripheral complement of the LPC1769/68/67/66/65/64/63 includes up to 512 kB of
flash memory, up to 64 kB of data memory, Ethernet MAC, USB Device/Host/OTG
interface, 8-channel general purpose DMA controller, 4 UARTs, 2 CAN channels, 2 SSP
controllers, SPI interface, 3 I2C-bus interfaces, 2-input plus 2-output I2S-bus interface,
8-channel 12-bit ADC, 10-bit DAC, motor control PWM, Quadrature Encoder interface,
four general purpose timers, 6-output general purpose PWM, ultra-low power Real-Time
Clock (RTC) with separate battery supply, and up to 70 general purpose I/O pins.
The LPC1769/68/67/66/65/64/63 are pin-compatible to the 100-pin LPC236x
ARM7-based microcontroller series.
2. Features and benefits
ARM Cortex-M3 processor, running at frequencies of up to 100 MHz
(LPC1768/67/66/65/64/63) or of up to 120 MHz (LPC1769). A Memory Protection Unit
(MPU) supporting eight regions is included.
ARM Cortex-M3 built-in Nested Vectored Interrupt Controller (NVIC).
Up to 512 kB on-chip flash programming memory. Enhanced flash memory accelerator
enables high-speed 120 MHz operation with zero wait states.
In-System Programming (ISP) and In-Application Programming (IAP) via on-chip
bootloader software.
On-chip SRAM includes:
32/16 kB of SRAM on the CPU with local code/data bus for high-performance CPU
access.
LPC1769/68/67/66/65/64/63
32-bit ARM Cortex-M3 microcontroller; up to 512 kB flash and
64 kB SRAM with Ethernet, USB 2.0 Host/Device/OTG, CAN
Rev. 9.4 — 4 April 2014 Product data sheet
LPC1769_68_67_66_65_64_63 All information provided in this document is subject to legal disclaimers. © NXP Semiconductors N.V. 2014. All rights reserved.
Product data sheet Rev. 9.4 — 4 April 2014 2 of 88
NXP Semiconductors LPC1769/68/67/66/65/64/63
32-bit ARM Cortex-M3 microcontroller
Two/one 16 kB SRAM blocks with separate access paths for higher throughput.
These SRAM blocks may be used for Ethernet, USB, and DMA memory, as well as
for general purpose CPU instruction and data storage.
Eight channel General Purpose DMA controller (GPDMA) on the AHB multilayer
matrix that can be used with SSP, I2S-bus, UART, Analog-to-Digital and
Digital-to-Analog converter peripherals, timer match signals, and for
memory-to-memory transfers.
Multilayer AHB matrix interconnect provides a separate bus for each AHB master.
AHB masters include the CPU, General Purpose DMA controller, Ethernet MAC, and
the USB interface. This interconnect provides communication with no arbitration
delays.
Split APB bus allows high throughput with few stalls between the CPU and DMA.
Serial interfaces:
Ethernet MAC with RMII interface and dedicated DMA controller. (Not available on
all parts, see Table 2.)
USB 2.0 full-speed device/Host/OTG controller with dedicated DMA controller and
on-chip PHY for device, Host, and OTG functions. (Not available on all parts, see
Table 2.)
Four UARTs with fractional baud rate generation, internal FIFO, and DMA support.
One UART has modem control I/O and RS-485/EIA-485 support, and one UART
has IrDA support.
CAN 2.0B controller with two channels. (Not available on all parts, see Table 2.)
SPI controller with synchronous, serial, full duplex communication and
programmable data length.
Two SSP controllers with FIFO and multi-protocol capabilities. The SSP interfaces
can be used with the GPDMA controller.
Three enhanced I2C bus interfaces, one with an open-drain output supporting full
I2C specification and Fast mode plus with data rates of 1 Mbit/s, two with standard
port pins. Enhancements include multiple address recognition and monitor mode.
I2S (Inter-IC Sound) interface for digital audio input or output, with fractional rate
control. The I2S-bus interface can be used with the GPDMA. The I2S-bus interface
supports 3-wire and 4-wire data transmit and receive as well as master clock
input/output. (Not available on all parts, see Table 2.)
Other peripherals:
70 (100 pin package) General Purpose I/O (GPIO) pins with configurable
pull-up/down resistors. All GPIOs support a new, configurable open-drain operating
mode. The GPIO block is accessed through the AHB multilayer bus for fast access
and located in memory such that it supports Cortex-M3 bit banding and use by the
General Purpose DMA Controller.
12-bit Analog-to-Digital Converter (ADC) with input multiplexing among eight pins,
conversion rates up to 200 kHz, and multiple result registers. The 12-bit ADC can
be used with the GPDMA controller.
10-bit Digital-to-Analog Converter (DAC) with dedicated conversion timer and DMA
support. (Not available on all parts, see Table 2)
Four general purpose timers/counters, with a total of eight capture inputs and ten
compare outputs. Each timer block has an external count input. Specific timer
events can be selected to generate DMA requests.
One motor control PWM with support for three-phase motor control.
LPC1769_68_67_66_65_64_63 All information provided in this document is subject to legal disclaimers. © NXP Semiconductors N.V. 2014. All rights reserved.
Product data sheet Rev. 9.4 — 4 April 2014 3 of 88
NXP Semiconductors LPC1769/68/67/66/65/64/63
32-bit ARM Cortex-M3 microcontroller
Quadrature encoder interface that can monitor one external quadrature encoder.
One standard PWM/timer block with external count input.
RTC with a separate power domain and dedicated RTC oscillator. The RTC block
includes 20 bytes of battery-powered backup registers.
WatchDog Timer (WDT). The WDT can be clocked from the internal RC oscillator,
the RTC oscillator, or the APB clock.
ARM Cortex-M3 system tick timer, including an external clock input option.
Repetitive interrupt timer provides programmable and repeating timed interrupts.
Each peripheral has its own clock divider for further power savings.
Standard JTAG test/debug interface for compatibility with existing tools. Serial Wire
Debug and Serial Wire Trace Port options.
Emulation trace module enables non-intrusive, high-speed real-time tracing of
instruction execution.
Integrated PMU (Power Management Unit) automatically adjusts internal regulators to
minimize power consumption during Sleep, Deep sleep, Power-down, and Deep
power-down modes.
Four reduced power modes: Sleep, Deep-sleep, Power-down, and Deep power-down.
Single 3.3 V power supply (2.4 V to 3.6 V).
Four external interrupt inputs configurable as edge/level sensitive. All pins on Port 0
and Port 2 can be used as edge sensitive interrupt sources.
Non-maskable Interrupt (NMI) input.
Clock output function that can reflect the main oscillator clock, IRC clock, RTC clock,
CPU clock, and the USB clock.
The Wake-up Interrupt Controller (WIC) allows the CPU to automatically wake up from
any priority interrupt that can occur while the clocks are stopped in deep sleep,
Power-down, and Deep power-down modes.
Processor wake-up from Power-down mode via any interrupt able to operate during
Power-down mode (includes external interrupts, RTC interrupt, USB activity, Ethernet
wake-up interrupt, CAN bus activity, Port 0/2 pin interrupt, and NMI).
Brownout detect with separate threshold for interrupt and forced reset.
Power-On Reset (POR).
Crystal oscillator with an operating range of 1 MHz to 25 MHz.
4 MHz internal RC oscillator trimmed to 1 % accuracy that can optionally be used as a
system clock.
PLL allows CPU operation up to the maximum CPU rate without the need for a
high-frequency crystal. May be run from the main oscillator, the internal RC oscillator,
or the RTC oscillator.
USB PLL for added flexibility.
Code Read Protection (CRP) with different security levels.
Unique device serial number for identification purposes.
Available as LQFP100 (14 mm 14 mm 1.4 mm), TFBGA1001 (9 mm 9 mm 0.7
mm), and WLCSP100 (5.074 5.074 0.6 mm) package.
1. LPC1768/65 only.
LPC1769_68_67_66_65_64_63 All information provided in this document is subject to legal disclaimers. © NXP Semiconductors N.V. 2014. All rights reserved.
Product data sheet Rev. 9.4 — 4 April 2014 4 of 88
NXP Semiconductors LPC1769/68/67/66/65/64/63
32-bit ARM Cortex-M3 microcontroller
3. Applications
4. Ordering information
4.1 Ordering options
eMetering Alarm systems
Lighting White goods
Industrial networking Motor control
Table 1. Ordering information
Type number Package
Name Description Version
LPC1769FBD100 LQFP100 plastic low profile quad flat package; 100 leads; body 14 14 1.4 mm SOT407-1
LPC1768FBD100 LQFP100 plastic low profile quad flat package; 100 leads; body 14 14 1.4 mm SOT407-1
LPC1768FET100 TFBGA100 plastic thin fine-pitch ball grid array package; 100 balls; body 9 9 0.7 mm SOT926-1
LPC1768UK WLCSP100 wafer level chip-scale package; 100 balls; 5.074 5.074 0.6 mm -
LPC1767FBD100 LQFP100 plastic low profile quad flat package; 100 leads; body 14 14 1.4 mm SOT407-1
LPC1766FBD100 LQFP100 plastic low profile quad flat package; 100 leads; body 14 14 1.4 mm SOT407-1
LPC1765FBD100 LQFP100 plastic low profile quad flat package; 100 leads; body 14 14 1.4 mm SOT407-1
LPC1765FET100 TFBGA100 plastic thin fine-pitch ball grid array package; 100 balls; body 9 9 0.7 mm SOT926-1
LPC1764FBD100 LQFP100 plastic low profile quad flat package; 100 leads; body 14 14 1.4 mm SOT407-1
LPC1763FBD100 LQFP100 plastic low profile quad flat package; 100 leads; body 14 14 1.4 mm SOT407-1
Table 2. Ordering options
Type number Flash SRAM in kB Ethernet USB CAN I2S DAC Maximum
CPU
operating
frequency
CPU AHB
SRAM0
AHB
SRAM1
Total
LPC1769FBD100 512 kB 32 16 16 64 yes Device/Host/OTG 2 yes yes 120 MHz
LPC1768FBD100 512 kB 32 16 16 64 yes Device/Host/OTG 2 yes yes 100 MHz
LPC1768FET100 512 kB 32 16 16 64 yes Device/Host/OTG 2 yes yes 100 MHz
LPC1768UK 512 kB 32 16 16 64 yes Device/Host/OTG 2 yes yes 100 MHz
LPC1767FBD100 512 kB 32 16 16 64 yes no no yes yes 100 MHz
LPC1766FBD100 256 kB 32 16 16 64 yes Device/Host/OTG 2 yes yes 100 MHz
LPC1765FBD100 256 kB 32 16 16 64 no Device/Host/OTG 2 yes yes 100 MHz
LPC1765FET100 256 kB 32 16 16 64 no Device/Host/OTG 2 yes yes 100 MHz
LPC1764FBD100 128 kB 16 16 - 32 yes Device only 2 no no 100 MHz
LPC1763FBD100 256 kB 32 16 16 64 no no no yes yes 100 MHz
LPC1769_68_67_66_65_64_63 All information provided in this document is subject to legal disclaimers. © NXP Semiconductors N.V. 2014. All rights reserved.
Product data sheet Rev. 9.4 — 4 April 2014 5 of 88
NXP Semiconductors LPC1769/68/67/66/65/64/63
32-bit ARM Cortex-M3 microcontroller
5. Marking
The LPC176x devices typically have the following top-side marking:
LPC176xxxx
xxxxxxx
xxYYWWR[x]
The last/second to last letter in the third line (field ‘R’) will identify the device revision. This
data sheet covers the following revisions of the LPC176x:
Field ‘YY’ states the year the device was manufactured. Field ‘WW’ states the week the
device was manufactured during that year.
Table 3. Device revision table
Revision identifier (R) Revision description
‘-’ Initial device revision
‘A’ Second device revision
‘B’ Third device revision
LPC1769_68_67_66_65_64_63 All information provided in this document is subject to legal disclaimers. © NXP Semiconductors N.V. 2014. All rights reserved.
Product data sheet Rev. 9.4 — 4 April 2014 6 of 88
NXP Semiconductors LPC1769/68/67/66/65/64/63
32-bit ARM Cortex-M3 microcontroller
6. Block diagram
(1) Not available on all parts. See Table 2.
Fig 1. Block diagram
SRAM 32/64 kB
ARM
CORTEX-M3
TEST/DEBUG
INTERFACE
EMULATION
TRACE MODULE
FLASH
ACCELERATOR
FLASH
512/256/128 kB
DMA
CONTROLLER
ETHERNET
CONTROLLER
WITH DMA(1)
USB HOST/
DEVICE/OTG
CONTROLLER
WITH DMA(1)
I-code
bus
D-code
bus
system
bus
AHB TO
APB
BRIDGE 0
HIGH-SPEED
GPIO
AHB TO
APB
BRIDGE 1
CLOCK
GENERATION,
POWER CONTROL,
SYSTEM
FUNCTIONS
XTAL1
XTAL2
RESET
clocks and
controls
JTAG
interface
debug
port
USB PHY
SSP0
UART2/3
I2S(1)
I2C2
RI TIMER
TIMER2/3
EXTERNAL INTERRUPTS
SYSTEM CONTROL
MOTOR CONTROL PWM
QUADRATURE ENCODER
SSP1
UART0/1
CAN1/2(1)
I2C0/1
SPI0
TIMER 0/1
WDT
PWM1
12-bit ADC
PIN CONNECT
GPIO INTERRUPT CONTROL
RTC
BACKUP REGISTERS
32 kHz
OSCILLATOR
APB slave group 0 APB slave group 1
DAC(1)
RTC POWER DOMAIN
LPC1769/68/67/
66/65/64/63
master master master
002aad944
slave slave slave slave
slave
ROM
slave
MULTILAYER AHB MATRIX
P0 to
P4
SDA2
SCL2
SCK0
SSEL0
MISO0
MOSI0
SCK1
SSEL1
MISO1
MOSI1
RXD2/3
TXD2/3
PHA, PHB
INDEX
EINT[3:0]
AOUT
MCOA[2:0]
MCOB[2:0]
MCI[2:0]
MCABORT
4 × MAT2
2 × MAT3
2 × CAP2
2 × CAP3
3 × I2SRX
3 × I2STX
TX_MCLK
RX_MCLK
RTCX1
RTCX2
VBAT
PWM1[7:0]
2 × MAT0/1
2 × CAP0/1
RD1/2
TD1/2
SDA0/1
SCL0/1
AD0[7:0]
SCK/SSEL
MOSI/MISO
8 × UART1
RXD0/TXD0
P0, P2
PCAP1[1:0]
RMII pins USB pins
CLKOUT
MPU
= connected to DMA
LPC1769_68_67_66_65_64_63 All information provided in this document is subject to legal disclaimers. © NXP Semiconductors N.V. 2014. All rights reserved.
Product data sheet Rev. 9.4 — 4 April 2014 7 of 88
NXP Semiconductors LPC1769/68/67/66/65/64/63
32-bit ARM Cortex-M3 microcontroller
7. Pinning information
7.1 Pinning
Fig 2. Pin configuration LQFP100 package
Fig 3. Pin configuration TFBGA100 package
LPC176xFBD100
50
1
25
75
51
26
76
100
002aad945
002aaf723
LPC1768/65FET100
Transparent top view
J
G
K
H
F
E
D
C
B
A
1 2 3 4 5 6 7 8 9 10
ball A1
index area
LPC1769_68_67_66_65_64_63 All information provided in this document is subject to legal disclaimers. © NXP Semiconductors N.V. 2014. All rights reserved.
Product data sheet Rev. 9.4 — 4 April 2014 8 of 88
NXP Semiconductors LPC1769/68/67/66/65/64/63
32-bit ARM Cortex-M3 microcontroller
Fig 4. Pin configuration WLCSP100 package
Transparent top view
1
A
B
C
D
E
F
G
H
J
K
2 3 4 5 6 7 8 9 10
LPC1768UK
bump A1
index area
aaa-009522
Table 4. Pin allocation table TFBGA100
Pin Symbol Pin Symbol Pin Symbol Pin Symbol
Row A
1 TDO/SWO 2 P0[3]/RXD0/AD0[6] 3 VDD(3V3) 4 P1[4]/ENET_TX_EN
5 P1[10]/ENET_RXD1 6 P1[16]/ENET_MDC 7 VDD(REG)(3V3) 8 P0[4]/I2SRX_CLK/
RD2/CAP2[0]
9 P0[7]/I2STX_CLK/
SCK1/MAT2[1]
10 P0[9]/I2STX_SDA/
MOSI1/MAT2[3]
11 - 12 -
Row B
1 TMS/SWDIO 2 RTCK 3 VSS 4 P1[1]/ENET_TXD1
5 P1[9]/ENET_RXD0 6 P1[17]/
ENET_MDIO
7 VSS 8 P0[6]/I2SRX_SDA/
SSEL1/MAT2[0]
9 P2[0]/PWM1[1]/TXD1 10 P2[1]/PWM1[2]/RXD1 11 - 12 -
Row C
1 TCK/SWDCLK 2 TRST 3 TDI 4 P0[2]/TXD0/AD0[7]
5 P1[8]/ENET_CRS 6 P1[15]/
ENET_REF_CLK
7 P4[28]/RX_MCLK/
MAT2[0]/TXD3
8 P0[8]/I2STX_WS/
MISO1/MAT2[2]
9 VSS 10 VDD(3V3) 11 - 12 -
Row D
1 P0[24]/AD0[1]/
I2SRX_WS/CAP3[1]
2 P0[25]/AD0[2]/
I2SRX_SDA/TXD3
3 P0[26]/AD0[3]/
AOUT/RXD3
4 n.c.
5 P1[0]/ENET_TXD0 6 P1[14]/ENET_RX_ER 7 P0[5]/I2SRX_WS/
TD2/CAP2[1]
8 P2[2]/PWM1[3]/
CTS1/TRACEDATA[3]
9 P2[4]/PWM1[5]/
DSR1/TRACEDATA[1]
10 P2[5]/PWM1[6]/
DTR1/TRACEDATA[0]
11 - 12 -
Row E
1 VSSA 2 VDDA 3 VREFP 4 n.c.
5 P0[23]/AD0[0]/
I2SRX_CLK/CAP3[0]
6 P4[29]/TX_MCLK/
MAT2[1]/RXD3
7 P2[3]/PWM1[4]/
DCD1/TRACEDATA[2]
8 P2[6]/PCAP1[0]/
RI1/TRACECLK
LPC1769_68_67_66_65_64_63 All information provided in this document is subject to legal disclaimers. © NXP Semiconductors N.V. 2014. All rights reserved.
Product data sheet Rev. 9.4 — 4 April 2014 9 of 88
NXP Semiconductors LPC1769/68/67/66/65/64/63
32-bit ARM Cortex-M3 microcontroller
9 P2[7]/RD2/RTS1 10 P2[8]/TD2/TXD2 11 - 12 -
Row F
1 VREFN 2 RTCX1 3 RESET 4 P1[31]/SCK1/
AD0[5]
5 P1[21]/MCABORT/
PWM1[3]/SSEL0
6 P0[18]/DCD1/
MOSI0/MOSI
7 P2[9]/USB_CONNECT/
RXD2
8 P0[16]/RXD1/
SSEL0/SSEL
9 P0[17]/CTS1/
MISO0/MISO
10 P0[15]/TXD1/
SCK0/SCK
11 - 12 -
Row G
1 RTCX2 2 VBAT 3 XTAL2 4 P0[30]/USB_D
5 P1[25]/MCOA1/
MAT1[1]
6 P1[29]/MCOB2/
PCAP1[1]/MAT0[1]
7 VSS 8 P0[21]/RI1/RD1
9 P0[20]/DTR1/SCL1 10 P0[19]/DSR1/SDA1 11 - 12 -
Row H
1 P1[30]/VBUS/
AD0[4]
2 XTAL1 3 P3[25]/MAT0[0]/
PWM1[2]
4 P1[18]/USB_UP_LED/
PWM1[1]/CAP1[0]
5 P1[24]/MCI2/
PWM1[5]/MOSI0
6 VDD(REG)(3V3) 7 P0[10]/TXD2/
SDA2/MAT3[0]
8 P2[11]/EINT1/
I2STX_CLK
9 VDD(3V3) 10 P0[22]/RTS1/TD1 11 - 12 -
Table 4. Pin allocation table TFBGA100 …continued
Pin Symbol Pin Symbol Pin Symbol Pin Symbol
LPC1769_68_67_66_65_64_63 All information provided in this document is subject to legal disclaimers. © NXP Semiconductors N.V. 2014. All rights reserved.
Product data sheet Rev. 9.4 — 4 April 2014 10 of 88
NXP Semiconductors LPC1769/68/67/66/65/64/63
32-bit ARM Cortex-M3 microcontroller
7.2 Pin description
Row J
1 P0[28]/SCL0/
USB_SCL
2 P0[27]/SDA0/
USB_SDA
3 P0[29]/USB_D+ 4 P1[19]/MCOA0/
USB_PPWR/
CAP1[1]
5 P1[22]/MCOB0/
USB_PWRD/
MAT1[0]
6 VSS 7 P1[28]/MCOA2/
PCAP1[0]/
MAT0[0]
8 P0[1]/TD1/RXD3/SCL1
9 P2[13]/EINT3/
I2STX_SDA
10 P2[10]/EINT0/NMI 11 - 12 -
Row K
1 P3[26]/STCLK/
MAT0[1]/PWM1[3]
2 VDD(3V3) 3 VSS 4 P1[20]/MCI0/
PWM1[2]/SCK0
5 P1[23]/MCI1/
PWM1[4]/MISO0
6 P1[26]/MCOB1/
PWM1[6]/CAP0[0]
7 P1[27]/CLKOUT
/USB_OVRCR/
CAP0[1]
8 P0[0]/RD1/TXD3/SDA1
9 P0[11]/RXD2/
SCL2/MAT3[1]
10 P2[12]/EINT2/
I2STX_WS
11 - 12 -
Table 4. Pin allocation table TFBGA100 …continued
Pin Symbol Pin Symbol Pin Symbol Pin Symbol
Table 5. Pin description
Symbol Pin/ball Type Description
LQFP100
TFBGA100
WLCSP100
P0[0] to P0[31] I/O Port 0: Port 0 is a 32-bit I/O port with individual direction controls for
each bit. The operation of port 0 pins depends upon the pin function
selected via the pin connect block. Pins 12, 13, 14, and 31 of this
port are not available.
P0[0]/RD1/TXD3/
SDA1
46 K8 H10 [1] I/O P0[0] — General purpose digital input/output pin.
I RD1 — CAN1 receiver input. (LPC1769/68/66/65/64 only).
O TXD3 — Transmitter output for UART3.
I/O SDA1 — I2C1 data input/output. (This is not an I2C-bus compliant
open-drain pin).
P0[1]/TD1/RXD3/
SCL1
47 J8 H9 [1] I/O P0[1] — General purpose digital input/output pin.
O TD1 — CAN1 transmitter output. (LPC1769/68/66/65/64 only).
I RXD3 — Receiver input for UART3.
I/O SCL1 — I2C1 clock input/output. (This is not an I2C-bus compliant
open-drain pin).
P0[2]/TXD0/AD0[7] 98 C4 B1 [2] I/O P0[2] — General purpose digital input/output pin.
O TXD0 — Transmitter output for UART0.
I AD0[7] — A/D converter 0, input 7.
P0[3]/RXD0/AD0[6] 99 A2 C3 [2] I/O P0[3] — General purpose digital input/output pin.
I RXD0 — Receiver input for UART0.
I AD0[6] — A/D converter 0, input 6.
LPC1769_68_67_66_65_64_63 All information provided in this document is subject to legal disclaimers. © NXP Semiconductors N.V. 2014. All rights reserved.
Product data sheet Rev. 9.4 — 4 April 2014 11 of 88
NXP Semiconductors LPC1769/68/67/66/65/64/63
32-bit ARM Cortex-M3 microcontroller
P0[4]/
I2SRX_CLK/
RD2/CAP2[0]
81 A8 G2 [1] I/O P0[4] — General purpose digital input/output pin.
I/O I2SRX_CLK — Receive Clock. It is driven by the master and
received by the slave. Corresponds to the signal SCK in the I2S-bus
specification. (LPC1769/68/67/66/65/63 only).
I RD2 — CAN2 receiver input. (LPC1769/68/66/65/64 only).
I CAP2[0] — Capture input for Timer 2, channel 0.
P0[5]/
I2SRX_WS/
TD2/CAP2[1]
80 D7 H1 [1] I/O P0[5] — General purpose digital input/output pin.
I/O I2SRX_WS — Receive Word Select. It is driven by the master and
received by the slave. Corresponds to the signal WS in the I2S-bus
specification. (LPC1769/68/67/66/65/63 only).
O TD2 — CAN2 transmitter output. (LPC1769/68/66/65/64 only).
I CAP2[1] — Capture input for Timer 2, channel 1.
P0[6]/
I2SRX_SDA/
SSEL1/MAT2[0]
79 B8 G3 [1] I/O P0[6] — General purpose digital input/output pin.
I/O I2SRX_SDA — Receive data. It is driven by the transmitter and read
by the receiver. Corresponds to the signal SD in the I2S-bus
specification. (LPC1769/68/67/66/65/63 only).
I/O SSEL1 — Slave Select for SSP1.
O MAT2[0] — Match output for Timer 2, channel 0.
P0[7]/
I2STX_CLK/
SCK1/MAT2[1]
78 A9 J1 [1] I/O P0[7] — General purpose digital input/output pin.
I/O I2STX_CLK — Transmit Clock. It is driven by the master and
received by the slave. Corresponds to the signal SCK in the I2S-bus
specification. (LPC1769/68/67/66/65/63 only).
I/O SCK1 — Serial Clock for SSP1.
O MAT2[1] — Match output for Timer 2, channel 1.
P0[8]/
I2STX_WS/
MISO1/MAT2[2]
77 C8 H2 [1] I/O P0[8] — General purpose digital input/output pin.
I/O I2STX_WS — Transmit Word Select. It is driven by the master and
received by the slave. Corresponds to the signal WS in the I2S-bus
specification. (LPC1769/68/67/66/65/63 only).
I/O MISO1 — Master In Slave Out for SSP1.
O MAT2[2] — Match output for Timer 2, channel 2.
P0[9]/
I2STX_SDA/
MOSI1/MAT2[3]
76 A10 H3 [1] I/O P0[9] — General purpose digital input/output pin.
I/O I2STX_SDA — Transmit data. It is driven by the transmitter and
read by the receiver. Corresponds to the signal SD in the I2S-bus
specification. (LPC1769/68/67/66/65/63 only).
I/O MOSI1 — Master Out Slave In for SSP1.
O MAT2[3] — Match output for Timer 2, channel 3.
P0[10]/TXD2/
SDA2/MAT3[0]
48 H7 H8 [1] I/O P0[10] — General purpose digital input/output pin.
O TXD2 — Transmitter output for UART2.
I/O SDA2 — I2C2 data input/output (this is not an open-drain pin).
O MAT3[0] — Match output for Timer 3, channel 0.
Table 5. Pin description …continued
Symbol Pin/ball Type Description
LQFP100
TFBGA100
WLCSP100
LPC1769_68_67_66_65_64_63 All information provided in this document is subject to legal disclaimers. © NXP Semiconductors N.V. 2014. All rights reserved.
Product data sheet Rev. 9.4 — 4 April 2014 12 of 88
NXP Semiconductors LPC1769/68/67/66/65/64/63
32-bit ARM Cortex-M3 microcontroller
P0[11]/RXD2/
SCL2/MAT3[1]
49 K9 J10 [1] I/O P0[11] — General purpose digital input/output pin.
I RXD2 — Receiver input for UART2.
I/O SCL2 — I2C2 clock input/output (this is not an open-drain pin).
O MAT3[1] — Match output for Timer 3, channel 1.
P0[15]/TXD1/
SCK0/SCK
62 F10 H6 [1] I/O P0[15] — General purpose digital input/output pin.
O TXD1 — Transmitter output for UART1.
I/O SCK0 — Serial clock for SSP0.
I/O SCK — Serial clock for SPI.
P0[16]/RXD1/
SSEL0/SSEL
63 F8 J5 [1] I/O P0[16] — General purpose digital input/output pin.
I RXD1 — Receiver input for UART1.
I/O SSEL0 — Slave Select for SSP0.
I/O SSEL — Slave Select for SPI.
P0[17]/CTS1/
MISO0/MISO
61 F9 K6 [1] I/O P0[17] — General purpose digital input/output pin.
I CTS1 — Clear to Send input for UART1.
I/O MISO0 — Master In Slave Out for SSP0.
I/O MISO — Master In Slave Out for SPI.
P0[18]/DCD1/
MOSI0/MOSI
60 F6 J6 [1] I/O P0[18] — General purpose digital input/output pin.
I DCD1 — Data Carrier Detect input for UART1.
I/O MOSI0 — Master Out Slave In for SSP0.
I/O MOSI — Master Out Slave In for SPI.
P0[19]/DSR1/
SDA1
59 G10 K7 [1] I/O P0[19] — General purpose digital input/output pin.
I DSR1 — Data Set Ready input for UART1.
I/O SDA1 — I2C1 data input/output (this is not an I2C-bus compliant
open-drain pin).
P0[20]/DTR1/SCL1 58 G9 J7 [1] I/O P0[20] — General purpose digital input/output pin.
O DTR1 — Data Terminal Ready output for UART1. Can also be
configured to be an RS-485/EIA-485 output enable signal.
I/O SCL1 — I2C1 clock input/output (this is not an I2C-bus compliant
open-drain pin).
P0[21]/RI1/RD1 57 G8 H7 [1] I/O P0[21] — General purpose digital input/output pin.
I RI1 — Ring Indicator input for UART1.
I RD1 — CAN1 receiver input. (LPC1769/68/66/65/64 only).
P0[22]/RTS1/TD1 56 H10 K8 [1] I/O P0[22] — General purpose digital input/output pin.
O RTS1 — Request to Send output for UART1. Can also be
configured to be an RS-485/EIA-485 output enable signal.
O TD1 — CAN1 transmitter output. (LPC1769/68/66/65/64 only).
Table 5. Pin description …continued
Symbol Pin/ball Type Description
LQFP100
TFBGA100
WLCSP100
LPC1769_68_67_66_65_64_63 All information provided in this document is subject to legal disclaimers. © NXP Semiconductors N.V. 2014. All rights reserved.
Product data sheet Rev. 9.4 — 4 April 2014 13 of 88
NXP Semiconductors LPC1769/68/67/66/65/64/63
32-bit ARM Cortex-M3 microcontroller
P0[23]/AD0[0]/
I2SRX_CLK/
CAP3[0]
9 E5 D5 [2] I/O P0[23] — General purpose digital input/output pin.
I AD0[0] — A/D converter 0, input 0.
I/O I2SRX_CLK — Receive Clock. It is driven by the master and
received by the slave. Corresponds to the signal SCK in the I2S-bus
specification. (LPC1769/68/67/66/65/63 only).
I CAP3[0] — Capture input for Timer 3, channel 0.
P0[24]/AD0[1]/
I2SRX_WS/
CAP3[1]
8 D1 B4 [2] I/O P0[24] — General purpose digital input/output pin.
I AD0[1] — A/D converter 0, input 1.
I/O I2SRX_WS — Receive Word Select. It is driven by the master and
received by the slave. Corresponds to the signal WS in the I2S-bus
specification. (LPC1769/68/67/66/65/63 only).
I CAP3[1] — Capture input for Timer 3, channel 1.
P0[25]/AD0[2]/
I2SRX_SDA/
TXD3
7 D2 A3 [2] I/O P0[25] — General purpose digital input/output pin.
I AD0[2] — A/D converter 0, input 2.
I/O I2SRX_SDA — Receive data. It is driven by the transmitter and read
by the receiver. Corresponds to the signal SD in the I2S-bus
specification. (LPC1769/68/67/66/65/63 only).
O TXD3 — Transmitter output for UART3.
P0[26]/AD0[3]/
AOUT/RXD3
6 D3 C5 [3] I/O P0[26] — General purpose digital input/output pin.
I AD0[3] — A/D converter 0, input 3.
O AOUT — DAC output (LPC1769/68/67/66/65/63 only).
I RXD3 — Receiver input for UART3.
P0[27]/SDA0/
USB_SDA
25 J2 C8 [4] I/O P0[27] — General purpose digital input/output pin. Output is
open-drain.
I/O SDA0 — I2C0 data input/output. Open-drain output (for I2C-bus
compliance).
I/O USB_SDA — USB port I2C serial data (OTG transceiver,
LPC1769/68/66/65 only).
P0[28]/SCL0/
USB_SCL
24 J1 B9 [4] I/O P0[28] — General purpose digital input/output pin. Output is
open-drain.
I/O SCL0 — I2C0 clock input/output. Open-drain output (for I2C-bus
compliance).
I/O USB_SCL — USB port I2C serial clock (OTG transceiver,
LPC1769/68/66/65 only).
P0[29]/USB_D+ 29 J3 B10 [5] I/O P0[29] — General purpose digital input/output pin.
I/O USB_D+ — USB bidirectional D+ line. (LPC1769/68/66/65/64 only).
P0[30]/USB_D 30 G4 C9 [5] I/O P0[30] — General purpose digital input/output pin.
I/O USB_D — USB bidirectional D line. (LPC1769/68/66/65/64 only).
Table 5. Pin description …continued
Symbol Pin/ball Type Description
LQFP100
TFBGA100
WLCSP100
LPC1769_68_67_66_65_64_63 All information provided in this document is subject to legal disclaimers. © NXP Semiconductors N.V. 2014. All rights reserved.
Product data sheet Rev. 9.4 — 4 April 2014 14 of 88
NXP Semiconductors LPC1769/68/67/66/65/64/63
32-bit ARM Cortex-M3 microcontroller
P1[0] to P1[31] I/O Port 1: Port 1 is a 32-bit I/O port with individual direction controls for
each bit. The operation of port 1 pins depends upon the pin function
selected via the pin connect block. Pins 2, 3, 5, 6, 7, 11, 12, and 13
of this port are not available.
P1[0]/
ENET_TXD0
95 D5 C1 [1] I/O P1[0] — General purpose digital input/output pin.
O ENET_TXD0 — Ethernet transmit data 0. (LPC1769/68/67/66/64
only).
P1[1]/
ENET_TXD1
94 B4 C2 [1] I/O P1[1] — General purpose digital input/output pin.
O ENET_TXD1 — Ethernet transmit data 1. (LPC1769/68/67/66/64
only).
P1[4]/
ENET_TX_EN
93 A4 D2 [1] I/O P1[4] — General purpose digital input/output pin.
O ENET_TX_EN — Ethernet transmit data enable.
(LPC1769/68/67/66/64 only).
P1[8]/
ENET_CRS
92 C5 D1 [1] I/O P1[8] — General purpose digital input/output pin.
I ENET_CRS — Ethernet carrier sense. (LPC1769/68/67/66/64 only).
P1[9]/
ENET_RXD0
91 B5 D3 [1] I/O P1[9] — General purpose digital input/output pin.
I ENET_RXD0 — Ethernet receive data. (LPC1769/68/67/66/64
only).
P1[10]/
ENET_RXD1
90 A5 E3 [1] I/O P1[10] — General purpose digital input/output pin.
I ENET_RXD1 — Ethernet receive data. (LPC1769/68/67/66/64
only).
P1[14]/
ENET_RX_ER
89 D6 E2 [1] I/O P1[14] — General purpose digital input/output pin.
I ENET_RX_ER — Ethernet receive error. (LPC1769/68/67/66/64
only).
P1[15]/
ENET_REF_CLK
88 C6 E1 [1] I/O P1[15] — General purpose digital input/output pin.
I ENET_REF_CLK — Ethernet reference clock.
(LPC1769/68/67/66/64 only).
P1[16]/
ENET_MDC
87 A6 F3 [1] I/O P1[16] — General purpose digital input/output pin.
O ENET_MDC — Ethernet MIIM clock (LPC1769/68/67/66/64 only).
P1[17]/
ENET_MDIO
86 B6 F2 [1] I/O P1[17] — General purpose digital input/output pin.
I/O ENET_MDIO — Ethernet MIIM data input and output.
(LPC1769/68/67/66/64 only).
Table 5. Pin description …continued
Symbol Pin/ball Type Description
LQFP100
TFBGA100
WLCSP100
LPC1769_68_67_66_65_64_63 All information provided in this document is subject to legal disclaimers. © NXP Semiconductors N.V. 2014. All rights reserved.
Product data sheet Rev. 9.4 — 4 April 2014 15 of 88
NXP Semiconductors LPC1769/68/67/66/65/64/63
32-bit ARM Cortex-M3 microcontroller
P1[18]/
USB_UP_LED/
PWM1[1]/
CAP1[0]
32 H4 D9 [1] I/O P1[18] — General purpose digital input/output pin.
O USB_UP_LED — USB GoodLink LED indicator. It is LOW when the
device is configured (non-control endpoints enabled), or when the
host is enabled and has detected a device on the bus. It is HIGH
when the device is not configured, or when host is enabled and has
not detected a device on the bus, or during global suspend. It
transitions between LOW and HIGH (flashes) when the host is
enabled and detects activity on the bus. (LPC1769/68/66/65/64
only).
O PWM1[1] — Pulse Width Modulator 1, channel 1 output.
I CAP1[0] — Capture input for Timer 1, channel 0.
P1[19]/MCOA0/
USB_PPWR/
CAP1[1]
33 J4 C10 [1] I/O P1[19] — General purpose digital input/output pin.
O MCOA0 — Motor control PWM channel 0, output A.
O USB_PPWR — Port Power enable signal for USB port.
(LPC1769/68/66/65 only).
I CAP1[1] — Capture input for Timer 1, channel 1.
P1[20]/MCI0/
PWM1[2]/SCK0
34 K4 E8 [1] I/O P1[20] — General purpose digital input/output pin.
I MCI0 — Motor control PWM channel 0, input. Also Quadrature
Encoder Interface PHA input.
O PWM1[2] — Pulse Width Modulator 1, channel 2 output.
I/O SCK0 — Serial clock for SSP0.
P1[21]/MCABORT/
PWM1[3]/
SSEL0
35 F5 E9 [1] I/O P1[21] — General purpose digital input/output pin.
O MCABORT — Motor control PWM, LOW-active fast abort.
O PWM1[3] — Pulse Width Modulator 1, channel 3 output.
I/O SSEL0 — Slave Select for SSP0.
P1[22]/MCOB0/
USB_PWRD/
MAT1[0]
36 J5 D10 [1] I/O P1[22] — General purpose digital input/output pin.
O MCOB0 — Motor control PWM channel 0, output B.
I USB_PWRD — Power Status for USB port (host power switch,
LPC1769/68/66/65 only).
O MAT1[0] — Match output for Timer 1, channel 0.
P1[23]/MCI1/
PWM1[4]/MISO0
37 K5 E7 [1] I/O P1[23] — General purpose digital input/output pin.
I MCI1 — Motor control PWM channel 1, input. Also Quadrature
Encoder Interface PHB input.
O PWM1[4] — Pulse Width Modulator 1, channel 4 output.
I/O MISO0 — Master In Slave Out for SSP0.
P1[24]/MCI2/
PWM1[5]/MOSI0
38 H5 F8 [1] I/O P1[24] — General purpose digital input/output pin.
I MCI2 — Motor control PWM channel 2, input. Also Quadrature
Encoder Interface INDEX input.
O PWM1[5] — Pulse Width Modulator 1, channel 5 output.
I/O MOSI0 — Master Out Slave in for SSP0.
Table 5. Pin description …continued
Symbol Pin/ball Type Description
LQFP100
TFBGA100
WLCSP100
LPC1769_68_67_66_65_64_63 All information provided in this document is subject to legal disclaimers. © NXP Semiconductors N.V. 2014. All rights reserved.
Product data sheet Rev. 9.4 — 4 April 2014 16 of 88
NXP Semiconductors LPC1769/68/67/66/65/64/63
32-bit ARM Cortex-M3 microcontroller
P1[25]/MCOA1/
MAT1[1]
39 G5 F9 [1] I/O P1[25] — General purpose digital input/output pin.
O MCOA1 — Motor control PWM channel 1, output A.
O MAT1[1] — Match output for Timer 1, channel 1.
P1[26]/MCOB1/
PWM1[6]/CAP0[0]
40 K6 E10 [1] I/O P1[26] — General purpose digital input/output pin.
O MCOB1 — Motor control PWM channel 1, output B.
O PWM1[6] — Pulse Width Modulator 1, channel 6 output.
I CAP0[0] — Capture input for Timer 0, channel 0.
P1[27]/CLKOUT
/USB_OVRCR/
CAP0[1]
43 K7 G9 [1] I/O P1[27] — General purpose digital input/output pin.
O CLKOUT — Clock output pin.
I USB_OVRCR — USB port Over-Current status. (LPC1769/68/66/65
only).
I CAP0[1] — Capture input for Timer 0, channel 1.
P1[28]/MCOA2/
PCAP1[0]/
MAT0[0]
44 J7 G10 [1] I/O P1[28] — General purpose digital input/output pin.
O MCOA2 — Motor control PWM channel 2, output A.
I PCAP1[0] — Capture input for PWM1, channel 0.
O MAT0[0] — Match output for Timer 0, channel 0.
P1[29]/MCOB2/
PCAP1[1]/
MAT0[1]
45 G6 G8 [1] I/O P1[29] — General purpose digital input/output pin.
O MCOB2 — Motor control PWM channel 2, output B.
I PCAP1[1] — Capture input for PWM1, channel 1.
O MAT0[1] — Match output for Timer 0, channel 1.
P1[30]/VBUS/
AD0[4]
21 H1 B8 [2] I/O P1[30] — General purpose digital input/output pin.
I VBUS — Monitors the presence of USB bus power.
(LPC1769/68/66/65/64 only).
Note: This signal must be HIGH for USB reset to occur.
I AD0[4] — A/D converter 0, input 4.
P1[31]/SCK1/
AD0[5]
20 F4 C7 [2] I/O P1[31] — General purpose digital input/output pin.
I/O SCK1 — Serial Clock for SSP1.
I AD0[5] — A/D converter 0, input 5.
P2[0] to P2[31] I/O Port 2: Port 2 is a 32-bit I/O port with individual direction controls for
each bit. The operation of port 2 pins depends upon the pin function
selected via the pin connect block. Pins 14 through 31 of this port
are not available.
P2[0]/PWM1[1]/
TXD1
75 B9 K1 [1] I/O P2[0] — General purpose digital input/output pin.
O PWM1[1] — Pulse Width Modulator 1, channel 1 output.
O TXD1 — Transmitter output for UART1.
P2[1]/PWM1[2]/
RXD1
74 B10 J2 [1] I/O P2[1] — General purpose digital input/output pin.
O PWM1[2] — Pulse Width Modulator 1, channel 2 output.
I RXD1 — Receiver input for UART1.
Table 5. Pin description …continued
Symbol Pin/ball Type Description
LQFP100
TFBGA100
WLCSP100
LPC1769_68_67_66_65_64_63 All information provided in this document is subject to legal disclaimers. © NXP Semiconductors N.V. 2014. All rights reserved.
Product data sheet Rev. 9.4 — 4 April 2014 17 of 88
NXP Semiconductors LPC1769/68/67/66/65/64/63
32-bit ARM Cortex-M3 microcontroller
P2[2]/PWM1[3]/
CTS1/
TRACEDATA[3]
73 D8 K2 [1] I/O P2[2] — General purpose digital input/output pin.
O PWM1[3] — Pulse Width Modulator 1, channel 3 output.
I CTS1 — Clear to Send input for UART1.
O TRACEDATA[3] — Trace data, bit 3.
P2[3]/PWM1[4]/
DCD1/
TRACEDATA[2]
70 E7 K3 [1] I/O P2[3] — General purpose digital input/output pin.
O PWM1[4] — Pulse Width Modulator 1, channel 4 output.
I DCD1 — Data Carrier Detect input for UART1.
O TRACEDATA[2] — Trace data, bit 2.
P2[4]/PWM1[5]/
DSR1/
TRACEDATA[1]
69 D9 J3 [1] I/O P2[4] — General purpose digital input/output pin.
O PWM1[5] — Pulse Width Modulator 1, channel 5 output.
I DSR1 — Data Set Ready input for UART1.
O TRACEDATA[1] — Trace data, bit 1.
P2[5]/PWM1[6]/
DTR1/
TRACEDATA[0]
68 D10 H4 [1] I/O P2[5] — General purpose digital input/output pin.
O PWM1[6] — Pulse Width Modulator 1, channel 6 output.
O DTR1 — Data Terminal Ready output for UART1. Can also be
configured to be an RS-485/EIA-485 output enable signal.
O TRACEDATA[0] — Trace data, bit 0.
P2[6]/PCAP1[0]/
RI1/TRACECLK
67 E8 K4 [1] I/O P2[6] — General purpose digital input/output pin.
I PCAP1[0] — Capture input for PWM1, channel 0.
I RI1 — Ring Indicator input for UART1.
O TRACECLK — Trace Clock.
P2[7]/RD2/
RTS1
66 E9 J4 [1] I/O P2[7] — General purpose digital input/output pin.
I RD2 — CAN2 receiver input. (LPC1769/68/66/65/64 only).
O RTS1 — Request to Send output for UART1. Can also be
configured to be an RS-485/EIA-485 output enable signal.
P2[8]/TD2/
TXD2
65 E10 H5 [1] I/O P2[8] — General purpose digital input/output pin.
O TD2 — CAN2 transmitter output. (LPC1769/68/66/65/64 only).
O TXD2 — Transmitter output for UART2.
P2[9]/
USB_CONNECT/
RXD2
64 F7 K5 [1] I/O P2[9] — General purpose digital input/output pin.
O USB_CONNECT — Signal used to switch an external 1.5 k
resistor under software control. Used with the SoftConnect USB
feature. (LPC1769/68/66/65/64 only).
I RXD2 — Receiver input for UART2.
P2[10]/EINT0/NMI 53 J10 K9 [6] I/O P2[10] — General purpose digital input/output pin. A LOW level on
this pin during reset starts the ISP command handler.
I EINT0 — External interrupt 0 input.
I NMI — Non-maskable interrupt input.
Table 5. Pin description …continued
Symbol Pin/ball Type Description
LQFP100
TFBGA100
WLCSP100
LPC1769_68_67_66_65_64_63 All information provided in this document is subject to legal disclaimers. © NXP Semiconductors N.V. 2014. All rights reserved.
Product data sheet Rev. 9.4 — 4 April 2014 18 of 88
NXP Semiconductors LPC1769/68/67/66/65/64/63
32-bit ARM Cortex-M3 microcontroller
P2[11]/EINT1/
I2STX_CLK
52 H8 J8 [6] I/O P2[11] — General purpose digital input/output pin.
I EINT1 — External interrupt 1 input.
I/O I2STX_CLK — Transmit Clock. It is driven by the master and
received by the slave. Corresponds to the signal SCK in the I2S-bus
specification. (LPC1769/68/67/66/65/63 only).
P2[12]/EINT2/
I2STX_WS
51 K10 K10 [6] I/O P2[12] — General purpose digital input/output pin.
I EINT2 — External interrupt 2 input.
I/O I2STX_WS — Transmit Word Select. It is driven by the master and
received by the slave. Corresponds to the signal WS in the I2S-bus
specification. (LPC1769/68/67/66/65/63 only).
P2[13]/EINT3/
I2STX_SDA
50 J9 J9 [6] I/O P2[13] — General purpose digital input/output pin.
I EINT3 — External interrupt 3 input.
I/O I2STX_SDA — Transmit data. It is driven by the transmitter and
read by the receiver. Corresponds to the signal SD in the I2S-bus
specification. (LPC1769/68/67/66/65/63 only).
P3[0] to P3[31] I/O Port 3: Port 3 is a 32-bit I/O port with individual direction controls for
each bit. The operation of port 3 pins depends upon the pin function
selected via the pin connect block. Pins 0 through 24, and 27
through 31 of this port are not available.
P3[25]/MAT0[0]/
PWM1[2]
27 H3 D8 [1] I/O P3[25] — General purpose digital input/output pin.
O MAT0[0] — Match output for Timer 0, channel 0.
O PWM1[2] — Pulse Width Modulator 1, output 2.
P3[26]/STCLK/
MAT0[1]/PWM1[3]
26 K1 A10 [1] I/O P3[26] — General purpose digital input/output pin.
I STCLK — System tick timer clock input. The maximum STCLK
frequency is 1/4 of the ARM processor clock frequency CCLK.
O MAT0[1] — Match output for Timer 0, channel 1.
O PWM1[3] — Pulse Width Modulator 1, output 3.
P4[0] to P4[31] I/O Port 4: Port 4 is a 32-bit I/O port with individual direction controls for
each bit. The operation of port 4 pins depends upon the pin function
selected via the pin connect block. Pins 0 through 27, 30, and 31 of
this port are not available.
P4[28]/RX_MCLK/
MAT2[0]/TXD3
82 C7 G1 [1] I/O P4[28] — General purpose digital input/output pin.
O RX_MCLK — I2S receive master clock. (LPC1769/68/67/66/65
only).
O MAT2[0] — Match output for Timer 2, channel 0.
O TXD3 — Transmitter output for UART3.
P4[29]/TX_MCLK/
MAT2[1]/RXD3
85 E6 F1 [1] I/O P4[29] — General purpose digital input/output pin.
O TX_MCLK — I2S transmit master clock. (LPC1769/68/67/66/65
only).
O MAT2[1] — Match output for Timer 2, channel 1.
I RXD3 — Receiver input for UART3.
Table 5. Pin description …continued
Symbol Pin/ball Type Description
LQFP100
TFBGA100
WLCSP100
LPC1769_68_67_66_65_64_63 All information provided in this document is subject to legal disclaimers. © NXP Semiconductors N.V. 2014. All rights reserved.
Product data sheet Rev. 9.4 — 4 April 2014 19 of 88
NXP Semiconductors LPC1769/68/67/66/65/64/63
32-bit ARM Cortex-M3 microcontroller
TDO/SWO 1 A1 A1 [1][7] O TDO — Test Data out for JTAG interface.
O SWO — Serial wire trace output.
TDI 2 C3 C4 [1][8] I TDI — Test Data in for JTAG interface.
TMS/SWDIO 3 B1 B3 [1][8] I TMS — Test Mode Select for JTAG interface.
I/O SWDIO — Serial wire debug data input/output.
TRST 4 C2 A2 [1][8] I TRST — Test Reset for JTAG interface.
TCK/SWDCLK 5 C1 D4 [1][7] I TCK — Test Clock for JTAG interface.
I SWDCLK — Serial wire clock.
RTCK 100 B2 B2 [1][7] O RTCK — JTAG interface control signal.
RSTOUT 14 - - - O RSTOUT — This is a 3.3 V pin. LOW on this pin indicates the
microcontroller being in Reset state.
RESET 17 F3 C6 [9] I External reset input: A LOW-going pulse as short as 50 ns on this
pin resets the device, causing I/O ports and peripherals to take on
their default states, and processor execution to begin at address 0.
TTL with hysteresis, 5 V tolerant.
XTAL1 22 H2 D7 [10][11] I Input to the oscillator circuit and internal clock generator circuits.
XTAL2 23 G3 A9 [10][11] O Output from the oscillator amplifier.
RTCX1 16 F2 A7 [10][11] I Input to the RTC oscillator circuit.
RTCX2 18 G1 B7 [10] O Output from the RTC oscillator circuit.
VSS 31,
41,
55,
72,
83,
97
B3,
B7,
C9,
G7,
J6,
K3
E5,
F5,
F6,
G5,
G6,
G7
[10] I ground: 0 V reference.
VSSA 11 E1 B5 [10] I analog ground: 0 V reference. This should nominally be the same
voltage as VSS, but should be isolated to minimize noise and error.
VDD(3V3) 28,
54,
71,
96
K2,
H9,
C10
, A3
E4,
E6,
F7,
G4
[10] I 3.3 V supply voltage: This is the power supply voltage for the I/O
ports.
VDD(REG)(3V3) 42,
84
H6,
A7
F4,
F0
[10] I 3.3 V voltage regulator supply voltage: This is the supply voltage
for the on-chip voltage regulator only.
VDDA 10 E2 A4 [10] I analog 3.3 V pad supply voltage: This should be nominally the
same voltage as VDD(3V3) but should be isolated to minimize noise
and error. This voltage is used to power the ADC and DAC. This pin
should be tied to 3.3 V if the ADC and DAC are not used.
VREFP 12 E3 A5 [10] I ADC positive reference voltage: This should be nominally the
same voltage as VDDA but should be isolated to minimize noise and
error. Level on this pin is used as a reference for ADC and DAC.
This pin should be tied to 3.3 V if the ADC and DAC are not used.
Table 5. Pin description …continued
Symbol Pin/ball Type Description
LQFP100
TFBGA100
WLCSP100
LPC1769_68_67_66_65_64_63 All information provided in this document is subject to legal disclaimers. © NXP Semiconductors N.V. 2014. All rights reserved.
Product data sheet Rev. 9.4 — 4 April 2014 20 of 88
NXP Semiconductors LPC1769/68/67/66/65/64/63
32-bit ARM Cortex-M3 microcontroller
[1] 5 V tolerant pad providing digital I/O functions with TTL levels and hysteresis. This pin is pulled up to a voltage level of 2.3 V to 2.6 V.
[2] 5 V tolerant pad providing digital I/O functions (with TTL levels and hysteresis) and analog input. When configured as a ADC input,
digital section of the pad is disabled and the pin is not 5 V tolerant. This pin is pulled up to a voltage level of 2.3 V to 2.6 V.
[3] 5 V tolerant pad providing digital I/O with TTL levels and hysteresis and analog output function. When configured as the DAC output,
digital section of the pad is disabled. This pin is pulled up to a voltage level of 2.3 V to 2.6 V.
[4] Open-drain 5 V tolerant digital I/O pad, compatible with I2C-bus 400 kHz specification. This pad requires an external pull-up to provide
output functionality. When power is switched off, this pin connected to the I2C-bus is floating and does not disturb the I2C lines.
Open-drain configuration applies to all functions on this pin.
[5] Pad provides digital I/O and USB functions. It is designed in accordance with the USB specification, revision 2.0 (Full-speed and
Low-speed mode only). This pad is not 5 V tolerant.
[6] 5 V tolerant pad with 10 ns glitch filter providing digital I/O functions with TTL levels and hysteresis. This pin is pulled up to a voltage
level of 2.3 V to 2.6 V.
[7] 5 V tolerant pad with TTL levels and hysteresis. Internal pull-up and pull-down resistors disabled.
[8] 5 V tolerant pad with TTL levels and hysteresis and internal pull-up resistor.
[9] 5 V tolerant pad with 20 ns glitch filter providing digital I/O function with TTL levels and hysteresis.
[10] Pad provides special analog functionality. A 32 kHz crystal oscillator must be used with the RTC.
[11] When the system oscillator is not used, connect XTAL1 and XTAL2 as follows: XTAL1 can be left floating or can be grounded
(grounding is preferred to reduce susceptibility to noise). XTAL2 should be left floating.
[12] When the RTC is not used, connect VBAT to VDD(REG)(3V3) and leave RTCX1 floating.
VREFN 15 F1 A6 I ADC negative reference voltage: This should be nominally the
same voltage as VSS but should be isolated to minimize noise and
error. Level on this pin is used as a reference for ADC and DAC.
VBAT 19 G2 A8 [10][12] I RTC pin power supply: 3.3 V on this pin supplies the power to the
RTC peripheral.
n.c. 13 D4,
E4
B6,
D6
- not connected.
Table 5. Pin description …continued
Symbol Pin/ball Type Description
LQFP100
TFBGA100
WLCSP100
LPC1769_68_67_66_65_64_63 All information provided in this document is subject to legal disclaimers. © NXP Semiconductors N.V. 2014. All rights reserved.
Product data sheet Rev. 9.4 — 4 April 2014 21 of 88
NXP Semiconductors LPC1769/68/67/66/65/64/63
32-bit ARM Cortex-M3 microcontroller
8. Functional description
8.1 Architectural overview
Remark: In the following, the notation LPC17xx refers to all parts:
LPC1769/68/67/66/65/64/63.
The ARM Cortex-M3 includes three AHB-Lite buses: the system bus, the I-code bus, and
the D-code bus (see Figure 1). The I-code and D-code core buses are faster than the
system bus and are used similarly to TCM interfaces: one bus dedicated for instruction
fetch (I-code) and one bus for data access (D-code). The use of two core buses allows for
simultaneous operations if concurrent operations target different devices.
The LPC17xx use a multi-layer AHB matrix to connect the ARM Cortex-M3 buses and
other bus masters to peripherals in a flexible manner that optimizes performance by
allowing peripherals that are on different slaves ports of the matrix to be accessed
simultaneously by different bus masters.
8.2 ARM Cortex-M3 processor
The ARM Cortex-M3 is a general purpose, 32-bit microprocessor, which offers high
performance and very low power consumption. The ARM Cortex-M3 offers many new
features, including a Thumb-2 instruction set, low interrupt latency, hardware divide,
interruptible/continuable multiple load and store instructions, automatic state save and
restore for interrupts, tightly integrated interrupt controller with wake-up interrupt
controller, and multiple core buses capable of simultaneous accesses.
Pipeline techniques are employed so that all parts of the processing and memory systems
can operate continuously. Typically, while one instruction is being executed, its successor
is being decoded, and a third instruction is being fetched from memory.
The ARM Cortex-M3 processor is described in detail in the Cortex-M3 Technical
Reference Manual that can be found on official ARM website.
8.3 On-chip flash program memory
The LPC17xx contain up to 512 kB of on-chip flash memory. A new two-port flash
accelerator maximizes performance for use with the two fast AHB-Lite buses.
8.4 On-chip SRAM
The LPC17xx contain a total of 64 kB on-chip static RAM memory. This includes the main
32 kB SRAM, accessible by the CPU and DMA controller on a higher-speed bus, and two
additional 16 kB each SRAM blocks situated on a separate slave port on the AHB
multilayer matrix.
This architecture allows CPU and DMA accesses to be spread over three separate RAMs
that can be accessed simultaneously.
8.5 Memory Protection Unit (MPU)
The LPC17xx have a Memory Protection Unit (MPU) which can be used to improve the
reliability of an embedded system by protecting critical data within the user application.
LPC1769_68_67_66_65_64_63 All information provided in this document is subject to legal disclaimers. © NXP Semiconductors N.V. 2014. All rights reserved.
Product data sheet Rev. 9.4 — 4 April 2014 22 of 88
NXP Semiconductors LPC1769/68/67/66/65/64/63
32-bit ARM Cortex-M3 microcontroller
The MPU allows separating processing tasks by disallowing access to each other's data,
disabling access to memory regions, allowing memory regions to be defined as read-only
and detecting unexpected memory accesses that could potentially break the system.
The MPU separates the memory into distinct regions and implements protection by
preventing disallowed accesses. The MPU supports up to 8 regions each of which can be
divided into 8 subregions. Accesses to memory locations that are not defined in the MPU
regions, or not permitted by the region setting, will cause the Memory Management Fault
exception to take place.
8.6 Memory map
The LPC17xx incorporates several distinct memory regions, shown in the following
figures. Figure 5 shows the overall map of the entire address space from the user
program viewpoint following reset. The interrupt vector area supports address remapping.
The AHB peripheral area is 2 MB in size and is divided to allow for up to 128 peripherals.
The APB peripheral area is 1 MB in size and is divided to allow for up to 64 peripherals.
Each peripheral of either type is allocated 16 kB of space. This allows simplifying the
address decoding for each peripheral.
xxxxxxxxxxxxxxxxxxxxx xxxxxxxxxxxxxxxxxxxxxxxxxx xxxxxxx x x x xxxxxxxxxxxxxxxxxxxxxxxxxxxxxx xxxxxxxxxxxxxxxxxxx xx xx xxxxx
xxxxxxxxxxxxxxxxxxxxxxxxxxx xxxxxxxxxxxxxxxxxxx xxxxxx xxxxxxxxxxxxxxxxxxxxxxxxxxxxxxxxxxx xxxxxxxxxxxx x x
xxxxxxxxxxxxxxxxxxxxx xxxxxxxxxxxxxxxxxxxxxxxxxxxxxx xxxxx xxxxxxxxxxxxxxxxxxxxxxxxxxxxxxxxxxxxxxxxxxxxxxxxxx xxxxxxxx
xxxxxxxxxxxxxxxxxxxxxxxxx xxxxxxxxxxxxxxxxxxxx xxx
LPC1769_68_67_66_65_64_63 All information provided in this document is subject to legal disclaimers. © NXP Semiconductors N.V. 2014. All rights reserved.
Product data sheet Rev. 9.4 — 4 April 2014 23 of 88
NXP Semiconductors LPC1769/68/67/66/65/64/63
32-bit ARM Cortex-M3 microcontroller
(1) Not available on all parts. See Table 2.
Fig 5. LPC17xx memory map
0x5000 0000
0x5000 4000
0x5000 8000
0x5000 C000
0x5020 0000
0x5001 0000
AHB peripherals
Ethernet controller(1)
USB controller(1)
reserved
127- 4 reserved
GPDMA controller
0
1
2
3
APB0 peripherals
0x4000 4000
0x4000 8000
0x4000 C000
0x4001 0000
0x4001 8000
0x4002 0000
0x4002 8000
0x4002 C000
0x4003 4000
0x4003 0000
0x4003 8000
0x4003 C000
0x4004 0000
0x4004 4000
0x4004 8000
0x4004 C000
0x4005 C000
0x4006 0000
0x4008 0000
0x4002 4000
0x4001 C000
0x4001 4000
WDT 0x4000 0000
timer 0
timer 1
UART0
UART1
reserved
reserved
SPI
RTC + backup registers
GPIO interrupts
pin connect
SSP1
ADC
CAN AF RAM(1)
CAN AF registers(1)
CAN common(1)
CAN1(1)
CAN2(1)
22 - 19 reserved
I2C1
31 - 24 reserved
0
1
2
3
4
5
6
7
8
9
10
11
12
13
14
15
16
17
18
23
reserved
reserved
32 kB local SRAM (LPC1769/8/7/6/5/3)
16 kB local SRAM (LPC1764)
reserved
reserved
private peripheral bus
0 GB 0x0000 0000
0.5 GB
4 GB
1 GB
0x0004 0000
0x0002 0000
0x0008 0000
0x1000 4000
0x1000 0000
0x1000 8000
0x1FFF 0000
0x1FFF 2000
0x2008 0000
0x2007 C000
0x2008 4000
0x2200 0000
0x200A 0000
0x2009 C000
0x2400 0000
0x4000 0000
0x4008 0000
0x4010 0000
0x4200 0000
0x4400 0000
0x5000 0000
0x5020 0000
0xE000 0000
0xE010 0000
0xFFFF FFFF
reserved
reserved
GPIO
reserved
reserved
reserved
reserved
APB0 peripherals
AHB peripherals
APB1 peripherals
AHB SRAM bit-band alias addressing
peripheral bit-band alias addressing
16 kB AHB SRAM1 (LPC1769/8/7/6/5)
16 kB AHB SRAM0
256 kB on-chip flash (LPC1766/65/63)
128 kB on-chip flash (LPC1764)
512 kB on-chip flash (LPC1769/8/7)
PWM1
8 kB boot ROM
0x0000 0000
0x0000 0400
active interrupt vectors
+ 256 words
I-code/D-code
memory space
002aad946
APB1 peripherals
0x4008 0000
0x4008 8000
0x4008 C000
0x4009 0000
0x4009 4000
0x4009 8000
0x4009 C000
0x400A 0000
0x400A 4000
0x400A 8000
0x400A C000
0x400B 0000
0x400B 4000
0x400B 8000
0x400B C000
0x400C 0000
0x400F C000
0x4010 0000
SSP0
DAC(1)
timer 2
timer 3
UART2
UART3
reserved
I2S(1)
I2C2
1 - 0 reserved
2
3
4
5
6
7
8
9
10
reserved
repetitive interrupt timer
11
12
reserved
motor control PWM
30 - 16 reserved
13
14
15
31 system control
QEI
LPC1769/68/67/66/65/64/63
LPC1769_68_67_66_65_64_63 All information provided in this document is subject to legal disclaimers. © NXP Semiconductors N.V. 2014. All rights reserved.
Product data sheet Rev. 9.4 — 4 April 2014 24 of 88
NXP Semiconductors LPC1769/68/67/66/65/64/63
32-bit ARM Cortex-M3 microcontroller
8.7 Nested Vectored Interrupt Controller (NVIC)
The NVIC is an integral part of the Cortex-M3. The tight coupling to the CPU allows for low
interrupt latency and efficient processing of late arriving interrupts.
8.7.1 Features
• Controls system exceptions and peripheral interrupts
• In the LPC17xx, the NVIC supports 33 vectored interrupts
• 32 programmable interrupt priority levels, with hardware priority level masking
• Relocatable vector table
• Non-Maskable Interrupt (NMI)
• Software interrupt generation
8.7.2 Interrupt sources
Each peripheral device has one interrupt line connected to the NVIC but may have several
interrupt flags. Individual interrupt flags may also represent more than one interrupt
source.
Any pin on Port 0 and Port 2 (total of 42 pins) regardless of the selected function, can be
programmed to generate an interrupt on a rising edge, a falling edge, or both.
8.8 Pin connect block
The pin connect block allows selected pins of the microcontroller to have more than one
function. Configuration registers control the multiplexers to allow connection between the
pin and the on-chip peripherals.
Peripherals should be connected to the appropriate pins prior to being activated and prior
to any related interrupt(s) being enabled. Activity of any enabled peripheral function that is
not mapped to a related pin should be considered undefined.
Most pins can also be configured as open-drain outputs or to have a pull-up, pull-down, or
no resistor enabled.
8.9 General purpose DMA controller
The GPDMA is an AMBA AHB compliant peripheral allowing selected peripherals to have
DMA support.
The GPDMA enables peripheral-to-memory, memory-to-peripheral,
peripheral-to-peripheral, and memory-to-memory transactions. The source and
destination areas can each be either a memory region or a peripheral, and can be
accessed through the AHB master. The GPDMA controller allows data transfers between
the USB and Ethernet controllers and the various on-chip SRAM areas. The supported
APB peripherals are SSP0/1, all UARTs, the I2S-bus interface, the ADC, and the DAC.
Two match signals for each timer can be used to trigger DMA transfers.
Remark: The Ethernet controller is available on parts LPC1769/68/67/66/64. The USB
controller is available on parts LPC1769/68/66/65/64. The I2S-bus interface is available on
parts LPC1769/68/67/66/65. The DAC is available on parts LPC1769/68/67/66/65/63.
LPC1769_68_67_66_65_64_63 All information provided in this document is subject to legal disclaimers. © NXP Semiconductors N.V. 2014. All rights reserved.
Product data sheet Rev. 9.4 — 4 April 2014 25 of 88
NXP Semiconductors LPC1769/68/67/66/65/64/63
32-bit ARM Cortex-M3 microcontroller
8.9.1 Features
• Eight DMA channels. Each channel can support an unidirectional transfer.
• 16 DMA request lines.
• Single DMA and burst DMA request signals. Each peripheral connected to the DMA
Controller can assert either a burst DMA request or a single DMA request. The DMA
burst size is set by programming the DMA Controller.
• Memory-to-memory, memory-to-peripheral, peripheral-to-memory, and
peripheral-to-peripheral transfers are supported.
• Scatter or gather DMA is supported through the use of linked lists. This means that
the source and destination areas do not have to occupy contiguous areas of memory.
• Hardware DMA channel priority.
• AHB slave DMA programming interface. The DMA Controller is programmed by
writing to the DMA control registers over the AHB slave interface.
• One AHB bus master for transferring data. The interface transfers data when a DMA
request goes active.
• 32-bit AHB master bus width.
• Incrementing or non-incrementing addressing for source and destination.
• Programmable DMA burst size. The DMA burst size can be programmed to more
efficiently transfer data.
• Internal four-word FIFO per channel.
• Supports 8, 16, and 32-bit wide transactions.
• Big-endian and little-endian support. The DMA Controller defaults to little-endian
mode on reset.
• An interrupt to the processor can be generated on a DMA completion or when a DMA
error has occurred.
• Raw interrupt status. The DMA error and DMA count raw interrupt status can be read
prior to masking.
8.10 Fast general purpose parallel I/O
Device pins that are not connected to a specific peripheral function are controlled by the
GPIO registers. Pins may be dynamically configured as inputs or outputs. Separate
registers allow setting or clearing any number of outputs simultaneously. The value of the
output register may be read back as well as the current state of the port pins.
LPC17xx use accelerated GPIO functions:
• GPIO registers are accessed through the AHB multilayer bus so that the fastest
possible I/O timing can be achieved.
• Mask registers allow treating sets of port bits as a group, leaving other bits
unchanged.
• All GPIO registers are byte and half-word addressable.
• Entire port value can be written in one instruction.
• Support for Cortex-M3 bit banding.
• Support for use with the GPDMA controller.
LPC1769_68_67_66_65_64_63 All information provided in this document is subject to legal disclaimers. © NXP Semiconductors N.V. 2014. All rights reserved.
Product data sheet Rev. 9.4 — 4 April 2014 26 of 88
NXP Semiconductors LPC1769/68/67/66/65/64/63
32-bit ARM Cortex-M3 microcontroller
Additionally, any pin on Port 0 and Port 2 (total of 42 pins) providing a digital function can
be programmed to generate an interrupt on a rising edge, a falling edge, or both. The
edge detection is asynchronous, so it may operate when clocks are not present such as
during Power-down mode. Each enabled interrupt can be used to wake up the chip from
Power-down mode.
8.10.1 Features
• Bit level set and clear registers allow a single instruction to set or clear any number of
bits in one port.
• Direction control of individual bits.
• All I/O default to inputs after reset.
• Pull-up/pull-down resistor configuration and open-drain configuration can be
programmed through the pin connect block for each GPIO pin.
8.11 Ethernet
Remark: The Ethernet controller is available on parts LPC1769/68/67/66/64. The
Ethernet block supports bus clock rates of up to 100 MHz (LPC1768/67/66/64) or 120
MHz (LPC1769). See Table 2.
The Ethernet block contains a full featured 10 Mbit/s or 100 Mbit/s Ethernet MAC
designed to provide optimized performance through the use of DMA hardware
acceleration. Features include a generous suite of control registers, half or full duplex
operation, flow control, control frames, hardware acceleration for transmit retry, receive
packet filtering and wake-up on LAN activity. Automatic frame transmission and reception
with scatter-gather DMA off-loads many operations from the CPU.
The Ethernet block and the CPU share the ARM Cortex-M3 D-code and system bus
through the AHB-multilayer matrix to access the various on-chip SRAM blocks for
Ethernet data, control, and status information.
The Ethernet block interfaces between an off-chip Ethernet PHY using the Reduced MII
(RMII) protocol and the on-chip Media Independent Interface Management (MIIM) serial
bus.
8.11.1 Features
• Ethernet standards support:
– Supports 10 Mbit/s or 100 Mbit/s PHY devices including 10 Base-T, 100 Base-TX,
100 Base-FX, and 100 Base-T4.
– Fully compliant with IEEE standard 802.3.
– Fully compliant with 802.3x full duplex flow control and half duplex back pressure.
– Flexible transmit and receive frame options.
– Virtual Local Area Network (VLAN) frame support.
• Memory management:
– Independent transmit and receive buffers memory mapped to shared SRAM.
– DMA managers with scatter/gather DMA and arrays of frame descriptors.
– Memory traffic optimized by buffering and pre-fetching.
LPC1769_68_67_66_65_64_63 All information provided in this document is subject to legal disclaimers. © NXP Semiconductors N.V. 2014. All rights reserved.
Product data sheet Rev. 9.4 — 4 April 2014 27 of 88
NXP Semiconductors LPC1769/68/67/66/65/64/63
32-bit ARM Cortex-M3 microcontroller
• Enhanced Ethernet features:
– Receive filtering.
– Multicast and broadcast frame support for both transmit and receive.
– Optional automatic Frame Check Sequence (FCS) insertion with Cyclic
Redundancy Check (CRC) for transmit.
– Selectable automatic transmit frame padding.
– Over-length frame support for both transmit and receive allows any length frames.
– Promiscuous receive mode.
– Automatic collision back-off and frame retransmission.
– Includes power management by clock switching.
– Wake-on-LAN power management support allows system wake-up: using the
receive filters or a magic frame detection filter.
• Physical interface:
– Attachment of external PHY chip through standard RMII interface.
– PHY register access is available via the MIIM interface.
8.12 USB interface
Remark: The USB controller is available as device/Host/OTG controller on parts
LPC1769/68/66/65 and as device-only controller on part LPC1764.
The Universal Serial Bus (USB) is a 4-wire bus that supports communication between a
host and one or more (up to 127) peripherals. The host controller allocates the USB
bandwidth to attached devices through a token-based protocol. The bus supports hot
plugging and dynamic configuration of the devices. All transactions are initiated by the
host controller.
The USB interface includes a device, Host, and OTG controller with on-chip PHY for
device and Host functions. The OTG switching protocol is supported through the use of an
external controller. Details on typical USB interfacing solutions can be found in
Section 15.1.
8.12.1 USB device controller
The device controller enables 12 Mbit/s data exchange with a USB Host controller. It
consists of a register interface, serial interface engine, endpoint buffer memory, and a
DMA controller. The serial interface engine decodes the USB data stream and writes data
to the appropriate endpoint buffer. The status of a completed USB transfer or error
condition is indicated via status registers. An interrupt is also generated if enabled. When
enabled, the DMA controller transfers data between the endpoint buffer and the on-chip
SRAM.
8.12.1.1 Features
• Fully compliant with USB 2.0 specification (full speed).
• Supports 32 physical (16 logical) endpoints with a 4 kB endpoint buffer RAM.
• Supports Control, Bulk, Interrupt and Isochronous endpoints.
• Scalable realization of endpoints at run time.
LPC1769_68_67_66_65_64_63 All information provided in this document is subject to legal disclaimers. © NXP Semiconductors N.V. 2014. All rights reserved.
Product data sheet Rev. 9.4 — 4 April 2014 28 of 88
NXP Semiconductors LPC1769/68/67/66/65/64/63
32-bit ARM Cortex-M3 microcontroller
• Endpoint Maximum packet size selection (up to USB maximum specification) by
software at run time.
• Supports SoftConnect and GoodLink features.
• While USB is in the Suspend mode, the part can enter one of the reduced power
modes and wake up on USB activity.
• Supports DMA transfers with all on-chip SRAM blocks on all non-control endpoints.
• Allows dynamic switching between CPU-controlled slave and DMA modes.
• Double buffer implementation for Bulk and Isochronous endpoints.
8.12.2 USB host controller
The host controller enables full- and low-speed data exchange with USB devices attached
to the bus. It consists of a register interface, a serial interface engine, and a DMA
controller. The register interface complies with the OHCI specification.
8.12.2.1 Features
• OHCI compliant.
• One downstream port.
• Supports port power switching.
8.12.3 USB OTG controller
USB OTG is a supplement to the USB 2.0 specification that augments the capability of
existing mobile devices and USB peripherals by adding host functionality for connection to
USB peripherals.
The OTG Controller integrates the host controller, device controller, and a master-only
I2C-bus interface to implement OTG dual-role device functionality. The dedicated I2C-bus
interface controls an external OTG transceiver.
8.12.3.1 Features
• Fully compliant with On-The-Go supplement to the USB 2.0 Specification, Revision
1.0a.
• Hardware support for Host Negotiation Protocol (HNP).
• Includes a programmable timer required for HNP and Session Request Protocol
(SRP).
• Supports any OTG transceiver compliant with the OTG Transceiver Specification
(CEA-2011), Rev. 1.0.
8.13 CAN controller and acceptance filters
Remark: The CAN controllers are available on parts LPC1769/68/66/65/64. See Table 2.
The Controller Area Network (CAN) is a serial communications protocol which efficiently
supports distributed real-time control with a very high level of security. Its domain of
application ranges from high-speed networks to low cost multiplex wiring.
The CAN block is intended to support multiple CAN buses simultaneously, allowing the
device to be used as a gateway, switch, or router among a number of CAN buses in
industrial or automotive applications.
LPC1769_68_67_66_65_64_63 All information provided in this document is subject to legal disclaimers. © NXP Semiconductors N.V. 2014. All rights reserved.
Product data sheet Rev. 9.4 — 4 April 2014 29 of 88
NXP Semiconductors LPC1769/68/67/66/65/64/63
32-bit ARM Cortex-M3 microcontroller
8.13.1 Features
• Two CAN controllers and buses.
• Data rates to 1 Mbit/s on each bus.
• 32-bit register and RAM access.
• Compatible with CAN specification 2.0B, ISO 11898-1.
• Global Acceptance Filter recognizes standard (11-bit) and extended-frame (29-bit)
receive identifiers for all CAN buses.
• Acceptance Filter can provide FullCAN-style automatic reception for selected
Standard Identifiers.
• FullCAN messages can generate interrupts.
8.14 12-bit ADC
The LPC17xx contain a single 12-bit successive approximation ADC with eight channels
and DMA support.
8.14.1 Features
• 12-bit successive approximation ADC.
• Input multiplexing among 8 pins.
• Power-down mode.
• Measurement range VREFN to VREFP.
• 12-bit conversion rate: 200 kHz.
• Individual channels can be selected for conversion.
• Burst conversion mode for single or multiple inputs.
• Optional conversion on transition of input pin or Timer Match signal.
• Individual result registers for each ADC channel to reduce interrupt overhead.
• DMA support.
8.15 10-bit DAC
The DAC allows to generate a variable analog output. The maximum output value of the
DAC is VREFP.
Remark: The DAC is available on parts LPC1769/68/67/66/65/63. See Table 2.
8.15.1 Features
• 10-bit DAC
• Resistor string architecture
• Buffered output
• Power-down mode
• Selectable output drive
• Dedicated conversion timer
• DMA support
LPC1769_68_67_66_65_64_63 All information provided in this document is subject to legal disclaimers. © NXP Semiconductors N.V. 2014. All rights reserved.
Product data sheet Rev. 9.4 — 4 April 2014 30 of 88
NXP Semiconductors LPC1769/68/67/66/65/64/63
32-bit ARM Cortex-M3 microcontroller
8.16 UARTs
The LPC17xx each contain four UARTs. In addition to standard transmit and receive data
lines, UART1 also provides a full modem control handshake interface and support for
RS-485/9-bit mode allowing both software address detection and automatic address
detection using 9-bit mode.
The UARTs include a fractional baud rate generator. Standard baud rates such as
115200 Bd can be achieved with any crystal frequency above 2 MHz.
8.16.1 Features
• Maximum UART data bit rate of 6.25 Mbit/s.
• 16 B Receive and Transmit FIFOs.
• Register locations conform to 16C550 industry standard.
• Receiver FIFO trigger points at 1 B, 4 B, 8 B, and 14 B.
• Built-in fractional baud rate generator covering wide range of baud rates without a
need for external crystals of particular values.
• Auto baud capabilities and FIFO control mechanism that enables software flow
control implementation.
• UART1 equipped with standard modem interface signals. This module also provides
full support for hardware flow control (auto-CTS/RTS).
• Support for RS-485/9-bit/EIA-485 mode (UART1).
• UART3 includes an IrDA mode to support infrared communication.
• All UARTs have DMA support.
8.17 SPI serial I/O controller
The LPC17xx contain one SPI controller. SPI is a full duplex serial interface designed to
handle multiple masters and slaves connected to a given bus. Only a single master and a
single slave can communicate on the interface during a given data transfer. During a data
transfer the master always sends 8 bits to 16 bits of data to the slave, and the slave
always sends 8 bits to 16 bits of data to the master.
8.17.1 Features
• Maximum SPI data bit rate of 12.5 Mbit/s
• Compliant with SPI specification
• Synchronous, serial, full duplex communication
• Combined SPI master and slave
• Maximum data bit rate of one eighth of the input clock rate
• 8 bits to 16 bits per transfer
8.18 SSP serial I/O controller
The LPC17xx contain two SSP controllers. The SSP controller is capable of operation on
a SPI, 4-wire SSI, or Microwire bus. It can interact with multiple masters and slaves on the
bus. Only a single master and a single slave can communicate on the bus during a given
LPC1769_68_67_66_65_64_63 All information provided in this document is subject to legal disclaimers. © NXP Semiconductors N.V. 2014. All rights reserved.
Product data sheet Rev. 9.4 — 4 April 2014 31 of 88
NXP Semiconductors LPC1769/68/67/66/65/64/63
32-bit ARM Cortex-M3 microcontroller
data transfer. The SSP supports full duplex transfers, with frames of 4 bits to 16 bits of
data flowing from the master to the slave and from the slave to the master. In practice,
often only one of these data flows carries meaningful data.
8.18.1 Features
• Maximum SSP speed of 50 Mbit/s (master) or 8 Mbit/s (slave)
• Compatible with Motorola SPI, 4-wire Texas Instruments SSI, and National
Semiconductor Microwire buses
• Synchronous serial communication
• Master or slave operation
• 8-frame FIFOs for both transmit and receive
• 4-bit to 16-bit frame
• DMA transfers supported by GPDMA
8.19 I2C-bus serial I/O controllers
The LPC17xx each contain three I2C-bus controllers.
The I2C-bus is bidirectional for inter-IC control using only two wires: a Serial Clock line
(SCL) and a Serial DAta line (SDA). Each device is recognized by a unique address and
can operate as either a receiver-only device (e.g., an LCD driver) or a transmitter with the
capability to both receive and send information (such as memory). Transmitters and/or
receivers can operate in either master or slave mode, depending on whether the chip has
to initiate a data transfer or is only addressed. The I2C is a multi-master bus and can be
controlled by more than one bus master connected to it.
8.19.1 Features
• I2C0 is a standard I2C compliant bus interface with open-drain pins. I2C0 also
supports Fast mode plus with bit rates up to 1 Mbit/s.
• I2C1 and I2C2 use standard I/O pins with bit rates of up to 400 kbit/s (Fast I2C-bus).
• Easy to configure as master, slave, or master/slave.
• Programmable clocks allow versatile rate control.
• Bidirectional data transfer between masters and slaves.
• Multi-master bus (no central master).
• Arbitration between simultaneously transmitting masters without corruption of serial
data on the bus.
• Serial clock synchronization allows devices with different bit rates to communicate via
one serial bus.
• Serial clock synchronization can be used as a handshake mechanism to suspend and
resume serial transfer.
• The I2C-bus can be used for test and diagnostic purposes.
• All I2C-bus controllers support multiple address recognition and a bus monitor mode.
LPC1769_68_67_66_65_64_63 All information provided in this document is subject to legal disclaimers. © NXP Semiconductors N.V. 2014. All rights reserved.
Product data sheet Rev. 9.4 — 4 April 2014 32 of 88
NXP Semiconductors LPC1769/68/67/66/65/64/63
32-bit ARM Cortex-M3 microcontroller
8.20 I2S-bus serial I/O controllers
Remark: The I2S-bus interface is available on parts LPC1769/68/67/66/65/63. See
Table 2.
The I2S-bus provides a standard communication interface for digital audio applications.
The I2S-bus specification defines a 3-wire serial bus using one data line, one clock line,
and one word select signal. The basic I2S-bus connection has one master, which is
always the master, and one slave. The I2S-bus interface provides a separate transmit and
receive channel, each of which can operate as either a master or a slave.
8.20.1 Features
• The interface has separate input/output channels each of which can operate in master
or slave mode.
• Capable of handling 8-bit, 16-bit, and 32-bit word sizes.
• Mono and stereo audio data supported.
• The sampling frequency can range from 16 kHz to 96 kHz (16, 22.05, 32, 44.1, 48,
96) kHz.
• Support for an audio master clock.
• Configurable word select period in master mode (separately for I2S-bus input and
output).
• Two 8-word FIFO data buffers are provided, one for transmit and one for receive.
• Generates interrupt requests when buffer levels cross a programmable boundary.
• Two DMA requests, controlled by programmable buffer levels. These are connected
to the GPDMA block.
• Controls include reset, stop and mute options separately for I2S-bus input and I2S-bus
output.
8.21 General purpose 32-bit timers/external event counters
The LPC17xx include four 32-bit timer/counters. The timer/counter is designed to count
cycles of the system derived clock or an externally-supplied clock. It can optionally
generate interrupts, generate timed DMA requests, or perform other actions at specified
timer values, based on four match registers. Each timer/counter also includes two capture
inputs to trap the timer value when an input signal transitions, optionally generating an
interrupt.
8.21.1 Features
• A 32-bit timer/counter with a programmable 32-bit prescaler.
• Counter or timer operation.
• Two 32-bit capture channels per timer, that can take a snapshot of the timer value
when an input signal transitions. A capture event may also generate an interrupt.
• Four 32-bit match registers that allow:
– Continuous operation with optional interrupt generation on match.
– Stop timer on match with optional interrupt generation.
– Reset timer on match with optional interrupt generation.
LPC1769_68_67_66_65_64_63 All information provided in this document is subject to legal disclaimers. © NXP Semiconductors N.V. 2014. All rights reserved.
Product data sheet Rev. 9.4 — 4 April 2014 33 of 88
NXP Semiconductors LPC1769/68/67/66/65/64/63
32-bit ARM Cortex-M3 microcontroller
• Up to four external outputs corresponding to match registers, with the following
capabilities:
– Set LOW on match.
– Set HIGH on match.
– Toggle on match.
– Do nothing on match.
• Up to two match registers can be used to generate timed DMA requests.
8.22 Pulse width modulator
The PWM is based on the standard Timer block and inherits all of its features, although
only the PWM function is pinned out on the LPC17xx. The Timer is designed to count
cycles of the system derived clock and optionally switch pins, generate interrupts or
perform other actions when specified timer values occur, based on seven match registers.
The PWM function is in addition to these features, and is based on match register events.
The ability to separately control rising and falling edge locations allows the PWM to be
used for more applications. For instance, multi-phase motor control typically requires
three non-overlapping PWM outputs with individual control of all three pulse widths and
positions.
Two match registers can be used to provide a single edge controlled PWM output. One
match register (PWMMR0) controls the PWM cycle rate, by resetting the count upon
match. The other match register controls the PWM edge position. Additional single edge
controlled PWM outputs require only one match register each, since the repetition rate is
the same for all PWM outputs. Multiple single edge controlled PWM outputs will all have a
rising edge at the beginning of each PWM cycle, when an PWMMR0 match occurs.
Three match registers can be used to provide a PWM output with both edges controlled.
Again, the PWMMR0 match register controls the PWM cycle rate. The other match
registers control the two PWM edge positions. Additional double edge controlled PWM
outputs require only two match registers each, since the repetition rate is the same for all
PWM outputs.
With double edge controlled PWM outputs, specific match registers control the rising and
falling edge of the output. This allows both positive going PWM pulses (when the rising
edge occurs prior to the falling edge), and negative going PWM pulses (when the falling
edge occurs prior to the rising edge).
8.22.1 Features
• One PWM block with Counter or Timer operation (may use the peripheral clock or one
of the capture inputs as the clock source).
• Seven match registers allow up to 6 single edge controlled or 3 double edge
controlled PWM outputs, or a mix of both types. The match registers also allow:
– Continuous operation with optional interrupt generation on match.
– Stop timer on match with optional interrupt generation.
– Reset timer on match with optional interrupt generation.
LPC1769_68_67_66_65_64_63 All information provided in this document is subject to legal disclaimers. © NXP Semiconductors N.V. 2014. All rights reserved.
Product data sheet Rev. 9.4 — 4 April 2014 34 of 88
NXP Semiconductors LPC1769/68/67/66/65/64/63
32-bit ARM Cortex-M3 microcontroller
• Supports single edge controlled and/or double edge controlled PWM outputs. Single
edge controlled PWM outputs all go high at the beginning of each cycle unless the
output is a constant low. Double edge controlled PWM outputs can have either edge
occur at any position within a cycle. This allows for both positive going and negative
going pulses.
• Pulse period and width can be any number of timer counts. This allows complete
flexibility in the trade-off between resolution and repetition rate. All PWM outputs will
occur at the same repetition rate.
• Double edge controlled PWM outputs can be programmed to be either positive going
or negative going pulses.
• Match register updates are synchronized with pulse outputs to prevent generation of
erroneous pulses. Software must ‘release’ new match values before they can become
effective.
• May be used as a standard 32-bit timer/counter with a programmable 32-bit prescaler
if the PWM mode is not enabled.
8.23 Motor control PWM
The motor control PWM is a specialized PWM supporting 3-phase motors and other
combinations. Feedback inputs are provided to automatically sense rotor position and use
that information to ramp speed up or down. An abort input is also provided that causes the
PWM to immediately release all motor drive outputs. At the same time, the motor control
PWM is highly configurable for other generalized timing, counting, capture, and compare
applications.
8.24 Quadrature Encoder Interface (QEI)
A quadrature encoder, also known as a 2-channel incremental encoder, converts angular
displacement into two pulse signals. By monitoring both the number of pulses and the
relative phase of the two signals, the user can track the position, direction of rotation, and
velocity. In addition, a third channel, or index signal, can be used to reset the position
counter. The quadrature encoder interface decodes the digital pulses from a quadrature
encoder wheel to integrate position over time and determine direction of rotation. In
addition, the QEI can capture the velocity of the encoder wheel.
8.24.1 Features
• Tracks encoder position.
• Increments/decrements depending on direction.
• Programmable for 2 or 4 position counting.
• Velocity capture using built-in timer.
• Velocity compare function with “less than” interrupt.
• Uses 32-bit registers for position and velocity.
• Three position compare registers with interrupts.
• Index counter for revolution counting.
• Index compare register with interrupts.
• Can combine index and position interrupts to produce an interrupt for whole and
partial revolution displacement.
LPC1769_68_67_66_65_64_63 All information provided in this document is subject to legal disclaimers. © NXP Semiconductors N.V. 2014. All rights reserved.
Product data sheet Rev. 9.4 — 4 April 2014 35 of 88
NXP Semiconductors LPC1769/68/67/66/65/64/63
32-bit ARM Cortex-M3 microcontroller
• Digital filter with programmable delays for encoder input signals.
• Can accept decoded signal inputs (clk and direction).
• Connected to APB.
8.25 Repetitive Interrupt (RI) timer
The repetitive interrupt timer provides a free-running 32-bit counter which is compared to
a selectable value, generating an interrupt when a match occurs. Any bits of the
timer/compare can be masked such that they do not contribute to the match detection.
The repetitive interrupt timer can be used to create an interrupt that repeats at
predetermined intervals.
8.25.1 Features
• 32-bit counter running from PCLK. Counter can be free-running or be reset by a
generated interrupt.
• 32-bit compare value.
• 32-bit compare mask. An interrupt is generated when the counter value equals the
compare value, after masking. This allows for combinations not possible with a simple
compare.
8.26 ARM Cortex-M3 system tick timer
The ARM Cortex-M3 includes a system tick timer (SYSTICK) that is intended to generate
a dedicated SYSTICK exception at a 10 ms interval. In the LPC17xx, this timer can be
clocked from the internal AHB clock or from a device pin.
8.27 Watchdog timer
The purpose of the watchdog is to reset the microcontroller within a reasonable amount of
time if it enters an erroneous state. When enabled, the watchdog will generate a system
reset if the user program fails to ‘feed’ (or reload) the watchdog within a predetermined
amount of time.
8.27.1 Features
• Internally resets chip if not periodically reloaded.
• Debug mode.
• Enabled by software but requires a hardware reset or a watchdog reset/interrupt to be
disabled.
• Incorrect/Incomplete feed sequence causes reset/interrupt if enabled.
• Flag to indicate watchdog reset.
• Programmable 32-bit timer with internal prescaler.
• Selectable time period from (Tcy(WDCLK) 256 4) to (Tcy(WDCLK) 232 4) in
multiples of Tcy(WDCLK) 4.
• The Watchdog Clock (WDCLK) source can be selected from the Internal RC (IRC)
oscillator, the RTC oscillator, or the APB peripheral clock. This gives a wide range of
potential timing choices of Watchdog operation under different power reduction
LPC1769_68_67_66_65_64_63 All information provided in this document is subject to legal disclaimers. © NXP Semiconductors N.V. 2014. All rights reserved.
Product data sheet Rev. 9.4 — 4 April 2014 36 of 88
NXP Semiconductors LPC1769/68/67/66/65/64/63
32-bit ARM Cortex-M3 microcontroller
conditions. It also provides the ability to run the WDT from an entirely internal source
that is not dependent on an external crystal and its associated components and wiring
for increased reliability.
• Includes lock/safe feature.
8.28 RTC and backup registers
The RTC is a set of counters for measuring time when system power is on, and optionally
when it is off. The RTC on the LPC17xx is designed to have extremely low power
consumption, i.e. less than 1 A. The RTC will typically run from the main chip power
supply, conserving battery power while the rest of the device is powered up. When
operating from a battery, the RTC will continue working down to 2.1 V. Battery power can
be provided from a standard 3 V Lithium button cell.
An ultra-low power 32 kHz oscillator will provide a 1 Hz clock to the time counting portion
of the RTC, moving most of the power consumption out of the time counting function.
The RTC includes a calibration mechanism to allow fine-tuning the count rate in a way
that will provide less than 1 second per day error when operated at a constant voltage and
temperature. A clock output function (see Section 8.29.4) makes measuring the oscillator
rate easy and accurate.
The RTC contains a small set of backup registers (20 bytes) for holding data while the
main part of the LPC17xx is powered off.
The RTC includes an alarm function that can wake up the LPC17xx from all reduced
power modes with a time resolution of 1 s.
8.28.1 Features
• Measures the passage of time to maintain a calendar and clock.
• Ultra low power design to support battery powered systems.
• Provides Seconds, Minutes, Hours, Day of Month, Month, Year, Day of Week, and
Day of Year.
• Dedicated power supply pin can be connected to a battery or to the main 3.3 V.
• Periodic interrupts can be generated from increments of any field of the time registers.
• Backup registers (20 bytes) powered by VBAT.
• RTC power supply is isolated from the rest of the chip.
8.29 Clocking and power control
8.29.1 Crystal oscillators
The LPC17xx include three independent oscillators. These are the main oscillator, the IRC
oscillator, and the RTC oscillator. Each oscillator can be used for more than one purpose
as required in a particular application. Any of the three clock sources can be chosen by
software to drive the main PLL and ultimately the CPU.
Following reset, the LPC17xx will operate from the Internal RC oscillator until switched by
software. This allows systems to operate without any external crystal and the bootloader
code to operate at a known frequency.
LPC1769_68_67_66_65_64_63 All information provided in this document is subject to legal disclaimers. © NXP Semiconductors N.V. 2014. All rights reserved.
Product data sheet Rev. 9.4 — 4 April 2014 37 of 88
NXP Semiconductors LPC1769/68/67/66/65/64/63
32-bit ARM Cortex-M3 microcontroller
See Figure 6 for an overview of the LPC17xx clock generation.
8.29.1.1 Internal RC oscillator
The IRC may be used as the clock source for the WDT, and/or as the clock that drives the
PLL and subsequently the CPU. The nominal IRC frequency is 4 MHz. The IRC is
trimmed to 1 % accuracy over the entire voltage and temperature range.
Upon power-up or any chip reset, the LPC17xx use the IRC as the clock source. Software
may later switch to one of the other available clock sources.
8.29.1.2 Main oscillator
The main oscillator can be used as the clock source for the CPU, with or without using the
PLL. The main oscillator also provides the clock source for the dedicated USB PLL.
The main oscillator operates at frequencies of 1 MHz to 25 MHz. This frequency can be
boosted to a higher frequency, up to the maximum CPU operating frequency, by the main
PLL. The clock selected as the PLL input is PLLCLKIN. The ARM processor clock
frequency is referred to as CCLK elsewhere in this document. The frequencies of
PLLCLKIN and CCLK are the same value unless the PLL is active and connected. The
clock frequency for each peripheral can be selected individually and is referred to as
PCLK. Refer to Section 8.29.2 for additional information.
8.29.1.3 RTC oscillator
The RTC oscillator can be used as the clock source for the RTC block, the main PLL,
and/or the CPU.
Fig 6. LPC17xx clocking generation block diagram
MAIN
OSCILLATOR
INTERNAL
RC
OSCILLATOR
RTC
OSCILLATOR
MAIN PLL
WATCHDOG
TIMER
REAL-TIME
CLOCK
CPU
CLOCK
DIVIDER
PERIPHERAL
CLOCK
GENERATOR
USB BLOCK
ARM
CORTEX-M3
ETHERNET
BLOCK
DMA
GPIO
NVIC
USB
CLOCK
DIVIDER
system
clock
select
(CLKSRCSEL)
USB clock config
(USBCLKCFG)
CPU clock config
(CCLKCFG)
pllclk
CCLK/8
CCLK/6
CCLK/4
CCLK/2
CCLK
pclkWDT
rtclk = 1Hz
usbclk
(48 MHz)
cclk
USB PLL
USB PLL enable
main PLL enable
32 kHz
APB peripherals
LPC17xx
002aad947
LPC1769_68_67_66_65_64_63 All information provided in this document is subject to legal disclaimers. © NXP Semiconductors N.V. 2014. All rights reserved.
Product data sheet Rev. 9.4 — 4 April 2014 38 of 88
NXP Semiconductors LPC1769/68/67/66/65/64/63
32-bit ARM Cortex-M3 microcontroller
8.29.2 Main PLL (PLL0)
The PLL0 accepts an input clock frequency in the range of 32 kHz to 25 MHz. The input
frequency is multiplied up to a high frequency, then divided down to provide the actual
clock used by the CPU and/or the USB block.
The PLL0 input, in the range of 32 kHz to 25 MHz, may initially be divided down by a
value ‘N’, which may be in the range of 1 to 256. This input division provides a wide range
of output frequencies from the same input frequency.
Following the PLL0 input divider is the PLL0 multiplier. This can multiply the input divider
output through the use of a Current Controlled Oscillator (CCO) by a value ‘M’, in the
range of 1 through 32768. The resulting frequency must be in the range of 275 MHz to
550 MHz. The multiplier works by dividing the CCO output by the value of M, then using a
phase-frequency detector to compare the divided CCO output to the multiplier input. The
error value is used to adjust the CCO frequency.
The PLL0 is turned off and bypassed following a chip Reset and by entering Power-down
mode. PLL0 is enabled by software only. The program must configure and activate the
PLL0, wait for the PLL0 to lock, and then connect to the PLL0 as a clock source.
8.29.3 USB PLL (PLL1)
The LPC17xx contain a second, dedicated USB PLL1 to provide clocking for the USB
interface.
The PLL1 receives its clock input from the main oscillator only and provides a fixed
48 MHz clock to the USB block only. The PLL1 is disabled and powered off on reset. If the
PLL1 is left disabled, the USB clock will be supplied by the 48 MHz clock from the main
PLL0.
The PLL1 accepts an input clock frequency in the range of 10 MHz to 25 MHz only. The
input frequency is multiplied up the range of 48 MHz for the USB clock using a Current
Controlled Oscillators (CCO). It is insured that the PLL1 output has a 50 % duty cycle.
8.29.4 RTC clock output
The LPC17xx feature a clock output function intended for synchronizing with external
devices and for use during system development to allow checking the internal clocks
CCLK, IRC clock, main crystal, RTC clock, and USB clock in the outside world. The RTC
clock output allows tuning the RTC frequency without probing the pin, which would distort
the results.
8.29.5 Wake-up timer
The LPC17xx begin operation at power-up and when awakened from Power-down mode
by using the 4 MHz IRC oscillator as the clock source. This allows chip operation to
resume quickly. If the main oscillator or the PLL is needed by the application, software will
need to enable these features and wait for them to stabilize before they are used as a
clock source.
When the main oscillator is initially activated, the wake-up timer allows software to ensure
that the main oscillator is fully functional before the processor uses it as a clock source
and starts to execute instructions. This is important at power on, all types of Reset, and
LPC1769_68_67_66_65_64_63 All information provided in this document is subject to legal disclaimers. © NXP Semiconductors N.V. 2014. All rights reserved.
Product data sheet Rev. 9.4 — 4 April 2014 39 of 88
NXP Semiconductors LPC1769/68/67/66/65/64/63
32-bit ARM Cortex-M3 microcontroller
whenever any of the aforementioned functions are turned off for any reason. Since the
oscillator and other functions are turned off during Power-down mode, any wake-up of the
processor from Power-down mode makes use of the wake-up timer.
The Wake-up Timer monitors the crystal oscillator to check whether it is safe to begin
code execution. When power is applied to the chip, or when some event caused the chip
to exit Power-down mode, some time is required for the oscillator to produce a signal of
sufficient amplitude to drive the clock logic. The amount of time depends on many factors,
including the rate of VDD(3V3) ramp (in the case of power on), the type of crystal and its
electrical characteristics (if a quartz crystal is used), as well as any other external circuitry
(e.g., capacitors), and the characteristics of the oscillator itself under the existing ambient
conditions.
8.29.6 Power control
The LPC17xx support a variety of power control features. There are four special modes of
processor power reduction: Sleep mode, Deep-sleep mode, Power-down mode, and
Deep power-down mode. The CPU clock rate may also be controlled as needed by
changing clock sources, reconfiguring PLL values, and/or altering the CPU clock divider
value. This allows a trade-off of power versus processing speed based on application
requirements. In addition, Peripheral Power Control allows shutting down the clocks to
individual on-chip peripherals, allowing fine tuning of power consumption by eliminating all
dynamic power use in any peripherals that are not required for the application. Each of the
peripherals has its own clock divider which provides even better power control.
Integrated PMU (Power Management Unit) automatically adjust internal regulators to
minimize power consumption during Sleep, Deep sleep, Power-down, and Deep
power-down modes.
The LPC17xx also implement a separate power domain to allow turning off power to the
bulk of the device while maintaining operation of the RTC and a small set of registers for
storing data during any of the power-down modes.
8.29.6.1 Sleep mode
When Sleep mode is entered, the clock to the core is stopped. Resumption from the Sleep
mode does not need any special sequence but re-enabling the clock to the ARM core.
In Sleep mode, execution of instructions is suspended until either a Reset or interrupt
occurs. Peripheral functions continue operation during Sleep mode and may generate
interrupts to cause the processor to resume execution. Sleep mode eliminates dynamic
power used by the processor itself, memory systems and related controllers, and internal
buses.
8.29.6.2 Deep-sleep mode
In Deep-sleep mode, the oscillator is shut down and the chip receives no internal clocks.
The processor state and registers, peripheral registers, and internal SRAM values are
preserved throughout Deep-sleep mode and the logic levels of chip pins remain static.
The output of the IRC is disabled but the IRC is not powered down for a fast wake-up later.
The RTC oscillator is not stopped because the RTC interrupts may be used as the
wake-up source. The PLL is automatically turned off and disconnected. The CCLK and
USB clock dividers automatically get reset to zero.
LPC1769_68_67_66_65_64_63 All information provided in this document is subject to legal disclaimers. © NXP Semiconductors N.V. 2014. All rights reserved.
Product data sheet Rev. 9.4 — 4 April 2014 40 of 88
NXP Semiconductors LPC1769/68/67/66/65/64/63
32-bit ARM Cortex-M3 microcontroller
The Deep-sleep mode can be terminated and normal operation resumed by either a
Reset or certain specific interrupts that are able to function without clocks. Since all
dynamic operation of the chip is suspended, Deep-sleep mode reduces chip power
consumption to a very low value. Power to the flash memory is left on in Deep-sleep
mode, allowing a very quick wake-up.
On wake-up from Deep-sleep mode, the code execution and peripherals activities will
resume after 4 cycles expire if the IRC was used before entering Deep-sleep mode. If the
main external oscillator was used, the code execution will resume when 4096 cycles
expire. PLL and clock dividers need to be reconfigured accordingly.
8.29.6.3 Power-down mode
Power-down mode does everything that Deep-sleep mode does, but also turns off the
power to the IRC oscillator and the flash memory. This saves more power but requires
waiting for resumption of flash operation before execution of code or data access in the
flash memory can be accomplished.
On the wake-up of Power-down mode, if the IRC was used before entering Power-down
mode, it will take IRC 60 s to start-up. After this 4 IRC cycles will expire before the code
execution can then be resumed if the code was running from SRAM. In the meantime, the
flash wake-up timer then counts 4 MHz IRC clock cycles to make the 100 s flash start-up
time. When it times out, access to the flash will be allowed. Users need to reconfigure the
PLL and clock dividers accordingly.
8.29.6.4 Deep power-down mode
The Deep power-down mode can only be entered from the RTC block. In Deep
power-down mode, power is shut off to the entire chip with the exception of the RTC
module and the RESET pin.
The LPC17xx can wake up from Deep power-down mode via the RESET pin or an alarm
match event of the RTC.
8.29.6.5 Wake-up interrupt controller
The Wake-up Interrupt Controller (WIC) allows the CPU to automatically wake up from
any enabled priority interrupt that can occur while the clocks are stopped in Deep sleep,
Power-down, and Deep power-down modes.
The WIC works in connection with the Nested Vectored Interrupt Controller (NVIC). When
the CPU enters Deep sleep, Power-down, or Deep power-down mode, the NVIC sends a
mask of the current interrupt situation to the WIC.This mask includes all of the interrupts
that are both enabled and of sufficient priority to be serviced immediately. With this
information, the WIC simply notices when one of the interrupts has occurred and then it
wakes up the CPU.
The WIC eliminates the need to periodically wake up the CPU and poll the interrupts
resulting in additional power savings.
8.29.7 Peripheral power control
A Power Control for Peripherals feature allows individual peripherals to be turned off if
they are not needed in the application, resulting in additional power savings.
LPC1769_68_67_66_65_64_63 All information provided in this document is subject to legal disclaimers. © NXP Semiconductors N.V. 2014. All rights reserved.
Product data sheet Rev. 9.4 — 4 April 2014 41 of 88
NXP Semiconductors LPC1769/68/67/66/65/64/63
32-bit ARM Cortex-M3 microcontroller
8.29.8 Power domains
The LPC17xx provide two independent power domains that allow the bulk of the device to
have power removed while maintaining operation of the RTC and the backup Registers.
On the LPC17xx, I/O pads are powered by the 3.3 V (VDD(3V3)) pins, while the
VDD(REG)(3V3) pin powers the on-chip voltage regulator which in turn provides power to the
CPU and most of the peripherals.
Depending on the LPC17xx application, a design can use two power options to manage
power consumption.
The first option assumes that power consumption is not a concern and the design ties the
VDD(3V3) and VDD(REG)(3V3) pins together. This approach requires only one 3.3 V power
supply for both pads, the CPU, and peripherals. While this solution is simple, it does not
support powering down the I/O pad ring “on the fly” while keeping the CPU and
peripherals alive.
The second option uses two power supplies; a 3.3 V supply for the I/O pads (VDD(3V3)) and
a dedicated 3.3 V supply for the CPU (VDD(REG)(3V3)). Having the on-chip voltage regulator
powered independently from the I/O pad ring enables shutting down of the I/O pad power
supply “on the fly”, while the CPU and peripherals stay active.
The VBAT pin supplies power only to the RTC domain. The RTC requires a minimum of
power to operate, which can be supplied by an external battery. The device core power
(VDD(REG)(3V3)) is used to operate the RTC whenever VDD(REG)(3V3) is present. Therefore,
there is no power drain from the RTC battery when VDD(REG)(3V3) is available.
LPC1769_68_67_66_65_64_63 All information provided in this document is subject to legal disclaimers. © NXP Semiconductors N.V. 2014. All rights reserved.
Product data sheet Rev. 9.4 — 4 April 2014 42 of 88
NXP Semiconductors LPC1769/68/67/66/65/64/63
32-bit ARM Cortex-M3 microcontroller
8.30 System control
8.30.1 Reset
Reset has four sources on the LPC17xx: the RESET pin, the Watchdog reset, power-on
reset (POR), and the BrownOut Detection (BOD) circuit. The RESET pin is a Schmitt
trigger input pin. Assertion of chip Reset by any source, once the operating voltage attains
a usable level, causes the RSTOUT pin to go LOW and starts the wake-up timer (see
description in Section 8.29.5). The wake-up timer ensures that reset remains asserted
until the external Reset is de-asserted, the oscillator is running, a fixed number of clocks
have passed, and the flash controller has completed its initialization. Once reset is
de-asserted, or, in case of a BOD-triggered reset, once the voltage rises above the BOD
threshold, the RSTOUT pin goes HIGH.
When the internal Reset is removed, the processor begins executing at address 0, which
is initially the Reset vector mapped from the Boot Block. At that point, all of the processor
and peripheral registers have been initialized to predetermined values.
Fig 7. Power distribution
REAL-TIME CLOCK
BACKUP REGISTERS
REGULATOR
32 kHz
OSCILLATOR
RTC POWER DOMAIN
MAIN POWER DOMAIN
002aad978
RTCX1
VBAT
VDD(REG)(3V3)
RTCX2
VDD(3V3)
VSS
to memories,
peripherals,
oscillators,
PLLs
to core
to I/O pads
ADC
DAC
ADC POWER DOMAIN
VDDA
VREFP
VREFN
VSSA
LPC17xx
ULTRA LOW-POWER
REGULATOR
POWER
SELECTOR
LPC1769_68_67_66_65_64_63 All information provided in this document is subject to legal disclaimers. © NXP Semiconductors N.V. 2014. All rights reserved.
Product data sheet Rev. 9.4 — 4 April 2014 43 of 88
NXP Semiconductors LPC1769/68/67/66/65/64/63
32-bit ARM Cortex-M3 microcontroller
8.30.2 Brownout detection
The LPC17xx include 2-stage monitoring of the voltage on the VDD(REG)(3V3) pins. If this
voltage falls below 2.2 V, the BOD asserts an interrupt signal to the Vectored Interrupt
Controller. This signal can be enabled for interrupt in the Interrupt Enable Register in the
NVIC in order to cause a CPU interrupt; if not, software can monitor the signal by reading
a dedicated status register.
The second stage of low-voltage detection asserts reset to inactivate the LPC17xx when
the voltage on the VDD(REG)(3V3) pins falls below 1.85 V. This reset prevents alteration of
the flash as operation of the various elements of the chip would otherwise become
unreliable due to low voltage. The BOD circuit maintains this reset down below 1 V, at
which point the power-on reset circuitry maintains the overall reset.
Both the 2.2 V and 1.85 V thresholds include some hysteresis. In normal operation, this
hysteresis allows the 2.2 V detection to reliably interrupt, or a regularly executed event
loop to sense the condition.
8.30.3 Code security (Code Read Protection - CRP)
This feature of the LPC17xx allows user to enable different levels of security in the system
so that access to the on-chip flash and use of the JTAG and ISP can be restricted. When
needed, CRP is invoked by programming a specific pattern into a dedicated flash location.
IAP commands are not affected by the CRP.
There are three levels of the Code Read Protection.
CRP1 disables access to chip via the JTAG and allows partial flash update (excluding
flash sector 0) using a limited set of the ISP commands. This mode is useful when CRP is
required and flash field updates are needed but all sectors can not be erased.
CRP2 disables access to chip via the JTAG and only allows full flash erase and update
using a reduced set of the ISP commands.
Running an application with level CRP3 selected fully disables any access to chip via the
JTAG pins and the ISP. This mode effectively disables ISP override using P2[10] pin, too.
It is up to the user’s application to provide (if needed) flash update mechanism using IAP
calls or call reinvoke ISP command to enable flash update via UART0.
8.30.4 APB interface
The APB peripherals are split into two separate APB buses in order to distribute the bus
bandwidth and thereby reducing stalls caused by contention between the CPU and the
GPDMA controller.
CAUTION
If level three Code Read Protection (CRP3) is selected, no future factory testing can be
performed on the device.
LPC1769_68_67_66_65_64_63 All information provided in this document is subject to legal disclaimers. © NXP Semiconductors N.V. 2014. All rights reserved.
Product data sheet Rev. 9.4 — 4 April 2014 44 of 88
NXP Semiconductors LPC1769/68/67/66/65/64/63
32-bit ARM Cortex-M3 microcontroller
8.30.5 AHB multilayer matrix
The LPC17xx use an AHB multilayer matrix. This matrix connects the instruction (I-code)
and data (D-code) CPU buses of the ARM Cortex-M3 to the flash memory, the main
(32 kB) static RAM, and the Boot ROM. The GPDMA can also access all of these
memories. The peripheral DMA controllers, Ethernet, and USB can access all SRAM
blocks. Additionally, the matrix connects the CPU system bus and all of the DMA
controllers to the various peripheral functions.
8.30.6 External interrupt inputs
The LPC17xx include up to 46 edge sensitive interrupt inputs combined with up to four
level sensitive external interrupt inputs as selectable pin functions. The external interrupt
inputs can optionally be used to wake up the processor from Power-down mode.
8.30.7 Memory mapping control
The Cortex-M3 incorporates a mechanism that allows remapping the interrupt vector table
to alternate locations in the memory map. This is controlled via the Vector Table Offset
Register contained in the NVIC.
The vector table may be located anywhere within the bottom 1 GB of Cortex-M3 address
space. The vector table must be located on a 128 word (512 byte) boundary because the
NVIC on the LPC17xx is configured for 128 total interrupts.
8.31 Emulation and debugging
Debug and trace functions are integrated into the ARM Cortex-M3. Serial wire debug and
trace functions are supported in addition to a standard JTAG debug and parallel trace
functions. The ARM Cortex-M3 is configured to support up to eight breakpoints and four
watch points.
LPC1769_68_67_66_65_64_63 All information provided in this document is subject to legal disclaimers. © NXP Semiconductors N.V. 2014. All rights reserved.
Product data sheet Rev. 9.4 — 4 April 2014 45 of 88
NXP Semiconductors LPC1769/68/67/66/65/64/63
32-bit ARM Cortex-M3 microcontroller
9. Limiting values
[1] The following applies to the limiting values:
a) This product includes circuitry specifically designed for the protection of its internal devices from the damaging effects of excessive
static charge. Nonetheless, it is suggested that conventional precautions be taken to avoid applying greater than the rated
maximum.
b) Parameters are valid over operating temperature range unless otherwise specified. All voltages are with respect to VSS unless
otherwise noted.
c) The limiting values are stress ratings only. Operating the part at these values is not recommended, and proper operation is not
guaranteed. The conditions for functional operation are specified in Table 8.
[2] Maximum/minimum voltage above the maximum operating voltage (see Table 8) and below ground that can be applied for a short time
(< 10 ms) to a device without leading to irrecoverable failure. Failure includes the loss of reliability and shorter lifetime of the device.
[3] See Table 19 for maximum operating voltage.
[4] Including voltage on outputs in 3-state mode.
[5] VDD present or not present. Compliant with the I2C-bus standard. 5.5 V can be applied to this pin when VDD is powered down.
[6] The maximum non-operating storage temperature is different than the temperature for required shelf life which should be determined
based on required shelf lifetime. Please refer to the JEDEC spec (J-STD-033B.1) for further details.
[7] Human body model: equivalent to discharging a 100 pF capacitor through a 1.5 k series resistor.
Table 6. Limiting values
In accordance with the Absolute Maximum Rating System (IEC 60134).[1]
Symbol Parameter Conditions Min Max Unit
VDD(3V3) supply voltage (3.3 V) external rail [2] 0.5 +4.6 V
VDD(REG)(3V3) regulator supply voltage (3.3 V) [2] 0.5 +4.6 V
VDDA analog 3.3 V pad supply
voltage
[2] 0.5 +4.6 V
Vi(VBAT) input voltage on pin VBAT for the RTC [2] 0.5 +4.6 V
Vi(VREFP) input voltage on pin VREFP [2] 0.5 +4.6 V
VIA analog input voltage on ADC related pins [2][3] 0.5 +5.1 V
VI input voltage 5 V tolerant digital I/O pins;
VDD 2.4 V
[2][4] 0.5 +5.5 VI
VDD = 0 V 0.5 +3.6
5 V tolerant open-drain pins
PIO0_27 and PIO0_28
[2][5] 0.5 +5.5
IDD supply current per supply pin - 100 mA
ISS ground current per ground pin - 100 mA
Ilatch I/O latch-up current (0.5VDD(3V3)) < VI <
(1.5VDD(3V3)); Tj < 125 C
- 100 mA
Tstg storage temperature [6] 65 +150 C
Ptot(pack) total power dissipation (per
package)
based on package heat
transfer, not device power
consumption
- 1.5 W
VESD electrostatic discharge voltage human body model; all pins [7] 4000 +4000 V
LPC1769_68_67_66_65_64_63 All information provided in this document is subject to legal disclaimers. © NXP Semiconductors N.V. 2014. All rights reserved.
Product data sheet Rev. 9.4 — 4 April 2014 46 of 88
NXP Semiconductors LPC1769/68/67/66/65/64/63
32-bit ARM Cortex-M3 microcontroller
10. Thermal characteristics
The average chip junction temperature, Tj (C), can be calculated using the following
equation:
(1)
• Tamb = ambient temperature (C)
• Rth(j-a) = the package junction-to-ambient thermal resistance (C/W)
• PD = sum of internal and I/O power dissipation
The internal power dissipation is the product of IDD and VDD. The I/O power dissipation of
the I/O pins is often small and many times can be negligible. However it can be significant
in some applications.
Table 7. Thermal resistance (15 %)
Symbol Parameter Conditions Max/Min Unit
LQFP100
Rth(j-a) thermal resistance from
junction to ambient
JEDEC (4.5 in 4 in); still air 38.01 C/W
Single-layer (4.5 in 3 in); still air 55.09 C/W
Rth(j-c) thermal resistance from
junction to case
9.065 C/W
TFBGA100
Rth(j-a) thermal resistance from
junction to ambient
JEDEC (4.5 in 4 in); still air 55.2 C/W
Single-layer (4.5 in 3 in); still air 45.6 C/W
Rth(j-c) thermal resistance from
junction to case
9.5 C/W
Tj = Tamb + PD Rthj – a
LPC1769_68_67_66_65_64_63 All information provided in this document is subject to legal disclaimers. © NXP Semiconductors N.V. 2014. All rights reserved.
Product data sheet Rev. 9.4 — 4 April 2014 47 of 88
NXP Semiconductors LPC1769/68/67/66/65/64/63
32-bit ARM Cortex-M3 microcontroller
11. Static characteristics
Table 8. Static characteristics
Tamb = 40 C to +85 C, unless otherwise specified.
Symbol Parameter Conditions Min Typ[1] Max Unit
Supply pins
VDD(3V3) supply voltage (3.3 V) external rail [2] 2.4 3.3 3.6 V
VDD(REG)(3V3) regulator supply voltage
(3.3 V)
2.4 3.3 3.6 V
VDDA analog 3.3 V pad supply
voltage
[3][4] 2.5 3.3 3.6 V
Vi(VBAT) input voltage on pin
VBAT
[5] 2.1 3.3 3.6 V
Vi(VREFP) input voltage on pin
VREFP
[3] 2.5 3.3 VDDA V
IDD(REG)(3V3) regulator supply current
(3.3 V)
active mode; code
while(1){}
executed from flash; all
peripherals disabled;
PCLK = CCLK⁄8
CCLK = 12 MHz; PLL
disabled
[6][7]- 7 - mA
CCLK = 100 MHz; PLL
enabled
[6][7]- 42 - mA
CCLK = 100 MHz; PLL
enabled (LPC1769)
[6][8]- 50 - mA
CCLK = 120 MHz; PLL
enabled (LPC1769)
[6][8]- 67 - mA
sleep mode [6][9]- 2 - mA
deep sleep mode [6][10]- 240 - A
power-down mode [6][10]- 31 - A
deep power-down mode;
RTC running
[11]- 630 - nA
IBAT battery supply current deep power-down mode;
RTC running
VDD(REG)(3V3) present [12]- 530 - nA
VDD(REG)(3V3) not
present
[13] -
1.1 - A
IDD(IO) I/O supply current deep sleep mode [14][15]- 40 - nA
power-down mode [14][15]- 40 - nA
deep power-down mode [14]- 10 - nA
LPC1769_68_67_66_65_64_63 All information provided in this document is subject to legal disclaimers. © NXP Semiconductors N.V. 2014. All rights reserved.
Product data sheet Rev. 9.4 — 4 April 2014 48 of 88
NXP Semiconductors LPC1769/68/67/66/65/64/63
32-bit ARM Cortex-M3 microcontroller
IDD(ADC) ADC supply current active mode;
ADC powered
[16][17]- 1.95 - mA
ADC in Power-down
mode
[16][18]- <0.2 - A
deep sleep mode [16]- 38 - nA
power-down mode [16]- 38 - nA
deep power-down mode [16]- 24 - nA
II(ADC) ADC input current on pin VREFP
deep sleep mode [19]- 100 - nA
power-down mode [19]- 100 - nA
deep power-down
mode
[19]- 100 - nA
Standard port pins, RESET, RTCK
IIL LOW-level input current VI = 0 V; on-chip pull-up
resistor disabled
- 0.5 10 nA
IIH HIGH-level input
current
VI = VDD(3V3); on-chip
pull-down resistor
disabled
- 0.5 10 nA
IOZ OFF-state output
current
VO = 0 V; VO = VDD(3V3);
on-chip pull-up/down
resistors disabled
- 0.5 10 nA
VI input voltage pin configured to provide
a digital function
[20][21]
[22]
0 - 5.0 V
VO output voltage output active 0 - VDD(3V3) V
VIH HIGH-level input
voltage
0.7VDD(3V3)- - V
VIL LOW-level input voltage - - 0.3VDD(3V3) V
Vhys hysteresis voltage 0.4 - - V
VOH HIGH-level output
voltage
IOH = 4 mA VDD(3V3)
0.4
- - V
VOL LOW-level output
voltage
IOL = 4 mA - - 0.4 V
IOH HIGH-level output
current
VOH = VDD(3V3) 0.4 V 4 - - mA
IOL LOW-level output
current
VOL = 0.4 V 4 - - mA
IOHS HIGH-level short-circuit
output current
VOH = 0 V [23]- - 45 mA
IOLS LOW-level short-circuit
output current
VOL = VDD(3V3) [23]- - 50 mA
Ipd pull-down current VI = 5 V 10 50 150 A
Ipu pull-up current VI = 0 V 15 50 85 A
VDD(3V3) < VI < 5 V 0 0 0 A
Table 8. Static characteristics …continued
Tamb = 40 C to +85 C, unless otherwise specified.
Symbol Parameter Conditions Min Typ[1] Max Unit
LPC1769_68_67_66_65_64_63 All information provided in this document is subject to legal disclaimers. © NXP Semiconductors N.V. 2014. All rights reserved.
Product data sheet Rev. 9.4 — 4 April 2014 49 of 88
NXP Semiconductors LPC1769/68/67/66/65/64/63
32-bit ARM Cortex-M3 microcontroller
[1] Typical ratings are not guaranteed. The values listed are at room temperature (25 C), nominal supply voltages.
[2] For USB operation 3.0 V VDD((3V3) 3.6 V. Guaranteed by design.
[3] VDDA and VREFP should be tied to VDD(3V3) if the ADC and DAC are not used.
[4] VDDA for DAC specs are from 2.7 V to 3.6 V.
I2C-bus pins (P0[27] and P0[28])
VIH HIGH-level input
voltage
0.7VDD(3V3)- - V
VIL LOW-level input voltage - - 0.3VDD(3V3) V
Vhys hysteresis voltage - 0.05
VDD(3V3)
- V
VOL LOW-level output
voltage
IOLS = 3 mA - - 0.4 V
ILI input leakage current VI = VDD(3V3) [24]- 2 4 A
VI = 5 V - 10 22 A
Oscillator pins
Vi(XTAL1) input voltage on pin
XTAL1
0.5 1.8 1.95 V
Vo(XTAL2) output voltage on pin
XTAL2
0.5 1.8 1.95 V
Vi(RTCX1) input voltage on pin
RTCX1
0.5 - 3.6 V
Vo(RTCX2) output voltage on pin
RTCX2
0.5 - 3.6 V
USB pins (LPC1769/68/66/65/64 only)
IOZ OFF-state output
current
0 V < VI < 3.3 V [2]- - 10 A
VBUS bus supply voltage [2]- - 5.25 V
VDI differential input
sensitivity voltage
(D+) (D) [2] 0.2 - - V
VCM differential common
mode voltage range
includes VDI range [2] 0.8 - 2.5 V
Vth(rs)se single-ended receiver
switching threshold
voltage
[2] 0.8 - 2.0 V
VOL LOW-level output
voltage for
low-/full-speed
RL of 1.5 k to 3.6 V [2]- - 0.18 V
VOH HIGH-level output
voltage (driven) for
low-/full-speed
RL of 15 k to GND [2] 2.8 - 3.5 V
Ctrans transceiver capacitance pin to GND [2]- - 20 pF
ZDRV driver output
impedance for driver
which is not high-speed
capable
with 33 series resistor;
steady state drive
[2][25] 36 - 44.1
Table 8. Static characteristics …continued
Tamb = 40 C to +85 C, unless otherwise specified.
Symbol Parameter Conditions Min Typ[1] Max Unit
LPC1769_68_67_66_65_64_63 All information provided in this document is subject to legal disclaimers. © NXP Semiconductors N.V. 2014. All rights reserved.
Product data sheet Rev. 9.4 — 4 April 2014 50 of 88
NXP Semiconductors LPC1769/68/67/66/65/64/63
32-bit ARM Cortex-M3 microcontroller
[5] The RTC typically fails when Vi(VBAT) drops below 1.6 V.
[6] VDD(REG)(3V3) = 3.3 V; Tamb = 25 C for all power consumption measurements.
[7] Applies to LPC1768/67/66/65/64/63.
[8] Applies to LPC1769 only.
[9] IRC running at 4 MHz; main oscillator and PLL disabled; PCLK = CCLK⁄8.
[10] BOD disabled.
[11] On pin VDD(REG)(3V3). IBAT = 530 nA. VDD(REG)(3V3) = 3.0 V; VBAT = 3.0 V; Tamb = 25 C.
[12] On pin VBAT; IDD(REG)(3V3) = 630 nA; VDD(REG)(3V3) = 3.0 V; VBAT = 3.0 V; Tamb = 25 C.
[13] On pin VBAT; VBAT = 3.0 V; Tamb = 25 C.
[14] All internal pull-ups disabled. All pins configured as output and driven LOW. VDD(3V3) = 3.3 V; Tamb = 25 C.
[15] TCK/SWDCLK pin needs to be externally pulled LOW.
[16] On pin VDDA; VDDA = 3.3 V; Tamb = 25 C. The ADC is powered if the PDN bit in the AD0CR register is set to 1 and in Power-down mode
of the PDN bit is set to 0.
[17] The ADC is powered if the PDN bit in the AD0CR register is set to 1. See LPC17xx user manual UM10360_1.
[18] The ADC is in Power-down mode if the PDN bit in the AD0CR register is set to 0. See LPC17xx user manual UM10360_1.
[19] Vi(VREFP) = 3.3 V; Tamb = 25 C.
[20] Including voltage on outputs in 3-state mode.
[21] VDD(3V3) supply voltages must be present.
[22] 3-state outputs go into 3-state mode in Deep power-down mode.
[23] Allowed as long as the current limit does not exceed the maximum current allowed by the device.
[24] To VSS.
[25] Includes external resistors of 33 1 % on D+ and D.
11.1 Power consumption
Conditions: BOD disabled.
Fig 8. Deep-sleep mode: typical regulator supply current IDD(Reg)(3V3) versus
temperature
002aaf568
temperature (°C)
−40 −15 10 35 60 85
250
350
300
400
IDD(Reg)(3V3)
(μA)
200
3.6 V
3.3 V
2.4 V
LPC1769_68_67_66_65_64_63 All information provided in this document is subject to legal disclaimers. © NXP Semiconductors N.V. 2014. All rights reserved.
Product data sheet Rev. 9.4 — 4 April 2014 51 of 88
NXP Semiconductors LPC1769/68/67/66/65/64/63
32-bit ARM Cortex-M3 microcontroller
Conditions: BOD disabled.
Fig 9. Power-down mode: Typical regulator supply current IDD(Reg)(3V3) versus
temperature
Conditions: VDD(REG)(3V3) floating; RTC running.
Fig 10. Deep power-down mode: Typical battery supply current IBAT versus temperature
002aaf569
40
80
120
0
temperature (°C)
−40 −15 10 35 60 85
IDD(Reg)(3V3)
(μA)
3.6 V
3.3 V
2.4 V
002aag119
1.0
1.4
1.8
0.6
temperature (°C)
-40 -15 10 35 60 85
IBAT)
(μA)
Vi(VBAT) = 3.6 V
3.3 V
3.0 V
2.4 V
LPC1769_68_67_66_65_64_63 All information provided in this document is subject to legal disclaimers. © NXP Semiconductors N.V. 2014. All rights reserved.
Product data sheet Rev. 9.4 — 4 April 2014 52 of 88
NXP Semiconductors LPC1769/68/67/66/65/64/63
32-bit ARM Cortex-M3 microcontroller
Conditions: VBAT = 3.0 V; VDD(REG)(3V3) = 3.0 V; RTC running.
Fig 11. Deep power-down mode: Typical regulator supply current IDD(REG)(3V3) and battery
supply current IBAT versus temperature
002aag120
temperature (°C)
-40 -15 10 35 60 85
0.8
1.6
0.4
1.2
2.0
0
IDD(REG)(3V3)
IBAT
IDD(REG)(3V3)/IBAT
(μA)
LPC1769_68_67_66_65_64_63 All information provided in this document is subject to legal disclaimers. © NXP Semiconductors N.V. 2014. All rights reserved.
Product data sheet Rev. 9.4 — 4 April 2014 53 of 88
NXP Semiconductors LPC1769/68/67/66/65/64/63
32-bit ARM Cortex-M3 microcontroller
11.2 Peripheral power consumption
The supply current per peripheral is measured as the difference in supply current between
the peripheral block enabled and the peripheral block disabled in the PCONP register. All
other blocks are disabled and no code is executed. Measured on a typical sample at
Tamb = 25 C. The peripheral clock PCLK = CCLK/4.
[1] The combined current of several peripherals running at the same time can be less than the sum of each individual peripheral current
measured separately.
Table 9. Power consumption for individual analog and digital blocks
Peripheral Conditions Typical supply current in mA;
CCLK =
Notes
12 MHz 48 MHz 100 MHz
Timer 0.03 0.11 0.23 Average current per timer
UART 0.07 0.26 0.53 Average current per UART
PWM 0.05 0.20 0.41
Motor control
PWM
0.05 0.21 0.42
I2C 0.02 0.08 0.16 Average current per I2C
SPI 0.02 0.06 0.13
SSP1 0.04 0.16 0.32
ADC PCLK = 12 MHz for CCLK = 12 MHz
and 48 MHz; PCLK = 12.5 MHz for
CCLK = 100 MHz
2.12 2.09 2.07
CAN PCLK = CCLK/6 0.13 0.49 1.00 Average current per CAN
CAN0, CAN1,
acceptance filter
PCLK = CCLK/6 0.22 0.85 1.73 Both CAN blocks and
acceptance filter[1]
DMA PCLK = CCLK 1.33 5.10 10.36
QEI 0.05 0.20 0.41
GPIO 0.33 1.27 2.58
I2S 0.09 0.34 0.70
USB and PLL1 0.94 1.32 1.94
Ethernet Ethernet block enabled in the PCONP
register; Ethernet not connected.
0.49 1.87 3.79
Ethernet
connected
Ethernet initialized, connected to
network, and running web server
example.
- - 5.19
LPC1769_68_67_66_65_64_63 All information provided in this document is subject to legal disclaimers. © NXP Semiconductors N.V. 2014. All rights reserved.
Product data sheet Rev. 9.4 — 4 April 2014 54 of 88
NXP Semiconductors LPC1769/68/67/66/65/64/63
32-bit ARM Cortex-M3 microcontroller
11.3 Electrical pin characteristics
Conditions: VDD(REG)(3V3) = VDD(3V3) = 3.3 V; standard port pins.
Fig 12. Typical HIGH-level output voltage VOH versus HIGH-level output source current
IOH
Conditions: VDD(REG)(3V3) = VDD(3V3) = 3.3 V; standard port pins.
Fig 13. Typical LOW-level output current IOL versus LOW-level output voltage VOL
IOH (mA)
0 8 16 24
002aaf112
2.8
2.4
3.2
3.6
VOH
(V)
2.0
T = 85 °C
25 °C
−40 °C
VOL (V)
0 0.2 0.4 0.6
002aaf111
5
10
15
IOL
(mA)
0
T = 85 °C
25 °C
−40 °C
LPC1769_68_67_66_65_64_63 All information provided in this document is subject to legal disclaimers. © NXP Semiconductors N.V. 2014. All rights reserved.
Product data sheet Rev. 9.4 — 4 April 2014 55 of 88
NXP Semiconductors LPC1769/68/67/66/65/64/63
32-bit ARM Cortex-M3 microcontroller
Conditions: VDD(REG)(3V3) = VDD(3V3) = 3.3 V; standard port pins.
Fig 14. Typical pull-up current Ipu versus input voltage VI
Conditions: VDD(REG)(3V3) = VDD(3V3) = 3.3 V; standard port pins.
Fig 15. Typical pull-down current Ipd versus input voltage VI
0 1 2 3 4 5
002aaf108
−30
−50
−10
10
Ipu
(μA)
−70
T = 85 °C
25 °C
−40 °C
VI (V)
002aaf109
VI (V)
0 1 2 3 4 5
10
70
50
30
90
Ipd
(μA)
−10
T = 85 °C
25 °C
−40 °C
LPC1769_68_67_66_65_64_63 All information provided in this document is subject to legal disclaimers. © NXP Semiconductors N.V. 2014. All rights reserved.
Product data sheet Rev. 9.4 — 4 April 2014 56 of 88
NXP Semiconductors LPC1769/68/67/66/65/64/63
32-bit ARM Cortex-M3 microcontroller
12. Dynamic characteristics
12.1 Flash memory
[1] Number of program/erase cycles.
[2] Programming times are given for writing 256 bytes from RAM to the flash. Data must be written to the flash in blocks of 256 bytes.
12.2 External clock
[1] Parameters are valid over operating temperature range unless otherwise specified.
[2] Typical ratings are not guaranteed. The values listed are at room temperature (25 C), nominal supply voltages.
Table 10. Flash characteristics
Tamb = 40 C to +85 C, unless otherwise specified.
Symbol Parameter Conditions Min Typ Max Unit
Nendu endurance [1] 10000 100000 - cycles
tret retention time powered 10 - - years
unpowered 20 - - years
ter erase time sector or multiple
consecutive sectors
95 100 105 ms
tprog programming time [2] 0.95 1 1.05 ms
Table 11. Dynamic characteristic: external clock
Tamb = 40 C to +85 C; VDD(3V3) over specified ranges.[1]
Symbol Parameter Conditions Min Typ[2] Max Unit
fosc oscillator frequency 1 - 25 MHz
Tcy(clk) clock cycle time 40 - 1000 ns
tCHCX clock HIGH time Tcy(clk) 0.4 - - ns
tCLCX clock LOW time Tcy(clk) 0.4 - - ns
tCLCH clock rise time - - 5 ns
tCHCL clock fall time - - 5 ns
Fig 16. External clock timing (with an amplitude of at least Vi(RMS) = 200 mV)
tCHCL tCLCX
tCHCX
Tcy(clk)
tCLCH
002aaa907
LPC1769_68_67_66_65_64_63 All information provided in this document is subject to legal disclaimers. © NXP Semiconductors N.V. 2014. All rights reserved.
Product data sheet Rev. 9.4 — 4 April 2014 57 of 88
NXP Semiconductors LPC1769/68/67/66/65/64/63
32-bit ARM Cortex-M3 microcontroller
12.3 Internal oscillators
[1] Parameters are valid over operating temperature range unless otherwise specified.
[2] Typical ratings are not guaranteed. The values listed are at room temperature (25 C), nominal supply voltages.
12.4 I/O pins
[1] Applies to standard I/O pins.
Table 12. Dynamic characteristic: internal oscillators
Tamb = 40 C to +85 C; 2.7 V VDD(REG)(3V3) 3.6 V.[1]
Symbol Parameter Conditions Min Typ[2] Max Unit
fosc(RC) internal RC oscillator frequency - 3.96 4.02 4.04 MHz
fi(RTC) RTC input frequency - - 32.768 - kHz
Conditions: Frequency values are typical values. 4 MHz 1 % accuracy is guaranteed for
2.7 V VDD(REG)(3V3) 3.6 V and Tamb = 40 C to +85 C. Variations between parts may cause
the IRC to fall outside the 4 MHz 1 % accuracy specification for voltages below 2.7 V.
Fig 17. Internal RC oscillator frequency versus temperature
002aaf107
temperature (°C)
-40 -15 10 35 60 85
4.024
4.032
4.020
4.028
4.036
fosc(RC)
(MHz)
4.016
VDD(REG)(3V3) = 3.6 V
3.3 V
3.0 V
2.7 V
2.4 V
Table 13. Dynamic characteristic: I/O pins[1]
Tamb = 40 C to +85 C; VDD(3V3) over specified ranges.
Symbol Parameter Conditions Min Typ Max Unit
tr rise time pin configured as output 3.0 - 5.0 ns
tf fall time pin configured as output 2.5 - 5.0 ns
LPC1769_68_67_66_65_64_63 All information provided in this document is subject to legal disclaimers. © NXP Semiconductors N.V. 2014. All rights reserved.
Product data sheet Rev. 9.4 — 4 April 2014 58 of 88
NXP Semiconductors LPC1769/68/67/66/65/64/63
32-bit ARM Cortex-M3 microcontroller
12.5 I2C-bus
[1] See the I2C-bus specification UM10204 for details.
[2] Parameters are valid over operating temperature range unless otherwise specified.
[3] A device must internally provide a hold time of at least 300 ns for the SDA signal (with respect to the
VIH(min) of the SCL signal) to bridge the undefined region of the falling edge of SCL.
[4] Cb = total capacitance of one bus line in pF.
[5] The maximum tf for the SDA and SCL bus lines is specified at 300 ns. The maximum fall time for the SDA
output stage tf is specified at 250 ns. This allows series protection resistors to be connected in between the
SDA and the SCL pins and the SDA/SCL bus lines without exceeding the maximum specified tf.
[6] In Fast-mode Plus, fall time is specified the same for both output stage and bus timing. If series resistors
are used, designers should allow for this when considering bus timing.
[7] tHD;DAT is the data hold time that is measured from the falling edge of SCL; applies to data in transmission
and the acknowledge.
[8] The maximum tHD;DAT could be 3.45 s and 0.9 s for Standard-mode and Fast-mode but must be less than
the maximum of tVD;DAT or tVD;ACK by a transition time (see the I2C-bus specification UM10204). This
maximum must only be met if the device does not stretch the LOW period (tLOW) of the SCL signal. If the
clock stretches the SCL, the data must be valid by the set-up time before it releases the clock.
[9] tSU;DAT is the data set-up time that is measured with respect to the rising edge of SCL; applies to data in
transmission and the acknowledge.
[10] A Fast-mode I2C-bus device can be used in a Standard-mode I2C-bus system but the requirement tSU;DAT =
250 ns must then be met. This will automatically be the case if the device does not stretch the LOW period
of the SCL signal. If such a device does stretch the LOW period of the SCL signal, it must output the next
data bit to the SDA line tr(max) + tSU;DAT = 1000 + 250 = 1250 ns (according to the Standard-mode I2C-bus
specification) before the SCL line is released. Also the acknowledge timing must meet this set-up time.
Table 14. Dynamic characteristic: I2C-bus pins[1]
Tamb = 40 C to +85 C.[2]
Symbol Parameter Conditions Min Max Unit
fSCL SCL clock
frequency
Standard-mode 0 100 kHz
Fast-mode 0 400 kHz
Fast-mode Plus 0 1 MHz
tf fall time [3][4][5][6] of both SDA and
SCL signals
Standard-mode
- 300 ns
Fast-mode 20 + 0.1 Cb 300 ns
Fast-mode Plus - 120 ns
tLOW LOW period of
the SCL clock
Standard-mode 4.7 - s
Fast-mode 1.3 - s
Fast-mode Plus 0.5 - s
tHIGH HIGH period of
the SCL clock
Standard-mode 4.0 - s
Fast-mode 0.6 - s
Fast-mode Plus 0.26 - s
tHD;DAT data hold time [3][7][8] Standard-mode 0 - s
Fast-mode 0 - s
Fast-mode Plus 0 - s
tSU;DAT data set-up
time
[9][10] Standard-mode 250 - ns
Fast-mode 100 - ns
Fast-mode Plus 50 - ns
LPC1769_68_67_66_65_64_63 All information provided in this document is subject to legal disclaimers. © NXP Semiconductors N.V. 2014. All rights reserved.
Product data sheet Rev. 9.4 — 4 April 2014 59 of 88
NXP Semiconductors LPC1769/68/67/66/65/64/63
32-bit ARM Cortex-M3 microcontroller
12.6 I2S-bus interface
Remark: The I2S-bus interface is available on parts LPC1769/68/67/66/65/63. See
Table 2.
[1] CCLK = 20 MHz; peripheral clock to the I2S-bus interface PCLK = CCLK⁄4; I2S clock cycle time Tcy(clk) = 1600 ns, corresponds to the SCK
signal in the I2S-bus specification.
Fig 18. I2C-bus pins clock timing
002aaf425
tf
70 %
SDA 30 %
tf
70 %
30 %
S
70 %
30 %
70 %
30 %
tHD;DAT
SCL
1 / fSCL
70 %
30 %
70 %
30 %
tVD;DAT
tHIGH
tLOW
tSU;DAT
Table 15. Dynamic characteristics: I2S-bus interface pins
Tamb = 40 C to +85 C.
Symbol Parameter Conditions Min Typ Max Unit
common to input and output
tr rise time [1] - - 35 ns
tf fall time [1] - - 35 ns
tWH pulse width HIGH on pins I2STX_CLK and
I2SRX_CLK
[1] 0.495 Tcy(clk) - - -
tWL pulse width LOW on pins I2STX_CLK and
I2SRX_CLK
[1] - - 0.505 Tcy(clk) ns
output
tv(Q) data output valid time on pin I2STX_SDA [1] - - 30 ns
on pin I2STX_WS [1] - - 30 ns
input
tsu(D) data input set-up time on pin I2SRX_SDA [1] 3.5 - - ns
th(D) data input hold time on pin I2SRX_SDA [1] 4.0 - - ns
LPC1769_68_67_66_65_64_63 All information provided in this document is subject to legal disclaimers. © NXP Semiconductors N.V. 2014. All rights reserved.
Product data sheet Rev. 9.4 — 4 April 2014 60 of 88
NXP Semiconductors LPC1769/68/67/66/65/64/63
32-bit ARM Cortex-M3 microcontroller
Fig 19. I2S-bus timing (output)
Fig 20. I2S-bus timing (input)
002aad992
I2STX_CLK
I2STX_SDA
I2STX_WS
Tcy(clk) tf tr
tWH tWL
tv(Q)
tv(Q)
002aae159
Tcy(clk) tf tr
tWH
tsu(D) th(D)
tsu(D) tsu(D)
tWL
I2SRX_CLK
I2SRX_SDA
I2SRX_WS
LPC1769_68_67_66_65_64_63 All information provided in this document is subject to legal disclaimers. © NXP Semiconductors N.V. 2014. All rights reserved.
Product data sheet Rev. 9.4 — 4 April 2014 61 of 88
NXP Semiconductors LPC1769/68/67/66/65/64/63
32-bit ARM Cortex-M3 microcontroller
12.7 SSP interface
[1] The peripheral clock for SSP is PCLK = CCLK = 20 MHz.
Table 16. Dynamic characteristic: SSP interface
Tamb = 25C; VDD(3V3) over specified ranges.
Symbol Parameter Conditions Min Typ Max Unit
SSP interface
tsu(SPI_MISO) SPI_MISO set-up time measured in SPI Master mode;
see Figure 21
[1] 30 - ns
Fig 21. MISO line set-up time in SSP Master mode
tsu(SPI_MISO)
SCK
shifting edges
MOSI
MISO
002aad326
sampling edges
LPC1769_68_67_66_65_64_63 All information provided in this document is subject to legal disclaimers. © NXP Semiconductors N.V. 2014. All rights reserved.
Product data sheet Rev. 9.4 — 4 April 2014 62 of 88
NXP Semiconductors LPC1769/68/67/66/65/64/63
32-bit ARM Cortex-M3 microcontroller
12.8 USB interface
Remark: The USB controller is available as a device/Host/OTG controller on parts
LPC1769/68/66/65 and as device-only controller on part LPC1764.
[1] Characterized but not implemented as production test. Guaranteed by design.
Table 17. Dynamic characteristics: USB pins (full-speed)
CL = 50 pF; Rpu = 1.5 k on D+ to VDD(3V3); 3.0 V VDD(3V3) 3.6 V.
Symbol Parameter Conditions Min Typ Max Unit
tr rise time 10 % to 90 % 8.5 - 13.8 ns
tf fall time 10 % to 90 % 7.7 - 13.7 ns
tFRFM differential rise and fall time
matching
tr / tf - - 109 %
VCRS output signal crossover voltage 1.3 - 2.0 V
tFEOPT source SE0 interval of EOP see Figure 22 160 - 175 ns
tFDEOP source jitter for differential transition
to SE0 transition
see Figure 22 2 - +5 ns
tJR1 receiver jitter to next transition 18.5 - +18.5 ns
tJR2 receiver jitter for paired transitions 10 % to 90 % 9 - +9 ns
tEOPR1 EOP width at receiver must reject as
EOP; see
Figure 22
[1] 40 - - ns
tEOPR2 EOP width at receiver must accept as
EOP; see
Figure 22
[1] 82 - - ns
Fig 22. Differential data-to-EOP transition skew and EOP width
002aab561
TPERIOD
differential
data lines
crossover point
source EOP width: tFEOPT
receiver EOP width: tEOPR1, tEOPR2
crossover point
extended
differential data to
SE0/EOP skew
n × TPERIOD + tFDEOP
LPC1769_68_67_66_65_64_63 All information provided in this document is subject to legal disclaimers. © NXP Semiconductors N.V. 2014. All rights reserved.
Product data sheet Rev. 9.4 — 4 April 2014 63 of 88
NXP Semiconductors LPC1769/68/67/66/65/64/63
32-bit ARM Cortex-M3 microcontroller
12.9 SPI
[1] TSPICYC = (Tcy(PCLK) n) 0.5 %, n is the SPI clock divider value (n 8); PCLK is derived from the
processor clock CCLK.
[2] Timing parameters are measured with respect to the 50 % edge of the clock SCK and the 10 % (90 %)
edge of the data signal (MOSI or MISO).
Table 18. Dynamic characteristics of SPI pins
Tamb = 40 C to +85 C.
Symbol Parameter Min Typ Max Unit
Tcy(PCLK) PCLK cycle time 10 - - ns
TSPICYC SPI cycle time [1] 79.6 - - ns
tSPICLKH SPICLK HIGH time 0.485 TSPICYC - - ns
tSPICLKL SPICLK LOW time - 0.515 TSPICYC ns
SPI master
tSPIDSU SPI data set-up time [2] 0 - - ns
tSPIDH SPI data hold time [2] 2 Tcy(PCLK) 5 - - ns
tSPIQV SPI data output valid time [2] 2 Tcy(PCLK) + 30 - - ns
tSPIOH SPI output data hold time [2] 2 Tcy(PCLK) + 5 - - ns
SPI slave
tSPIDSU SPI data set-up time [2] 0 - - ns
tSPIDH SPI data hold time [2] 2 Tcy(PCLK) + 5 - - ns
tSPIQV SPI data output valid time [2] 2 Tcy(PCLK) + 35 - - ns
tSPIOH SPI output data hold time [2] 2 Tcy(PCLK) + 15 - - ns
Fig 23. SPI master timing (CPHA = 1)
SCK (CPOL = 0)
MOSI
MISO
002aad986
TSPICYC tSPICLKH tSPICLKL
tSPIDSU tSPIDH
tSPIQV
DATA VALID DATA VALID
tSPIOH
SCK (CPOL = 1)
DATA VALID DATA VALID
LPC1769_68_67_66_65_64_63 All information provided in this document is subject to legal disclaimers. © NXP Semiconductors N.V. 2014. All rights reserved.
Product data sheet Rev. 9.4 — 4 April 2014 64 of 88
NXP Semiconductors LPC1769/68/67/66/65/64/63
32-bit ARM Cortex-M3 microcontroller
Fig 24. SPI master timing (CPHA = 0)
Fig 25. SPI slave timing (CPHA = 1)
SCK (CPOL = 0)
MOSI
MISO
002aad987
TSPICYC tSPICLKH tSPICLKL
tSPIDSU tSPIDH
DATA VALID DATA VALID
tSPIOH
SCK (CPOL = 1)
DATA VALID DATA VALID
tSPIQV
SCK (CPOL = 0)
MOSI
MISO
002aad988
TSPICYC tSPICLKH tSPICLKL
tSPIDSU tSPIDH
tSPIQV
DATA VALID DATA VALID
tSPIOH
SCK (CPOL = 1)
DATA VALID DATA VALID
LPC1769_68_67_66_65_64_63 All information provided in this document is subject to legal disclaimers. © NXP Semiconductors N.V. 2014. All rights reserved.
Product data sheet Rev. 9.4 — 4 April 2014 65 of 88
NXP Semiconductors LPC1769/68/67/66/65/64/63
32-bit ARM Cortex-M3 microcontroller
13. ADC electrical characteristics
[1] VDDA and VREFP should be tied to VDD(3V3) if the ADC and DAC are not used.
[2] The ADC is monotonic, there are no missing codes.
[3] The differential linearity error (ED) is the difference between the actual step width and the ideal step width. See Figure 27.
[4] The integral non-linearity (EL(adj)) is the peak difference between the center of the steps of the actual and the ideal transfer curve after
appropriate adjustment of gain and offset errors. See Figure 27.
[5] The offset error (EO) is the absolute difference between the straight line which fits the actual curve and the straight line which fits the
ideal curve. See Figure 27.
[6] ADCOFFS value (bits 7:4) = 2 in the ADTRM register. See LPC17xx user manual UM10360.
[7] The gain error (EG) is the relative difference in percent between the straight line fitting the actual transfer curve after removing offset
error, and the straight line which fits the ideal transfer curve. See Figure 27.
[8] The absolute error (ET) is the maximum difference between the center of the steps of the actual transfer curve of the non-calibrated
ADC and the ideal transfer curve. See Figure 27.
[9] See Figure 28.
[10] The conversion frequency corresponds to the number of samples per second.
Fig 26. SPI slave timing (CPHA = 0)
SCK (CPOL = 0)
MOSI
MISO
002aad989
TSPICYC tSPICLKH tSPICLKL
tSPIDSU tSPIDH
tSPIQV
DATA VALID DATA VALID
tSPIOH
SCK (CPOL = 1)
DATA VALID DATA VALID
Table 19. ADC characteristics (full resolution)
VDDA = 2.5 V to 3.6 V; Tamb = 40 C to +85 C unless otherwise specified; ADC frequency 13 MHz; 12-bit resolution.[1]
Symbol Parameter Conditions Min Typ Max Unit
VIA analog input voltage 0 - VDDA V
Cia analog input capacitance - - 15 pF
ED differential linearity error [2][3]- - 1 LSB
EL(adj) integral non-linearity [4]- - 3 LSB
EO offset error [5][6]- - 2 LSB
EG gain error [7]- - 0.5 %
ET absolute error [8]- - 4 LSB
Rvsi voltage source interface
resistance
[9]- - 7.5 k
fclk(ADC) ADC clock frequency - - 13 MHz
fc(ADC) ADC conversion frequency [10]- - 200 kHz
LPC1769_68_67_66_65_64_63 All information provided in this document is subject to legal disclaimers. © NXP Semiconductors N.V. 2014. All rights reserved.
Product data sheet Rev. 9.4 — 4 April 2014 66 of 88
NXP Semiconductors LPC1769/68/67/66/65/64/63
32-bit ARM Cortex-M3 microcontroller
[1] VDDA and VREFP should be tied to VDD(3V3) if the ADC and DAC are not used.
[2] The ADC is monotonic, there are no missing codes.
[3] The differential linearity error (ED) is the difference between the actual step width and the ideal step width. See Figure 27.
[4] The integral non-linearity (EL(adj)) is the peak difference between the center of the steps of the actual and the ideal transfer curve after
appropriate adjustment of gain and offset errors. See Figure 27.
[5] The offset error (EO) is the absolute difference between the straight line which fits the actual curve and the straight line which fits the
ideal curve. See Figure 27.
[6] The gain error (EG) is the relative difference in percent between the straight line fitting the actual transfer curve after removing offset
error, and the straight line which fits the ideal transfer curve. See Figure 27.
[7] The conversion frequency corresponds to the number of samples per second.
Table 20. ADC characteristics (lower resolution)
Tamb = 40 C to +85 C unless otherwise specified; 12-bit ADC used as 10-bit resolution ADC.[1]
Symbol Parameter Conditions Min Typ Max Unit
ED differential linearity error [2][3] - 1 - LSB
EL(adj) integral non-linearity [4] - 1.5 - LSB
EO offset error [5] - 2 - LSB
EG gain error [6] - 2 - LSB
fclk(ADC) ADC clock frequency 3.0 V VDDA 3.6 V - - 33 MHz
2.7 V VDDA < 3.0 V - - 25 MHz
fc(ADC) ADC conversion frequency 3 V VDDA 3.6 V [7]- - 500 kHz
2.7 V VDDA < 3.0 V [7]- - 400 kHz
LPC1769_68_67_66_65_64_63 All information provided in this document is subject to legal disclaimers. © NXP Semiconductors N.V. 2014. All rights reserved.
Product data sheet Rev. 9.4 — 4 April 2014 67 of 88
NXP Semiconductors LPC1769/68/67/66/65/64/63
32-bit ARM Cortex-M3 microcontroller
(1) Example of an actual transfer curve.
(2) The ideal transfer curve.
(3) Differential linearity error (ED).
(4) Integral non-linearity (EL(adj)).
(5) Center of a step of the actual transfer curve.
Fig 27. 12-bit ADC characteristics
002aad948
4095
4094
4093
4092
4091
(2)
(1)
1 2 3 4 5 6 7 4090 4091 4092 4093 4094 4095 4096
7
6
5
4
3
2
1
0
4090
(5)
(4)
(3)
1 LSB
(ideal)
code
out
VREFP − VREFN
4096
offset
error
EO
gain
error
EG
offset error
EO
VIA (LSBideal)
1 LSB =
LPC1769_68_67_66_65_64_63 All information provided in this document is subject to legal disclaimers. © NXP Semiconductors N.V. 2014. All rights reserved.
Product data sheet Rev. 9.4 — 4 April 2014 68 of 88
NXP Semiconductors LPC1769/68/67/66/65/64/63
32-bit ARM Cortex-M3 microcontroller
14. DAC electrical characteristics
Remark: The DAC is available on parts LPC1769/68/67/66/65/63. See Table 2.
The values of resistor components Ri1 and Ri2 vary with temperature and input voltage and are
process-dependent (see Table 21).
Parasitic resistance and capacitance from the pad are not included in this figure.
Fig 28. ADC interface to pins AD0[n]
Table 21. ADC interface components
Component Range Description
Ri1 2 k to 5.2 k Switch-on resistance for channel selection switch. Varies with
temperature, input voltage, and process.
Ri2 100 to 600 Switch-on resistance for the comparator input switch. Varies
with temperature, input voltage, and process.
C1 750 fF Parasitic capacitance from the ADC block level.
C2 65 fF Parasitic capacitance from the ADC block level.
C3 2.2 pF Sampling capacitor.
LPC17xx
AD0[n]
750 fF 65 fF Cia
2.2 pF
Rvsi
Ri2
100 Ω - 600 Ω
Ri1
2 kΩ - 5.2 kΩ
VSS VEXT
002aaf197
ADC
COMPARATOR
BLOCK C1
C3
C2
Table 22. DAC electrical characteristics
VDDA = 2.7 V to 3.6 V; Tamb = 40 C to +85 C unless otherwise specified
Symbol Parameter Conditions Min Typ Max Unit
ED differential linearity error - 1 - LSB
EL(adj) integral non-linearity - 1.5 - LSB
EO offset error - 0.6 - %
EG gain error - 0.6 - %
CL load capacitance - 200 - pF
RL load resistance 1 - - k
LPC1769_68_67_66_65_64_63 All information provided in this document is subject to legal disclaimers. © NXP Semiconductors N.V. 2014. All rights reserved.
Product data sheet Rev. 9.4 — 4 April 2014 69 of 88
NXP Semiconductors LPC1769/68/67/66/65/64/63
32-bit ARM Cortex-M3 microcontroller
15. Application information
15.1 Suggested USB interface solutions
Remark: The USB controller is available as a device/Host/OTG controller on parts
LPC1769/68/66/65 and as device-only controller on part LPC1764.
If the LPC1769/68/67/66/65/64/63 VDD is always greater than 0 V while VBUS = 5 V, the
VBUS pin can be connected directly to the VBUS pin on the USB connector.
This applies to bus powered devices where the USB cable supplies the system power. For
systems where VDD can be 0 V and VBUS is directly applied to the VBUS pin, precautions
must be taken to reduce the voltage to below 3.6 V.
The maximum allowable voltage on the VBUS pin is 3.6 V. One method is to use a voltage
divider to connect the VBUS pin to the VBUS on the USB connector.
The voltage divider ratio should be such that the VBUS pin will be greater than 0.7VDD to
indicate a logic HIGH while below the 3.6 V allowable maximum voltage.
Use the following operating conditions:
VBUSmax = 5.25 V
VDD = 3.6 V
The voltage divider would need to provide a reduction of 3.6 V/5.25 V or ~0.686 V.
Fig 29. USB interface on a bus-powered device
LPC17xx
VDD(3V3)
R1
1.5 kΩ
R2
USB_UP_LED
002aad940
USB-B
connector
USB_D+
USB_D−
VBUS
VSS
RS = 33 Ω
RS = 33 Ω
LPC1769_68_67_66_65_64_63 All information provided in this document is subject to legal disclaimers. © NXP Semiconductors N.V. 2014. All rights reserved.
Product data sheet Rev. 9.4 — 4 April 2014 70 of 88
NXP Semiconductors LPC1769/68/67/66/65/64/63
32-bit ARM Cortex-M3 microcontroller
Fig 30. USB interface on a bus-powered device where VBUS = 5 V, VDD not present
LPC17xx
VDD
R1
1.5 kΩ
R2
R3
USB-B
connector
USB_D+
USB_DUSB_
VBUS
VSS
RS = 33 Ω
RS = 33 Ω
aaa-008962
R2
USB_UP_LED
Fig 31. USB interface with soft-connect
LPC17xx
USB-B
connector
USB_D+
USB_CONNECT
SoftConnect switch
USB_D−
VBUS
VSS
VDD(3V3)
R1
1.5 kΩ
RS = 33 Ω
002aad939
RS = 33 Ω
USB_UP_LED
LPC1769_68_67_66_65_64_63 All information provided in this document is subject to legal disclaimers. © NXP Semiconductors N.V. 2014. All rights reserved.
Product data sheet Rev. 9.4 — 4 April 2014 71 of 88
NXP Semiconductors LPC1769/68/67/66/65/64/63
32-bit ARM Cortex-M3 microcontroller
Fig 32. USB OTG port configuration
USB_D+
USB_D−
USB_SDA
USB_SCL
RSTOUT
LPC17xx
Mini-AB
connector
33 Ω
33 Ω
VDD
VDD
002aad941
EINTn
RESET_N
ADR/PSW
SPEED
SUSPEND
OE_N/INT_N
SCL
SDA
INT_N
VBUS
ID
DP
DM
ISP1302
VSS
USB_UP_LED
VDD
Fig 33. USB host port configuration
USB_UP_LED
USB_D+
USB_D−
USB_PWRD
LPC17xx 15 kΩ 15 kΩ
USB-A
connector
33 Ω
33 Ω
002aad942
VDD
USB_OVRCR
USB_PPWR
LM3526-L
ENA
IN
5 V
FLAGA
OUTA
VDD
D+
D−
VBUS
VSS
LPC1769_68_67_66_65_64_63 All information provided in this document is subject to legal disclaimers. © NXP Semiconductors N.V. 2014. All rights reserved.
Product data sheet Rev. 9.4 — 4 April 2014 72 of 88
NXP Semiconductors LPC1769/68/67/66/65/64/63
32-bit ARM Cortex-M3 microcontroller
15.2 Crystal oscillator XTAL input and component selection
The input voltage to the on-chip oscillators is limited to 1.8 V. If the oscillator is driven by a
clock in slave mode, it is recommended that the input be coupled through a capacitor with
Ci = 100 pF. To limit the input voltage to the specified range, choose an additional
capacitor to ground Cg which attenuates the input voltage by a factor Ci/(Ci + Cg). In slave
mode, a minimum of 200 mV(RMS) is needed.
In slave mode the input clock signal should be coupled by means of a capacitor of 100 pF
(Figure 35), with an amplitude between 200 mV(RMS) and 1000 mV(RMS). This
corresponds to a square wave signal with a signal swing of between 280 mV and 1.4 V.
The XTALOUT pin in this configuration can be left unconnected.
External components and models used in oscillation mode are shown in Figure 36 and in
Table 23 and Table 24. Since the feedback resistance is integrated on chip, only a crystal
and the capacitances CX1 and CX2 need to be connected externally in case of
fundamental mode oscillation (the fundamental frequency is represented by L, CL and
RS). Capacitance CP in Figure 36 represents the parallel package capacitance and should
not be larger than 7 pF. Parameters FOSC, CL, RS and CP are supplied by the crystal
manufacturer.
Fig 34. USB device port configuration
LPC17xx
USB-B
connector
33 Ω
33 Ω
002aad943
USB_UP_LED
USB_CONNECT
VDD
VDD
D+
D−
USB_D+
USB_D−
VBUS VBUS
VSS
Fig 35. Slave mode operation of the on-chip oscillator
LPC1xxx
XTAL1
Ci
100 pF
Cg
002aae835
LPC1769_68_67_66_65_64_63 All information provided in this document is subject to legal disclaimers. © NXP Semiconductors N.V. 2014. All rights reserved.
Product data sheet Rev. 9.4 — 4 April 2014 73 of 88
NXP Semiconductors LPC1769/68/67/66/65/64/63
32-bit ARM Cortex-M3 microcontroller
15.3 XTAL and RTCX Printed Circuit Board (PCB) layout guidelines
The crystal should be connected on the PCB as close as possible to the oscillator input
and output pins of the chip. Take care that the load capacitors Cx1, Cx2, and Cx3 in case of
third overtone crystal usage have a common ground plane. The external components
must also be connected to the ground plain. Loops must be made as small as possible in
Fig 36. Oscillator modes and models: oscillation mode of operation and external crystal
model used for CX1/CX2 evaluation
Table 23. Recommended values for CX1/CX2 in oscillation mode (crystal and external
components parameters): low frequency mode
Fundamental oscillation
frequency FOSC
Crystal load
capacitance CL
Maximum crystal
series resistance RS
External load
capacitors CX1/CX2
1 MHz to 5 MHz 10 pF < 300 18 pF, 18 pF
20 pF < 300 39 pF, 39 pF
30 pF < 300 57 pF, 57 pF
5 MHz to 10 MHz 10 pF < 300 18 pF, 18 pF
20 pF < 200 39 pF, 39 pF
30 pF < 100 57 pF, 57 pF
10 MHz to 15 MHz 10 pF < 160 18 pF, 18 pF
20 pF < 60 39 pF, 39 pF
15 MHz to 20 MHz 10 pF < 80 18 pF, 18 pF
Table 24. Recommended values for CX1/CX2 in oscillation mode (crystal and external
components parameters): high frequency mode
Fundamental oscillation
frequency FOSC
Crystal load
capacitance CL
Maximum crystal
series resistance RS
External load
capacitors CX1, CX2
15 MHz to 20 MHz 10 pF < 180 18 pF, 18 pF
20 pF < 100 39 pF, 39 pF
20 MHz to 25 MHz 10 pF < 160 18 pF, 18 pF
20 pF < 80 39 pF, 39 pF
002aaf424
LPC1xxx
XTALIN XTALOUT
CX1 CX2
XTAL
= CL CP
RS
L
LPC1769_68_67_66_65_64_63 All information provided in this document is subject to legal disclaimers. © NXP Semiconductors N.V. 2014. All rights reserved.
Product data sheet Rev. 9.4 — 4 April 2014 74 of 88
NXP Semiconductors LPC1769/68/67/66/65/64/63
32-bit ARM Cortex-M3 microcontroller
order to keep the noise coupled in via the PCB as small as possible. Also parasitics
should stay as small as possible. Values of Cx1 and Cx2 should be chosen smaller
accordingly to the increase in parasitics of the PCB layout.
15.4 Standard I/O pin configuration
Figure 37 shows the possible pin modes for standard I/O pins with analog input function:
• Digital output driver: Open-drain mode enabled/disabled
• Digital input: Pull-up enabled/disabled
• Digital input: Pull-down enabled/disabled
• Digital input: Repeater mode enabled/disabled
• Analog input
The default configuration for standard I/O pins is input with pull-up enabled. The weak
MOS devices provide a drive capability equivalent to pull-up and pull-down resistors.
Fig 37. Standard I/O pin configuration with analog input
PIN
VDD VDD
ESD
VSS
ESD
strong
pull-up
strong
pull-down
VDD
weak
pull-up
weak
pull-down
open-drain enable
output enable
repeater mode
enable
pull-up enable
pull-down enable
data output
data input
analog input
select analog input
002aaf272
pin configured
as digital output
driver
pin configured
as digital input
pin configured
as analog input
LPC1769_68_67_66_65_64_63 All information provided in this document is subject to legal disclaimers. © NXP Semiconductors N.V. 2014. All rights reserved.
Product data sheet Rev. 9.4 — 4 April 2014 75 of 88
NXP Semiconductors LPC1769/68/67/66/65/64/63
32-bit ARM Cortex-M3 microcontroller
15.5 Reset pin configuration
Fig 38. Reset pin configuration
VSS
reset
002aaf274
VDD
VDD
VDD
Rpu ESD
ESD
20 ns RC
GLITCH FILTER
PIN
LPC1769_68_67_66_65_64_63 All information provided in this document is subject to legal disclaimers. © NXP Semiconductors N.V. 2014. All rights reserved.
Product data sheet Rev. 9.4 — 4 April 2014 76 of 88
NXP Semiconductors LPC1769/68/67/66/65/64/63
32-bit ARM Cortex-M3 microcontroller
15.6 ElectroMagnetic Compatibility (EMC)
Radiated emission measurements according to the IEC61967-2 standard using the
TEM-cell method are shown for part LPC1768.
[1] IEC levels refer to Appendix D in the IEC61967-2 Specification.
Table 25. ElectroMagnetic Compatibility (EMC) for part LPC1768 (TEM-cell method)
VDD = 3.3 V; Tamb = 25 C.
Parameter Frequency band System clock = Unit
12 MHz 24 MHz 48 MHz 72 MHz 100 MHz
Input clock: IRC (4 MHz)
maximum
peak level
150 kHz to 30 MHz 7 6 4 7 7 dBV
30 MHz to 150 MHz +1 +5 +11 +16 +9 dBV
150 MHz to 1 GHz 2 +4 +11 +12 +19 dBV
IEC level[1] - O O N M L -
Input clock: crystal oscillator (12 MHz)
maximum
peak level
150 kHz to 30 MHz 5 4 4 7 8 dBV
30 MHz to 150 MHz 1 +5 +10 +15 +7 dBV
150 MHz to 1 GHz 1 +6 +11 +10 +16 dBV
IEC level[1] - O O N M M -
LPC1769_68_67_66_65_64_63 All information provided in this document is subject to legal disclaimers. © NXP Semiconductors N.V. 2014. All rights reserved.
Product data sheet Rev. 9.4 — 4 April 2014 77 of 88
NXP Semiconductors LPC1769/68/67/66/65/64/63
32-bit ARM Cortex-M3 microcontroller
16. Package outline
Fig 39. Package outline SOT407-1 (LQFP100)
UNIT
A
max. A1 A2 A3 bp c E(1) e HE L Lp v w y Z θ
OUTLINE REFERENCES
VERSION
EUROPEAN
PROJECTION ISSUE DATE
IEC JEDEC JEITA
mm 1.6 0.15
0.05
1.45
1.35
0.25
0.27
0.17
0.20
0.09
14.1
13.9
0.5
16.25
15.75
1.15
0.85
7
0
o
1 0.2 0.08 0.08 o
DIMENSIONS (mm are the original dimensions)
Note
1. Plastic or metal protrusions of 0.25 mm maximum per side are not included.
0.75
0.45
SOT407-1 136E20 MS-026
00-02-01
03-02-20
D(1) (1) (1)
14.1
13.9
HD
16.25
15.75
Z E
1.15
0.85
D
bp
e
θ
E
A1
A
Lp
detail X
L
(A 3 )
B
25
c
HD
bp
HE A2
v M B
D
ZD
A
ZE
e
v M A
X
1
100
76
75 51
50
26
y
pin 1 index
w M
w M
0 5 10 mm
scale
LQFP100: plastic low profile quad flat package; 100 leads; body 14 x 14 x 1.4 mm SOT407-1
LPC1769_68_67_66_65_64_63 All information provided in this document is subject to legal disclaimers. © NXP Semiconductors N.V. 2014. All rights reserved.
Product data sheet Rev. 9.4 — 4 April 2014 78 of 88
NXP Semiconductors LPC1769/68/67/66/65/64/63
32-bit ARM Cortex-M3 microcontroller
Fig 40. Package outline SOT926-1 (TFBGA100)
OUTLINE REFERENCES
VERSION
EUROPEAN
PROJECTION
ISSUE DATE
IEC JEDEC JEITA
SOT926-1 - - - - - - - - -
SOT926-1
05-12-09
05-12-22
UNIT A
max
mm 1.2 0.4
0.3
0.8
0.65
0.5
0.4
9.1
8.9
9.1
8.9
A1
DIMENSIONS (mm are the original dimensions)
TFBGA100: plastic thin fine-pitch ball grid array package; 100 balls; body 9 x 9 x 0.7 mm
A2 b D E e2
7.2
e
0.8
e1
7.2
v
0.15
w
0.05
y
0.08
y1
0.1
0 2.5 5 mm
scale
b
e2
e1
e
e
1/2 e
1/2 e
∅ v M C A B
∅ w M C
ball A1
index area
A
B
C
D
E
F
H
K
G
J
1 2 3 4 5 6 7 8 9 10
ball A1
index area
B A
E
D
C
y1 C y
X
detail X
A
A1
A2
LPC1769_68_67_66_65_64_63 All information provided in this document is subject to legal disclaimers. © NXP Semiconductors N.V. 2014. All rights reserved.
Product data sheet Rev. 9.4 — 4 April 2014 79 of 88
NXP Semiconductors LPC1769/68/67/66/65/64/63
32-bit ARM Cortex-M3 microcontroller
Fig 41. Package outline LPC1768UK (WLCSP100)
Outline References
version
European
projection Issue date
IEC JEDEC JEITA
wlcsp100_lpc1768uk_po
Unit
mm
max
nom
min
0.65
0.60
0.55
0.27
0.24
0.21
0.35
0.32
0.29
5.104
5.074
5.044
5.104
5.074
5.044
4.5 4.5 0.15
A
Dimensions (mm are the original dimensions)
A1 A2
0.385
0.360
0.335
b D E
0.05
e y
0.5
e1 e2 v
0.05
w
ball A1
index area
X
detail X
C
y
A
A2
A1
ball A1
index area
LPC1768UK 11-10-19
13-11-04
WLCSP100: wafer level chip-scale package; 100 balls; 5.074 x 5.074 x 0.6 mm LPC1768UK
0
scale
3 mm
D B
E
A
1
K
J
H
G
F
E
D
C
B
A
2 3 4 5 6 7 8 9 10
e1
e b Ø v C A B
Ø w C
1/2 e
e2
e
1/2 e
LPC1769_68_67_66_65_64_63 All information provided in this document is subject to legal disclaimers. © NXP Semiconductors N.V. 2014. All rights reserved.
Product data sheet Rev. 9.4 — 4 April 2014 80 of 88
NXP Semiconductors LPC1769/68/67/66/65/64/63
32-bit ARM Cortex-M3 microcontroller
17. Soldering
Fig 42. Reflow soldering for the LQFP100 package
SOT407-1
DIMENSIONS in mm
occupied area
Footprint information for reflow soldering of LQFP100 package
Ax
Bx
Gx
Hy Gy
Hx
By Ay
P2 P1
D2 (8×) D1
(0.125)
P1 P2 Ax Ay Bx By C D1 D2 Gx Gy Hx Hy
sot407-1
solder land
C
Generic footprint pattern
Refer to the package outline drawing for actual layout
0.500 0.560 17.300 17.300 14.300 14.300 1.500 0.280 0.400 14.500 14.500 17.550 17.550
LPC1769_68_67_66_65_64_63 All information provided in this document is subject to legal disclaimers. © NXP Semiconductors N.V. 2014. All rights reserved.
Product data sheet Rev. 9.4 — 4 April 2014 81 of 88
NXP Semiconductors LPC1769/68/67/66/65/64/63
32-bit ARM Cortex-M3 microcontroller
Fig 43. Reflow soldering of the TFBGA100 package
DIMENSIONS in mm
P SL SP SR Hx Hy
Hx
Hy
SOT926-1
solder land plus solder paste
occupied area
Footprint information for reflow soldering of TFBGA100 package
solder land
solder paste deposit
solder resist
P
P
SL
SP
SR
Generic footprint pattern
Refer to the package outline drawing for actual layout
detail X
see detail X
sot926-1_fr
0.80 0.330 0.400 0.480 9.400 9.400
LPC1769_68_67_66_65_64_63 All information provided in this document is subject to legal disclaimers. © NXP Semiconductors N.V. 2014. All rights reserved.
Product data sheet Rev. 9.4 — 4 April 2014 82 of 88
NXP Semiconductors LPC1769/68/67/66/65/64/63
32-bit ARM Cortex-M3 microcontroller
18. Abbreviations
Table 26. Abbreviations
Acronym Description
ADC Analog-to-Digital Converter
AHB Advanced High-performance Bus
AMBA Advanced Microcontroller Bus Architecture
APB Advanced Peripheral Bus
BOD BrownOut Detection
CAN Controller Area Network
DAC Digital-to-Analog Converter
DMA Direct Memory Access
EOP End Of Packet
GPIO General Purpose Input/Output
IRC Internal RC
IrDA Infrared Data Association
JTAG Joint Test Action Group
MAC Media Access Control
MIIM Media Independent Interface Management
OHCI Open Host Controller Interface
OTG On-The-Go
PHY Physical Layer
PLL Phase-Locked Loop
PWM Pulse Width Modulator
RIT Repetitive Interrupt Timer
RMII Reduced Media Independent Interface
SE0 Single Ended Zero
SPI Serial Peripheral Interface
SSI Serial Synchronous Interface
SSP Synchronous Serial Port
TCM Tightly Coupled Memory
TTL Transistor-Transistor Logic
UART Universal Asynchronous Receiver/Transmitter
USB Universal Serial Bus
LPC1769_68_67_66_65_64_63 All information provided in this document is subject to legal disclaimers. © NXP Semiconductors N.V. 2014. All rights reserved.
Product data sheet Rev. 9.4 — 4 April 2014 83 of 88
NXP Semiconductors LPC1769/68/67/66/65/64/63
32-bit ARM Cortex-M3 microcontroller
19. Revision history
Table 27. Revision history
Document ID Release
date
Data sheet status Change
notice
Supersedes
LPC1769_68_67_66_65_64_63 v.9.4 20140404 Product data sheet - LPC1769_68_67_66_65_64 v.9.3
Modifications: • Added LPC1768UK.
• Table 5 “Pin description”: Changed RX_MCLK and TX_MCLK type from INPUT
to OUTPUT.
LPC1769_68_67_66_65_64_63 v.9.3 20140108 Product data sheet - LPC1769_68_67_66_65_64 v.9.2
Modifications: • Table 7 “Thermal resistance (±15 %)”:
– Added TFBGA100.
– Added 15 % to table title.
LPC1769_68_67_66_65_64_63 v.9.2 20131021 Product data sheet - LPC1769_68_67_66_65_64 v.9.1
Modifications: • Table 8 “Static characteristics”:
– Added Table note 3 “VDDA and VREFP should be tied to VDD(3V3) if the
ADC and DAC are not used.”
– Added Table note 4 “VDDA for DAC specs are from 2.7 V to 3.6 V.”
– VDDA/VREFP spec changed from 2.7 V to 2.5 V.
• Table 19 “ADC characteristics (full resolution)”:
– Added Table note 1 “VDDA and VREFP should be tied to VDD(3V3) if the
ADC and DAC are not used.”
– VDDA changed from 2.7 V to 2.5 V.
• Table 20 “ADC characteristics (lower resolution)”: Added Table note 1 “VDDA
and VREFP should be tied to VDD(3V3) if the ADC and DAC are not used.”
LPC1769_68_67_66_65_64_63 v.9.1 20130916 Product data sheet - LPC1769_68_67_66_65_64 v.9
Modifications: • Added Table 7 “Thermal resistance”.
• Table 6 “Limiting values”:
– Updated min/max values for VDD(3V3) and VDD(REG)(3V3).
– Updated conditions for VI.
– Updated table notes.
• Table 8 “Static characteristics”: Added Table note 15 “TCK/SWDCLK pin needs
to be externally pulled LOW.”
• Updated Section 15.1 “Suggested USB interface solutions”.
• Added Section 5 “Marking”.
• Changed title of Figure 31 from “USB interface on a self-powered device” to
“USB interface with soft-connect”.
LPC1769_68_67_66_65_64_63 v.9 20120810 Product data sheet - LPC1769_68_67_66_65_64 v.8
Modifications: • Remove table note “The peak current is limited to 25 times the corresponding
maximum current.” from Table 5 “Limiting values”.
• Change VDD(3V3) to VDD(REG)(3V3) in Section 11.3 “Internal oscillators”.
• Glitch filter constant changed to 10 ns in Table note 6 in Table 4.
• Description of RESET function updated in Table 4.
• Pull-up value added for GPIO pins in Table 4.
• Pin configuration diagram for LQFP100 package corrected (Figure 2).
LPC1769_68_67_66_65_64_63 v.8 20111114 Product data sheet - LPC1769_68_67_66_65_64 v.7
LPC1769_68_67_66_65_64_63 All information provided in this document is subject to legal disclaimers. © NXP Semiconductors N.V. 2014. All rights reserved.
Product data sheet Rev. 9.4 — 4 April 2014 84 of 88
NXP Semiconductors LPC1769/68/67/66/65/64/63
32-bit ARM Cortex-M3 microcontroller
Modifications: • Pin description of USB_UP_LED pin updated in Table 4.
• Ri1 and Ri2 labels in Figure 27 updated.
• Part LPC1765FET100 added.
• Table note 10 updated in Table 4.
• Table note 1 updated in Table 12.
• Pin description of STCLK pin updated in Table 4.
• Electromagnetic compatibility data added in Section 14.6.
• Section 16 added.
LPC1769_68_67_66_65_64_63 v.7 20110405 Product data sheet - LPC1769_68_67_66_65_64 v.6
Modifications: • Pin description of pins P0[29] and P0[30] updated in Table note 5 of Table 4.
Pins are not 5 V tolerant.
• Typical value for Parameter Nendu added in Table 9.
• Parameter Vhys for I2C bus pins: typical value corrected Vhys = 0.05VDD(3V3) in
Table 7.
• Condition 3.0 V VDD(3V3) 3.6 V added in Table 16.
• Typical values for parameters IDD(REG)(3V3) and IBAT with condition Deep
power-down mode corrected in Table 7 and Table note 9, Table note 10, and
Table note 11 updated.
• For Deep power-down mode, Figure 9 updated and Figure 10 added.
LPC1769_68_67_66_65_64_63 v.6 20100825 Product data sheet - LPC1769_68_67_66_65_64 v.5
Modifications: • Part LPC1768TFBGA added.
• Section 7.30.2; BOD level corrected.
• Added Section 10.2.
LPC1769_68_67_66_65_64_63 v.5 20100716 Product data sheet - LPC1769_68_67_66_65_64 v.4
LPC1769_68_67_66_65_64 v.4 20100201 Product data sheet - LPC1768_67_66_65_64 v.3
LPC1768_67_66_65_64 v.3 20091119 Product data sheet - LPC1768_66_65_64 v.2
LPC1768_66_65_64 v.2 20090211 Objective data sheet - LPC1768_66_65_64 v.1
LPC1768_66_65_64 v.1 20090115 Objective data sheet - -
Table 27. Revision history …continued
Document ID Release
date
Data sheet status Change
notice
Supersedes
LPC1769_68_67_66_65_64_63 All information provided in this document is subject to legal disclaimers. © NXP Semiconductors N.V. 2014. All rights reserved.
Product data sheet Rev. 9.4 — 4 April 2014 85 of 88
NXP Semiconductors LPC1769/68/67/66/65/64/63
32-bit ARM Cortex-M3 microcontroller
20. Legal information
20.1 Data sheet status
[1] Please consult the most recently issued document before initiating or completing a design.
[2] The term ‘short data sheet’ is explained in section “Definitions”.
[3] The product status of device(s) described in this document may have changed since this document was published and may differ in case of multiple devices. The latest product status
information is available on the Internet at URL http://www.nxp.com.
20.2 Definitions
Draft — The document is a draft version only. The content is still under
internal review and subject to formal approval, which may result in
modifications or additions. NXP Semiconductors does not give any
representations or warranties as to the accuracy or completeness of
information included herein and shall have no liability for the consequences of
use of such information.
Short data sheet — A short data sheet is an extract from a full data sheet
with the same product type number(s) and title. A short data sheet is intended
for quick reference only and should not be relied upon to contain detailed and
full information. For detailed and full information see the relevant full data
sheet, which is available on request via the local NXP Semiconductors sales
office. In case of any inconsistency or conflict with the short data sheet, the
full data sheet shall prevail.
Product specification — The information and data provided in a Product
data sheet shall define the specification of the product as agreed between
NXP Semiconductors and its customer, unless NXP Semiconductors and
customer have explicitly agreed otherwise in writing. In no event however,
shall an agreement be valid in which the NXP Semiconductors product is
deemed to offer functions and qualities beyond those described in the
Product data sheet.
20.3 Disclaimers
Limited warranty and liability — Information in this document is believed to
be accurate and reliable. However, NXP Semiconductors does not give any
representations or warranties, expressed or implied, as to the accuracy or
completeness of such information and shall have no liability for the
consequences of use of such information. NXP Semiconductors takes no
responsibility for the content in this document if provided by an information
source outside of NXP Semiconductors.
In no event shall NXP Semiconductors be liable for any indirect, incidental,
punitive, special or consequential damages (including - without limitation - lost
profits, lost savings, business interruption, costs related to the removal or
replacement of any products or rework charges) whether or not such
damages are based on tort (including negligence), warranty, breach of
contract or any other legal theory.
Notwithstanding any damages that customer might incur for any reason
whatsoever, NXP Semiconductors’ aggregate and cumulative liability towards
customer for the products described herein shall be limited in accordance
with the Terms and conditions of commercial sale of NXP Semiconductors.
Right to make changes — NXP Semiconductors reserves the right to make
changes to information published in this document, including without
limitation specifications and product descriptions, at any time and without
notice. This document supersedes and replaces all information supplied prior
to the publication hereof.
Suitability for use — NXP Semiconductors products are not designed,
authorized or warranted to be suitable for use in life support, life-critical or
safety-critical systems or equipment, nor in applications where failure or
malfunction of an NXP Semiconductors product can reasonably be expected
to result in personal injury, death or severe property or environmental
damage. NXP Semiconductors and its suppliers accept no liability for
inclusion and/or use of NXP Semiconductors products in such equipment or
applications and therefore such inclusion and/or use is at the customer’s own
risk.
Applications — Applications that are described herein for any of these
products are for illustrative purposes only. NXP Semiconductors makes no
representation or warranty that such applications will be suitable for the
specified use without further testing or modification.
Customers are responsible for the design and operation of their applications
and products using NXP Semiconductors products, and NXP Semiconductors
accepts no liability for any assistance with applications or customer product
design. It is customer’s sole responsibility to determine whether the NXP
Semiconductors product is suitable and fit for the customer’s applications and
products planned, as well as for the planned application and use of
customer’s third party customer(s). Customers should provide appropriate
design and operating safeguards to minimize the risks associated with their
applications and products.
NXP Semiconductors does not accept any liability related to any default,
damage, costs or problem which is based on any weakness or default in the
customer’s applications or products, or the application or use by customer’s
third party customer(s). Customer is responsible for doing all necessary
testing for the customer’s applications and products using NXP
Semiconductors products in order to avoid a default of the applications and
the products or of the application or use by customer’s third party
customer(s). NXP does not accept any liability in this respect.
Limiting values — Stress above one or more limiting values (as defined in
the Absolute Maximum Ratings System of IEC 60134) will cause permanent
damage to the device. Limiting values are stress ratings only and (proper)
operation of the device at these or any other conditions above those given in
the Recommended operating conditions section (if present) or the
Characteristics sections of this document is not warranted. Constant or
repeated exposure to limiting values will permanently and irreversibly affect
the quality and reliability of the device.
Terms and conditions of commercial sale — NXP Semiconductors
products are sold subject to the general terms and conditions of commercial
sale, as published at http://www.nxp.com/profile/terms, unless otherwise
agreed in a valid written individual agreement. In case an individual
agreement is concluded only the terms and conditions of the respective
agreement shall apply. NXP Semiconductors hereby expressly objects to
applying the customer’s general terms and conditions with regard to the
purchase of NXP Semiconductors products by customer.
No offer to sell or license — Nothing in this document may be interpreted or
construed as an offer to sell products that is open for acceptance or the grant,
conveyance or implication of any license under any copyrights, patents or
other industrial or intellectual property rights.
Document status[1][2] Product status[3] Definition
Objective [short] data sheet Development This document contains data from the objective specification for product development.
Preliminary [short] data sheet Qualification This document contains data from the preliminary specification.
Product [short] data sheet Production This document contains the product specification.
LPC1769_68_67_66_65_64_63 All information provided in this document is subject to legal disclaimers. © NXP Semiconductors N.V. 2014. All rights reserved.
Product data sheet Rev. 9.4 — 4 April 2014 86 of 88
NXP Semiconductors LPC1769/68/67/66/65/64/63
32-bit ARM Cortex-M3 microcontroller
Export control — This document as well as the item(s) described herein
may be subject to export control regulations. Export might require a prior
authorization from competent authorities.
Non-automotive qualified products — Unless this data sheet expressly
states that this specific NXP Semiconductors product is automotive qualified,
the product is not suitable for automotive use. It is neither qualified nor tested
in accordance with automotive testing or application requirements. NXP
Semiconductors accepts no liability for inclusion and/or use of
non-automotive qualified products in automotive equipment or applications.
In the event that customer uses the product for design-in and use in
automotive applications to automotive specifications and standards, customer
(a) shall use the product without NXP Semiconductors’ warranty of the
product for such automotive applications, use and specifications, and (b)
whenever customer uses the product for automotive applications beyond
NXP Semiconductors’ specifications such use shall be solely at customer’s
own risk, and (c) customer fully indemnifies NXP Semiconductors for any
liability, damages or failed product claims resulting from customer design and
use of the product for automotive applications beyond NXP Semiconductors’
standard warranty and NXP Semiconductors’ product specifications.
20.4 Trademarks
Notice: All referenced brands, product names, service names and trademarks
are the property of their respective owners.
I2C-bus — logo is a trademark of NXP Semiconductors N.V.
21. Contact information
For more information, please visit: http://www.nxp.com
For sales office addresses, please send an email to: salesaddresses@nxp.com
LPC1769_68_67_66_65_64_63 All information provided in this document is subject to legal disclaimers. © NXP Semiconductors N.V. 2014. All rights reserved.
Product data sheet Rev. 9.4 — 4 April 2014 87 of 88
continued >>
NXP Semiconductors LPC1769/68/67/66/65/64/63
32-bit ARM Cortex-M3 microcontroller
22. Contents
1 General description . . . . . . . . . . . . . . . . . . . . . . 1
2 Features and benefits . . . . . . . . . . . . . . . . . . . . 1
3 Applications . . . . . . . . . . . . . . . . . . . . . . . . . . . . 4
4 Ordering information. . . . . . . . . . . . . . . . . . . . . 4
4.1 Ordering options . . . . . . . . . . . . . . . . . . . . . . . . 4
5 Marking . . . . . . . . . . . . . . . . . . . . . . . . . . . . . . . . 5
6 Block diagram . . . . . . . . . . . . . . . . . . . . . . . . . . 6
7 Pinning information. . . . . . . . . . . . . . . . . . . . . . 7
7.1 Pinning . . . . . . . . . . . . . . . . . . . . . . . . . . . . . . . 7
7.2 Pin description . . . . . . . . . . . . . . . . . . . . . . . . 10
8 Functional description . . . . . . . . . . . . . . . . . . 21
8.1 Architectural overview . . . . . . . . . . . . . . . . . . 21
8.2 ARM Cortex-M3 processor . . . . . . . . . . . . . . . 21
8.3 On-chip flash program memory . . . . . . . . . . . 21
8.4 On-chip SRAM . . . . . . . . . . . . . . . . . . . . . . . . 21
8.5 Memory Protection Unit (MPU). . . . . . . . . . . . 21
8.6 Memory map. . . . . . . . . . . . . . . . . . . . . . . . . . 22
8.7 Nested Vectored Interrupt Controller
(NVIC). . . . . . . . . . . . . . . . . . . . . . . . . . . . . . . 24
8.7.1 Features . . . . . . . . . . . . . . . . . . . . . . . . . . . . . 24
8.7.2 Interrupt sources. . . . . . . . . . . . . . . . . . . . . . . 24
8.8 Pin connect block . . . . . . . . . . . . . . . . . . . . . . 24
8.9 General purpose DMA controller . . . . . . . . . . 24
8.9.1 Features . . . . . . . . . . . . . . . . . . . . . . . . . . . . . 25
8.10 Fast general purpose parallel I/O . . . . . . . . . . 25
8.10.1 Features . . . . . . . . . . . . . . . . . . . . . . . . . . . . . 26
8.11 Ethernet . . . . . . . . . . . . . . . . . . . . . . . . . . . . . 26
8.11.1 Features . . . . . . . . . . . . . . . . . . . . . . . . . . . . . 26
8.12 USB interface . . . . . . . . . . . . . . . . . . . . . . . . 27
8.12.1 USB device controller . . . . . . . . . . . . . . . . . . . 27
8.12.1.1 Features . . . . . . . . . . . . . . . . . . . . . . . . . . . . . 27
8.12.2 USB host controller . . . . . . . . . . . . . . . . . . . . 28
8.12.2.1 Features . . . . . . . . . . . . . . . . . . . . . . . . . . . . . 28
8.12.3 USB OTG controller . . . . . . . . . . . . . . . . . . . . 28
8.12.3.1 Features . . . . . . . . . . . . . . . . . . . . . . . . . . . . . 28
8.13 CAN controller and acceptance filters . . . . . . 28
8.13.1 Features . . . . . . . . . . . . . . . . . . . . . . . . . . . . . 29
8.14 12-bit ADC . . . . . . . . . . . . . . . . . . . . . . . . . . . 29
8.14.1 Features . . . . . . . . . . . . . . . . . . . . . . . . . . . . . 29
8.15 10-bit DAC . . . . . . . . . . . . . . . . . . . . . . . . . . . 29
8.15.1 Features . . . . . . . . . . . . . . . . . . . . . . . . . . . . . 29
8.16 UARTs. . . . . . . . . . . . . . . . . . . . . . . . . . . . . . . 30
8.16.1 Features . . . . . . . . . . . . . . . . . . . . . . . . . . . . . 30
8.17 SPI serial I/O controller. . . . . . . . . . . . . . . . . . 30
8.17.1 Features . . . . . . . . . . . . . . . . . . . . . . . . . . . . . 30
8.18 SSP serial I/O controller . . . . . . . . . . . . . . . . . 30
8.18.1 Features. . . . . . . . . . . . . . . . . . . . . . . . . . . . . 31
8.19 I2C-bus serial I/O controllers . . . . . . . . . . . . . 31
8.19.1 Features. . . . . . . . . . . . . . . . . . . . . . . . . . . . . 31
8.20 I2S-bus serial I/O controllers . . . . . . . . . . . . . 32
8.20.1 Features. . . . . . . . . . . . . . . . . . . . . . . . . . . . . 32
8.21 General purpose 32-bit timers/external
event counters . . . . . . . . . . . . . . . . . . . . . . . . 32
8.21.1 Features. . . . . . . . . . . . . . . . . . . . . . . . . . . . . 32
8.22 Pulse width modulator . . . . . . . . . . . . . . . . . . 33
8.22.1 Features. . . . . . . . . . . . . . . . . . . . . . . . . . . . . 33
8.23 Motor control PWM . . . . . . . . . . . . . . . . . . . . 34
8.24 Quadrature Encoder Interface
(QEI) . . . . . . . . . . . . . . . . . . . . . . . . . . . . . . . 34
8.24.1 Features. . . . . . . . . . . . . . . . . . . . . . . . . . . . . 34
8.25 Repetitive Interrupt (RI) timer. . . . . . . . . . . . . 35
8.25.1 Features. . . . . . . . . . . . . . . . . . . . . . . . . . . . . 35
8.26 ARM Cortex-M3 system tick timer . . . . . . . . . 35
8.27 Watchdog timer . . . . . . . . . . . . . . . . . . . . . . . 35
8.27.1 Features. . . . . . . . . . . . . . . . . . . . . . . . . . . . . 35
8.28 RTC and backup registers . . . . . . . . . . . . . . . 36
8.28.1 Features. . . . . . . . . . . . . . . . . . . . . . . . . . . . . 36
8.29 Clocking and power control . . . . . . . . . . . . . . 36
8.29.1 Crystal oscillators. . . . . . . . . . . . . . . . . . . . . . 36
8.29.1.1 Internal RC oscillator . . . . . . . . . . . . . . . . . . . 37
8.29.1.2 Main oscillator . . . . . . . . . . . . . . . . . . . . . . . . 37
8.29.1.3 RTC oscillator . . . . . . . . . . . . . . . . . . . . . . . . 37
8.29.2 Main PLL (PLL0) . . . . . . . . . . . . . . . . . . . . . . 38
8.29.3 USB PLL (PLL1) . . . . . . . . . . . . . . . . . . . . . . 38
8.29.4 RTC clock output . . . . . . . . . . . . . . . . . . . . . . 38
8.29.5 Wake-up timer . . . . . . . . . . . . . . . . . . . . . . . . 38
8.29.6 Power control . . . . . . . . . . . . . . . . . . . . . . . . . 39
8.29.6.1 Sleep mode . . . . . . . . . . . . . . . . . . . . . . . . . . 39
8.29.6.2 Deep-sleep mode. . . . . . . . . . . . . . . . . . . . . . 39
8.29.6.3 Power-down mode. . . . . . . . . . . . . . . . . . . . . 40
8.29.6.4 Deep power-down mode . . . . . . . . . . . . . . . . 40
8.29.6.5 Wake-up interrupt controller . . . . . . . . . . . . . 40
8.29.7 Peripheral power control . . . . . . . . . . . . . . . . 40
8.29.8 Power domains . . . . . . . . . . . . . . . . . . . . . . . 41
8.30 System control . . . . . . . . . . . . . . . . . . . . . . . . 42
8.30.1 Reset . . . . . . . . . . . . . . . . . . . . . . . . . . . . . . . 42
8.30.2 Brownout detection . . . . . . . . . . . . . . . . . . . . 43
8.30.3 Code security
(Code Read Protection - CRP) . . . . . . . . . . . 43
8.30.4 APB interface . . . . . . . . . . . . . . . . . . . . . . . . . 43
8.30.5 AHB multilayer matrix . . . . . . . . . . . . . . . . . . 44
8.30.6 External interrupt inputs . . . . . . . . . . . . . . . . . 44
8.30.7 Memory mapping control . . . . . . . . . . . . . . . . 44
8.31 Emulation and debugging . . . . . . . . . . . . . . . 44
NXP Semiconductors LPC1769/68/67/66/65/64/63
32-bit ARM Cortex-M3 microcontroller
© NXP Semiconductors N.V. 2014. All rights reserved.
For more information, please visit: http://www.nxp.com
For sales office addresses, please send an email to: salesaddresses@nxp.com
Date of release: 4 April 2014
Document identifier: LPC1769_68_67_66_65_64_63
Please be aware that important notices concerning this document and the product(s)
described herein, have been included in section ‘Legal information’.
9 Limiting values. . . . . . . . . . . . . . . . . . . . . . . . . 45
10 Thermal characteristics . . . . . . . . . . . . . . . . . 46
11 Static characteristics. . . . . . . . . . . . . . . . . . . . 47
11.1 Power consumption . . . . . . . . . . . . . . . . . . . . 50
11.2 Peripheral power consumption . . . . . . . . . . . . 53
11.3 Electrical pin characteristics . . . . . . . . . . . . . . 54
12 Dynamic characteristics . . . . . . . . . . . . . . . . . 56
12.1 Flash memory. . . . . . . . . . . . . . . . . . . . . . . . . 56
12.2 External clock . . . . . . . . . . . . . . . . . . . . . . . . . 56
12.3 Internal oscillators. . . . . . . . . . . . . . . . . . . . . . 57
12.4 I/O pins . . . . . . . . . . . . . . . . . . . . . . . . . . . . . . 57
12.5 I2C-bus . . . . . . . . . . . . . . . . . . . . . . . . . . . . . . 58
12.6 I2S-bus interface . . . . . . . . . . . . . . . . . . . . . . 59
12.7 SSP interface . . . . . . . . . . . . . . . . . . . . . . . . . 61
12.8 USB interface . . . . . . . . . . . . . . . . . . . . . . . . 62
12.9 SPI . . . . . . . . . . . . . . . . . . . . . . . . . . . . . . . . . 63
13 ADC electrical characteristics . . . . . . . . . . . . 65
14 DAC electrical characteristics . . . . . . . . . . . . 68
15 Application information. . . . . . . . . . . . . . . . . . 69
15.1 Suggested USB interface solutions . . . . . . . . 69
15.2 Crystal oscillator XTAL input and component
selection . . . . . . . . . . . . . . . . . . . . . . . . . . . . . 72
15.3 XTAL and RTCX Printed Circuit Board
(PCB) layout guidelines . . . . . . . . . . . . . . . . . 73
15.4 Standard I/O pin configuration . . . . . . . . . . . . 74
15.5 Reset pin configuration. . . . . . . . . . . . . . . . . . 75
15.6 ElectroMagnetic Compatibility (EMC) . . . . . . . 76
16 Package outline . . . . . . . . . . . . . . . . . . . . . . . . 77
17 Soldering . . . . . . . . . . . . . . . . . . . . . . . . . . . . . 80
18 Abbreviations. . . . . . . . . . . . . . . . . . . . . . . . . . 82
19 Revision history. . . . . . . . . . . . . . . . . . . . . . . . 83
20 Legal information. . . . . . . . . . . . . . . . . . . . . . . 85
20.1 Data sheet status . . . . . . . . . . . . . . . . . . . . . . 85
20.2 Definitions. . . . . . . . . . . . . . . . . . . . . . . . . . . . 85
20.3 Disclaimers . . . . . . . . . . . . . . . . . . . . . . . . . . . 85
20.4 Trademarks. . . . . . . . . . . . . . . . . . . . . . . . . . . 86
21 Contact information. . . . . . . . . . . . . . . . . . . . . 86
22 Contents . . . . . . . . . . . . . . . . . . . . . . . . . . . . . . 87
1. General description
The PCF8574/74A provides general-purpose remote I/O expansion via the two-wire
bidirectional I2C-bus (serial clock (SCL), serial data (SDA)).
The devices consist of eight quasi-bidirectional ports, 100 kHz I2C-bus interface, three
hardware address inputs and interrupt output operating between 2.5 V and 6 V. The
quasi-bidirectional port can be independently assigned as an input to monitor interrupt
status or keypads, or as an output to activate indicator devices such as LEDs. System
master can read from the input port or write to the output port through a single register.
The low current consumption of 2.5 A (typical, static) is great for mobile applications and
the latched output ports directly drive LEDs.
The PCF8574 and PCF8574A are identical, except for the different fixed portion of the
slave address. The three hardware address pins allow eight of each device to be on the
same I2C-bus, so there can be up to 16 of these I/O expanders PCF8574/74A together on
the same I2C-bus, supporting up to 128 I/Os (for example, 128 LEDs).
The active LOW open-drain interrupt output (INT) can be connected to the interrupt logic
of the microcontroller and is activated when any input state differs from its corresponding
input port register state. It is used to indicate to the microcontroller that an input state has
changed and the device needs to be interrogated without the microcontroller continuously
polling the input register via the I2C-bus.
The internal Power-On Reset (POR) initializes the I/Os as inputs with a weak internal
pull-up 100 A current source.
2. Features and benefits
I2C-bus to parallel port expander
100 kHz I2C-bus interface (Standard-mode I2C-bus)
Operating supply voltage 2.5 V to 6 V with non-overvoltage tolerant I/O held to VDD
with 100 A current source
8-bit remote I/O pins that default to inputs at power-up
Latched outputs directly drive LEDs
Total package sink capability of 80 mA
Active LOW open-drain interrupt output
Eight programmable slave addresses using three address pins
Low standby current (2.5 A typical)
40 C to +85 C operation
ESD protection exceeds 2000 V HBM per JESD22-A114 and 1000 V CDM per
JESD22-C101
PCF8574; PCF8574A
Remote 8-bit I/O expander for I2C-bus with interrupt
Rev. 5 — 27 May 2013 Product data sheet
PCF8574_PCF8574A All information provided in this document is subject to legal disclaimers. © NXP B.V. 2013. All rights reserved.
Product data sheet Rev. 5 — 27 May 2013 2 of 33
NXP Semiconductors PCF8574; PCF8574A
Remote 8-bit I/O expander for I2C-bus with interrupt
Latch-up testing is done to JEDEC standard JESD78 which exceeds 100 mA
Packages offered: DIP16, SO16, SSOP20
3. Applications
LED signs and displays
Servers
Key pads
Industrial control
Medical equipment
PLC
Cellular telephones
Mobile devices
Gaming machines
Instrumentation and test measurement
4. Ordering information
4.1 Ordering options
Table 1. Ordering information
Type number Topside mark Package
Name Description Version
PCF8574P PCF8574P DIP16 plastic dual in-line package; 16 leads (300 mil) SOT38-4
PCF8574AP PCF8574AP
PCF8574T/3 PCF8574T SO16 plastic small outline package; 16 leads; body width 7.5 mm SOT162-1
PCF8574AT/3 PCF8574AT
PCF8574TS/3 8574TS SSOP20 plastic shrink small outline package; 20 leads;
body width 4.4 mm
SOT266-1
PCF8574ATS/3 8574A
Table 2. Ordering options
Type number Orderable
part number
Package Packing method Minimum
order
quantity
Temperature range
PCF8574P PCF8574P,112 DIP16 Standard marking
* IC’s tube - DSC bulk pack
1000 Tamb = 40 C to +85 C
PCF8574AP PCF8574AP,112 DIP16 Standard marking
* IC’s tube - DSC bulk pack
1000 Tamb = 40 C to +85 C
PCF8574T/3 PCF8574T/3,512 SO16 Standard marking
* tube dry pack
1920 Tamb = 40 C to +85 C
PCF8574T/3,518 SO16 Reel 13” Q1/T1
*standard mark SMD dry pack
1000 Tamb = 40 C to +85 C
PCF8574AT/3 PCF8574AT/3,512 SO16 Standard marking
* tube dry pack
1920 Tamb = 40 C to +85 C
PCF8574AT/3,518 SO16 Reel 13” Q1/T1
*standard mark SMD dry pack
1000 Tamb = 40 C to +85 C
PCF8574_PCF8574A All information provided in this document is subject to legal disclaimers. © NXP B.V. 2013. All rights reserved.
Product data sheet Rev. 5 — 27 May 2013 3 of 33
NXP Semiconductors PCF8574; PCF8574A
Remote 8-bit I/O expander for I2C-bus with interrupt
5. Block diagram
PCF8574TS/3 PCF8574TS/3,112 SSOP20 Standard marking
* IC’s tube - DSC bulk pack
1350 Tamb = 40 C to +85 C
PCF8574TS/3,118 SSOP20 Reel 13” Q1/T1
*standard mark SMD
2500 Tamb = 40 C to +85 C
PCF8574ATS/3 PCF8574ATS/3,118 SSOP20 Reel 13” Q1/T1
*standard mark SMD
2500 Tamb = 40 C to +85 C
Table 2. Ordering options …continued
Type number Orderable
part number
Package Packing method Minimum
order
quantity
Temperature range
Fig 1. Block diagram
Fig 2. Simplified schematic diagram of P0 to P7
002aad624
INT
I2C-BUS
CONTROL
LP FILTER
PCF8574
PCF8574A
INTERRUPT
LOGIC
A0
A1
A2
INPUT
FILTER
SHIFT
REGISTER
SDA
SCL 8 bits
write pulse
read pulse
POWER-ON
VDD RESET
VSS
I/O
PORT
P0
P1
P2
P3
P4
P5
P6
P7
002aac109
write pulse
read pulse
D
CI
S
FF
Q
power-on reset
data from Shift Register
Itrt(pu)
100 μA
IOH
IOL
VDD
P0 to P7
VSS
D
CI
S
FF
Q
data to Shift Register to interrupt logic
PCF8574_PCF8574A All information provided in this document is subject to legal disclaimers. © NXP B.V. 2013. All rights reserved.
Product data sheet Rev. 5 — 27 May 2013 4 of 33
NXP Semiconductors PCF8574; PCF8574A
Remote 8-bit I/O expander for I2C-bus with interrupt
6. Pinning information
6.1 Pinning
6.2 Pin description
Fig 3. Pin configuration for DIP16 Fig 4. Pin configuration for SO16 Fig 5. Pin configuration for
SSOP20
PCF8574P
PCF8574AP
A0 VDD
A1 SDA
A2 SCL
P0 INT
P1 P7
P2 P6
P3 P5
VSS P4
002aad625
1
2
3
4
5
6
7
8
10
9
12
11
14
13
16
15
A0 VDD
A1 SDA
A2 SCL
P0 INT
P1 P7
P2 P6
P3 P5
VSS P4
PCF8574T/3
PCF8574AT/3
002aad626
1
2
3
4
5
6
7
8
10
9
12
11
14
13
16
15
PCF8574TS/3
PCF8574ATS/3
P7
SCL P6
n.c. n.c.
SDA P5
P4
A0
A1 P3
n.c. n.c.
A2 P2
P0 P1
002aad627
1
2
3
4
5
6
7
8
9
10
12
11
14
13
16
15
18
17
20
19
VDD
INT
VSS